Abstract
Background
Chondrocyte viability, apoptosis, and migration are closely related to cartilage injury in osteoarthritis (OA) joints. Exosomes are identified as potential therapeutic agents for OA.
Objective
This study aimed to investigate the role of exosomes derived from osteocytes in OA, particularly focusing on their effects on cartilage repair and molecular mechanisms.
Methods
An injury cell model was established by treating chondrocytes with IL-1β. Cartilage repair was evaluated using cell counting kit-8, flow cytometry, scratch test, and Western Blot. Molecular mechanisms were analyzed using quantitative real-time PCR, bioinformatic analysis, and Western Blot. An OA mouse model was established to explore the role of exosomal DLX2 in vivo.
Results
Osteocyte-released exosomes promoted cell viability and migration, and inhibited apoptosis and extracellular matrix (ECM) deposition. Moreover, exosomes upregulated DLX2 expression, and knockdown of DLX2 activated the Wnt pathway. Additionally, exosomes attenuated OA in mice by transmitting DLX2.
Conclusion
Osteocyte-derived exosomal DLX2 alleviated IL-1β-induced cartilage repair and inactivated the Wnt pathway, thereby alleviating OA progression. The findings suggested that osteocyte-derived exosomes may hold promise as a treatment for OA.
Introduction
Osteoarthritis (OA) is a common joint disease that can significantly limit the activity of adults. It affects the lives of approximately 250 million people [Citation1]. Overloading and joint damage lead to cartilage destruction, which, if not properly managed, can result in OA and gradual degeneration as the condition progresses [Citation2,Citation3]. Current treatment options for cartilage injury include cartilage repair surgery, cartilage transplantation, and non-steroidal anti-inflammatory drugs [Citation4,Citation5]. However, OA cannot be fully cured. Chondrocytes, the only type of cells that make up cartilage tissues, change growth, differentiation, autophagy, and death when OA occurs [Citation6]. Therefore, restoring the normal function of chondrocytes is crucial for cartilage repair, understanding the pathogenesis of OA, and exploring new therapeutic strategies.
Exosomes are small extracellular vesicles, typically 30–150 nm in diameter, that are rich in various substances such as nucleic acids, proteins, saccharides, and liquid. They act as communicators in cell-to-cell communication [Citation7,Citation8]. Exosomes are reported to mediate cartilage repair processes by promoting cell growth, infiltration, matrix synthesis, and inhibiting regenerative immune responses [Citation9]. Exosomes have also been found to impede cartilage injury and effectively attenuate OA [Citation10]. Osteocytes are abundant bone cells of the osteoblast lineage that maintain bone homeostasis and regulate skeletal responses [Citation11,Citation12]. It has been revealed that osteocytes maintain cortices and trabeculae by balancing bone formation (osteogenic differentiation) and absorption (osteoclastogenesis). Exosomes are secreted by several bone cells, such as osteocytes, osteoblasts, osteoclasts, and bone marrow mesenchymal stem cells [Citation13]. Osteocyte-derived exosomes (OCY-exos) regulate intercellular communication in the bone microenvironment. Previous studies have identified that OCY-exos are involved in skeletal metabolism and diseases, such as periodontitis and benign prostatic hyperplasia [Citation14,Citation15]. However, the functions of OCY-exos in OA and the underlying mechanism have not been elucidated.
In the present study, we performed in vitro experiments to investigate the impact of exosomes derived from osteocytes on chondrocyte viability, apoptosis, migration, and matrix synthesis. Our findings suggest that the DLX2-mediated Wnt pathway plays a key role in regulating these effects. Moreover, in vivo experiments provide additional evidence of the involvement of exosomes in OA.
Materials and methods
Osteocyte culture
Mouse osteocyte cell line MLO-Y4 was acquired from Tongpai Biotechnology Co, Ltd (Shanghai, China) and cultured in α-MEM (Gibco, Grand Island, USA) containing 10% inactivated fetal bovine serum (FBS; Gibco) and 1% penicillin/streptomycin (Gibco) at 37 °C with 5% CO2.
Exosome isolation and authentication
Exosomes were isolated from MLO-Y4 cells as previously described [Citation15]. Briefly, the cell culture medium supernatant was collected after centrifuging at 3000g for 0.5 h. ExoQuick-TC exosome precipitation solution (System Biosciences, Mountain View, USA) was used for exosome extraction. Equal volumes of the supernatant and ExoQuick solution were incubated overnight at 4 °C. The exosomes were obtained by centrifugation at 1500g for 0.5 h and resuspended in PBS. The characteristics of OCY-exos were authenticated using transmission electron microscopy (TEM), nanoparticle tracking analysis (NTA), and Western Blot.
