Abstract
Leaf dew is an important input in farmland ecosystems, but information regarding the nutrients from leaf dew deposition in paddy fields is scarce. Leaf dew samples were monitored and collected in situ from the Sanjiang Plain paddy experimental station during the rice growing periods in 2008, 2009, and 2010. The pH and essential nutrients [ammonium nitrogen (), nitrate nitrogen (
), and phosphorus (P)] in paddy leaf dew were investigated, the nutrients in guttation and condensed dew were distinguished. In addition, the nutrient contributions of foliar fertilizer to Oryza sativa L (rice) were compared with those of leaf dew. The results indicate that the mean pH of leaf dew on rice is 6.3 (n=56), lower than the pH of rain and surface water. Condensed dew contributes about 97.7% of the nitrogen (N) and 95.7% of the phosphorus (P) in paddy leaf dew. Guttation provides a minor part of the N and P in leaf dew. The amount of leaf dewfall is much larger than the volume of foliar fertilization, with the amount of
,
, and P deposition from leaf dew almost 100 higher than from foliar fertilization. Compared with foliar fertilizer, leaf dew is a natural and potential fertilizer. Leaf dew is an important pathway for nutrient transfer within paddies.
Introduction
Dew, a crucial factor in the water cycle of farmland ecosystems, plays an important role in the water balance of paddy fields (Xu et al., Citation2011a). The contribution of guttation to leaf wetness in paddy rice cannot be ignored (Luo & Goudriaan, Citation2000). In the present study, paddy leaf dew is defined as the mixture of dew condensed on paddy leaves and guttation. Guttation is the loss of water and dissolved materials from uninjured plant organs, and it is a common phenomenon in higher plants. Some nutrients in dew, as well as its pH, influence plant growth in paddies (Singh et al., Citation2006). As a form of wet deposition, dew is a major pathway for acidic pollutants from the atmosphere to enter the biosphere (Valenta et al., Citation1986). For example, acidic dew is potentially more damaging than acid rain on surface structures. Acidic dew damages the protective surfaces of leaves, interferes with guard cells, and poisons plant cells (Chiwa et al., Citation2003). On the other hand, ammonium nitrogen and nitrate nitrogen in dew are beneficial to plants.
To improve grain yields, large amounts of fertilizers are used in China annually (Kahrl et al., Citation2010), but they are not fully efficient (Raun & Johnson, Citation1999). In the black soil paddy fields in Northeast China, 22.2–46.1% of the nitrogen (N) from applied chemical fertilizer is absorbed by paddy plants, and about 43% of the nitrate and 10% of the phosphorus (P) from the total fertilizer applied are lost each year (Han et al., Citation2003; Zhu & Yan, Citation2010). Only 10–35% of the N remain in the soil (Datta et al., Citation1988), with 15.3% of the N lost through lateral seepage, surface runoff, and artificial drainage (Han et al., Citation2003; Zhu & Yan, Citation2010). The losses through gaseous (NH3) emissions comprise 30–39% (Cai et al., Citation2002). Phosphorus is also lost through surface drainage (Chung et al., Citation2003 and Zhang et al., Citation2007). Some of the N in fertilizers is not lost by volatilization because the NH3 and N2O in the air condense on leaves at night, which are reabsorbed by the rice plants. Although the nutrients in the surface water of paddies are the main source of nutrients for rice, according to Krupa (Citation2003), the N from dry and wet depositions account for about 10–30% of the total nitrogen assimilated by the plants. Some nutrients from dry deposition on leaves that dissolve in dew are assimilated by plants (Peuke et al., Citation1998) and guttation from leaves may contain some nutrients. Nutrients can be directly absorbed through the leaves (Grammatikopoulos & Manetas, Citation1994; Sergi et al., Citation1999). The nutrients on leaves, which act as foliar fertilizers, are beneficial to rice growth. The ,
, and HPO4 or H2PO4 species are the main forms of N and P that can be absorbed by plants (Franco & Munns, Citation1982; Karthikeyan et al., Citation2009; Sun et al., Citation2011). The
uptake by rice is highly efficient (Bloom, Citation1985). The pH,
,
, and P concentrations in paddy leaf dew are easily analyzed. With leaf dew acting as a surfactant by which nutrients adhere onto the leaves, the recognition of leaf dew as an important factor in nutrient uptake is particularly significant. However, leaf dew has received little attention as a nutrition source in agricultural systems, and few studies have focused on the quality of dew because collection is difficult (Muselli et al., Citation2002; Lekouch et al., Citation2010). Studies on the chemical characterization of dew in farmland ecosystems are scarce.
