ABSTRACT
Non-invasive brain stimulation techniques allow targeted modulation of brain regions and have emerged as a promising tool for vision rehabilitation. This review presents an overview of studies that have examined the use of non-invasive brain stimulation techniques for improving vision and visual functions. A description of the proposed neural mechanisms that underpin non-invasive brain stimulation effects is also provided. The clinical implications of non-invasive brain stimulation in vision rehabilitation are examined, including their safety, effectiveness, and potential applications in specific conditions such as amblyopia, post-stroke hemianopia, and central vision loss associated with age-related macular degeneration. Additionally, the future directions of research in this field are considered, including the need for larger and more rigorous clinical trials to validate the efficacy of these techniques. Overall, this review highlights the potential for brain stimulation techniques as a promising avenue for improving visual function in individuals with impaired vision and underscores the importance of continued research in this field.
Introduction
Non-invasive brain stimulation (NIBS) techniques use electrical currents or magnetic fields to stimulate specific areas of the brain by transiently altering the excitability of targeted neural populations.Citation1 Two commonly used methods are repetitive transcranial magnetic stimulation (rTMS) and transcranial electrical stimulation (tES). Both methods exert an effect on the underlying cortex, including changes in cortical excitability and alterations in various neurotransmitter concentrations, which in turn can influence neural function (). Although NIBS has historically been employed as a research tool, these methods are now being applied in clinical and therapeutic settings and have emerged as promising tools for improving visual functions.Citation14
Table 1. Table summarising the key features and proposed mechanisms for TMS and tES.
rTMS and tES both evolved as NIBS techniques with applications in psychiatry and neurology. The US Food and Drug administration (FDA) granted approval for the use of rTMS to treat major depressive disorder in 2008, and ongoing clinical trials have facilitated the clinical adoption of rTMS in the US for conditions such as migraine with aura, obsessive compulsive disorder, and smoking cessation.Citation15 Both rTMS and tES are currently being used in a range of clinical populations and are showing promise as tools for vision rehabilitation. This is an exciting prospect as it opens up the possibility of a novel treatment approach, especially for vision disorders where optical and medical management are the only viable options currently.
In this review, an introductory resource on NIBS techniques and their applications in vision rehabilitation will be provided. Various stimulation techniques and their use in vision-related conditions are discussed, including amblyopia, post-stroke hemianopia, and age-related macular degeneration. This review will offer a high-level understanding of the current state, advancements, and challenges of NIBS in vision rehabilitation. Additionally, emerging trends and future research directions will be explored. By the end of this review, readers will gain insights into different stimulation approaches, their underlying mechanisms, and their potential for improving visual function. The goal of this review is to offer a primer for clinicians and individuals interested in NIBS for vision rehabilitation.
Methods
The studies provided in this review are part of a larger systematic review,Citation14 which employed the following search strategy. Search terms are categorised into two main themes: ‘brain area’ and ‘non-invasive brain stimulation’. Each theme was combined using the Boolean operator ‘AND’ while within each theme, relevant terms were combined using the Boolean operator ‘OR’. The search terms included the terms ‘Primary visual cortex’, ‘V1’, ‘Extrastriate cortex’, ‘V2’, ‘V3’, ‘V3a’, ‘V4’, ‘V5’, ‘Middle temporal’, ‘MT’, ‘Medial superior temporal’, ‘MST’, ‘Occipital pole’, and ‘Occipital lobe’.
The search terms for non-invasive brain stimulation techniques included ‘Transcranial direct current stimulation’, ‘tDCS’, ‘Transcranial alternating current stimulation’, ‘tACS’, ‘Transcranial random noise stimulation’, ‘tRNS’, ‘Transcranial magnetic stimulation’, ‘TMS’, ‘Repetitive transcranial magnetic stimulation’, ‘rTMS’, ‘Continuous theta burst stimulation’, ‘cTBS’, and ‘Theta burst stimulation’, ‘TBS’. Studies were selected for relevance to vision and for a clinical audience for this present review.
While this review does not cover transcorneal and transorbital stimulation, it is worth mentioning that these additional electrical stimulation techniques have demonstrated evidence of being effective for vision rehabilitation (see Perin et al.Citation16 for review). The diverse nature of visual disorders, which involves distinct structural changes and impairments in different cerebral regions associated with vision, underscores the need for tailored approaches and applications of electrical stimulation in visual rehabilitation.
Transcranial magnetic stimulation
TMS has been used for over three decades to study human brain physiology, and uses a varying magnetic field to induce a brief electric current within nearby brain tissue ().Citation2 A single pulse of TMS can trigger action potentials, whereas repeated pulses can have either excitatory or inhibitory effects on neural activity depending on the configuration of the pulse train ().
Figure 2. Schematic showing facilitatory and inhibitory TMS and tES protocols. There is no inhibitory tRNS protocol. MSO = maximum stimulator intensity.
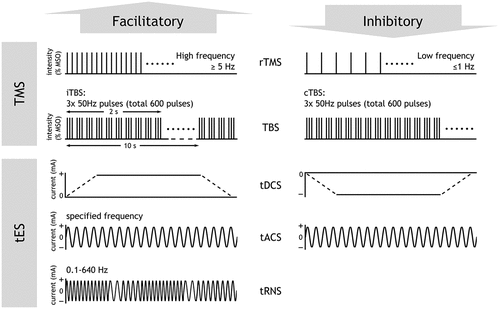
Single-pulse TMS, when delivered over the primary visual cortex (V1), can induce visual percepts referred to as phosphenes (spots of light in the visual field)Citation17 and transient scotomas (visual suppression).Citation18 Owing to the high temporal fidelity of TMS, single pulses applied during a task can reveal the roles of specific brain areas, and when they are active in connection to the specific task being performed. For instance, single pulse TMS over V1 can impair visual perception when delivered at specific time points relative to the onset of visual stimuli.Citation19 When multiple stimulation pulses are delivered, rTMS can facilitate or inhibit neuronal firing thresholds.