TEM
The exosomes were added to the carbon-pre-coated copper mesh. After fixing with 1% glutaraldehyde, the copper mesh was stained with 10 μL uranyl acetate for 30 s in the dark. After drying, the images of exosomes were photographed under a TEM at 120 kV.
NTA
The exosomes were diluted using PBS until there were 20–100 objects per frame. Then, the samples were analyzed using the Nanosight LM10 system (Nanosight Technology, Malvern) with the NTA analytical software (version 2.3).
Isolation and culture of chondrocytes
Primary chondrocytes were harvested from C57BL/6 mice. Briefly, the cartilage tissues were isolated from the knee joint of the right hindlegs and cut into pieces of less than 1 mm3. The tissues were digested with 0.2% trypsin (Gibco) at 37 °C for 0.5 h and treated with 0.2% collagenase II (Gibco) for 2 h. The chondrocytes were maintained in DMEM supplemented with 10% FBS and 1% penicillin/streptomycin at 37 °C with 5% CO2. The medium was changed every 2 days. Upon reaching 80–90% cell confluence, the chondrocytes were passaged. The passage 3 (P3) chondrocytes were used for in vitro experiments.
Cell treatment
Chondrocytes were treated with 10 ng/mL IL-1β (Sangon, Shanghai, China) for 24 h to induce cell injury. OCY-exos (5 μg/mL) were added to the culture medium of chondrocytes to incubate for 48 h. Additionally, 10 mM GW4869 (an exosome inhibitor; Sigma-Aldrich, St. Louis, USA) was added to the culture medium to inhibit exosome release. To suppress the Wnt/β-catenin pathway, 10 μM XAV-939 was incubated with the chondrocytes for 24 h. To inhibit RNA transcription, chondrocytes were treated with 30 μL of 1 mg/mL actinomycin D (Sigma-Aldrich) for 0, 4, 8, and 12 h.
Western Blot
The antibodies (Abcam, Cambridge, USA) shown below were used to measure their protein levels: anti-CD63 (ab134045, 1/2000), anti-CD81 (ab109201, 1/2000), anti-Alix (ab88388, 1/1000), anti-collagen II (ab34712, 1/1000), anti-SOX9 (ab185230, 1/5000), anti-MMP13 (ab39012, 1/6000), anti-GAPDH (ab9485,1/2500), anti-Wnt3a (ab219412,1/1000), anti-Dkk1 (ab109416, 1/3000), anti-cyclin D1 (ab16663,1/200), anti-cMyc (ab32072, 1/1000), anti-sclerostin (SOST; ab85799, 1/1000), anti-β-actin (ab8227, 1/2000), and anti-rabbit IgG (ab205718,1/10000). Total protein was extracted from chondrocytes, OCY-exos, and serum exosomes using RIPA buffer on ice and separated using 12% SDS-PAGE. The products were transferred to PVDF membranes. The samples were incubated with the primary antibodies overnight at 4 °C and the secondary antibody for 1 h at room temperature. Each band was visualized using the SignalFire ECL reagent (Cell Signaling Technology, Danvers, USA).
Determination of cell viability
Chondrocytes were seeded into 96-well plates and treated with IL-1β, OCY-exos, GW4689, and/or XAV-939. Afterward, chondrocytes were incubated with 10 μL cell counting kit-8 (CCK-8) reagent (Dojingdo, Tokyo, Japan) for 2 h. The absorbance was measured at 450 nm using a microplate reader (Bio-Rad, Hercules, USA).
Determination of cell apoptosis
An Annexin V-PE/7-AAD apoptosis kit (Procell, Wuhan, China) was used for apoptosis detection using flow cytometry. Chondrocytes were centrifuged at 300g for 5 min and the precipitates were re-suspended in 500 μL binding buffer. The cell suspension was stained with 5 μL of Annexin V-PE and 5 μL of 7-AAD for 15 min in the dark at room temperature. The samples were detected using a flow cytometer (Beckman Coulter, Miami, USA) within 1 h.
Determination of cell migration
A wound healing assay was conducted to determine cell migration capability. The cells were seeded into 6-well plates and incubated at 37 °C with 5% CO2 until about 100% cell confluence. The wound was made using sterile pipette tips. Cell debris was removed by washing with PBS. The images were observed at 0 and 24 h of incubation under a light microscope (Olympus, Tokyo, Japan).
Cell transfection
Short hairpin RNA (Sh)-DLX2 and sh-negative control (NC) were designed and synthesized in Genepharma (Shanghai). MLO-Y4 cells were transfected with these vectors in 6-well plates using Lipofectamine 2000 (Invitrogen, Carlsbad, USA) in line with the manufacturer’s protocol. After 48 h, the transfected cells were harvested and the efficiency was measured.