The Sanjiang Plain is an important grain base in China. The annual dewfall in paddies is about 30 mm (Xu et al., Citation2011a). The phenomenon of chemical fertilizer loss is serious (Zhu & Yan, Citation2010). Leaf dew has always been ignored as a nutrient input. Identifying the nutrients in leaf dew is necessary for determining its significance in agricultural practices. The objectives of the present study are (1) to analyze the pH, ,
, and P concentrations in leaf dew; (2) to differentiate the nutrient sources of leaf dew; and (3) to calculate the amounts of deposition of
,
, and P in leaf dew on rice.
Materials and methods
Study site
The experiment was conducted at Sanjiang Plain paddy experimental station (47° 35′ N, 133° 31′ E), Chinese Academy of Sciences, Tongjiang, Heilongjiang Province, Northeast China. At the station, the elevation is about 56 m above sea level and the average annual temperature is 1.9°C. About 65% of the annual precipitation, which ranges from 550 to 600 mm, occurs from June to September (Jia et al., Citation2006). The cropping pattern in the paddy was carried out as follows: rice seedlings were planted at the end of May or at the beginning of June, artificial drainage (breaking the bund [also referred to as dikes, banks or levees] of the paddy and allowing the surface water to flow into the drainage ditch) was performed in mid-to-late August, and rice was reaped during the first 10 days of October. The 5th to 24th of May was the germination to emergence stage. Foliar fertilizer applied during the tillering and booting stages. Foliar fertilizer, mainly composed of potassium dihydrogen phosphate (KH2PO4) and urea, was applied twice during the tillering and booting stages.
Sample collection
The leaf dew samples were collected 30 min before sunrise during heavy-dew days. A total of 168 leaf dew samples were collected from the tillering stage to the ripening stage during the rice growing seasons in 2008, 2009, and 2010. After washing with distilled water and drying, a polyethylene basin was used to collect the leaf dew water. All samples were then placed in 50 mL polyethylene bottles (Takenaka et al., Citation2003). To avoid contamination during collection, the leaf dew water was collected in situ. The collector was placed close to the roots, and the rice stem was slightly shaken until the leaf dew dropped into the collector. The leaf dew was then poured from the collector into the polyethylene bottles. The steps were repeated until the polyethylene bottles were full. The required 50 mL leaf dew was collected from about 25 to 35 strains of rice. The rice were distributed in the four corners of a 20 m×20 m field, designated as Experimental field I.
The vapor sources of the paddy leaf dew consist of two parts, the leaf exudates (guttation) and plant transpiration, and the evaporated surface water mixed with vapor in the air (condensed dew). Considering the nutrients from the dissolved dry deposition on the leaves contribute to the nutrients in dew, the samples were collected directly from the rice leaves. However, the samples collected directly on the rice leaves also contained water and nutrients from guttation. To distinguish the sources of N and P in leaf dew, samples of the two parts were separately collected in 2010. The amount of guttation is heavy during the rice development stage (Luo & Goudriaan, Citation2000); therefore, guttation was collected in July and August. To avoid disturbance from dust on the rice leaves, the leaves were washed with distilled water at sunset before guttation. The rice leaves were then immediately covered with 0.5 m×0.3 m (length×width) plastic bags (). The bottoms of the bags were sealed to prevent the entrance of vapor from the atmosphere. The amount of guttation was small, some of which condensed in the bag and some still clung to the rice leaves during collection. To collect the guttation that still clung to the leaves at sunrise, the stems of the rice plants close to the bottom of bag were lightly shaken until the guttation on the leaves dropped into the bag. The water in the bag was considered guttation. Condensed dew was collected using 1.5 m×1.5 m (length×width) clean plastic sheets. The plastic sheets were placed on the paddy canopy at sunset to collect the water that accumulates on them. The leaf dew and the samples from these two parts were collected at the same time. A total of 90 guttation and condensed dew samples were collected. The samples were placed in 50 mL polyethylene bottles. The surface water was collected using a 100 mL polyethylene bottle when the leaf dew was collected. Rain was collected from the same site using polyethylene containers during the experimental period. All samples were collected in triplicate each time. In total, 168 surface water and 105 rain samples were collected.
