Abstract
Purpose
High sodium intake is an accepted risk factor for hypertension, while low Na+ intake has also been associated with increased risk of cardiovascular events. In this cross-sectional study, we examined the association of 24-h urinary Na+ excretion with haemodynamics and volume status.
Materials and methods
Haemodynamics were recorded in 510 normotensive and never-treated hypertensive subjects using whole-body impedance cardiography and tonometric radial artery pulse wave analysis. The results were examined in sex-specific tertiles of 24-h Na+ excretion, and comparisons between normotensive and hypertensive participants were also performed. Regression analysis was used to investigate factors associated with volume status. The findings were additionally compared to 28 patients with primary aldosteronism.
Results
The mean values of 24-h urinary Na+ excretion in tertiles of the 510 participants were 94, 148 and 218 mmol, respectively. Average tertile age (43.4–44.7 years), office blood pressure and pulse wave velocity were corresponding in the tertiles. Plasma electrolytes, lipids, vitamin D metabolites, parathyroid hormone, renin activity, aldosterone, creatinine and insulin sensitivity did not differ in the tertiles. In supine laboratory recordings, there were no differences in aortic systolic and diastolic blood pressure, heart rate, cardiac output and systemic vascular resistance. Extracellular water volume was higher in the highest versus lowest tertile of Na+ excretion. In regression analysis, body surface area and 24-h Na+ excretion were independent explanatory variables for extracellular water volume. No differences in urine Na+ excretion and extracellular water volume were found between normotensive and hypertensive participants. When compared with the 510 participants, patients with primary aldosteronism had 6.0% excess in extracellular water (p = .003), and 24-h Na+ excretion was not related with extracellular water volume.
Conclusion
In the absence of mineralocorticoid excess, Na+ intake, as evaluated from 24-h Na+ excretion, predominantly influences extracellular water volume without a clear effect on blood pressure.
Plain language summary
We evaluated sodium intake in 510 subjects by measuring their 24-h sodium excretion to the urine and examined whether sodium intake was related with alterations in cardiovascular function and fluid balance. All participants were without blood pressure lowering medications.
Blood pressure was recorded by a device that senses the radial artery pulsations form the wrist. The amount of blood pumped by the heart, the transfer of pressure waves following cardiac contractions and body fluid status were evaluated using bioimpedance, a method recording changes in body electrical resistance.
For the analyses, the participants were divided into tertiles according to their 24-h sodium excretions. We also compared results between normotensive and hypertensive subjects.
The 24-h sodium excretion in the tertiles corresponded to about 6 g, 9 g and 13 g of salt intake per day, respectively. There were no differences between the tertiles in age, routine laboratory analyses, blood pressure, large arterial stiffness, amount blood pumped by the heart and resistance to blood flow in the arteries. However, there was more extracellular fluid in the highest versus the lowest tertile of sodium excretion. Further statistics indicated that extracellular fluid volume in the body was mainly determined by body size, but it was also moderately influenced by sodium intake.
No differences in 24-h sodium excretion and extracellular water volume were found between normotensive and hypertensive participants.
In subjects not using blood pressure lowering medications, sodium intake predominantly influences the amount of extracellular fluid without a clear effect on blood pressure.
Introduction
Globally, high prevalence of hypertension has detrimental consequences by predisposing to stroke, myocardial infarction and other forms of cardiovascular disease [Citation1]. Even modest elevations in blood pressure (BP) are known to increase the risk of cardiovascular disease [Citation2,Citation3]. In up to 90% of cases, high BP is caused by primary hypertension, the underlying causes of which are not fully understood [Citation1]. The treatment goals for hypertension are not properly attained in any part of the world, and especially the non-pharmacological treatments are often inadequately utilised [Citation4].
Excess dietary Na+ intake is an acknowledged risk factor for hypertension [Citation1,Citation4]. A close to linear relationship between dietary Na+ intake and BP level was identified in a recent meta-analysis [Citation5]. The World Health Organisation (WHO) recommends that as a preventive measure against cardiovascular disease we should consume less than 5 g of salt (∼2.0 g of Na+) per day [Citation6,Citation7]. The European Society of Hypertension (ESH) guidelines recommend that Na+ intake should be limited to 2.0 g per day in all hypertensive patients [Citation1]. As the daily Na+ intakes vary enormously between individuals of different sizes, the consumption of Na+ should be analysed and reported in values related to body size [Citation8].
However, contradictory findings about Na+ intake and cardiovascular risk have also been published. In a pooled analysis of 133,118 individuals, high Na+ intake was related to increased risk of cardiovascular events and death in hypertensive populations, but low Na+ intake was also associated with increased risk of cardiovascular events and death in hypertensive and normotensive subjects [Citation9]. In the Prospective Urban Rural Epidemiology study comprising 95,767 participants, Na+ intake was associated with cardiovascular disease and strokes only in communities where the mean intake exceeded 5 g/day [Citation10]. In 2,807 adult patients with type 1 diabetes, urinary Na+ excretion was nonlinearly associated with all-cause mortality, so that individuals with either highest or lowest urinary Na+ excretion had reduced survival [Citation11]. Recently, strict Na+ intake restriction was even reported to impair prognosis of patients with heart failure with preserved ejection fraction [Citation12].
The amount of urinary Na+ is often thought to reflect the amount of ingested Na+ [Citation13]. However, the true evaluation of Na+ ingestion by urinary analyses would require several consecutive collections of 24-h urine Na+ excretion [Citation14], which has not been feasible in most hypertension studies. Moreover, our understanding of Na+ homeostasis has been reshaped by the notion of water-free tissue Na+ storage [Citation15]. Excess Na+ excretion into urine predisposes to renal water loss, and to excrete Na+ the body makes a great effort to conserve water and protect the body from dehydration [Citation16]. The proposed ability of tissues to buffer the effects of variations in Na+ intake on BP and cardiovascular function is a field of active research [Citation14,Citation16,Citation17].
