Abstract
Blood lipid management is a key approach in the prevention of chronic kidney disease (CKD). Remnant cholesterol (RC) plays an important role in the development of multiple diseases via chronic inflammation. The aim of our study was to determine the relationship between RC and CKD and explore the role of inflammation in this relationship. The 7696 subjects from the Chinese Health and Nutrition Survey were divided into four subgroups according to the quartile of RC. The estimated glomerular filtration rate was calculated using the CKD Epidemiology Collaboration equation. Fasting RC was calculated as total cholesterol minus low-density lipoprotein cholesterol and high-density lipoprotein cholesterol. Logistic regression analysis was employed to evaluate the relationships between RC and CKD. Mediation analysis was undertaken to identify potential mediators of high-sensitivity C-reactive protein (hs-CRP) and white blood cells (WBCs). Of all participants, the mean age was 51 years, and the male accounted for 47.8%. The multivariable-adjusted odds ratios (95% CIs) for the highest versus lowest quartile of remnant cholesterol were 1.40 (1.10–1.78, p for trend = 0.006) for CKD. RC and preinflammatory markers have combined effect on CKD. The preinflammatory state, presented by increased hs-CRP or WBCs, partially mediated the association between RC and CKD with proportion of 10.14% (p = 0.002) and 11.65% (p = 0.012), respectively. In conclusion, this study suggested a positive relationship between RC and CKD, which was partially mediated by preinflammatory state. These findings highlight the importance of RC and inflammation in renal dysfunction.
What is already known on this subject?: Dyslipidemia plays an important role in the development of chronic kidney disease (CKD). Remnant cholesterol (RC), as a triglyceride-rich particle, can contribute to target organ damage, primarily through inflammatory pathways. However, the relationship between RC and CKD in the community-dwelling population, particularly the role of inflammation, is not yet fully understood.
What do the results of this study add?: This study shows that RC was significantly associated with CKD. RC and preinflammatory status exhibit a combined effect on CKD. Preinflammatory state, presented by increased high-sensitivity C-reactive protein or white blood cells, partially mediated the association between RC and CKD.
What are the implications of these findings for clinical practice and/or further research?: The study provides us with a better understanding of the role of RC and inflammation in kidney dysfunction and raises the awareness of RC in the management of CKD.
IMPACT STATEMENT
Introduction
Chronic kidney disease (CKD), a widespread disease that manifests as decreased renal function, is increasingly prevalent in China, affecting about 10% of the adult population [Citation1]. CKD is often asymptomatic, and there is very limited treatment to help preclude its progression. Therefore, identifying and managing risk factors in patients with early CKD may slow down the progression to end-stage renal disease.
Hyperlipidemia has a multifaceted impact on kidney dysfunction and its subsequent complications [Citation2]. The lipid profile of CKD patients is typically characterized by increased triglyceride-rich lipoprotein particles (TRLs), elevated low-density lipoprotein cholesterol (LDL-C) and decreased high-density lipoprotein cholesterol (HDL-C) [Citation3,Citation4]. These lipid abnormalities also contribute to the progression of renal insufficiency as confirmed by epidemiological evidence [Citation5–7]. Experimental studies have also shown that in the context of hyperlipidemia, increased glomerulosclerosis and tubulointerstitial injury promote the development and progression of renal dysfunction [Citation8,Citation9]. Individuals with familial hypercholesterolemia have an increased risk of CKD [Citation10]. In-depth research on dyslipidemia, especially cholesterol abnormalities, may have great significance for the prevention of CKD.
Remnant cholesterol (RC), a novel lipoprotein agent, describes the cholesterol content of TRLs that consists of very low-density lipoprotein (VLDL), intermediate-density lipoprotein (IDL), and chylomicron remnants [Citation11]. As one of the typical blood lipid disorders in CKD patients, elevated RC has received much attention in recent years [Citation3,Citation12]. However, although previous studies have investigated the association of RC with CKD, the evidence remains controversial [Citation5,Citation12–21]. These inconsistent effects may be more or less limited by sample size, different study locations, confounding factors, and the effect of triglycerides (TGs) levels that are closely related to RC. Whether the relationship between RC and CKD still exists in the more extensive Chinese community population is still unknown. Furthermore, lipid disorders have been observed in CKD patients in relation to inflammatory and oxidative processes [Citation22,Citation23]. Unlike other types of cholesterol, elevated RC is more likely to cause inflammation, which promotes disease progression [Citation24,Citation25]. For that reason, it is critical to elucidate the relationship between RC and CKD in general populations and the role of inflammation in the relationship between RC and CKD.
Based on the established evidence of blood lipid disorder and the progression of renal dysfunction, we aimed to retrospectively investigate whether RC levels are positively associated with prevalent CKD in the Chinese Health and Nutrition Survey (CHNS) and to elucidate the magnitude of preinflammatory state mediation in this relationship.