The application of foliar fertilizer is a common method of improving grain yield. Foliar fertilizer was applied twice each year in July at around 8:30 in the morning in the normal field. The spray time and the concentration of the foliar fertilizer during each application are displayed in . The foliar fertilizer used in the Sanjiang Plain was concentrated fertilizer diluted with water. The concentrated fertilizer was the same; however, the volume of water used was not an exact value but a range, causing the slight differences in the N and P concentration in the foliar fertilizer each year. The volume of foliar fertilizer applied was about 500 L per hectare. Except for Experimental field I, the other fields (normal field) were all sprayed with foliar fertilizer. To prevent contamination of the leaf dew with foliar fertilizer, the leaf dew water was collected from the Experimental field I during the experiment period each time. Therefore, despite differences in N and P concentrations in the foliar fertilizer, it did not affect the chemical characteristics of leaf dew and the comparison between the N and P depositions from leaf dew and foliar fertilizer.
Table I. Foliar fertilizer spraying schedule and concentrations of
,
, and P in the foliar fertilizer per application from 2008 to 2010 in the Sanjiang Plain.
Dew monitoring
The method for monitoring dewfall was validated by Yan and Xu in 2010. The dew monitoring in situ was carried out from 10 June to 30 September in 2008, 6 June to 15 October in 2009, and 1 July to 6 October in 2010 in the Sanjiang Plain paddy experimental station. A wooden stick used as a condenser for monitoring dewfall was weighed daily at sunset and sunrise with an electronic balance (accuracy within 0.001 g). The 18 cm×3.5 cm×3.5 cm (length×width×height) poplar woodstick was polished. For the experiment plot, an observation shelf was set up, with a bottom layer (5 cm above surface water level) and a canopy layer. The height of the canopy layer can be adjusted with the varying height of rice. The condensers were set at the bottom and on the canopy of the rice. The factual dew per unit area was computed as their average. For each height, triple parallel condensers were placed when the air temperature (°C) (T a ) reached the dew point temperature (°C) (T d ) after sunset. The condensers were kept in each plot until sunrise. The condensers were gathered when T a was higher than T d , and then weighed again. Everyday meteorological factors, including T a and T d , were measured at hourly intervals during condensation time by the meteorological station at Sanjiang Plain paddy experimental station.
Calculation
Dew intensity and dewfall were calculated with the following formulas:
where I is the dew intensity (mm); W r is the weight of condenser before sunrise (g); W s is the weight of the condenser after sunset (g); S is the surface area of the condenser (cm2); and 10 is the conversion factor.
where DF is the total dewfall (mm-days); DF
i
is the dewfall in a particular period (mm-days); and LAI is the leaf area index. LAI
i
is the LAI in a particular period (cm2/cm2); is the average I in a particular period (mm); and D is the dew days. D
i
is the D in a particular period (days); 2 is the coefficient of a leaf side; and n is the time for measuring LAI (Yan & Xu, Citation2010).
According to Xu et al. (Citation2011b), based on isotopic mass conservation to quantitatively partition the contribution of different vapor sources of leaf dew, guttation and transpiration from the plant account for about 30% of the paddy leaf dew during the rainy season. The evaporation of surface water mixed with vapor in air (condensed dew) accounts for the remaining portion. Therefore, dewfall comprises about 70% of the total leaf dewfall.
The LAI of the paddy was measured using a LAI-2000 Plant Canopy Analyzer over a 10-day interval. The LAI was measured in each growth stage from the tillering stage to the mature grain stage. Distinguishing dew from rain was difficult, so dewfall was recorded as zero for the night if there was a rainfall during the measuring period.