In this cross-sectional study we tested the hypothesis that 24-h urinary Na+ excretion is related with BP, cardiac output, systemic vascular resistance (SVR), or extracellular water volume in 510 subjects naïve to hypertensive medications and in 28 patients with primary aldosteronism. Cardiovascular function and extracellular water volume were examined using pulse wave analysis and whole-body impedance cardiography. The present results suggest that 24-h urinary Na+ excretion influences extracellular water volume in the absence of an effect on BP.
Subjects and methods
Study population
The present study is part of a project focussing on haemodynamics in primary and secondary hypertension (DYNAMIC-study), and data for the present project was extracted from altogether 1350 participants, among whom 565 had BP within the normal range, 533 had the diagnosis of primary hypertension and 252 had the diagnosis of secondary hypertension. The study was performed according to the principles of the Declaration of Helsinki and was approved by the ethics committee of the Tampere University Hospital (code R06086M) and the Finnish Medicines Agency (Eudra-CT number 2006-002065-39) and was registered in a database of clinical trials (code NCT01742702).
The present study comprised 510 normotensive and hypertensive subjects without antihypertensive medications, aged 19–72 years, and 28 patients with primary aldosteronism, aged 34–70 years, all of whom had available data of 24-h Na+ and K+ excretion. The normotensive and untreated hypertensive participants were recruited via newspaper announcements and notices in Tampere University Hospital, University of Tampere, Varala Sports Institute and local occupational health care providers [Citation18–20]. All participants were interviewed and examined by a licenced physician, and they gave signed informed consent. Use of medications was documented, and quantity of alcohol consumption was evaluated as standard drinks (∼12 g of absolute alcohol) per week. Smoking history was recorded, and cigarette consumption estimated as pack-years [Citation18–20]. Seated office BP was measured with a manual sphygmomanometer (Heine Gamma G7, Herrsching, Germany), and phase I and V Korotkoff sounds were used to identify systolic and diastolic BP [Citation1].
The 510 subjects (263 men and 247 women, age range 19–72 years) were selected as follows: (1) no antihypertensives and other medications with direct influences on BP, (2) available data on 24-h Na+ and K+ excretion, (3) no history of cardiovascular or cerebrovascular disease, (4) sinus rhythm, (5) no diabetes mellitus, (6) no evidence of chronic kidney disease, (7) no secondary hypertension [Citation1], (8) no psychiatric illness other than mild depression or anxiety and (9) no history of alcohol or substance abuse. Based on seated office BP measurements, 231 (45.3%) of the participants were normotensive (90 men and 141 women), and 279 (54.7%) were hypertensive (157 men, 122 women of whom 82 were post-menopausal; 21 women and 36 men had isolated diastolic hypertension; 21 women and 28 men had isolated systolic hypertension; cut-off level 140/90 mmHg) [Citation1]. In total 203 (39.8%) of the participants used medications. Sixty-four women used hormones for contraception or hormone replacement, 23 had a hormone-releasing intrauterine device. The other medications were: vitamin-D supplement (n = 75), antidepressant (n = 24), statin (n = 13), thyroxine (n = 12), antihistamine (n = 11), inhaled glucocorticoid (n = 11), low-dose acetylsalicylic-acid (n = 5), inhaled beta2-agonist (n = 3), allopurinol (n = 2), antiepileptic (n = 1), azathioprine (n = 1), benzodiazepine (n = 1), cholestyramine (n = 1), etoricoxib (n = 1), ezetimibe (n = 1), mesalazine (n = 1), pregabalin (n = 1) and tamoxifen (n = 1).
The 28 patients with primary aldosteronism were referred to Tampere University Hospital for the diagnostics of secondary hypertension: 10 women and 18 men, mean (standard deviation, SD) age 56.0 (10.6) years, height 172.3 (9.5) cm, weight 92.1 (21.9) kg and BMI 30.7 (4.9). On average they used 3.3 (1.9) antihypertensive medications (range 0–8): calcium channel blocker (22), beta blocker (16), prazosin (12), angiotensin receptor blocker (11), angiotensin converting enzyme inhibitor (7), moxonidine (5), thiazide (4), furosemide (3), spironolactone (2), minoxidil (1). Their other medications were: potassium supplement (16), statin (9), low dose of acetylsalicylic acid (7), vitamin D supplement (6), insulin (3), proton pump inhibitor (3), thyroxine (3), antidepressant (2), 5-alpha-reductase inhibitor plus alpha-blocker (2), antirheumatic (2), incretin mimetic (2), metformin (2), opioids (2), asthma medication (1), vitamin B12 injection (1), antihistamine (1), gabapentin (1), sodium-glucose cotransporter-2 inhibitor (1) and benzodiazepine (1).
Laboratory analyses
The participants collected 24-h urine, and blood and urine samples were taken after about 12 h of fasting. Plasma and urine electrolytes, and plasma cystatin C, creatinine, C-reactive protein (CRP), glucose, uric acid and lipid determinations were performed using Cobas Integra 700/800 (F. Hoffmann-LaRoche Ltd., Basel, Switzerland) or Cobas 6000, module c501 (Roche Diagnostics, Basel, Switzerland). Plasma calcidiol (25(OH)D3) and calcitriol (1,25(OH)2D3) were analysed using enzyme immunoassay (Immunodiagnostic Systems, Boldon, UK) and parathyroid hormone (PTH) and insulin using electrochemiluminescence immunoassay (Cobas e411, Roche Diagnostics). Quantitative insulin sensitivity check index (QUICKI) was calculated from fasting plasma insulin and glucose [Citation21]. Blood cell counts were analysed using ADVIA 120 or 2120 (Bayer Health Care, Tarrytown, NY, USA). Kidney disease exclusion was by estimated GFR (CKD-EPI cystatin C formula [Citation22]) and by refractometric dipstick analysis and nephelometric analysis of urine albumin (Clinitec Atlas or Advantus and BN Prospec, respectively, Siemens Healthcare GmbH, Erlangen, Germany).