The excitatory or inhibitory effect on the stimulated brain region depends on whether the pulses are delivered at high (≥5 Hz) or low frequencies (0.1–1 Hz), respectively.Citation20 Theta burst stimulation (TBS) is another form of rTMS, in which pulses are delivered in continuous (cTBS) or intermittent (iTBS) bursts of three 50 Hz pulse trains.Citation21 Compared to traditional rTMS paradigms, cTBS and iTBS are becoming more widely used as they provide more powerful, long-lasting effects and require shorter stimulation durations (less than 2–3 minutes). rTMS can produce powerful ‘offline’ effects, where the effects outlast the period of stimulation. Therefore, these protocols are frequently used to examine the role of specific brain regions in plasticity and behaviour.
Transcranial electrical stimulation
Transcranial electrical stimulation (tES) is another form of NIBS, which alters brain activity by passing an electrical current through the cortex, using two or more electrodes placed on the scalp (). The different types of tES include transcranial direct current stimulation (tDCS), transcranial alternating current stimulation (tACS), and transcranial random noise stimulation (tRNS). For each of these tES types, a 1–2 mA current is applied for up to 20 minutes using electrodes that are typically 20–35 cm2 in size.Citation22 Each tES type causes different behavioural and neuronal changes owing to the different electrical stimulation patterns used ().
tDCS is the most commonly used form of tES and involves the one-way propagation of an electrical current between an anodal (positive) and cathodal (negative) electrode.Citation23 tDCS causes an increase in excitability in cortical regions below the anodal electrode, and a decrease in regions located below the cathodal electrode. tACS uses a sinusoidal current to modulate cortical activity in a frequency-specific manner and can entrain neural oscillations. It has been used to investigate the role of brain oscillations in cognition and perception,Citation24 as well as to treat various neurological and psychiatric disorders such as depression and chronic pain.Citation25 tRNS employs an alternating current with random amplitude and frequency. By enhancing cortical excitability, tRNS promotes the strengthening of neural connections in the stimulated region.Citation26
Rationale for applying NIBS to vision rehabilitation
NIBS is a promising tool for research and clinical use due to its ability to alter cortical excitability, modulate neurotransmitter levels,Citation27 and influence distributed connected neural networks and facilitate the transfer of information between different brain regions.Citation28 In the context of the visual cortex, there is evidence that brain stimulation can open windows of neuroplasticity, which can be harnessed for vision rehabilitation and improving visual function in adults.Citation29–34
Neuroplasticity refers to the process of changing the strength and efficacy of connections between neurons to improve the overall function of the brain in response to external stimuli over time. The ‘critical period’ of visual development refers to a specific window of time during early development when the visual system is particularly sensitive to environmental input and is capable of rapid and profound changes in response to visual experience. This critical period is a period of heightened neuroplasticity, during which the network of cortical areas that support vision is highly adaptable and can be shaped by sensory experience.Citation35
Beyond this critical period, many areas of the brain, including the visual cortex, become less malleable and more resistant to change. Factors such as declines in gene expression related to neuroplasticity and reductions in neuromodulators such as dopamine and acetylcholine contribute to the closure of the sensitive period and the limited capacity for sensory experience to shape cortical function.Citation36
Despite the closure of the sensitive period for visual development, some degree of neuroplasticity persists in the brain into later childhood, adolescence, and adulthood, as evidenced by studies in amblyopia.Citation37 This indicates that targeted approaches may still be effective in inducing neuroplasticity during these periods outside of the critical period. However, given that the capacity for sensory experiences to shape cortical function declines with age, enhancing neural plasticity becomes critical for effective vision rehabilitation, especially in adults. NIBS represents a promising avenue for manipulating the mechanisms that control cortical plasticity and improving visual function in adults.
The facilitatory and inhibitory effects of NIBS are thought to reflect long-term potentiation or long-term depression like mechanisms, which refer to the strengthening and weakening of synaptic connections, respectively (). By inducing long term potentiation or depression, NIBS may be able to modulate the strength and structure of neural connections in the visual cortex, leading to improvements in visual performance. While the exact mechanisms are not yet fully understood, ongoing research focusses on finding key brain circuit elements that regulate brain plasticity and identifying potential therapeutic targets to promote neuroplasticity in the visual system, both during and beyond early life.Citation38
Figure 3. Simplified schematic showing the insertion of a receptor (e.g., NMDA receptor) to the synaptic membrane in response to an increase in intracellular Ca+2, demonstrating long term potentiation (LTP; top right). Removal of a receptor from the membrane is shown for long term depression (LTD; top left), resulting in a decreased postsynaptic response. Long term potentiation and depression mediate the strengthening and weakening of synaptic connections, respectively, resulting in increased and decreased postsynaptic responses (bottom right and left panels).
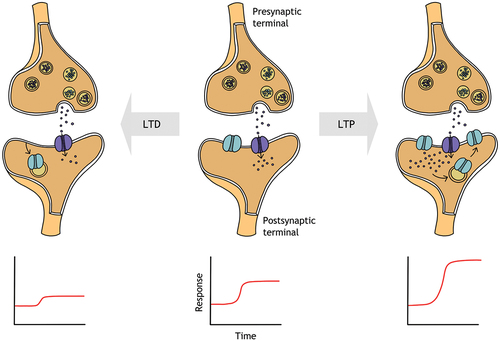
Proposed mechanisms of NIBS
Emerging evidence in the literature suggests that NIBS may be a promising way to manage disorders of vision and visual function such as amblyopia, age-related macular degeneration (AMD), hemianopia and other visual field deficits. There is now sufficient evidence to support the effectiveness of NIBS techniques in enhancing visual acuity,Citation39 contrast sensitivity,Citation40–42 and motion perceptionCitation43–45 in typically sighted individuals.