Guttation, condensed dew, and dust on the leaves all contributed some nutrients to the leaf dew. Therefore, the relationship of nutrients in each part was described by the following formula:
where C 1 is the nutrient concentration of guttation; V 1 is the amount of guttation; C 2 is the nutrient concentration of the condensed dew; V 2 is the amount of condensed dew; C 3 is the nutrient concentration of dry deposition dissolved in atmospheric vapor; C 4 is the nutrient concentration of leaf dew; and V 4 is the amount of leaf dew. The nutrients in condensed dew contain two parts: from the atmosphere and from the dissolved dry deposition. Thus, Formula 5 is simplified into Formula 6.
The volume-weighted average should be used in calculating the depositions of ,
, and P in leaf dew. A limitation in the method of collecting leaf dew is that it could not be collected every time it condenses. Therefore, the average concentrations of
,
, and P were used. Because leaf dew was little in October 2010 and it leaded to collecting leaf dew samples was difficult. The depositions of
,
, and P in October 2010 were derived through extrapolation.
Sample analysis
The analyses were carried out in a laboratory in Sanjiang Experimental Station, Northeast Institute of Geography and Agroecology, Chinese Academy of Sciences. The pH which measured using a pH-meter (BS14-PH60, Germany) was immediately determined after collecting the samples. The samples were filtered through a 0.45 µm pore membrane. The ,
, TN, and P concentrations in each sample were then immediately measured using a discrete auto analyzer (Smartchem 200, Italy).
The statistical analyses were carried out using Statistical Product and Service Solutions (SPSS) version 16.0 (Inc., USA). To test the normal distribution of ,
, and P concentrations, Q–Q probability plots were employed. The
,
, and P concentrations were analyzed using one-way ANOVA, and the significance limit was set to P <0.05. Least Significant Difference or Tamhane's T2 procedure was used to determine the significant differences between each group of concentrations. The mean separation of pH among rain, surface water, and leaf dew was determined using a t-test at a 0.05 level of significance. The data of samples are all the mean of three replicates. The difference were considered significant if P was lower than 0.05.
Results
Nutrients in leaf dew, guttation, and condensed dew
The nutrient concentrations in guttation were low, as shown in . Statistical analysis indicated that the ,
, and P concentrations in the rain, surface water, guttation, condensed dew, and leaf dew, and the pH of the rain, surface water, and leaf dew all followed a normal distribution. The
(P=0.0000) and
(P=0.0000) concentrations in guttation were significantly lower than in condensed dew. The P concentration in guttation was significantly higher than that in condensed dew (P=0.0002).
Figure 2. Variations in nutrient concentrations in leaf dew, guttation, and condensed dew collected from the Sanjiang Plain in 2010. The (P=0.0000) and
(P=0.0000) concentrations in guttation were significantly lower than in condensed dew. The P concentration in the guttation was significantly higher than that in condensed dew (P=0.0002).
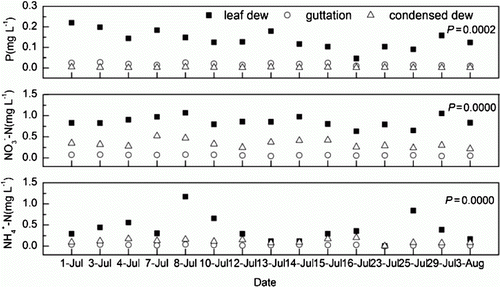
Nutrient and pH in leaf dew, rain, and surface water
The ,
, and P concentrations were analyzed to assess the nutrient concentrations in leaf dew and to compare them with those in rain (). The
concentration was significantly higher than the
and P concentrations (P=0.0000) in leaf dew. The trends of
,
, and P concentrations in leaf dew and rain fluctuated and exhibited irregularities.
Figure 3. Variations in pH, ,
, and P concentrations in rain and leaf dew in 2008, 2009, and 2010 in the Sanjiang Plain. The number under the date represents the growth stage scale of the rice, during which the leaf dew was collected. The
concentration was significantly higher than the
and P concentrations (P=0.0000) in leaf dew.