Quantitative real-time polymerase chain reaction (qRT-PCR)
Total RNA was extracted from chondrocytes and OCY-exos using TRIzol reagent (Invitrogen). After the determination of RNA purity at 260/280 nm and integrity using agarose gel electrophoresis, reverse transcription was performed using the Quant cDNA first-strand synthesis kit (TianGen, Beijing, China). Subsequently, the SuperReal premix plus (SYBR Green) (TianGen) was used for qPCR on the LightCycle real-time PCR system (Roche, Basel, Switzerland). The expression of mRNAs was calculated using the 2−ΔΔCt method. GAPDH functioned as the internal control.
Primary murine osteocyte isolation
Primary osteocytes were isolated as previously described [Citation16]. Long bones were acquired from C57BL/6 mice (2–3 weeks old). After the soft tissues were removed, the bones were cut into pieces and washed using Hank’s balanced salt solution (HBSS; Sigma-Aldrich). Bone pieces were digested using 0.75% collagenase I solution (Sigma-Aldrich) three times, followed by alternate digestion using 0.75% collagenase I and 5 mM ethylenediaminetetraacetic acid (EDTA; Sigma-Aldrich). The digestive solution containing suspended cells was centrifuged at 200×g for 5 min, and the cells were collected and resuspended in the culture medium (α-MEM + 10% FBS + 1% penicillin/streptomycin). The cells were seeded in a collagen-coated petri dish, and the culture conditions were the same as those for MLO-Y4 cells.
Animal study
C57BL/6 mice (male, 10 weeks old) were used to establish the OA mouse model using destabilization of the medial meniscus (DMM) surgery. All mice were randomly divided into 4 groups: control, OA, OA + exo + sh-NC, and OA + exo + sh-DLX2 groups (6 mice/group). The DMM mouse model was generated as previously described [Citation17]. The mice were anesthetized, and the medial meniscal ligament on the right knee joints was transected. The mice in the control group were exposed to the same meniscal ligament without transection. Exosomes used in the animal study were extracted from primary murine osteocytes. From week 5 to week 8, OA mice were intra-articularly injected with 5 µL exos combined with sh-NC or sh-DLX2 twice a week. After 8 weeks of operation, the mice were sacrificed by intraperitoneal injection of an overdose of sodium pentobarbital sodium. The joint tissues were obtained and fixed in paraformaldehyde for the preparation of the paraffin section. The serum samples were acquired from mice in the control and OA groups, and serum exosomes (S-exo) were isolated.
Immunohistochemistry (IHC) assay
For the IHC assay, the sections were dewaxed and rehydrated. The endogenous peroxidase activity was quenched by H2O2 and the sections were blocked with goat serum. The sections were incubated with primary antibody (Abcam) against collagen II (ab34712, 1/100), SOX9 (ab185230, 1/2000), and MMP13 (ab39012, 1/1000) at 4 °C overnight and incubated with the secondary antibody (ab205718, 1/2000) at 37 °C for 1 h. The results were observed under a microscope.
Hematoxylin-eosin (H&E) assay
The paraffin sections were stained with hematoxylin (Sigma-Aldrich) for 5 min, followed by stained with eosin (Sigma-Aldrich) for 3 min. The histopathology results were imaged using a microscope (Olympus).
Statistical analysis
All experiments had three-time repetition. The results were analyzed using the GraphPad Prism 8.0 software and are shown as mean ± SD. Comparisons were analyzed using the unpaired Student’s t-test (two groups) or one-way ANOVA (multiple groups). p < 0.05 means a statistically significant difference.
Results
Exosomes were successfully isolated from osteocytes
First, we isolated exosomes from the culture medium of MLO-Y4 cells, and the characteristics of OCY-exos were identified. The results of TEM showed that the OCY-exos exhibited a double-layer cup membrane (). The diameter of OCY-exos was mainly between 100 and 200 nm (). Besides, the OCY-exos had higher levels of CD63, CD81, and Alix than that MLO-Y4 cells (). The results showed that we successfully isolated exosomes from MLO-Y4 cells.
Figure 1. Exosomes were successfully isolated from osteocytes. (A) The morphology of OCY-exos was assessed using TEM. Scale bar: 100 nm. (B) The particle size of OCY-exos was examined using NTA. (C) The surface markers of exosomes including CD63, CD81, and alix were analyzed by Western Blot.
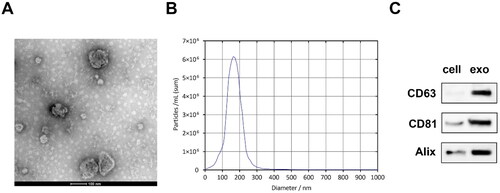
OCY-exos facilitate IL-1β-mediated cell viability, migration, and ECM deposition, and suppresses apoptosis.