Additionally, NIBS modulates cortical excitability measures such as visual evoked potentialsCitation39,Citation46,Citation47 – see Bello et al.Citation14 for review. Therefore, NIBS can modulate neural activity in specific regions of the brain involved in visual processing, leading to improvements in visual function. The following sections will explore the various proposed mechanisms of NIBS, providing an overview of how NIBS exerts its effects to modulate behaviour.
Modulation of NMDA receptors
N-methyl-D-aspartate (NMDA) receptors are a subtype of glutamate receptors, and the activation of pre- and postsynaptic NMDA receptors is a characteristic of long term potentiation and depression. The activation of postsynaptic NMDA receptors induces an influx of Ca+2, triggering a cascade of intracellular signalling pathways that lead to long term changes in synaptic strength.Citation48 Studies using pharmacological agents and motor cortex NIBS have provided indirect evidence for the involvement of NMDA receptors in long-term potentiation, depression-like changes, and the therapeutic effects of NIBS. For example, dextromethorphan, an NMDA receptor antagonist, blocked the effects of anodal and cathodal tDCS, indicating the role of NMDA receptors in tDCS-induced neuroplasticity.Citation4 Another study showed that D-Cycloserine, a partial NMDA receptor agonist, prolonged the duration of increased cortical excitability induced by anodal tDCS.Citation5 Additionally, memantine, an NMDA receptor antagonist, blocked the after-effects of cTBS and iTBS, further supporting the importance of NMDA receptors in producing lasting effects of TBS.Citation6
NIBS techniques may also trigger the release of neurotrophic factors, such as BDNF, which play crucial roles in nervous system development and maintenance. Blood derived neurotrophic factor (BDNF) is one such protein, which affects synaptic plasticity and NMDA receptor function.Citation49 The expression of BDNF genes can affect synaptic plasticity in both animal and human cortex, influencing the efficacy of NIBS techniques.Citation50,Citation51
GABA-mediated intracortical inhibition
While BDNF and NMDA receptors are involved in promoting synaptic plasticity, GABA-mediated intracortical inhibition is another possible mechanism that has been identified through which NIBS may enhance neuroplasticity in the visual cortex. GABA is an inhibitory neurotransmitter that regulates neural activity and synaptic plasticity in the brain, and gating neuroplasticity within the mature visual cortex.Citation38,Citation52
Recent studies have shown that intracortical inhibition is a critical limiting factor for cortical plasticity in adults and that reducing it can significantly enhance plasticity in the adult visual cortex – see Karmarker and DanCitation53 for review. However, the direction of GABA modulation following excitatory and inhibitory NIBS protocols in the visual cortex is inconsistent,Citation54–56 and remains an area of ongoing study.
Local GABA levels are correlated with behaviour in both the primary motor and visual cortices – see Li et al.Citation57 for review. Visual cortex rTMS has demonstrated improvements in visual acuity and other visual functions in adults with amblyopia.Citation29 Repeated sessions of visual cortex cTBS have led to long-lasting improvements in visual functions such as visual acuity, stereoacuity, and suppressive balance (a measure of binocular function) in adults with amblyopia.Citation32
Evidence from animal studies suggest the reduction in GABA-mediated cortical inhibition from visual cortex NIBS may explain the observed improvements in amblyopic visual function.Citation58 This idea is supported by previous studies of the human motor cortex, which have extensively investigated GABAergic activity and shown that specific TMS protocolsCitation7,Citation8 and anodal tDCSCitation9 can reduce GABA concentration in this area, potentially leading to similar effects in the visual cortex.
Improving signal to noise ratio within neural systems
Stochastic resonance refers to the phenomenon where previously undetectable or sub-threshold stimuli can become detectable by adding an appropriate level of noise.Citation59 tRNS applied over the visual cortex improves visual stimulus detectionCitation60 and motion discrimination,Citation61 and this is attributed to tRNS inducing random activity in target neurons, increasing sensitivity to external inputs. Applying tRNS and other NIBS techniques that harness stochastic resonance holds promise for vision rehabilitation, due to its potential in facilitating the detection of weak signals and promoting learning and neural plasticity.Citation60 Notably, visual cortex tRNS has demonstrated improvements in contrast sensitivity and visual acuity in individuals with amblyopia,Citation62 highlighting the potential of NIBS techniques to enhance visual function and provide new treatment options for visual impairments.
Heterogeneity of NIBS effects
Several factors can influence individual responses to NIBS techniques for inducing plasticity. While there is currently limited research specifically focussed on factors affecting the induction of plasticity in the human visual cortex, animal studies have provided evidence of significant sex differences in processes relevant to cortical plasticity.Citation63,Citation64 A retrospective analysis combining data from three previous studies, including 24 females and 22 males, found that females exhibited stronger facilitatory effects when anodal tDCS was applied to the visual cortex.Citation65 While this study is the only one specifically examining the visual cortex, other studies have indicated that sex differences may be influenced by hormone levels. For example, a study on the motor cortex showed reduced plasticity in older post-menopausal females compared to pre-menopausal females.Citation66
Furthermore, hormonal variations across the menstrual cycle may further influence short-term plasticity induced by rTMS.Citation67 Genetic factors, such as polymorphisms of neurotrophins like BDNF Val66Met variant, also contribute to individual responses to NIBS, affecting the effectiveness of protocols like tDCS and TBS,Citation68 which rely on NMDA receptors for their aftereffects.Citation4,Citation6
Other factors, such as physical activity,Citation69,Citation70 time of measurement,Citation71 attention,Citation72,Citation73 pharmacology,Citation4,Citation6,Citation7 age,Citation66,Citation74 and geneticsCitation50 have been found to influence individual responses to NIBS. However, studies investigating these factors primarily focus on non-visual cortex areas, and there is a lack of systematic evidence and large-scale studies specifically examining their relevance to vision rehabilitation. Despite this, considering these factors is important when evaluating the efficacy of NIBS targeting the visual cortex, as it can help understand individual variability in response to NIBS and potentially optimise its effectiveness in vision rehabilitation.