Materials and methods
Study setting and population
This study used data from the CHNS, an ongoing longitudinal community-based cohort study carried out by the national and local governments of China that included data for more than 15,000 individuals in approximately nine provinces. Trained researchers conducted household surveys using standard questionnaires called ‘Adult Survey’, which was designed and permitted by CHNS and is available in both Chinese and English [Citation26]. Face-to-face interviews were performed to obtain information about participants. Study details were described previously [Citation27], and the relevant protocol was published elsewhere [Citation26]. Ultimately, 1,055 participants without RC or creatinine measurements, 741 participants who were under 18 years of age, and 57 pregnant females were excluded. After the exclusions, the present study included 7,696 participants from the 2009 CHNS wave (Supplementary Figure S1), and data including demographic information, healthy behaviors, health status, and laboratory examinations were presented. The subjects of this study were defined as general Chinese adults aged ≥18 years.
Study protocols and ethics approval were derived from the Institutional Review Committees of the University of North Carolina at Chapel Hill and the National Institute for Nutrition and Health at Beijing and were attributed to the two centers. The study was carried out under the guiding principles of the Declaration of Helsinki (as revised in Fortaleza, Brazil in October 2013). Each participant in our study was enrolled in China and assigned the informed consent.
Measures
RC (mmol/L) was calculated as total cholesterol (TC) (mmol/L) minus LDL-C (mmol/L) minus HDL-C (mmol/L) [Citation28], and blood samples were obtained in a fasting state. Renal function was estimated by the estimated glomerular filtration rate (eGFR) calculated using the CKD Epidemiology Collaboration (CKD-EPI) equation [Citation29]. CKD was diagnosed based on an eGFR of <60 mL·min−1·1.73 m−2.
Definitions
Height and weight were measured while the subjects were wearing light clothing and standing without shoes. Body mass index (BMI) was calculated as weight (kg)/height (m)2. Health behaviors (smoking and alcohol consumption), education levels and history of hypertension were self-reported. Smoking was defined as any previous smoking (yes/no), and alcohol consumption was defined as alcohol consumption greater than three times per week (yes/no). Diabetes was defined according to the American Diabetes Association criteria [Citation30].
From the fasting blood samples, hemoglobin and white blood cells (WBCs) were measured with a Beckman Coulter LH751 (Beckman Coulter, USA). Creatinine, uric acid, blood urea nitrogen (BUN), fasting blood glucose, HDL-C, LDL-C, TGs, TC, apolipoprotein A (apoA), and apolipoprotein B (apoB) were measured using a Hitachi 7600 machine (Randox, UK and Kyowa, Japan). Glycosylated hemoglobin A1c (HbA1c) was detected by an HLC-723 G7/D10/PDQ A1c (Tosoh, Japan/Bio-Rad, USA/Primus, USA), high-sensitivity C-reactive protein (hs-CRP) was measured by a Hitachi 7600 (Denka Seiken, Japan), and elevated hs-CRP was indicated as ≥ 5 mg/L. Based on previous studies, hs-CRP and WBCs were identified as markers for preinflammatory states [Citation31,Citation32].
Statistical analyses
Participant characteristics are described according to the quartiles of RC. Continuous variables are expressed as the means ± standard deviations for normal distributions or medians and interquartile ranges (25% to 75%) for skewed distributions, and categorical variables are presented as relative frequencies (percentages). Student’s t-test for normally distributed data, the Mann–Whitney U-rank test for nonnormally distributed data and the chi-squared test/Fisher’s exact test for categorical variables were used to discriminate significant differences between the groups. P values for trends were calculated with each quartile of the RC taken as a unit by using linear regression analyses and the Wald chi-squared test for trend analysis of continuous and categorical variables across the four groups.
Associations of RC with hs-CRP and WBCs was shown by generalized additive models, adjusting for age and sex. The independent association of RC with CKD was evaluated using logistic models with odds ratios (ORs) and 95% confidence intervals (CIs). The combined effects of RC, hs-CRP and WBCs on CKD were also explored. Potential covariates that were significant in the baseline comparison without collinearity or that were considered of clinical importance were included in the multivariate models. Four main models were established for covariate adjustment: Model 1, unadjusted; Model 2, adjusted for age, sex, and BMI; Model 3, adjusted for variables in Model 2, educational level, residence, smoking, and alcohol consumption; and Model 4, adjusted for variables in Model 3, hypertension and diabetes. The Akaike information criterion (AIC) and Bayesian information criterion (BIC) for the four models in our study were calculated.