Then, to explore the effect of OCY-exos on cartilage repair, we established an injury chondrocyte model that was stimulated by IL-1β. The model cells were treated with OCY-exos and GW4689, and cellular processes were determined. Cell viability was inhibited by IL-1β, which was promoted by OCY-exos, and GW4689 reversed the promotion of OCY-exos (). Inversely, IL-1β induced the apoptosis of chondrocytes. OCY-exos inhibited apoptosis of IL-1β-treated cells, and GW4689 abrogated the inhibition (). Cell migration of IL-1β-induced chondrocytes was promoted by OCY-exos, which was reversed by GW4689 (). In addition, OCY-exos upregulated collagen II and SOX9 levels, and downregulated MMP13 levels in IL-1β-treated chondrocytes, while GW4689 partly abolished the effects induced by OCY-exos (). The results indicated that OCY-exos promoted cell viability and migration, and inhibited apoptosis and ECM degradation of chondrocytes induced by IL-1β.
Figure 2. OCY-exos facilitates IL-1β-mediated cell viability, migration, and ECM deposition, and suppresses apoptosis. After the chondrocytes were treated with IL-1β, OCY-exos, and GW4689, (A) cell viability was evaluated by CCK-8; (B) apoptosis was assessed by flow cytometry and quantified in (C); (D) migration was analyzed using a wound healing assay and quantified in (E); and (F) a Western Blot was used to measure the protein levels of collagen II, SOX9, and MMP13. ***, ###, and &&& p < 0.001.
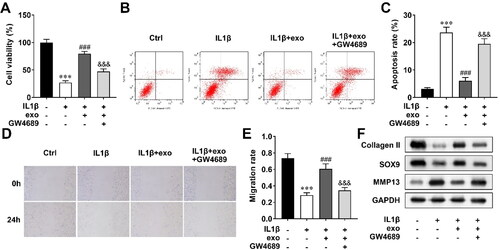
OCY-exos elevate DLX2 expression
To investigate which gene is associated with OCY-exo-mediated cartilage repair, we measured the expression of bone formation-related genes, including BMPR2, DLX2, Foxo3, and KLF4. The results showed that OCY-exos significantly upregulated the levels of DLX2 and Foxo3, but did not regulate the levels of BMPR2 and KLF4 in chondrocytes (). The most significantly upregulated DLX2 was chosen to be studied in the following study. Moreover, we confirmed that DLX2 was encapsulated by exosomes and carried to chondrocytes. As shown in Supplementary Figure 1(A), DLX2 was expressed in exosomes, and shDLX2 transfection reduced its expression. After inhibiting transcription with actinomycin D, OCY-exos treatment increased the expression of DLX2 in chondrocytes (Supplementary Figure 1B). The results suggest that increased DLX2 in chondrocytes is carried and transmitted by OCY-exos.
OCY-exos/DLX2 regulate cell viability, apoptosis, migration, and ECM deposition
To clarify whether OCY-exos regulated biological behaviors by regulating DLX2, we transfected sh-NC and sh-DLX2 into MLO-Y4 cells to interfere with DLX2 expression. The efficiency showed that DLX2 expression was significantly reduced in MLO-Y4 cells following sh-DLX2 transfection, compared with sh-NC (). The exosomes were then isolated from transfected MLO-Y4 cells. The expression of DLX2 was reduced in exosomes from sh-DLX2-transfected cells (). Then, these OCY-exos were co-cultured with IL-1β-stimulated chondrocytes. Knockdown of DLX2 suppressed the viability of OCY-exo-treated chondrocytes (). Silencing of DLX2 facilitated apoptosis of chondrocytes treated with OCY-exos (). Besides, DLX2 depletion suppressed the migration of chondrocytes (). The protein levels of collagen II and SOX9 were decreased, whereas MMP3 levels were increased by DLX2 knockdown in OCY-exo-treated cells (). Taken together, the knockdown of DLX2 reversed OCY-exos-mediated cellular processes including cell viability, apoptosis, migration, and ECM deposition.
Figure 4. OCY-exos/DLX2 regulates cell viability, apoptosis, migration, and ECM deposition. (A) The efficiency was examined using qRT-PCR after sh-NC and sh-DLX2 transfected into MLO-Y4 cells. (B) The levels of DLX2 were measured using qRT-PCR in exosomes isolated from transfected cells. Following chondrocytes treated with IL-1β and OCY-exos, (C) CCK-8 was performed to evaluate cell viability; (D) apoptosis was assessed by flow cytometry and quantified in (E); (F) wound healing assay assessed cell migration and was quantified in (G); (H) the protein levels of collagen II, SOX9, and MMP13 were measured using Western Blot. ***p < 0.001. **p < 0.01.