Risk factors
While NIBS has shown promise in improving visual function, there are potential risks associated with its use in visual rehabilitation or in a clinical or research setting. Risks may vary based on the NIBS technique, stimulation parameters, and individual characteristics. Potential risks include headache and discomfort, cognitive and mood effects, and visual disturbances, and the rare risk of inducing seizures, especially in individuals with epilepsy or neurological conditions.Citation75 Headache and discomfort are usually transient and can be managed with over-the-counter pain relievers.
NIBS techniques may also induce changes in cognitive or mood states, particularly in individuals with pre-existing psychiatric or neurological conditions, but the clinical significance is not yet fully understood. Additionally, NIBS techniques may interfere with the function of medical devices such as pacemakers or cochlear implants, requiring careful screening and monitoring during stimulation.
The potential risks of NIBS in visual rehabilitation are low but should be weighed against the potential benefits. Established safety guidelinesCitation75–77 and use of screening questionnairesCitation78 can be used by the researcher or by treating physicians to assess the risk/benefit ratio of administering NIBS. To minimise adverse events, thorough patient screening and monitoring of stimulation parameters by trained professionals are crucial, considering potential risks and side effects. These considerations are particularly important as the number of at-home stimulation studies increase,Citation79,Citation80 and brain stimulator devices become more accessible direct to consumers. The home use tDCS guidelines by Charvet et al.Citation81 provide valuable guidance on the safe and effective use of at-home stimulation devices for clinical research and practice.
Eye care practitioners, like optometrists in primary care, could play a valuable role in monitoring individuals using at-home brain stimulator devices. Their expertise in eye care and community accessibility makes them well-placed to assess the safety and effectiveness of NIBS interventions. Collaboration between eyecare professionals and researchers is necessary to establish appropriate monitoring protocols and identify relevant clinical tests for assessing the effectiveness of at-home NIBS in specific visual functions. For example, in the context of vision rehabilitation, optometrists can conduct regular perimetry tests to assess improvements in the visual field when NIBS is being used to enhance residual function in individuals with glaucoma.
Advancements and challenges in NIBS for visual rehabilitation
The field of NIBS for visual rehabilitation is currently an active area of research, with many studies investigating the potential applications in improving visual function for various conditions. These conditions include amblyopia,Citation29,Citation31,Citation32,Citation62,Citation82,Citation83 post-stroke hemianopia,Citation33,Citation84–88 and central vision loss resulting from conditions such as AMD.Citation34,Citation89 While the results thus far are promising, there is still much to be explored or understood.
Studies on the effectiveness of NIBS in treating amblyopia have revealed improvements in several visual functions. Ding et al.Citation82 applied anodal tDCS to individuals with amblyopia which resulted in enhanced contrast sensitivity and larger visual evoked potential amplitudes in the amblyopic eye. Similar improvements in contrast sensitivity have been observed following rTMS,Citation29 cTBS,Citation30 and anodal tDCS.Citation31 Additionally, cTBS has been found to improve visual acuity, suppressive imbalance, and stereoacuity in individuals with amblyopia.Citation32
Combination approaches, such as anodal tDCS with dichoptic video gaming, have also demonstrated the potential to enhance the recovery of stereopsis in adults with amblyopia.Citation31,Citation90 The growing evidence supports the efficacy of NIBS in amblyopia and shows promising results.
For hemianopia, a combined approach of NIBS and visual trainingCitation33,Citation84–86 is more effective in restoring visual function, particularly in the visual field, compared to NIBS alone.Citation87,Citation88 The timing of stimulation may also impact its efficacy, with evidence suggesting that applying stimulation during the acute or subacute phase after stroke, when plasticity is at its highest, may be more beneficial.Citation91
To date, there are comparatively fewer studies on the use of NIBS for vision rehabilitation in central vision loss. Research in this area often focusses on specific visual phenomena found in peripheral vision, such as visual crowding and lateral masking. Lateral masking is a visual phenomenon where a central target Gabor patch is presented between two flanker patches of the same orientation, and the ability to detect the target can either be increased (collinear facilitation) or decreased (collinear inhibition) depending on the distance between the target and the flankers.
Intensive perceptual learning (visual training) can reduce collinear lateral inhibition in peripheral vision,Citation93 and recent work by Raveendran et al.Citation92 demonstrated that visual cortex anodal tDCS can reduce collinear inhibition in typically-sighted individuals. More recently, Raveendran et al.Citation34 were able to replicate this effect in individuals with AMD, offering a potential approach to improving residual visual function in central vision loss. In a recent randomised, double-blind clinical study, combined visual cortex anodal tDCS and perceptual learning of a rapid serial visual presentation reading task was shown to be effective in improving English reading individuals with AMD.Citation89
Despite the promising results, challenges remain in developing optimal treatment protocols for different types of visual impairments. The optimal parameters for NIBS, such as duration, intensity, and location of stimulation, are still being investigated, and there is no consensus on the best rehabilitative approach for different types of visual impairment. Understanding the specific mechanisms by which NIBS exerts its effects on the visual system is also a challenge, and may vary depending on the NIBS technique and individual characteristics. As more evidence becomes available, it can guide the design of optimal NIBS protocols for larger clinical trials in targeted populations, ultimately advancing the field of vision rehabilitation.
Future directions
For NIBS to be a viable therapeutic intervention for vision rehabilitation, it should be able to induce long-lasting changes in behaviour and function, in either individuals with or without visual impairment. In terms of the underlying neurophysiology, by modulating mechanisms that control the strength of synaptic plasticity in the cortex, NIBS has the potential to fill a gap in the management and rehabilitation of visual impairments.