Subgroup analysis was employed to examine the effect of RC on CKD in the various subgroups, including age (</≥ 65 years), sex (male/female), hypertension (yes/no), diabetes (yes/no), smoking (yes/no), alcohol consumption (yes/no), educational level (junior high school and below/high school and above) and residence (urban/rural) subgroups. The modifications and interactions of the subgroups were tested using the likelihood ratio test.
Mediation analysis refers to the determination of the mediating effect of the mediator through the direct effect, indirect effect, and total effect on the dependent variable from both independent and mediator variables. We investigated whether the association between RC and CKD was mediated by WBCs and hs-CRP, adjusted for the covariate in Model 4. In our research, the total exposure-outcome (RC-CKD) effect consisted of a direct effect (RC-CKD) and an indirect effect by mediation variable (RC-Elevated hs-CRP/WBC-CKD). Regression models were used to detect the mediating role of inflammatory markers on the association of RC with CKD in all participants through 10,000 sample bootstraps. Each regression model consists of three equations. Formula 1 regresses the mediator (Elevated hs-CRP/WBC) on the independent variable (RC). Formula 2 regresses the dependent variable (CKD) on the mediator (Elevated hs-CRP/WBC). Formula 3′ regresses the dependent variable (CKD) on the independent variable (RC) for direct effect. Formula 3 regresses the dependent variable (CKD) on the independent variable (RC) for total effect. The percentage of the total effect contributed by the mediator is calculated as follows: the standardized coefficients of the Formula 1 × the standardized coefficients of Formula 2/Formula 3 × 100%. With the inflammatory markers introduced, a mediation effect existed when the effect of RC on CKD was reduced to 0 (complete mediation) or different from 0 (partial mediation). To clarify the above relationship, a schematic diagram was plotted (Supplementary Figure S2).
Several sensitivity analyses were carried out to test the robustness of the results. First, a multivariate adjusted model considering conventional cholesterol and TGs was conducted. Second, the association between RC and eGFR instead of CKD was elucidated by a multivariate adjusted linear regression model, expressed as β coefficients and 95% CIs. The proportion of missing data in the analytic sample was not greater than 2%, and missing data were then interpolated using the method of last observation carried forward or using the means and medians for continuous variables and skewed variables. Comparisons where P was < 0.05 (two-sided) were considered to be statistically significant. All analyses were performed with Stata 15.0, R (version 3.4.3) and EmpowerStats (http://www.empowerstats.com, X&Y Solutions, Inc., Boston, MA, USA).
Results
Baseline characteristics
There were 3,682 men and 4,014 women aged 18–99 years included in this study. The median RC level was 0.37 mmol/L (IQR: 0.20–0.65 mmol/L). The baseline characteristics of the study participants by quartile of RC are shown in . Compared to those in the lowest quartile (Q1) of residual cholesterol, participants with the highest RC levels (Q4) were more likely to be male; to have a higher BMI, education level, uric acid, TGs, TC, ApoB, hs-CRP, and WBCs; and to have a higher prevalence of hypertension and diabetes but were more likely to have a lower level of HDL-C. Based on whether CKD existed, a comparison of the two groups is also presented (Supplementary Table S1). The prevalence of CKD (eGFR < 60 mL·min−1·1.73 m−2) was 12.1% (932 patients), and the mean (SD) ages of the CKD and non-CKD groups were 68 (11) and 48 (14), respectively. A higher proportion of participants with CKD were female, had a low education level, had increased LDL-C, TC, hs-CRP, and WBCs and had a higher prevalence of hypertension and diabetes. TGs were not significantly different between the two groups. The correlation among the baseline characteristics was also calculated to identify potential collinearity (Supplementary Figure S3).
Table 1. Baseline information according to quartiles of RC.
Remnant cholesterol associated with hs-CRP and WBCs
In order to clarify the relationship between RC and preinflammatory markers, the dose–response relationships are presented in . After adjusting for age and sex, higher levels of RC were associated with higher levels of hs-CRP and WBCs. As RC levels increased, hs-CRP and WBCs initially increased rapidly, then began to fatten at the highest levels of RC.
Figure 1. Associations of RC with hs-CRP (a) and WBCs (b). Models adjusted for age and sex. Red curves are GAM functions with 95% confidence intervals in dotted line. Data outside 1% of the population was not included because the wide confidence intervals.