Plasma renin activity was measured using GammaCoat Plasma Renin Activity assay (Diasorin S.p.A., Saluggia, Italy), while due to a change in routine clinical analysis methods direct plasma renin concentration was determined in six aldosteronism patients according to the instructions of the assay manufacturer (LIAISON immunoanalyser, DiaSorin, Saluggia, Italy) [Citation23]. For aldosterone analyses in the 510 participants without antihypertensive medications active aldosterone radioimmunoassay (RIA) (Diagnostics Systems Laboratories, Beckman Coulter, Webster, Texas, USA) was used (intra-assay and inter-assay coefficients of variation (CV) for plasma samples 4.5% and 9.8%, respectively, detection limit 7.6 pg/ml, recovery not significantly different from 100%). In the aldosteronism patients plasma and urine aldosterone concentrations were analysed using liquid chromatography–mass spectrometry (API 4000 LC–MS/MS System, AB Sciex PTE Ltd, Singapore) which allows a high degree of specificity in complex biological samples (CV 2.8–5.1% for plasma and 4.5–8.6% for urine, limit of quantitation 40 pmol/l for plasma and 6.65 nmol/l for urine, recovery not significantly different from 100%) [Citation24]. All above laboratory analyses were carried out in SFS-EN ISO 15189:2013 accredited clinical laboratories with ISO 9001 quality management system and assay validation procedures.
Plasma N-terminal pro-atrial natriuretic peptide (NT-proANP) and N-terminal pro-B-type natriuretic peptide (NT-proBNP) were determined by Tampere University Immunopharmacology Research Group using enzyme-linked immunosorbent assays (NT-ProANP DuoSet ELISA, R&D Systems Europe Ltd, Abingdon, United Kingdom; NT-proBNP ELISA, Abcam, Cambridge, UK) with interassay coefficients of variation of 7.2% and 7.5%, respectively.
Haemodynamic measurements
Haemodynamics were recorded by research nurses in a quiet laboratory. In the DYNAMIC study, the participants are instructed to refrain from caffeine-containing products, smoking and heavy meals for ≥4 h and alcohol use for ≥24 h before the recordings [Citation18–20,Citation25,Citation26]. After 10 min of supine rest, BP was measured twice using a manual sphygmomanometer (Heine Gamma G7). Then supine haemodynamic data was collected for 5 min. The mean haemodynamic values during each minute were used in the analyses.
Left radial pulse waves were recorded using a tonometric sensor (Colin BP-508T, Colin Medical Instruments Corp., USA) fixed on the radial pulsation as described earlier [Citation18–20,Citation25,Citation26]. The tonometric measurement was calibrated twice during the 5-min recording by contralateral oscillometric brachial BP measurements. Aortic BP and pulse wave forms were derived from the tonometric signal of the Colin apparatus with the SphygmoCorR software (SphygmoCorR PWMx, AtCor Medical, Australia) [Citation27].
Whole-body impedance cardiography (CircMonR device, JR Medical Ltd., Estonia) was used to record heart rate, stroke volume, cardiac output, aortic-to-popliteal pulse wave velocity (PWV), extracellular water (ECW) volume and ECW balance as previously described [Citation28–30]. ECW volume was calculated using the formula ECW = k × (height2/Z). The coefficient k (Ω × cm) comes from blood resistivity and the relation between the distance of voltage electrodes and body height, while Z is the recorded impedance of the body. The ECW balance was calculated as ECW/ECWpredicted, the latter being assessed with the formula 2.4 × (0.0236 × Height0.725 × Weight0.423 – 1.229) for males and 2.6 × (0.0248 × H0.725 * W0.423 – 1.9549) for females [Citation31–33]. The ECW balance correlates highly with ECW:body surface area (BSA) ratio (in the present study population Pearson r = 0.881) and reflects the body size-related amount of ECW. SVR was evaluated from the tonometric BP and cardiac output so that normal central venous pressure (4 mmHg) was subtracted from mean arterial pressure and the value was divided by cardiac output [Citation19,Citation20,Citation26,Citation30].
The present ECW volume method correlates well with 51Cr-EDTA dilution-measured ECW volume (r = 0.74, bias 0.2 ± 1.1 litres) and with perioperative weight changes in patients [Citation28]. The cardiac output values measured using CircmonR correlate well with values measured using thermodilution and direct oxygen Fick methods [Citation30]. The whole-body impedance cardiography somewhat overestimates PWV, and a validated equation (PWV = PWVimpedance × 0.696 + 0.864) was used to calculate values corresponding to Doppler ultrasound and applanation tonometry methods [Citation29]. The repeatability and reproducibility of the measurements has been previously reported [Citation18,Citation19,Citation29].
Statistical analyses
The subjects were divided into sex-specific tertiles according to 24-h Na+ excretion to the urine. BSA was estimated using the formula 0.007184 × Height0.725 × Weight0.425 [Citation34]. One-way analysis of variance (ANOVA) and Kruskal–Wallis tests were applied to examine differences in the clinical characteristics and laboratory values, Levene’s test was used to examine homogeneity of variances, and Spearman correlations (rS) were calculated. The haemodynamic variables in the tertiles of 24-h Na+ excretion were compared using ANOVA for repeated measurements. The results were adjusted for BSA, as the intake of food and thus of the intake of Na+ depends on the bodily size of the individuals. The analyses of PWV were additionally adjusted for mean aortic pressure according to the recommendations [Citation35,Citation36]. The analyses also included comparisons between hypertensive and normotensive subjects, classified according to their seated office BPs [Citation1].