Note: 0 is germination stage; 1 is the seedling stage; 2 is the tillering stage; 3 is the stem elongation stage; 4 is panicle initiation to booting stage; 5 is the heading stage; 6 is flowering stage; 7 is the milk grain stage; 8 is the dough grain stage; 9 is the mature grain stage.
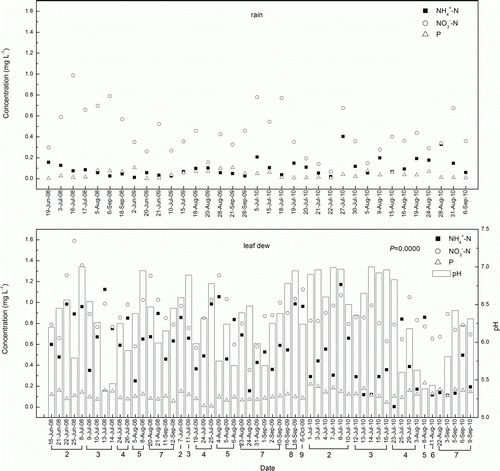
The mean concentrations of ,
, and P in leaf dew, rain, and surface water from the paddy are displayed in . The statistical analysis showed that the
(P=0.0000),
(P=0.0000), and P (P=0.0000) concentrations in leaf dew and surface water were significantly higher than in rain. Therefore, leaf dew provides more nutrients to paddies than rain. Similar results were reported in Southwestern Morocco (Lekouch et al., Citation2011), Santiago City, Chile (Rubio et al., Citation2002), North Central India (Singh et al., Citation2006), and Amman, Jordan (Jiries, Citation2001), where the chemical concentrations in dew were much higher than in rain. The higher concentrations in dew may be due to dry deposition on wet surfaces (Rubio et al., Citation2002) and the variations in composition at high altitude (cloud level) and low altitude (ground level) aerosols and gases to which dew and rain are exposed (Singh et al., Citation2006).
Figure 4. Mean concentration of ,
, and P in leaf dew (n=168), rain (n=105), and surface water (n=168) in 2008, 2009, and 2010 in the Sanjiang Plain (different letters represent significant differences at the 0.05 level; error bars indicate standard deviation, n=3).
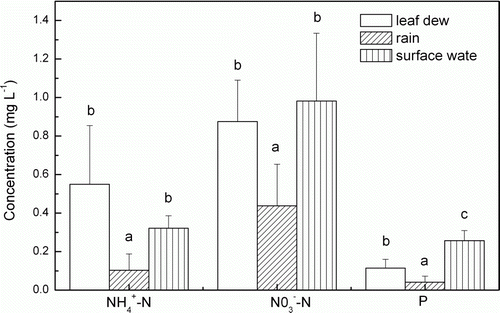
Although the N and P concentrations in surface water were high after using base fertilizer, according to the experiments by Liang et al., (Citation2008) and Zhang et al., (Citation2007), they rapidly decreased with time. The (P=0.0532) and
(P=0.0673) concentrations between leaf dew and surface water did not show significant differences.
pH is an important in assessing the nutrient contributions of dew to paddies. As shown in , paddy leaf dew pH varied between 5.4 and 7.0, with an average of 6.3 (n=56). The measured pH was highest in rainfall, followed by surface water, and then by dew.
Table II. The average value and the range of pH of leaf dew, rain, and surface water in 2008, 2009, and 2010, and the average ratio of
,
, and P in leaf dew, guttation, condensed dew, and dry deposition in 2010 in the Sanjiang Plain.
Discussion
Source of nutrients in leaf dew
The nutrients in leaf dew were from guttation, condensed dew, and dust on the leaves. The average ratio of nutrients in each component of the leaf dew is shown in . Guttation fluid contributed low amounts of nutrients to leaf dew. Condensed dew contributed about 30% of the N in leaf dew. The statistical analysis showed that the (P=0.0021),
(P=0.0036), and P (P=0.0000) concentrations in surface water were significantly higher than in water vapor. According to Equation (Equation5), about 70% N and more than 90% P were attributed to dry deposition. When dry deposition dissolves in condensed dew, the nutrients are directly absorbed by the rice leaves. Therefore, dew formation facilitates nutrient uptake. Aside from guttation fluid, the nutrients in condensed dew and dissolved dry deposition both contributed to the nutrients in dew. The N and P in condensed dew accounted for 97.7% of the N and 95.7% of the P in leaf dew, and condensed dew was the main contributor of N and P to leaf dew.