RC: remnant cholesterol; WBCs: white blood cells; hs-CRP: high-sensitivity C-reactive protein
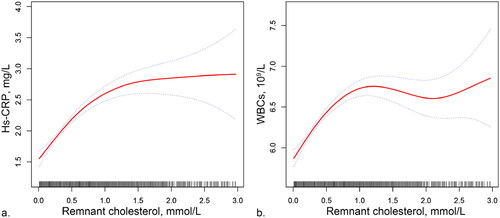
The association of RC with CKD
From the lowest quartile (Q1) to the highest quartile (Q4) of RC, the rates of CKD increased from 9.64% to 12.75% (p for trend: 0.002). Multivariable logistic regressions were performed, adjusting for age, sex, BMI, education, region, lifestyle factors, and comorbidities. As shown in , the ORs (95% CIs) for Q4 of RC were 1.37 (1.12–1.68), 1.54 (1.22–1.94), 1.53 (1.21–1.93), and 1.40 (1.10–1.78) for CKD in these models compared with Q1. Furthermore, the increase in each quartile in RC was also accompanied by an increased risk for CKD. The AIC and BIC values of the four models gradually declined, and Model 4 had the smallest AIC (4398.841) and BIC (4489.171), which was adopted for estimation (Supplementary Table S2). The subgroup analysis further verified the stability of the results. As shown in Supplementary Figure S4, the relationship between RC and CKD was consistent with the main findings in most strata. The sensitivity analysis showed that the associations of RC and CKD remained significant after further adjustment for TGs or conventional cholesterol levels based on model 4 (Supplementary Tables S3 and S4). RC was negatively correlated with eGFR after multivariate adjustment (Supplementary Table S5).
Table 2. ORs (95% CIs) For CKD in different quartiles of RC.
The combined effect of RC and preinflammatory markers on CKD
Patients in the highest tertile for RC, hs-CRP, and WBCs showed the most significant association with CKD (Supplementary Tables S6–S8). The combined effect of RC and pre-inflammatory markers on CKD is presented in . Compared to individuals in the lowest tertile of both RC and hs-CRP, the prevalence of CKD increased with higher levels of RC and hs-CRP (p for interaction: 0.827), with a multivariable adjusted OR of 2.47 (95% CI: 1.74–3.49) for those with both higher RC and hs-CRP. Similarly, the strongest association with CKD was observed in people with the highest tertile of both RC and WBCs (OR: 1.61, 95% CI: 1.17–2.22).
Figure 2. Combined effects of remnant cholesterol, hs-CRP and WBCs on CKD. a. Combined effects of RC and hs-CRP; b. Combined effects of RC and WBCs; c. Odds ratios of the combined effects. Model adjusted for age, sex and BMI, education, residence, smoking, and alcohol consumption, hypertension and diabetes.
RC: remnant cholesterol; CKD: chronic kidney disease; WBCs: white blood cells; hs-CRP: high-sensitivity C-reactive protein

Mediation analysis
We explored potential mediation effects in the relationship between RC and CKD and found that hs-CRP and WBCs significantly mediated the relationship between them. Significant indirect effects of hs-CRP and WBCs were observed between RC and the risk of CKD, with the two indices mediating 10.14% (p = 0.002) and 11.65% (p = 0.012) of the association after multivariate adjustment, respectively ().
Figure 3. Mediation analysis of the preinflammatory state on the association between RC and CKD. a. unadjusted; b. adjusted for age, sex, BMI, education, residence, smoking, alcohol consumption, hypertension and diabetes.
RC: remnant cholesterol; CKD: chronic kidney disease; hs-CRP: high-sensitivity C-reactive protein; WBCs: white blood cells
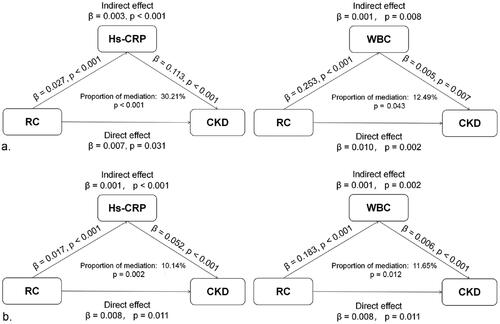
Discussion
In this study, we explored the relationship between RC and CKD in a wide range of community populations in China and came to the following conclusions: after adjusting for demographic characteristics, health behaviors, and comorbidities, RC and CKD maintained a significant association; dual elevated RC and preinflammatory markers were the strongest contributors to CKD; the preinflammatory state, indicated by elevated hs-CRP or WBCs, partially mediates the relationship between RC and CKD. Our findings not only clarify the relationship of RC and CKD prevalence in China, but also provide important insights into how RC affects CKD.