Variables for linear regression analysis with stepwise elimination were selected by utilising Spearman’s nonparametric correlation analysis for ECW, and p < .05 was used as the inclusion criterion. The regression model for the explanatory factors for ECW included age, sex, BSA, smoking status, alcohol consumption (standard doses per week); fasting plasma concentrations of glucose, insulin, K+, Na+, uric acid, calcium, phosphate, renin activity and aldosterone; 24-h Na+ excretion to the urine, nocturnal urine albumin excretion, estimated glomerular filtration rate, SVR and cardiac output. Smoking status was categorised using two discrete variables that enabled classification to current, previous, or never smokers. SPSS version 26.0 for Windows software (IBM SPSS, Armonk, NY, USA) was used for statistics. The Bonferroni correction was applied in all post-hoc analyses. The results are presented as mean and SD or median [25th–75th percentile], and p < .05 was considered statistically significant.
Results
Findings in 510 participants without antihypertensive medications
Among the 510 participants without antihypertensive medications, 263 (51.6%) of the subjects were men. Mean age in the sex-specific tertiles of 24-h Na+ excretion varied from 43.4 to 44.7 years (). Body weight was lowest in the lowest tertile and highest in the highest tertile, while BMI was highest in the highest tertile of Na+ excretion. BSA was higher in the highest versus the lowest tertile. There were no differences in the prevalence of smokers or alcohol consumption between the tertiles. Seated office BP, supine laboratory BP and aortic-to-popliteal PWV did not differ between the tertiles ().
Table 1. Demographics and clinical characteristics in 510 subjects, divided to tertiles according to 24-h Na+ excretion separately for sexes.
The 24-h urine volume was highest in the tertile with highest Na+ excretion (). The amounts of 24-h urine Na+, Na+/BSA, K+ and urine Na+/K+ ratio were different in all tertiles with highest values in the highest tertile. Comprehensive laboratory testing did not show any other differences between the tertiles, and the mean values of all test results were well within the normal range for each variable ().
Table 2. Urine, blood and plasma chemistry in 510 subjects divided to sex-specific tertiles according to 24-h Na+ excretion.
In the laboratory analyses of supine haemodynamics, the results are presented in BSA-adjusted tertiles of 24-h urinary Na+ excretion to eliminate the influence of differences in body size on the results [Citation8]. Supine aortic BP did not differ between the tertiles (). Heart rate, stroke volume, cardiac output and SVR were also similar in all tertiles (). ECW volume and ECW balance were higher in the highest than in the lowest tertile ().
Figure 1. Aortic systolic blood pressure (A) and aortic diastolic blood pressure (B) in tertiles of 24-h urinary Na+ excretion adjusted for body surface area; mean (circle) with standard error of the mean (whiskers); ANOVA for repeated measurements, Bonferroni correction in post-hoc analyses.
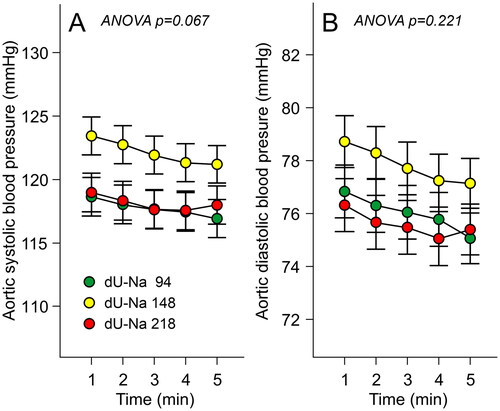
Figure 2. Heart rate (A), stroke volume (B), cardiac output (C) and systemic vascular resistance (D) in tertiles of 24-h urinary Na+ excretion adjusted for body surface area; mean (circle) with standard error of the mean (whiskers); ANOVA for repeated measurements, Bonferroni correction in post-hoc analyses.
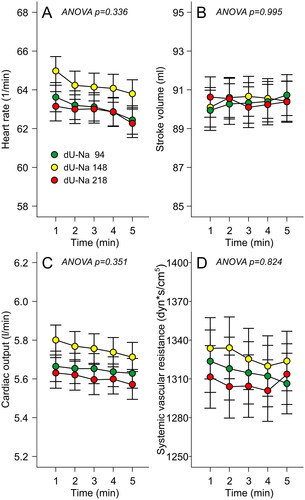
Figure 3. Extracellular water volume (A) and extracellular water balance (B) in tertiles of 24-h urinary Na+ excretion adjusted for body surface area; mean (circle) with standard error of the mean (whiskers); ANOVA for repeated measurements, Bonferroni correction in post-hoc analyses.
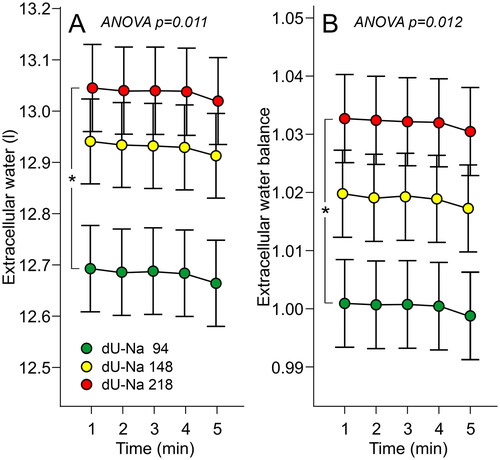
In the 510 subjects, 24-h Na+ excretion (rS=0.436, p < .001) and 24-h Na+ excretion/BSA (rS=0.242, p < .001) correlated with ECW volume. In linear regression analyses with stepwise elimination, the independent explanatory factors for ECW volume were BSA (beta 0.733) and 24-h Na+ excretion (beta 0.118) ().
Table 3. Linear regression analyses with stepwise elimination of explanatory factors for extracellular water volume in 510 subjects.