Despite the challenges that exist in the field, the field of NIBS for visual rehabilitation is rapidly advancing. Firstly, recent developments employ combination approaches, in which the potential benefits of combining NIBS with other rehabilitation methods, such as visual training, are being explored.
Secondly, there is growing recognition that optimal parameters for NIBS may vary depending on individual characteristics, such as age, genetics, cognitive status, and the location and extent of the lesion or damage. Personalised approaches to NIBS, which tailor the stimulation parameters to the individual, may lead to more targeted and effective interventions.
Third, new NIBS techniques with greater precision or deeper penetration of neural tissue than existing techniques are being developed. For example, high-definition TMS and tES are emerging techniques that may have potential applications in visual rehabilitation.
Lastly, there is increasing interest in understanding the long-term effects of NIBS on visual function. While many studies have demonstrated short-term improvements in visual acuity or other outcomes, it is not yet clear how long these effects last or whether they lead to meaningful improvements in daily life.
Overall, the field of NIBS for visual rehabilitation is developing rapidly, and there is significant potential for future research and clinical applications. As the field evolves, it is likely that more targeted and effective NIBS interventions will be developed, leading to novel ways of managing visual disorders and improved outcomes for individuals with visual impairments or deficits.
Acknowledgements
InnoHK initiative and the Hong Kong Special Administrative Region Government.
Disclosure statement
Both authors are co-inventors of a patent that combines non-invasive brain stimulation and perceptual learning for the treatment of presbyopia.
Additional information
Funding
References
- Merton PA, Morton HB. Stimulation of the cerebral cortex in the intact human subject. Nature 1980; 285: 227. doi:10.1038/285227a0
- Barker AT, Jalinous R, Freeston IL. Non-invasive magnetic stimulation of human motor cortex. Lancet 1985; 1: 1106–1107. doi:10.1016/S0140-6736(85)92413-4
- Siebner HR, Funke K, Aberra AS et al. Transcranial magnetic stimulation of the brain: what is stimulated? – A consensus and critical position paper. Clin Neurophysiol 2022; 140: 59–97. doi:10.1016/j.clinph.2022.04.022
- Liebetanz D, Nitsche MA, Tergau F et al. Pharmacological approach to the mechanisms of transcranial DC-stimulation-induced after-effects of human motor cortex excitability. Brain 2002; 125: 2238–2247. doi:10.1093/brain/awf238
- Nitsche MA, Jaussi W, Liebetanz D et al. Consolidation of human motor cortical neuroplasticity by D-cycloserine. Neuropsychopharmacology 2004; 29: 1573–1578. doi:10.1038/sj.npp.1300517
- Huang YZ, Chen RS, Rothwell JC et al. The after-effect of human theta burst stimulation is NMDA receptor dependent. Clin Neurophysiol 2007; 118: 1028–1032. doi:10.1016/j.clinph.2007.01.021
- Ziemann U, Lonnecker S, Steinhoff BJ et al. Effects of antiepileptic drugs on motor cortex excitability in humans: a transcranial magnetic stimulation study. Ann Neurol 1996; 40: 367–378. doi:10.1002/ana.410400306
- Stagg CJ, Bestmann S, Constantinescu AO et al. Relationship between physiological measures of excitability and levels of glutamate and GABA in the human motor cortex. J Physiol 2011; 589: 5845–5855. doi:10.1113/jphysiol.2011.216978
- Bachtiar V, Near J, Johansen-Berg H et al. Modulation of GABA and resting state functional connectivity by transcranial direct current stimulation. Elife 2015; 4: e08789. doi:10.7554/eLife.08789
- Fertonani A, Pirulli C, Miniussi C. Random noise stimulation improves neuroplasticity in perceptual learning. J Neurosci 2011; 31: 15416–15423. doi:10.1523/JNEUROSCI.2002-11.2011
- Antal A, Paulus W. Transcranial alternating current stimulation (tACS). Front Hum Neurosci 2013; 7: 317. doi:10.3389/fnhum.2013.00317
- Kanai R, Paulus W, Walsh V. Transcranial alternating current stimulation (tACS) modulates cortical excitability as assessed by TMS-induced phosphene thresholds. Clin Neurophysiol 2010; 121: 1551–1554. doi:10.1016/j.clinph.2010.03.022
- Liu A, Voroslakos M, Kronberg G et al. Immediate neurophysiological effects of transcranial electrical stimulation. Nat Commun 2018; 9: 5092. doi:10.1038/s41467-018-07233-7
- Bello UM, Wang J, Park ASY et al. Can visual cortex non-invasive brain stimulation improve normal visual function? A systematic review and meta-analysis. Front Neurosci 2023; 17: 1119200. doi:10.3389/fnins.2023.1119200
- Cohen SL, Bikson M, Badran BW et al. A visual and narrative timeline of US FDA milestones for Transcranial Magnetic Stimulation (TMS) devices. Brain Stimul 2022; 15: 73–75. doi:10.1016/j.brs.2021.11.010
- Perin C, Vigano B, Piscitelli D et al. Non-invasive current stimulation in vision recovery: a review of the literature. Restor Neurol Neurosci 2020; 38: 239–250. doi:10.3233/RNN-190948
- Marg E, Rudiak D. Phosphenes induced by magnetic stimulation over the occipital brain: description and probable site of stimulation. Optom Vis Sci 1994; 71: 301–311. doi:10.1097/00006324-199405000-00001
- Kastner S, Demmer I, Ziemann U. Transient visual field defects induced by transcranial magnetic stimulation over human occipital pole. Exp Brain Res 1998; 118: 19–26. doi:10.1007/s002210050251
- Corthout E, Uttl B, Walsh V et al. Timing of activity in early visual cortex as revealed by transcranial magnetic stimulation. Neuroreport 1999; 10: 2631–2634. doi:10.1097/00001756-199908200-00035
- Pascual-Leone A, Valls-Sole J, Wassermann EM et al. Responses to rapid-rate transcranial magnetic stimulation of the human motor cortex. Brain 1994; 117: 847–858. doi:10.1093/brain/117.4.847
- Huang YZ, Edwards MJ, Rounis E et al. Theta burst stimulation of the human motor cortex. Neuron 2005; 45: 201–206. doi:10.1016/j.neuron.2004.12.033
- Moreno-Duarte I, Gebodh N, Schestatsky P et al. Chapter 2 - transcranial electrical stimulation: transcranial direct current stimulation (tDCS), transcranial alternating current stimulation (tACS), transcranial pulsed current stimulation (tPCS), and transcranial random noise stimulation (tRNS). In: Cohen Kadosh R, editor. The stimulated brain. San Diego: Academic Press; 2014. p. 35–59.