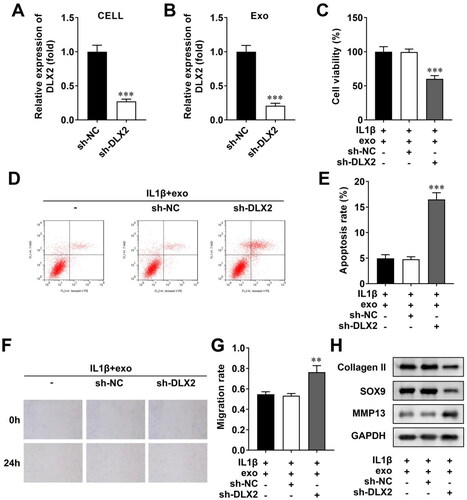
DLX2 regulates the Wnt pathway
To further explore the underlying mechanism of DLX2, the related factors of DLX2 were predicted. There were numerous molecules related to DLX2 (). The KEGG enrichment analysis indicated that DLX2 was associated with multiple signaling pathways including the Wnt signaling network (). To confirm the prediction, we used a Western Blot to measure the levels of factors related to the Wnt pathway. Knockdown of DLX2 upregulated Wnt3a, Dkk1, cyclin D1, and c-Myc protein levels (). The data indicated that silencing of DLX2 activated the Wnt pathway.
Figure 5. DLX2 Regulates the wnt pathway. (A) The interacted molecules of DLX2 were predicted using the STRING database and shown in the network. (B) The biological pathway related to DLX2 was predicted using KEGG enrichment analysis. (C) The protein levels of Wnt3a, Dkk1, cyclin D1, and c-myc were examined by Western Blot.
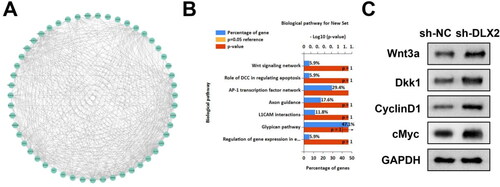
DLX2 mediates the biological behaviors via the Wnt pathway
To confirm that DLX2 regulates the Wnt pathway to participate in cellular processes, we used XAV-939 treatment to inhibit the activation of the Wnt pathway, Then, we found that XAV-939 promoted cell viability (), inhibited apoptosis (), and also facilitated migration of chondrocytes (). Additionally, XAV-939 treatment increased collagen II and SOX9 levels, and decreased MMP13 levels (). To sum up, inactivating the Wnt pathway reversed the biological functions of chondrocytes mediated by DLX2 knockdown.
Figure 6. DLX2 Mediate the biological behaviors via the wnt pathway. (A) CCK-8 evaluated cell viability; (B) flow cytometry assessed cell apoptosis, which was quantified in (C); scratch test evaluated migration, which was quantified in (E); (F) Western Blot measured the protein levels of collagen II, SOX9, and MMP13. **p < 0.01.
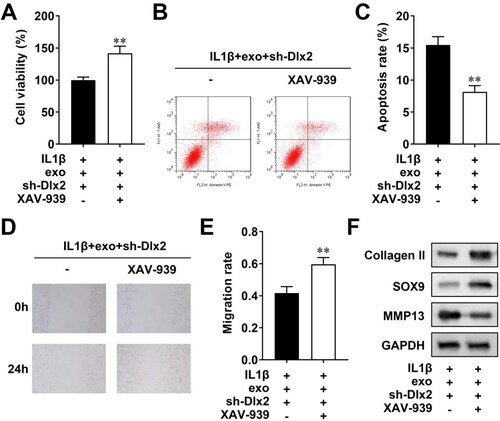
Primary OCY-exos attenuate OA by regulating DLX2
To identify the OCY-exos levels in OA, we established the OA mouse model. Serum exosomes were extracted from control and OA mice. SOST, a specific marker of osteocytes, was detected using a Western Blot. As shown in , serum exosomal SOST expression was higher in OA mice than that in control mice, suggesting that OCY-exos in the serum of OA mice was higher than that of normal mice. To investigate the role of OCY-exos/DLX2 in vivo, OA mice were treated with primary OCY-exos. According to H&E staining assay results, primary OCY-exos alleviated cartilage lesions in OA mice, and DLX2 knockdown abrogated the pathology affected by primary OCY-exos (). The results of the IHC assay showed that collagen II and SOX9 levels were reduced and MMP13 levels were elevated in OA mice. Primary OCY-exos upregulated collagen II and SOX9 levels and downregulated MMP13 levels in OA mice, while the knockdown of DLX2 abrogated the effects induced by primary OCY-exos (). The results demonstrated that OCY-exos alleviated OA by upregulating DLX2 expression.