Na+ excretion and volume status were also compared between normotensive and hypertensive subjects (). In addition to higher BP, the hypertensive group had male predominance (56% vs. 39%), higher age and higher BMI than the normotensive group. In analyses adjusted for these differences between the groups, ECW volume and ECW balance, urine Na+ and K+ excretion and urine Na+/K+ ratio were similar between hypertensive and normotensive subjects. However, the hypertensive group presented with 0.4 mmol/l higher plasma Na+ concentration and 38% higher plasma NT-proBNP concentration than the normotensive group ().
Table 4. Normotensive versus hypertensive subjects: demographic data and laboratory results adjusted for differences in sex distribution, age and body mass index.
Extracellular water volume and 24-h Na+ excretion in patients with primary aldosteronism
All primary aldosteronism patients (10 women, 18 men) were taking antihypertensive medications, but only two were treated with a mineralocorticoid receptor antagonist. Their lowest plasma K+ was in the hypokalaemic range, aldosterone:renin ratio was elevated, and 24-h urinary aldosterone excretion exceeded the normal range (). In these patients serum aldosterone was determined using tandem high-performance liquid chromatography − mass spectrometry, which yields lower values than RIA [Citation37] that was applied in the analyses of the 510 subjects above. RIA overestimates aldosterone concentrations due to cross-reactivity with aldosterone metabolites [Citation37].
Table 5. Laboratory characteristics in 28 primary aldosteronism patients (10 women, 18 men) treated with antihypertensive medications.
The aldosteronism patients continued to take their antihypertensive medications and presented with the following differences [mean (SD)] when compared with the 510 subjects above: age 56 (11) vs. 44 (12) years (p < .001), BMI 30.7 (4.9) vs. 26.2 (4.2) kg/m2 (p < .001) and BSA 2.05 (0.28) vs. 1.94 (0.22) m2 (p = .015), respectively. Mean seated office BP was 162 (20)/93 (10) mmHg and mean supine laboratory BP was 158 (18)/89 (10) mmHg in the aldosteronism group. When adjusted for the differences and sex and BSA, the primary aldosteronism patients presented with [mean (95% CI)] 0.58 (0.14–1.03) litre excess in ECW volume (p = .01) and 6.0% (2.6–9.8) excess in ECW balance (p = .003), when compared with the 510 subjects above.
In the aldosteronism patients, 24-h Na+ excretion (rS=0.250, p = .199) or 24-h Na+ excretion/BSA (rS=0.09, p = .654) did not correlate with ECW volume. Plasma NT-proANP [5.81 (3.81) ng/ml] and NT-proBNP [224 (237) pg/ml] were higher in the aldosteronism patients than in the normotensive and hypertensive subjects above (p < .01 for all comparisons).
Discussion
We performed non-invasive recordings of haemodynamics to examine whether Na+ intake, as evaluated from 24-h Na+ excretion, is associated with cardiovascular variables in 510 normotensive or never-treated hypertensive Caucasian subjects. None of the participants were previously diagnosed with diabetes, cardiovascular or cerebrovascular disease, renal insufficiency, and none had medications with direct cardiovascular influences. Supine haemodynamics were recorded using whole-body impedance cardiography and pulse wave analysis. Comprehensive analyses of blood chemistry, metabolic factors, hormones and renal function that cover many mechanisms related to the regulation of BP [Citation1], showed no differences between the tertiles of Na+ excretion. The inclusion of BSA in the adjustments took into consideration the influence of body size on the results [Citation8], as BSA was significantly correlated with urinary Na+ excretion in the participants.
The present regression analyses indicated a direct association between urinary Na+ excretion and ECW volume. These findings correspond well to the recent report by Rossitto et al. that tissue Na+ excess following high Na+ intake reflects accumulation of water-paralleled Na+ in the extracellular space [Citation15]. In many of the earlier studies focussing on the relation between Na+ excretion and BP, the detailed underlying components of haemodynamics that are involved in BP regulation were not addressed [Citation38–41].
High dietary Na+ intake is a widely accepted risk factor for hypertension [Citation1]. Several studies have examined the link between Na+ intake, BP and cardiovascular events [Citation5,Citation10,Citation12,Citation40]. An almost linear association between Na+ intake and cardiovascular risk was recently reported in a study that applied two or more 24-h urine samples to evaluate dietary Na+ intake [Citation42]. Previous studies have suggested that higher Na+ intake may elevate BP by increasing blood volume, cardiac output, SVR, arterial stiffness, sympathetic activity, and by causing impaired endothelium-mediated control of arterial tone, structural changes in the arterial tree, and adverse cardiac remodelling [Citation39,Citation41,Citation43–46]. Genome wide association studies have also revealed several genetic variants with physiological functions related to Na+ homeostasis [Citation47].
However, some studies relying mainly on spot urine samples have suggested that also low Na+ intake may be harmful and elevate the risk of cardiovascular disease and mortality [Citation9,Citation10,Citation48]. In the present study, 24-h urine Na+ excretion was not related with the level of BP, whether BP was measured by physician, research nurse, or recorded tonometrically in the laboratory. Moreover, urine Na+ excretion was not related to PWV, cardiac index, or SVR. In our study population, 279 subjects presented with elevated BP in the office, while the supine central BP values during laboratory measurements were predominantly within the normotensive range [Citation1]. Thus, our findings agree with the views of a recent systematic Cochrane database review, which concluded that sodium reduction to the recommended levels would decrease mean arterial pressure by ∼0.4 mmHg in normotensive and by ∼4 mmHg in hypertensive subjects [Citation40]. The reduction in BP may be considerably more pronounced in subjects with resistant hypertension [Citation41].