- Nitsche MA, Paulus W. Excitability changes induced in the human motor cortex by weak transcranial direct current stimulation. J Physiol 2000; 527: 633–639. doi:10.1111/j.1469-7793.2000.t01-1-00633.x
- Kanai R, Chaieb L, Antal A et al. Frequency-dependent electrical stimulation of the visual cortex. Curr Biol 2008; 18: 1839–1843. doi:10.1016/j.cub.2008.10.027
- Lee A, Yau CE, Mai AS et al. Transcranial alternating current stimulation and its effects on cognition and the treatment of psychiatric disorders: a systematic review and meta-analysis. Ther Adv Chronic Dis 2022; 13: 20406223221140390. doi:10.1177/20406223221140390
- Terney D, Chaieb L, Moliadze V et al. Increasing human brain excitability by transcranial high-frequency random noise stimulation. J Neurosci 2008; 28: 14147–14155. doi:10.1523/JNEUROSCI.4248-08.2008
- Stagg CJ, Nitsche MA. Physiological basis of transcranial direct current stimulation. Neuroscientist 2011; 17: 37–53. doi:10.1177/1073858410386614
- Shafi MM, Westover MB, Fox MD et al. Exploration and modulation of brain network interactions with noninvasive brain stimulation in combination with neuroimaging. Eur J Neurosci 2012; 35: 805–825. doi:10.1111/j.1460-9568.2012.08035.x
- Thompson B, Mansouri B, Koski L et al. Brain plasticity in the adult: modulation of function in amblyopia with rTMS. Curr Biol 2008; 18: 1067–1071. doi:10.1016/j.cub.2008.06.052
- Clavagnier S, Thompson B, Hess RF. Long lasting effects of daily theta burst rTMS sessions in the human amblyopic cortex. Brain Stimul 2013; 6: 860–867. doi:10.1016/j.brs.2013.04.002
- Spiegel DP, Byblow WD, Hess RF et al. Anodal transcranial direct current stimulation transiently improves contrast sensitivity and normalizes visual cortex activation in individuals with amblyopia. J Neurol Rehabil 2013; 27: 760–769. doi:10.1177/1545968313491006
- Tuna AR, Pinto N, Brardo FM et al. Transcranial magnetic stimulation in adults with amblyopia. J Neuroophthalmol 2020; 40: 185–192. doi:10.1097/WNO.0000000000000828
- Plow EB, Obretenova SN, Halko MA et al. Combining visual rehabilitative training and noninvasive brain stimulation to enhance visual function in patients with hemianopia: a comparative case study. PM R 2011; 3: 825–835. doi:10.1016/j.pmrj.2011.05.026
- Raveendran RN, Chow A, Tsang K et al. Reduction of collinear inhibition in observers with central vision loss using anodal transcranial direct current stimulation: a case series. Brain Stimul 2021; 14: 207–208. doi:10.1016/j.brs.2020.12.015
- Hubel DH, Wiesel TN. The period of susceptibility to the physiological effects of unilateral eye closure in kittens. J Physiol 1970; 206: 419–436. doi:10.1113/jphysiol.1970.sp009022
- Hensch TK, Quinlan EM. Critical periods in amblyopia. Vis Neurosci 2018; 35: E014. doi:10.1017/S0952523817000219
- Repka MX. Amblyopia outcomes through clinical trials and practice measurement: room for improvement: the LXXVII Edward Jackson Memorial Lecture. Am J Ophthalmol 2020; 219: A1–A26. doi:10.1016/j.ajo.2020.07.053
- Stryker MP, Lowel S. Amblyopia: new molecular/pharmacological and environmental approaches. Vis Neurosci 2018; 35: E018. doi:10.1017/S0952523817000256
- Reinhart RM, Xiao W, McClenahan LJ et al. Electrical stimulation of visual cortex can immediately improve spatial vision. Current Biology 2016; 26: 1867–1872. doi:10.1016/j.cub.2016.05.019
- Kraft A, Roehmel J, Olma MC et al. Transcranial direct current stimulation affects visual perception measured by threshold perimetry. Exp Brain Res 2010; 207: 283–290. doi:10.1007/s00221-010-2453-6
- Behrens JR, Kraft A, Irlbacher K et al. Long-lasting enhancement of visual perception with repetitive noninvasive transcranial direct current stimulation. Front Cell Neurosci 2017; 11: 238. doi:10.3389/fncel.2017.00238
- Battaglini L, Contemori G, Penzo S et al. tRNS effects on visual contrast detection. Neurosci Lett 2020; 717: 134696. doi:10.1016/j.neulet.2019.134696
- Zito GA, Senti T, Cazzoli D et al. Cathodal HD-tDCS on the right V5 improves motion perception in humans. Front Behav Neurosci 2015; 9: 257. doi:10.3389/fnbeh.2015.00257
- Battaglini L, Noventa S, Casco C. Anodal and cathodal electrical stimulation over V5 improves motion perception by signal enhancement and noise reduction. Brain Stimul 2017; 10: 773–779. doi:10.1016/j.brs.2017.04.128
- Wu D, Li C, Liu N et al. Visual motion perception improvements following direct current stimulation over V5 are dependent on initial performance. Exp Brain Res 2020; 238: 2409–2416. doi:10.1007/s00221-020-05842-7
- Dong G, Wang Y, Chen X. Anodal occipital tDCS enhances spontaneous alpha activity. Neurosci Lett 2020; 721: 134796. doi:10.1016/j.neulet.2020.134796