Previous epidemiological studies have shown that a drop in kidney function is associated with dyslipidemia, which may contribute to the development of CKD. Lipid and lipoprotein abnormalities, including TRLs, LDL-C, and/or HDL-C, related to RC have been shown to be good examples of metabolic characteristics in CKD patients [Citation3], while the role of RC in CKD remains controversial. Although many studies have evaluated the relationship between RC and CKD, few studies have been conducted in the general population, and the role of inflammation in that relationship is unknown. Single-center cross-sectional studies showed that higher RC was independently associated with proteinuria, prevalence, and severity of CKD [Citation12,Citation13,Citation17]. In addition, prospective cohort studies also found that an increase in RC was associated with the progression of renal impairment, suggesting that RC can be used as a monitoring indicator for the development of CKD [Citation16,Citation19]. Recent studies have indicated a significant association between elevated RC levels and CKD in populations with cardiovascular risk factors such as hypertension and diabetes [Citation18,Citation20,Citation21]. However, in the Chronic Renal Insufficiency Cohort study, TGs, TC, LDL-C, and HDL-C levels were not independently associated with the progression of kidney function decline [Citation5]. Another study also found no significant differences in VLDL and IDL levels between CKD patients and participants without CKD [Citation33]. Furthermore, Mendelian randomization studies of lipids and CKD also showed different results [Citation6,Citation34]. Our current studies based on the general population showed a strong positive relationship between RC and CKD independent of conventional cholesterol, suggesting that elevated RC may be related to the occurrence of CKD. Differences in study population, study area, measurement of RC and eGFR, and confounding factors may have contributed to the differences between the above studies and this research. Our study findings indicate that combining preinflammatory status with the evaluation of RC can better identify the risk of prevalent CKD. Previous research has established the association between RC and inflammation with CKD; however, the combined effect of these two factors has not been explored before. More importantly, the mediation analysis suggested that the preinflammatory state is potentially a pathway through which RC affects CKD.
Upon the association between RC and CKD, we found a combined effect between preinflammatory status and RC. Our findings are novel and provide insight into the role of inflammation in the pathogenic process of RC. A recent study of participants from the Copenhagen General Population Study, reported that dual elevated RC and low-grade inflammation pose the highest risk for atherosclerotic cardiovascular disease [Citation35]. Similarly, the current research confirms the combined effect of RC and preinflammatory status in CKD.
TRLs are the largest and least dense lipoprotein in plasma, mainly transporting TGs as energy to various target organs [Citation36]. Since TGs have been shown to be associated with the development of CKD in Chinese individuals [Citation7,Citation37,Citation38], RC, as the cholesterol component of TRLs, also has the potential to promote the development of CKD. However, until now, the mechanism of RC-induced CKD has been unclear. Nephron damage is the most common causes of kidney function decline [Citation39]. Residual cholesterol particles can be absorbed directly by mesangial cells in the glomerulus, leading to cytotoxicity, and they can also damage kidney function by stimulating microthrombus formation [Citation40,Citation41]. In addition, experimental studies have shown that excess cholesterol tends to accumulate in tissues, and podocytes and renal tubular epithelial cells may be the main targets of cholesterol deposition [Citation42,Citation43].
We found that the preinflammatory state played a mediating role in the relationship between RC and CKD, which is consistent with previous findings of the related inflammatory potential of RC in atherosclerosis [Citation25,Citation44,Citation45]. The mediation suggested that RC may cause structural and functional abnormalities of the kidney through renal tissue or systematic inflammation, inducing glomerulosclerosis, renal interstitial damage, and subsequent kidney function decline [Citation8]. Experiments have indicated that hyperlipidemia itself causes lipid vacuole formation and cholesterol ester deposition in the kidney, inducing kidney fibrosis mediated by the inflammatory pathway [Citation22,Citation46]. Inflammation can also exacerbate renal cholesteryl ester accumulation in vitro and in vivo, which in turn leads to elevated reactive oxygen species and endoplasmic reticulum stress markers and promotes renal lipid accumulation and foam cell formation [Citation47]. These evidences all support the important role of inflammation in RC and CKD; however, it is needed to recognize that traditional cholesterol dysfunction itself can also induce preinflammatory effects and may impact the progression of CKD. While our study elucidated the association between RC and preinflammatory status markers, preinflammatory status may also play a role in other traditional cholesterol-related pathways.
A recently proposed concept—that of cholesterol toxicity—has updated our understanding of the occurrence and development of many diseases associated with cholesterol [Citation48]. Therefore, the monitoring of RC as well as preinflammatory state, as a simple and important method, could further identify the risk of CKD at an early stage. Moreover, although guidelines now advocate lowering LDL-C in primary prevention to reduce the risk of cardiovascular disease in patients with CKD, the benefit of reducing RC remains unknown. Future studies are needed to provide evidence of improved kidney function and decreased cardiovascular risk resulting from RC reduction in patients with CKD.
We acknowledge several limitations in the present study. First, we could not draw causal conclusions regarding the association of RC and CKD due to the cross-sectional study design. Second, limited by the CHNS survey, we were unable to obtain information on proteinuria and lipid-lowering drug prescriptions to accurately screen those with early kidney injury and those treated with lipid-lowering therapy. In addition, there was no investigation about chronic glomerulonephritis in CHNS. The current study only assesses CKD based on eGFR and failed to further classify patients with CKD. Third, although we conducted our analyses via different methods to support our findings, we still realize that these results, as observed associations, may have been coincidental. Finally, mediation analysis assumed a certain sequence of effects, and our study design limited the directional determination of these effects, which served as a potential limitation that is generally encountered in cross-sectional studies.