Figure 7. Primary OCY-exos attenuates OA by regulating DLX2. (A) The protein levels of SOST (a specific osteocyte marker) in the serum exosomes from control and OA mice were measured using Western Blot. (B) The histopathological statue of cartilage was analyzed by H&E assay. (C) The protein expression of collagen II, SOX9, and MMP13 was evaluated in the cartilage tissues of mice using an IHC assay.
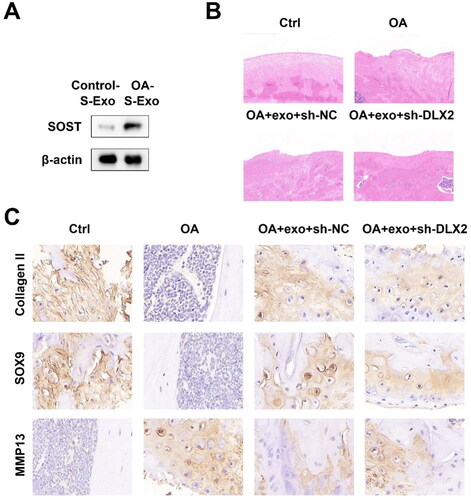
Discussion
The treatments for OA are based on its severity, with medication being used in the early stages and surgery in advanced patients; however, the efficacy remains unsatisfactory. Maintaining chondrocyte homeostasis is crucial for maintaining joint health. Chondrocyte apoptosis disrupts cartilage homeostasis, which is significantly associated with the severity of OA in patients [Citation18,Citation19]. The migration of chondrocytes promotes small cartilage defects [Citation20]. In addition, chondrocytes promote cartilage growth by releasing ECM, which is regulated by type II collagen, SOX9, and MMP13 [Citation21]. Therefore, inhibiting chondrocyte apoptosis and increasing growth and migration are the related mechanisms of cartilage repair and effective treatment of OA.
The emergence of exosomes brings new hope for bone formation and chondrogenesis due their powerful tissue repair function, which may be used in bone diseases, with a focus on OA, osteoporosis, and osteonecrosis [Citation22]. Several studies have revealed that exosomes, especially those secreted by mesenchymal stem cells and plasma, mediate cartilage repair by controlling cellular processes [Citation9,Citation23,Citation24]. OCY-exos are a type of bone-derived exosome that are involved in regulating bone metabolism and related diseases [Citation25]. For example, OCY-exos carry miR-218, and inhibition of exosomal miR-218 suppresses osteoblastic differentiation and affects the function of osteocytes [Citation26]. Additionally, OCY-exos promotes cell proliferation and osteogenesis of periodontal ligament stem cells, thus maintaining periodontal homeostasis [Citation27]. Osteocytes, as a major cell type, participate in the subchondral bone remodeling process, which plays a critical role in OA development [Citation28]. In a spontaneous OA mouse model, osteocytes with high cyclooxygenase-2 expression cause abnormal subchondral bone formation and thereby promote cartilage degeneration, supporting the important role of osteocytes in subchondral bone remodeling [Citation29]. However, it is largely unknown how the regulation of OCY-exos affects OA progression, particularly the impacts on cartilage repair. In this study, we first demonstrated that OCY-exos can promote cell viability, migration, and ECM production, and inhibit apoptosis of IL-1β-induced chondrocytes. Moreover, primary OCY-exos alleviated the pathology of OA in vivo. The findings suggested that OCY-exos attenuated cartilage injury, thereby decelerating the development of OA.
The DLX homeobox family contains six members (DLX1-6), with three gene pairs (DLX1/2, DLX3/4, and DLX5/6) located on different chromosomes and sharing intergenic enhancers. These genes are related to embryonic morphogenesis, including craniofacial structures, branchial arch, and brain development [Citation30]. DLX2, which bears strong sequence similarity to DLX3 and DLX5, is highly expressed in immature osteoblasts [Citation31]. Additionally, DLX2 facilitates chondrocyte differentiation in the early stage by increasing collagen II and impedes later differentiation via downregulating MMP13 [Citation32]. However, little is known about the mechanism of DLX2 in the regulation of cartilage repair and its association with OCY-exos. In this study, we found that OCY-exos carrying DLX2 upregulated the levels of DLX2 in chondrocytes, and confirmed that OCY-exos mediated chondrocyte biological behaviors by regulating DLX2. Moreover, the knockdown of DLX2 reversed the OCY-exos-improved cartilage pathology, suggesting a novel mechanism for the effects of OCY-exos on cartilage repair.