- Nakazono H, Ogata K, Takeda A et al. Transcranial alternating current stimulation of α but not β frequency sharpens multiple visual functions. Brain Stimul 2020; 13: 343–352. doi:10.1016/j.brs.2019.10.022
- MacDermott AB, Mayer ML, Westbrook GL et al. NMDA-receptor activation increases cytoplasmic calcium concentration in cultured spinal cord neurones. Nature 1986; 321: 519–522. doi:10.1038/321519a0
- Woo NH, Teng HK, Siao CJ et al. Activation of p75NTR by proBDNF facilitates hippocampal long-term depression. Nat Neurosci 2005; 8: 1069–1077. doi:10.1038/nn1510
- Cheeran B, Talelli P, Mori F et al. A common polymorphism in the brain-derived neurotrophic factor gene (BDNF) modulates human cortical plasticity and the response to rTMS. J Physiol 2008; 586: 5717–5725. doi:10.1113/jphysiol.2008.159905
- Fritsch B, Reis J, Martinowich K et al. Direct current stimulation promotes BDNF-dependent synaptic plasticity: potential implications for motor learning. Neuron 2010; 66: 198–204. doi:10.1016/j.neuron.2010.03.035
- Sale A, Berardi N, Spolidoro M et al. GABAergic inhibition in visual cortical plasticity. Front Cell Neurosci 2010; 4: 10. doi:10.3389/conf.fnins.2010.15.00035
- Karmarkar UR, Dan Y. Experience-dependent plasticity in adult visual cortex. Neuron 2006; 52: 577–585. doi:10.1016/j.neuron.2006.11.001
- Allen CP, Dunkley BT, Muthukumaraswamy SD et al. Enhanced awareness followed reversible inhibition of human visual cortex: a combined TMS, MRS and MEG study. PLOS ONE 2014; 9: e100350. doi:10.1371/journal.pone.0100350
- Rafique SA, Steeves JKE. Assessing differential effects of single and accelerated low-frequency rTMS to the visual cortex on GABA and glutamate concentrations. Brain Behav 2020; 10: e01845. doi:10.1002/brb3.1845
- Stoby KS, Rafique SA, Oeltzschner G et al. Continuous and intermittent theta burst stimulation to the visual cortex do not alter GABA and glutamate concentrations measured by magnetic resonance spectroscopy. Brain Behav 2022; 12: e2478. doi:10.1002/brb3.2478
- Li H, Heise KF, Chalavi S et al. The role of MRS-assessed GABA in human behavioral performance. Prog Neurobiol 2022; 212: 102247. doi:10.1016/j.pneurobio.2022.102247
- Sale A, Maya Vetencourt JF, Medini P et al. Environmental enrichment in adulthood promotes amblyopia recovery through a reduction of intracortical inhibition. Nat Neurosci 2007; 10: 679–681. doi:10.1038/nn1899
- Bulsara A, Jacobs EW, Zhou T et al. Stochastic resonance in a single neuron model: theory and analog simulation. J Theor Biol 1991; 152: 531–555. doi:10.1016/S0022-5193(05)80396-0
- van der Groen O, Wenderoth N. Transcranial random noise stimulation of visual cortex: stochastic resonance enhances central mechanisms of perception. J Neurosci 2016; 36: 5289–5298. doi:10.1523/JNEUROSCI.4519-15.2016
- van der Groen O, Tang MF, Wenderoth N et al. Stochastic resonance enhances the rate of evidence accumulation during combined brain stimulation and perceptual decision-making. PLOS Comput Biol 2018; 14: e1006301. doi:10.1371/journal.pcbi.1006301
- Donkor R, Silva AE, Teske C et al. Repetitive visual cortex transcranial random noise stimulation in adults with amblyopia. Sci Rep 2021; 11: 3029. doi:10.1038/s41598-020-80843-8
- Galea LA, Spritzer MD, Barker JM et al. Gonadal hormone modulation of hippocampal neurogenesis in the adult. Hippocampus 2006; 16: 225–232. doi:10.1002/hipo.20154
- McEwen BS. How do sex and stress hormones affect nerve cells? Ann N Y Acad Sci 1994; 743: 1–16; discussion 17–18. doi:10.1111/j.1749-6632.1994.tb55784.x
- Chaieb L, Antal A, Paulus W. Gender-specific modulation of short-term neuroplasticity in the visual cortex induced by transcranial direct current stimulation. Vis Neurosci 2008; 25: 77–81. doi:10.1017/S0952523808080097
- Tecchio F, Zappasodi F, Pasqualetti P et al. Age dependence of primary motor cortex plasticity induced by paired associative stimulation. Clin Neurophysiol 2008; 119: 675–682. doi:10.1016/j.clinph.2007.10.023
- Inghilleri M, Conte A, Curra A et al. Ovarian hormones and cortical excitability. An rTMS study in humans. Clin Neurophysiol 2004; 115: 1063–1068. doi:10.1016/j.clinph.2003.12.003
- Antal A, Chaieb L, Moliadze V et al. Brain-derived neurotrophic factor (BDNF) gene polymorphisms shape cortical plasticity in humans. Brain Stimul 2010; 3: 230–237. doi:10.1016/j.brs.2009.12.003
- Lulic T, El-Sayes J, Fassett HJ et al. Physical activity levels determine exercise-induced changes in brain excitability. PLOS ONE 2017; 12: e0173672. doi:10.1371/journal.pone.0173672
- Hendy AM, Andrushko JW, Della Gatta PA et al. Acute effects of high-intensity aerobic exercise on motor cortical excitability and inhibition in sedentary adults. Front Psychol 2022; 13: 814633. doi:10.3389/fpsyg.2022.814633