Conclusions
In summary, the present study demonstrated that RC is associated with CKD in the community-dwelling population, independently of traditional CKD risk factors. The preinflammatory state, indicated by elevated WBCs and hs-CRP, partially mediated the association between RC and CKD. RC and inflammation play important roles in the development of CKD. Management strategies focusing on reducing RC and alleviating inflammation might offer a novel approach to prevent the progression of CKD in the future.
Ethical approval
Study protocols and ethics approval were derived from the Institutional Review Committees of the University of North Carolina at Chapel Hill and the National Institute for Nutrition and Health at Beijing and were attributed to the two centers. Each participant in our study was enrolled in China and assigned the informed consent.
Author contributions
YG Yuan, XM Hu, SH Zhang, WM Wang, BY Yu, YQ Ou, YL Zhou, and HJ Dong contributed to the study conception and design. YG Yuan, XM Hu, SH Zhang, WM Wang, and BY Yu collected and analyzed the data, and interpreted the results. YG Yuan and XM Hu drafted the manuscript and prepared tables and figures. SH Zhang, WM Wang, BY Yu, YQ Ou, YL Zhou, and HJ Dong revised the manuscript for important intellectual content. YQ Ou, YL Zhou, and HJ Dong provided study supervision and funding.
Supplemental Material
Download MS Word (1.3 MB)Acknowledgments
We would like to thank the Chinese Health and Nutrition Survey research team, the field team, and every respondent for their time and efforts that they have devoted to the Chinese Health and Nutrition Survey project.
Disclosure statement
No potential conflict of interest was reported by the author(s).
Data availability statement
The data in this study are available at: https://www.cpc.unc.edu/projects/china.
Additional information
Funding
References
- GBD Chronic Kidney Disease Collaboration. Global, regional, and national burden of chronic kidney disease, 1990-2017: a systematic analysis for the global burden of disease study 2017. Lancet. 2020;395(10225):1–10. doi: 10.1016/S0140-6736(20)30045-3.
- Mitrofanova A, Merscher S, Fornoni A. Kidney lipid dysmetabolism and lipid droplet accumulation in chronic kidney disease. Nat Rev Nephrol. 2023;19(10):629–645. doi: 10.1038/s41581-023-00741-w.
- Noels H, Lehrke M, Vanholder R, et al. Lipoproteins and fatty acids in chronic kidney disease: molecular and metabolic alterations. Nat Rev Nephrol. 2021;17(8):528–542. doi: 10.1038/s41581-021-00423-5.
- Bajaj A, Xie D, Cedillo-Couvert E, et al. Lipids, apolipoproteins, and risk of atherosclerotic cardiovascular disease in persons with CKD. Am J Kidney Dis. 2019;73(6):827–836. doi: 10.1053/j.ajkd.2018.11.010.
- Rahman M, Yang W, Akkina S, et al. Relation of serum lipids and lipoproteins with progression of CKD: the CRIC study. Clin J Am Soc Nephrol. 2014;9(7):1190–1198. doi: 10.2215/CJN.09320913.
- Miao L, Min Y, Qi B, et al. Causal effect between total cholesterol and HDL cholesterol as risk factors for chronic kidney disease: a mendelian randomization study. BMC Nephrol. 2021;22(1):35. doi: 10.1186/s12882-020-02228-3.
- Zhang YB, Sheng LT, Wei W, et al. Association of blood lipid profile with incident chronic kidney disease: a mendelian randomization study. Atherosclerosis. 2020;300:19–25. doi: 10.1016/j.atherosclerosis.2020.03.020.
- Jiang Z, Wang Y, Zhao X, et al. Obesity and chronic kidney disease. Am J Physiol Endocrinol Metab. 2023;324(1):E24–E41. doi: 10.1152/ajpendo.00179.2022.
- Jao TM, Nangaku M, Wu CH, et al. ATF6α downregulation of PPARα promotes lipotoxicity-induced tubulointerstitial fibrosis. Kidney Int. 2019;95(3):577–589. doi: 10.1016/j.kint.2018.09.023.
- Emanuelsson F, Nordestgaard BG, Benn M. Familial hypercholesterolemia and risk of peripheral arterial disease and chronic kidney disease. J Clin Endocrinol Metab. 2018;103(12):4491–4500. doi: 10.1210/jc.2018-01058.
- Varbo A, Nordestgaard BG. Remnant lipoproteins. Curr Opin Lipidol. 2017;28(4):300–307. doi: 10.1097/MOL.0000000000000429.