Accumulating evidence has revealed that the Wnt pathway, whether overactivated or suppressed, is associated with the pathogenesis of OA [Citation33]. Activation of both canonical Wnt cascade activation of β-catenin and noncanonical Wnt/PCP and Wnt/Ca2+ cascades is involved in OA development. Wnt plays a crucial role in bone and cartilage formation, growth, and repair [Citation34]. TNFα has been reported to promote cartilage differentiation via activating the Wnt/β-catenin pathway, thus facilitating cartilage repair [Citation35]. Moreover, the Wnt/β-catenin pathway can be activated by DLX2 through the transcriptional activation of Wnt1 and then affect osteogenesis [Citation36]. Herein, we found that the Wnt pathway was the downstream pathway of DLX2 and could be activated by DLX2 knockdown, which contradicts a previous study [Citation36]. This discrepancy may be attributed to the complexity of the Wnt pathway and its unclear role in chondrogenesis. Moreover, we confirmed that OCY-exos regulated cartilage repair by the DLX2/Wnt pathway, indicating the importance of the Wnt pathway in cartilage repair, thereby enhancing our understanding of the involvement of OCY-exos in OA progression.
Conclusion
Articular injury induces the occurrence and development of OA. In this study, we clarified that OCY-exos delivered DLX2 into chondrocytes and further inactivated the Wnt pathway to facilitate cell viability, migration, and ECM generation, and inhibit apoptosis induced by IL-1β, thereby promoting cartilage repair. Moreover, OCY-exos attenuated OA progression. Our findings lay the groundwork for the development of OCY-exos therapy, which shows promise for the treatment of OA.
Author contributions
All authors participated in the design, interpretation of the studies analysis of the data, and review of the manuscript. WX drafted the work and revised it critically for important intellectual content; YZ, LL, LP, and LL were responsible for the acquisition, analysis, or interpretation of data for the work; SZ and WL made substantial contributions to the conception or design of the work. All authors read and approved the final manuscript.
Ethical approval
All animal experiments comply with the ARRIVE guidelines. Approval was granted by the Laboratory Animal Welfare and Ethics Committee of Chongqing University.
Disclosure statement
No potential conflict of interest was reported by the authors.
Data availability statement
The datasets used and/or analyzed during the current study are available from the corresponding author upon reasonable request.
Additional information
Funding
References
- Katz JN, Arant KR, Loeser RF. Diagnosis and treatment of hip and knee osteoarthritis: a review. JAMA. 2021;325(6):568–578.
- Abramoff B, Caldera FE. Osteoarthritis: pathology, diagnosis, and treatment options. Med Clin North Am. 2020;104(2):293–311.
- Loeser RF, Goldring SR, Scanzello CR, et al. Osteoarthritis: a disease of the joint as an organ. Arthritis Rheum. 2012;64(6):1697–1707.
- Krych AJ, Saris D, Stuart MJ, et al. Cartilage injury in the knee: assessment and treatment options. J Am Acad Orthop Surg. 2020;28(22):914–922.
- Borrelli JJ, Olson SA, Godbout C, et al. Understanding articular cartilage injury and potential treatments. J Orthop Trauma. 2019;33 Suppl 6(3):S6–S12.
- Charlier E, Deroyer C, Ciregia F, et al. Chondrocyte dedifferentiation and osteoarthritis (OA). Biochem Pharmacol. 2019;165:49–65.
- Kalluri R, LeBleu VS. The biology, function, and biomedical applications of exosomes. Science. 2020;367(6478):eaau6977.
- De Jong OG, Van Balkom BW, Schiffelers RM, et al. Extracellular vesicles: potential roles in regenerative medicine. Front Immunol. 2014;5:608.
- Zhang S, Chuah SJ, Lai RC, et al. MSC exosomes mediate cartilage repair by enhancing proliferation, attenuating apoptosis and modulating immune reactivity. Biomaterials. 2018;156:16–27.
- Wang Y, Yu D, Liu Z, et al. Exosomes from embryonic mesenchymal stem cells alleviate osteoarthritis through balancing synthesis and degradation of cartilage extracellular matrix. Stem Cell Res Ther. 2017;8(1):189.
- Cui J, Shibata Y, Zhu T, et al. Osteocytes in bone aging: advances, challenges, and future perspectives. Ageing Res Rev. 2022;77:101608.
- Karthik V, Guntur AR. Energy metabolism of osteocytes. Curr Osteoporos Rep. 2021;19(4):444–451.
- Wang Z, Zhao Z, Gao B, et al. Exosome mediated biological functions within skeletal microenvironment. Front Bioeng Biotechnol. 2022;10:953916.