- Sale MV, Ridding MC, Nordstrom MA. Cortisol inhibits neuroplasticity induction in human motor cortex. J Neurosci 2008; 28: 8285–8293. doi:10.1523/JNEUROSCI.1963-08.2008
- Stefan K, Wycislo M, Classen J. Modulation of associative human motor cortical plasticity by attention. J Neurophysiol 2004; 92: 66–72. doi:10.1152/jn.00383.2003
- Conte A, Gilio F, Iezzi E et al. Attention influences the excitability of cortical motor areas in healthy humans. Exp Brain Res 2007; 182: 109–117. doi:10.1007/s00221-007-0975-3
- Todd G, Kimber TE, Ridding MC et al. Reduced motor cortex plasticity following inhibitory rTMS in older adults. Clin Neurophysiol 2010; 121: 441–447. doi:10.1016/j.clinph.2009.11.089
- Rossi S, Hallett M, Rossini PM et al. Safety, ethical considerations, and application guidelines for the use of transcranial magnetic stimulation in clinical practice and research. Clin Neurophysiol 2009; 120: 2008–2039. doi:10.1016/j.clinph.2009.08.016
- Antal A, Alekseichuk I, Bikson M et al. Low intensity transcranial electric stimulation: safety, ethical, legal regulatory and application guidelines. Clin Neurophysiol 2017; 128: 1774–1809. doi:10.1016/j.clinph.2017.06.001
- Rossi S, Antal A, Bestmann S et al. Safety and recommendations for TMS use in healthy subjects and patient populations, with updates on training, ethical and regulatory issues: expert guidelines. Clin Neurophysiol 2021; 132: 269–306. doi:10.1016/j.clinph.2020.10.003
- Rossi S, Hallett M, Rossini PM et al. Screening questionnaire before TMS: an update. Clin Neurophysiol 2011; 122: 1686. doi:10.1016/j.clinph.2010.12.037
- Kasschau M, Reisner J, Sherman K et al. Transcranial direct current stimulation is feasible for remotely supervised home delivery in multiple sclerosis. Neuromodulation 2016; 19: 824–831. doi:10.1111/ner.12430
- de Winckel AV, Carey JR, Bisson TA et al. Home-based transcranial direct current stimulation plus tracking training therapy in people with stroke: an open-label feasibility study. J NeuroEng Rehabil 2018; 15: 83. doi:10.1186/s12984-018-0427-2
- Charvet LE, Shaw MT, Bikson M et al. Supervised transcranial direct current stimulation (tDCS) at home: a guide for clinical research and practice. Brain Stimul 2020; 13: 686–693. doi:10.1016/j.brs.2020.02.011
- Ding Z, Li J, Spiegel DP et al. The effect of transcranial direct current stimulation on contrast sensitivity and visual evoked potential amplitude in adults with amblyopia. Sci Rep 2016; 6: 19280. doi:10.1038/srep19280
- Bocci T, Nasini F, Caleo M et al. Unilateral application of cathodal tDCS reduces transcallosal inhibition and improves visual acuity in amblyopic patients. Front Behav Neurosci 2018; 12: 109. doi:10.3389/fnbeh.2018.00109
- Plow EB, Obretenova SN, Jackson ML et al. Temporal profile of functional visual rehabilitative outcomes modulated by transcranial direct current stimulation. Neuromodulation 2012; 15: 367–373. doi:10.1111/j.1525-1403.2012.00440.x
- Plow EB, Obretenova SN, Fregni F et al. Comparison of visual field training for hemianopia with active versus sham transcranial direct cortical stimulation. J Neurol Rehabil 2012; 26: 616–626. doi:10.1177/1545968311431963
- Alber R, Moser H, Gall C et al. Combined transcranial direct current stimulation and vision restoration training in subacute stroke rehabilitation: a pilot study. PM R 2017; 9: 787–794. doi:10.1016/j.pmrj.2016.12.003
- Larcombe SJ, Kulyomina Y, Antonova N et al. Visual training in hemianopia alters neural activity in the absence of behavioural improvement: a pilot study. Ophthalmic Physiol Opt 2018; 38: 538–549. doi:10.1111/opo.12584
- Raty S, Borrmann C, Granata G et al. Non-invasive electrical brain stimulation for vision restoration after stroke: an exploratory randomized trial (REVIS). Restor Neurol Neurosci 2021; 39: 221–235. doi:10.3233/RNN-211198
- Silva AE, Lyu A, Leat SJ et al. A differential effect of visual cortex tDCS on reading of English and Chinese in patients with central vision loss. Brain Stimul 2022; 15: 1215–1217. doi:10.1016/j.brs.2022.08.016
- Spiegel DP, Li J, Hess RF et al. Transcranial direct current stimulation enhances recovery of stereopsis in adults with amblyopia. Neurotherapeutics 2013; 10: 831–839. doi:10.1007/s13311-013-0200-y
- Murphy TH, Corbett D. Plasticity during stroke recovery: from synapse to behaviour. Nat Rev Neurosci 2009; 10: 861–872. doi:10.1038/nrn2735
- Maniglia M, Pavan A, Cuturi LF et al. Reducing crowding by weakening inhibitory lateral interactions in the periphery with perceptual learning. PLOS ONE 2011; 6: e25568. doi:10.1371/journal.pone.0025568
- Raveendran RN, Tsang K, Tiwana D et al. Anodal transcranial direct current stimulation reduces collinear lateral inhibition in normal peripheral vision. PLOS ONE 2020; 15: e0232276. doi:10.1371/journal.pone.0232276