- Yan P, Xu Y, Miao Y, et al. Association of remnant cholesterol with chronic kidney disease in Middle-aged and elderly Chinese: a population-based study. Acta Diabetol. 2021;58(12):1615–1625. doi: 10.1007/s00592-021-01765-z.
- Li B, Wang A, Wang Y, et al. A study on the correlation between remnant cholesterol and urinary albumin to creatinine ratio in Chinese community adults: a report from the REACTION study. J Diabetes. 2020;12(12):870–880.
- Hayashi T, Hirano T, Taira T, et al. Remarkable increase of apolipoprotein B48 level in diabetic patients with end-stage renal disease. Atherosclerosis. 2008;197(1):154–158. doi: 10.1016/j.atherosclerosis.2007.03.015.
- Bermudez-Lopez M, Forne C, Amigo N, et al. An in-depth analysis shows a hidden atherogenic lipoprotein profile in non-diabetic chronic kidney disease patients. Expert Opin Ther Targets. 2019;23(7):619–630.
- Zhai Q, Dou J, Wen J, et al. Association between changes in lipid indexes and early progression of kidney dysfunction in participants with normal estimated glomerular filtration rate: a prospective cohort study. Endocrine. 2022;76(2):312–323. doi: 10.1007/s12020-022-03012-z.
- Yuan Y, Zhou X, Ji L. Association between remnant cholesterol level and severity of chronic kidney disease in patients with type 2 diabetes. J Diabetes Complications. 2023;37(9):108585. doi: 10.1016/j.jdiacomp.2023.108585.
- Yuan T, Ding C, Xie Y, et al. Association between remnant cholesterol and chronic kidney disease in chinese hypertensive patients. Front Endocrinol (Lausanne). 2023;14:1189574. doi: 10.3389/fendo.2023.1189574.
- He X, Zou R, Du X, et al. Association of remnant cholesterol with decreased kidney function or albuminuria: a population-based study in the U.S. Lipids Health Dis. 2024;23(1):2. doi: 10.1186/s12944-023-01995-w.
- Zhu W, Liu Q, Liu F, et al. High remnant cholesterol as a risk factor for developing chronic kidney disease in patients with prediabetes and type 2 diabetes: a cross-sectional study of a US population. Acta Diabetol. 2024;61(6):745. Published online March 4, doi: 10.1007/s00592-024-02249-6.
- Jang SY, Kang M, Song E, et al. Remnant cholesterol is an independent risk factor for the incidence of chronic kidney disease in newly-diagnosed type 2 diabetes: a nationwide population-based study. Diabetes Res Clin Pract. 2024;210:111639. doi: 10.1016/j.diabres.2024.111639.
- Yamamoto T, Takabatake Y, Takahashi A, et al. High-Fat Diet-Induced lysosomal dysfunction and impaired autophagic flux contribute to lipotoxicity in the kidney. J Am Soc Nephrol. 2017;28(5):1534–1551. doi: 10.1681/ASN.2016070731.
- Sun Y, Ge X, Li X, et al. High-fat diet promotes renal injury by inducing oxidative stress and mitochondrial dysfunction. Cell Death Dis. 2020;11(10):914. doi: 10.1038/s41419-020-03122-4.
- Bornfeldt KE, Linton MF, Fisher EA, et al. JCL roundtable: lipids and inflammation in atherosclerosis. J Clin Lipidol. 2021;15(1):3–17.
- Varbo A, Benn M, Tybjærg-Hansen A, et al. Elevated remnant cholesterol causes both low-grade inflammation and ischemic heart disease, whereas elevated low-density lipoprotein cholesterol causes ischemic heart disease without inflammation. Circulation. 2013;128(12):1298–1309. doi: 10.1161/CIRCULATIONAHA.113.003008.
- Zhang B, Zhai FY, Du SF, et al. The China health and nutrition survey, 1989-2011. Obes Rev. 2014;15 Suppl 1(0 1):2–7. doi: 10.1111/obr.12119.
- Hu X, Appleton AA, Ou Y, et al. Abdominal volume index trajectories and risk of diabetes mellitus: results from the China health and nutrition survey. J Diabetes Investig. 2022;13(5):868–877. doi: 10.1111/jdi.13733.
- Qian S, You S, Sun Y, et al. Remnant cholesterol and common carotid artery Intima-Media thickness in patients with ischemic stroke. Circ Cardiovasc Imaging. 2021;14(4):e010953.
- Horio M, Imai E, Yasuda Y, et al. Modification of the CKD epidemiology collaboration (CKD-EPI) equation for Japanese: accuracy and use for population estimates. Am J Kidney Dis. 2010;56(1):32–38. doi: 10.1053/j.ajkd.2010.02.344.