The measurement of 24-h urine is not an optimal method to estimate dietary Na+ intake, as single 24-h urine collections at intakes ranging 6–12 g of NaCl per day were not appropriate to detect a 3 g difference in individual salt intake [Citation14]. Measurements of urine Na+ excretion should actually last for several days to detect the true amount of ingested Na+ [Citation42]. Nevertheless, the 24-h urine Na+ excretion remains a feasible measure to assess Na+ intake in larger groups of subjects [Citation49].
As the major extracellular cation, Na+ is rapidly distributed throughout the body. Infusion of hypertonic 7.5% NaCl solution causes extracellular fluid expansion, followed by gradual translocation of fluid also to the intracellular space [Citation50]. Previously, extracellular-to-intracellular fluid ratio was higher during high vs. low Na+ intake in 20 hypertensive patients with chronic kidney disease [Citation43], while Na+ excess in tissues during high Na+ intake has been found to reflect the extracellular accumulation of water and Na+ [Citation15]. In elderly men, Na+ intake directly correlated with leg oedema and differences in leg extracellular fluid contents between daytime and night-time [Citation51]. Our present results suggest a direct independent relation between 24-h Na+ excretion and ECW volume in the absence of an association with the level of BP. In the regression analysis, BSA and ECW volume were the only independent factors related with urinary 24-h Na+-excretion. The observed independent association between urine Na+ excretion and ECW was not driven by age, as no association was observed between age and 24-h Na+ excretion in the regression analyses.
The human skin and muscle may buffer dietary Na+ and thus result in water-free Na+ storage, and this mechanism may reduce the hemodynamic consequences of increased Na+ intake [Citation16,Citation17,Citation52]. The Na+ ions may be stored by the use of negatively charged glycosaminoglycans [Citation53], but the exact mechanisms how water-free Na+ is buffered in the human body and how this affects BP regulation remain largely unknown [Citation8]. An attractive hypothesis is that when these mechanisms fail, Na+ intake becomes a potential contributor to the pathophysiology of hypertension [Citation8,Citation16].
In the present study, we also compared Na+ excretion and ECW volume between normotensive and hypertensive subjects [Citation1]. Clear deviations in BPs between these groups prevailed also in supine laboratory measurements. We found no differences in ECW volume, ECW balance, renal function, Na+ excretion, K+ excretion, plasma renin activity and plasma aldosterone and NT-proANP concentrations between these groups. However, the hypertensive subjects presented with a small 0.4 mmol/l elevation in plasma Na+ concentration when compared with the normotensive subjects, but the significance of this finding remains unknown. ANP is released to the blood stream from secretory granules in response to cardiomyocyte stretch, and its concentration is higher in the atria than in the ventricles [Citation54]. BNP is synthetised in atrial and ventricular cardiomyocytes, but it is not stored to the same extent as ANP. In a pressure-burdened heart, left ventricular BNP synthesis increases with a subsequent elevation in plasma BNP [Citation55]. Thus, ANP release more acutely responds to increases in volume load while higher BNP levels more reflect sustained cardiac pressure and volume overloads [Citation54]. The plasma NT-proANP and NT-proBNP concentrations were similar in the tertiles of Na+ excretion, while NT-proANP were also similar in the normotensive and hypertensive subgroups. These findings are compatible with the view of corresponding blood volumes in these groups. In contrast, 38% elevation of plasma NT-proBNP in the hypertensive group indicates higher pressure load to the heart in these subjects [Citation54]. Altogether, several indirect findings suggest that there were no differences in the circulating blood volumes between the tertiles of Na+ excretion: similar haematocrit, haemoglobin, plasma renin activity and plasma concentrations of Na+, aldosterone, NT-proANP and NT-proBNP.
For comparison, we examined 28 patients with confirmed primary aldosteronism. Patients with primary aldosteronism are known to present with increased ECW volume when compared with patients with primary hypertension and normotensive subjects [Citation20]. The present aldosteronism group was characterised by 6.0% excess in ECW, and no relation was found between 24-h Na+ excretion and extracellular water volume in them. As expected, the NT-proANP and NT-proBNP concentrations in plasma were clearly elevated among the aldosteronism patients when compared with normotensive and hypertensive subjects. Obviously, increased ECW volume reflected the disruption of normal Na+ and water homeostasis due to mineralocorticoid excess in these patients.
Our study has limitations. Conclusions about causal relationships are precluded due to the cross-sectional approach. We applied indirect non-invasive methods with mathematical processing to derive ECW, cardiac output, SVR and PWV from the bioimpedance signal [Citation28–30] and central aortic BP from the tonometry signal [Citation27]. Even though the methods have been validated against direct or invasive measurements, the results must be interpreted with caution. Also, we cannot exclude potential multicollinearity problems that were not controlled for by the statistical methods, as many clinical characteristics and biochemical variables are correlated with each other. However, except for 24-h Na+ and K+ excretion, none of the other laboratory variables in the analyses were different between the Na+ excretion tertiles, and these variables were meticulously included in the regression analysis. The similarity of the laboratory result profiles has arguably increased the reliability of the comparisons between the tertiles of Na+ excretion. Although some methodological variations occurred in the current renin and aldosterone measurements, our findings indicate no significant differences in plasma renin activities and aldosterone concentrations between the tertiles of Na+ excretion that would indicate differences in circulating blood volumes between the tertiles. The participants had some concurrent illnesses that were well controlled but may have affected the results. Importantly, subjects with potential causes for abnormal BP and ECW volume regulation like renal disease, diabetes, or any severe acute or chronic illness were excluded.
To conclude, an independent direct association between 24-h Na+ excretion and ECW was observed in 510 normotensive or never-treated hypertensive subjects aged 19–72 years. All subjects were free of diabetes, cardiovascular or renal comorbidities and cardiovascular medications. The present results indicate that in the absence of mineralocorticoid excess, dietary Na+ intake predominantly influences ECW regulation, and this effect is observed without parallel changes in BP.