- Li J, Guo Y, Chen Y-Y, et al. miR-124-3p increases in high glucose induced osteocyte-derived exosomes and regulates galectin-3 expression: a possible mechanism in bone remodeling alteration in diabetic periodontitis. FASEB J. 2020;34(11):14234–14249.
- Wang YY, Xia K, Wang ZX, et al. Osteocyte exosomes accelerate benign prostatic hyperplasia development. Mol Cell Endocrinol. 2021;531:111301.
- Stern AR, Stern MM, Van Dyke ME, et al. Isolation and culture of primary osteocytes from the long bones of skeletally mature and aged mice. Biotechniques. 2012;52(6):361–373.
- Hwang HS, Park IY, Hong JI, et al. Comparison of joint degeneration and pain in male and female mice in DMM model of osteoarthritis. Osteoarthritis Cartilage. 2021;29(5):728–738.
- Huang H, Quan YY, Wang XP, et al. Gold nanoparticles of diameter 13 nm induce apoptosis in rabbit articular chondrocytes. Nanoscale Res Lett. 2016;11(1):249.
- Wang BW, Jiang Y, Yao ZL, et al. Aucubin protects chondrocytes against IL-1 beta-induced apoptosis in vitro and inhibits osteoarthritis in mice model. Drug Des Dev Ther. 2019;13:3529–3538.
- Danišovič L, Varga I, Polák S. Growth factors and chondrogenic differentiation of mesenchymal stem cells. Tissue Cell. 2012;44(2):69–73.
- Prein C, Beier F. ECM signaling in cartilage development and endochondral ossification. Curr Top Dev Biol. 2019;133:25–47.
- Bei HP, Hung PM, Yeung HL, et al. Bone-a-petite: engineering exosomes towards bone, osteochondral, and cartilage repair. Small. 2021;17(50):e2101741.
- Zhang Y, Wang X, Chen J, et al. Exosomes derived from platelet-rich plasma administration in site mediate cartilage protection in subtalar osteoarthritis. J Nanobiotechnol. 2022;20(1):56.
- Kim YG, Choi J, Kim K. Mesenchymal stem cell-derived exosomes for effective cartilage tissue repair and treatment of osteoarthritis. Biotechnol J. 2020;15(12):e2000082.
- Lyu H, Xiao Y, Guo Q, et al. The role of bone-derived exosomes in regulating skeletal metabolism and extraosseous diseases. Front Cell Dev Biol. 2020;8:89.
- Qin Y, Peng Y, Zhao W, et al. Myostatin inhibits osteoblastic differentiation by suppressing osteocyte-derived exosomal microRNA-218: a novel mechanism in muscle-bone communication. J Biol Chem. 2017;292(26):11021–11033.
- Lv P-Y, Gao P-F, Tian G-J, et al. Osteocyte-derived exosomes induced by mechanical strain promote human periodontal ligament stem cell proliferation and osteogenic differentiation via the miR-181b-5p/PTEN/AKT signaling pathway. Stem Cell Res Ther. 2020;11(1):295.
- Zhu X, Chan YT, Yung P, et al. Subchondral bone remodeling: a therapeutic target for osteoarthritis. Front Cell Dev Biol. 2020;8:607764.
- Tu M, Yang M, Yu N, et al. Inhibition of cyclooxygenase-2 activity in subchondral bone modifies a subtype of osteoarthritis. Bone Res. 2019;7(1):29.
- Tan Y, Testa JR. DLX Genes: roles in development and cancer. Cancers (Basel). 2021;13(12):3005.
- Li H, Marijanovic I, Kronenberg MS, et al. Expression and function of Dlx genes in the osteoblast lineage. Dev Biol. 2008;316(2):458–470.
- Zhang J, Zhang W, Shi J, et al. Dlx2 overexpression enhanced accumulation of type II collagen and aggrecan by inhibiting MMP13 expression in mice chondrocytes. Biochem Biophys Res Commun. 2018;503(2):528–535.
- Cheng J, Li M, Bai R. The Wnt signaling cascade in the pathogenesis of osteoarthritis and related promising treatment strategies. Front Physiol. 2022;13:954454.
- Lories RJ, Corr M, Lane NE. To Wnt or not to Wnt: the bone and joint health dilemma. Nat Rev Rheumatol. 2013;9(6):328–339.
- Voskamp C, Koevoet WJLM, Somoza RA, et al. Enhanced chondrogenic capacity of mesenchymal stem cells after TNFalpha pre-treatment. Front Bioeng Biotechnol. 2020;8:658.
- Zeng X, Wang Y, Dong Q, et al. DLX2 activates Wnt1 transcription and mediates Wnt/beta-catenin signal to promote osteogenic differentiation of hBMSCs. Gene. 2020;744:144564.