- American Diabetes Association Professional Practice Committee. 2. Classification and diagnosis of diabetes: standards of medical care in diabetes-2022. Diabetes Care. 2022;45(Suppl 1):S17–S38. doi: 10.2337/dc22-S002.
- Ion RM, Sibianu M, Hutanu A, et al. A comprehensive summary of the current understanding of the relationship between severe obesity, metabolic syndrome, and inflammatory status. J Clin Med. 2023;12(11):3818.
- Hotoura E, Giapros V, Kostoula A, et al. Pre-inflammatory mediators and lymphocyte subpopulations in preterm neonates with sepsis. Inflammation. 2012;35(3):1094–1101. doi: 10.1007/s10753-011-9416-3.
- Rysz-Gorzynska M, Gluba-Brzozka A, Banach M. High-Density lipoprotein and low-density lipoprotein subfractions in patients with chronic kidney disease. Curr Vasc Pharmacol. 2017;15(2):144–151. doi: 10.2174/1570161114666161003093032.
- Lanktree MB, Thériault S, Walsh M, et al. HDL cholesterol, LDL cholesterol, and triglycerides as risk factors for CKD: a mendelian randomization study. Am J Kidney Dis. 2018;71(2):166–172. doi: 10.1053/j.ajkd.2017.06.011.
- Doi T, Langsted A, Nordestgaard BG. Dual elevated remnant cholesterol and C-reactive protein in myocardial infarction, atherosclerotic cardiovascular disease, and mortality. Atherosclerosis. 2023;379:117141. doi: 10.1016/j.atherosclerosis.2023.05.010.
- Barter P. Lipoprotein metabolism and CKD: overview. Clin Exp Nephrol. 2014;18(2):243–246. doi: 10.1007/s10157-013-0866-9.
- Wang C, Wang L, Liang K, et al. Poor control of plasma triglycerides is associated with early decline of estimated glomerular filtration rates in New-Onset type 2 diabetes in China: results from a 3-Year Follow-Up study. J Diabetes Res. 2020;2020:3613041.
- Zhang L, Yuan Z, Chen W, et al. Serum lipid profiles, lipid ratios and chronic kidney disease in a chinese population. Int J Environ Res Public Health. 2014;11(8):7622–7635.
- Huang R, Fu P, Ma L. Kidney fibrosis: from mechanisms to therapeutic medicines. Signal Transduct Target Ther. 2023;8(1):129. doi: 10.1038/s41392-023-01379-7.
- Huang JK, Lee HC. Emerging evidence of pathological roles of very-low-density lipoprotein (VLDL). Int J Mol Sci. 2022;23(8):4300. doi: 10.3390/ijms23084300.
- Ding WY, Protty MB, Davies IG, et al. Relationship between lipoproteins, thrombosis, and atrial fibrillation. Cardiovasc Res. 2022;118(3):716–731. doi: 10.1093/cvr/cvab017.
- Ducasa GM, Mitrofanova A, Mallela SK, et al. ATP-binding cassette A1 deficiency causes cardiolipin-driven mitochondrial dysfunction in podocytes. J Clin Invest. 2019;129(8):3387–3400. doi: 10.1172/JCI125316.
- Honzumi S, Takeuchi M, Kurihara M, et al. The effect of cholesterol overload on mouse kidney and kidney-derived cells. Ren Fail. 2018;40(1):43–50. doi: 10.1080/0886022X.2017.1419974.
- Alyaydin E, Pogoda C, Dell Aquila A, et al. Cardiac allograft vasculopathy in a long-term follow-up after heart transplantation: role of remnant cholesterol in residual inflammation. Cardiol J. 2022;29(5):782–790. doi: 10.5603/CJ.a2022.0013.
- Varbo A, Nordestgaard BG. Remnant cholesterol and Triglyceride-Rich lipoproteins in atherosclerosis progression and cardiovascular disease. Arterioscler Thromb Vasc Biol. 2016;36(11):2133–2135. doi: 10.1161/ATVBAHA.116.308305.
- Yuan Q, Tang B, Zhang C. Signaling pathways of chronic kidney diseases, implications for therapeutics. Signal Transduct Target Ther. 2022;7(1):182. doi: 10.1038/s41392-022-01036-5.
- Zhong S, Zhao L, Li Q, et al. Inflammatory stress exacerbated mesangial foam cell formation and renal injury via disrupting cellular cholesterol homeostasis. Inflammation. 2015;38(3):959–971. doi: 10.1007/s10753-014-0058-0.
- Song Y, Liu J, Zhao K, et al. Cholesterol-induced toxicity: an integrated view of the role of cholesterol in multiple diseases. Cell Metab. 2021;33(10):1911–1925. doi: 10.1016/j.cmet.2021.09.001.