Acknowledgements
The authors thank research nurses Emmi Hirvelä, Paula Liikanen and Reeta Kulmala for invaluable technical assistance and Heini Huhtala, MSc, for statistical support. The authors wish to acknowledge CSC – IT Center for Science, Finland, for computational resources.
Disclosure statement
No potential conflict of interest was reported by the author(s).
Data availability statement
Analyses and datasets of the current study are not available publicly as the clinical database contains several indirect identifiers and the informed consent obtained does not allow publication of individual patient data. The datasets are available from the corresponding author on reasonable request.
Additional information
Funding
References
- Williams B, Mancia G, Spiering W, et al. 2018 ESC/ESH guidelines for the management of arterial hypertension. Eur Heart J. 2018;39(33):3021–3104.
- Vasan RS, Larson MG, Leip EP, et al. Impact of high-normal blood pressure on the risk of cardiovascular disease. N Engl J Med. 2001;345(18):1291–1297.
- Kshirsagar AV, Carpenter M, Bang H, et al. Blood pressure usually considered normal is associated with an elevated risk of cardiovascular disease. Am J Med. 2006;119(2):133–141.
- Verma N, Rastogi S, Chia Y-C, et al. Non-pharmacological management of hypertension. J Clin Hypertens. 2021;23(7):1275–1283.
- Filippini T, Malavolti M, Whelton PK, et al. Blood pressure effects of sodium reduction: dose-response meta-analysis of experimental studies. Circulation. 2021;143(16):1542–1567.
- Drewnowski A, Rehm CD, Maillot M, et al. The feasibility of meeting the WHO guidelines for sodium and potassium: a cross-national comparison study. BMJ Open. 2015;5(3):e006625.
- World Health Organization. Salt reduction; 2020. Available from: www.who.int/news-room/fact-sheets/detail/salt-reduction
- Bie P. Mechanisms of sodium balance: total body sodium, surrogate variables, and renal sodium excretion. Am J Physiol Regul Integr Comp Physiol. 2018;315(5):R945–R962.
- Mente A, O'Donnell M, Rangarajan S, et al. Associations of urinary sodium excretion with cardiovascular events in individuals with and without hypertension: a pooled analysis of data from four studies. Lancet. 2016;388(10043):465–475.
- Mente A, O'Donnell M, Rangarajan S, et al. Urinary sodium excretion, blood pressure, cardiovascular disease, and mortality: a community-level prospective epidemiological cohort study. Lancet. 2018;392(10146):496–506.
- Thomas MC, Moran J, Forsblom C, et al. The association between dietary sodium intake, ESRD, and all-cause mortality in patients with type 1 diabetes. Diabetes Care. 2011;34(4):861–866.
- Li J, Zhen Z, Huang P, et al. Salt restriction and risk of adverse outcomes in heart failure with preserved ejection fraction. Heart. 2022;108(17):1377–1382.
- Alonso S, Tan M, Wang C, et al. Impact of the 2003 to 2018 population salt intake reduction program in England. Hypertension. 2021;77(4):1086–1094.
- Lerchl K, Rakova N, Dahlmann A, et al. Agreement between 24-hour salt ingestion and sodium excretion in a controlled environment. Hypertension. 2015;66(4):850–857.
- Rossitto G, Mary S, Chen JY, et al. Tissue sodium excess is not hypertonic and reflects extracellular volume expansion. Nat Commun. 2020;11(1):4222.
- Minegishi S, Luft FC, Titze J, et al. Sodium handling and interaction in numerous organs. Am J Hypertens. 2020;33(8):687–694.
- Selvarajah V, Mäki-Petäjä KM, Pedro L, et al. Novel mechanism for buffering dietary salt in humans: effects of salt loading on skin sodium, vascular endothelial growth factor C, and blood pressure. Hypertension. 2017;70(5):930–937.
- Tahvanainen A, Koskela J, Tikkakoski A, et al. Analysis of cardiovascular responses to passive head‐up tilt using continuous pulse wave analysis and impedance cardiography. Scand J Clin Lab Invest. 2009;69(1):128–137.
- Tikkakoski AJ, Tahvanainen AM, Leskinen MH, et al. Hemodynamic alterations in hypertensive patients at rest and during passive head-up tilt. J Hypertens. 2013;31(5):906–915.
- Choudhary MK, Värri E, Matikainen N, et al. Primary aldosteronism: higher volume load, cardiac output and arterial stiffness than in essential hypertension. J Intern Med. 2021;289(1):29–41.
- Katz A, Nambi SS, Mather K, et al. Quantitative insulin sensitivity check index: a simple, accurate method for assessing insulin sensitivity in humans. J Clin Endocrinol Metab. 2000;85(7):2402–2410.
- Inker LA, Schmid CH, Tighiouart H, et al. Estimating glomerular filtration rate from serum creatinine and cystatin C. N Engl J Med. 2012;367(1):20–29.
- Juutilainen A, Savolainen K, Romppanen J, et al. Combination of LC–MS/MS aldosterone and automated direct renin in screening for primary aldosteronism. Clin Chim Acta. 2014;433:209–215.
- Turpeinen U, Hämäläinen E, Stenman U-H. Determination of aldosterone in serum by liquid chromatography–tandem mass spectrometry. J Chromatogr B. 2008;862(1-2):113–118.
- Choudhary MK, Eräranta A, Tikkakoski AJ, et al. Effect of present versus previous smoking on non-invasive haemodynamics. Sci Rep. 2018;8(1):13643.
- Kangas P, Tahvanainen A, Tikkakoski A, et al. Increased cardiac workload in the upright posture in men: noninvasive hemodynamics in men versus women. J Am Heart Assoc. 2016;5:e002883.
- Chen C-H, Nevo E, Fetics B, et al. Estimation of central aortic pressure waveform by mathematical transformation of radial tonometry pressure: validation of generalized transfer function. Circulation. 1997;95(7):1827–1836.
- Kööbi T, Kähönen M, Koskinen M, et al. Comparison of bioimpedance and radioisotope methods in the estimation of extracellular water volume before and after coronary artery bypass grafting operation. Clin Physiol. 2000;20(4):283–291.
- Kööbi T, Kähönen M, Iivainen T, et al. Simultaneous non-invasive assessment of arterial stiffness and haemodynamics–a validation study. Clin Physiol Funct Imaging. 2003;23(1):31–36.
- Kööbi T, Kaukinen S, Ahola T, et al. Non-invasive measurement of cardiac output: whole-body impedance cardiography in simultaneous comparison with thermodilution and direct oxygen Fick methods. Intensive Care Med. 1997;23(11):1132–1137.
- Tishchenko M. (Estimation of the stroke volume by integral rheogram of the human body) [in Russian]. Sechenov Physiol J. 1973;59:1216–1224.
- Nadler SB, Hidalgo JH, Bloch T. Prediction of blood volume in normal human adults. Surgery. 1962;51(2):224–232.
- Albert SN. Blood volume and extracellular fluid volume (American Lecture Series, Publication no. 807). 2nd ed. Springfield (IL): Thomas CC; 1971.
- Dubois F, Dubois E. A formula to estimate the approximate surface area if height and weight be known. Arch Intern Med. 1916;17:863–871.
- Laurent S, Cockcroft J, Van Bortel L, et al. Expert consensus document on arterial stiffness: methodological issues and clinical applications. Eur Heart J. 2006;27(21):2588–2605.
- Townsend RR, Wilkinson IB, Schiffrin EL, et al. Recommendations for improving and standardizing vascular research on arterial stiffness: a scientific statement from the American Heart Association. Hypertension. 2015;66(3):698–722.
- Funder JW, Carey RM, Mantero F, et al. The management of primary aldosteronism: case detection, diagnosis, and treatment: an endocrine society clinical practice guideline. J Clin Endocrinol Metab. 2016;101(5):1889–1916.
- Kesteloot H, Joossens JV. Relationship of serum sodium, potassium, calcium, and phosphorus with blood pressure. Belgian interuniversity research on nutrition and health. Hypertension. 1988;12(6):589–593.
- Baldo MP, Brant LCC, Cunha RS, et al. The association between salt intake and arterial stiffness is influenced by a sex-specific mediating effect through blood pressure in normotensive adults: the ELSA-Brasil study. J Clin Hypertens. 2019;21(12):1771–1779.
- Graudal NA, Hubeck-Graudal T, Jurgens G. Effects of low sodium diet versus high sodium diet on blood pressure, renin, aldosterone, catecholamines, cholesterol, and triglyceride. Cochrane Database Syst Rev. 2020;12(12):CD004022.
- Pimenta E, Gaddam KK, Oparil S, et al. Effects of dietary sodium reduction on blood pressure in subjects with resistant hypertension: results from a randomized trial. Hypertension. 2009;54(3):475–481.
- Ma Y, He FJ, Sun Q, et al. 24-hour urinary sodium and potassium excretion and cardiovascular risk. N Engl J Med. 2022;386(3):252–263.
- Campbell KL, Johnson DW, Bauer JD, et al. A randomized trial of sodium-restriction on kidney function, fluid volume and adipokines in CKD patients. BMC Nephrol. 2014;15:57.
- Feng W, Dell’Italia LJ, Sanders PW. Novel paradigms of salt and hypertension. J Am Soc Nephrol. 2017;28(5):1362–1369.
- Jablonski KL, Racine ML, Geolfos CJ, et al. Dietary sodium restriction reverses vascular endothelial dysfunction in middle-aged/older adults with moderately elevated systolic blood pressure. J Am Coll Cardiol. 2013;61(3):335–343.
- Selvaraj S, Djoussé L, Aguilar FG, et al. Association of estimated sodium intake with adverse cardiac structure and function: from the HyperGEN study. J Am Coll Cardiol. 2017;70(6):715–724.
- Razavi MA, Bazzano LA, Nierenberg J, et al. Advances in genomics research of blood pressure responses to dietary sodium and potassium intakes. Hypertension. 2021;78(1):4–15.
- Park CY, Jo G, Lee J, et al. Association between dietary sodium intake and disease burden and mortality in Koreans between 1998 and 2016: the Korea National Health and Nutrition Examination Survey. Nutr Res Pract. 2020;14(5):501–518.
- Terry AL, Cogswell ME, Wang C-Y, et al. Feasibility of collecting 24-h urine to monitor sodium intake in the National Health and Nutrition Examination Survey. Am J Clin Nutr. 2016;104(2):480–488.
- Svensén CH, Waldrop KS, Edsberg L, et al. Natriuresis and the extracellular volume expansion by hypertonic saline. J Surg Res. 2003;113(1):6–12.
- Yoshikawa M, Torimoto K, Hirayama A, et al. Daily salt intake is associated with leg edema and nocturnal urinary volume in elderly men. Neurourol Urodyn. 2020;39(5):1550–1556.
- Titze J, Maillet A, Lang R, et al. Long-term sodium balance in humans in a terrestrial space station simulation study. Am J Kidney Dis. 2002;40(3):508–516.
- Machnik A, Neuhofer W, Jantsch J, et al. Macrophages regulate salt-dependent volume and blood pressure by a vascular endothelial growth factor-C–dependent buffering mechanism. Nat Med. 2009;15(5):545–552.
- Ruskoaho H. Cardiac hormones as diagnostic tools in heart failure. Endocr Rev. 2003;24(3):341–356.
- Volpe M, Rubattu S, Burnett J. Natriuretic peptides in cardiovascular diseases: current use and perspectives. Eur Heart J. 2014;35(7):419–425.