pH of leaf dew
The mean pH of dew on rice was 6.3, lower than the pH of rain and surface water. The dew pH in Indianapolis ranges between 6.0 and 7.2 (Foster et al., Citation1990), whereas the average dew pH in Arkansas, USA is 6.37 (Wagner et al., Citation1992). In Zadar, Croatia, the dew pH is between 5.5 and 7.8, with a mean of 6.7 (Lekouch et al., Citation2010). In Bordeaux, France, the average dew pH is 6.3 (Beysens et al., Citation2006). The dewfall in these areas are acidic. Dew acidity is derived from CO2 absorption and the acidic aerosol dissolution during droplet growth (Lekouch et al., Citation2010). However, the rain pH in these areas is lower than dew pH because of the various quantities of acidic species (SO2, NOx) influenced by human activities, such as fluctuating pollutant production and wind dispersion, or preceding rain events that clear out the aerosols in these areas (Lekouch et al., Citation2010 and Beysens et al., Citation2006). In the Sanjiang Plain, no automobile exhaust, coal combustion, or industrial pollution were detected; thus, its rain pH was higher than in other areas.
Paddy plants absorb more than
(Bloom, Citation1985). Plants take in more cations than anions, export protons, and acidify the soil (Franco & Munns, Citation1982). Paddy roots release H+ and produce organic acids in this process (Janjit et al., Citation2007), which decreases the surface water pH to less than 7.0. Moreover, the guttation fluid from plants and the water vapor from surface water all contribute to paddy leaf dew. Some H+ secretions from leaves are also contained in leaf dew samples (Chen et al., Citation1990). The pH of leaf dew is lower than that of surface water because of such secretions. Although leaf dew pH is the lowest, plants could be damaged when the pH of acid rain reaches around 3.0 (Le et al., Citation1998). Thus, the dew in the paddies does not damage or endanger leaves.
As shown in , and
from condensed dew contributes about 30% N to paddy leaf dew. Dew condenses under aerobic conditions, and
is prone to transform into
, producing more
than
, which dissociates into H+. This phenomenon lowers the pH of leaf dew compared with that of surface water and rain. Rubio et al. (Citation2008) observed that nitrite concentration is strongly correlated with dew pH.
and
concentrations may be correlated with pH. The leaf dew samples contained many suspended solids because of the method used for leaf dew collection. The adsorption of P is weaker under acidic conditions, and leads to increased P concentrations in leaf dew when the pH is less than 7.0 (Yuan et al., Citation2010). Moreover, under alkaline conditions,
is more likely to be converted into NH3, and the
concentration declines with NH3 diffusion (Yuan et al., Citation2010). Therefore,
condensation is higher under acidic conditions. However, P and
condensation markedly increased on 13 July in 2008 and on 8 August 2010 with lower pH. Based on the statistical analysis, P (P=0.9060) and
(P=0.5051) concentrations do not correlate with pH, as presented in . Thus, pH does not affect the P and
concentrations in the Sanjiang Plain.
Leaf dew and foliar fertilizer
The contribution of leaf dew to paddies is limited because the former is temporal because of its limited condensation time. Foliar fertilizer and leaf dew both drop and cling onto rice leaves, supplying nutrients to the paddy. To compare the nutrients in leaf dew and foliar fertilizer, the deposition amounts of ,
, and P were calculated.
presents the daily and monthly dewfall. Dewfall levels reached their peak in August in each year and the variations in dewfall and leaf area index (LAI) was almost the same. The actual dewfall per unit field area was calculated using LAI, dew days, and dew amount. Thus, LAI determines dewfall. The concentrations and deposition of ,
, total nitrogen (TN) and P in leaf dew and foliar fertilizer are shown in . Part of the N in leaf dew was from NH3 diffusion. N concentration is dependent on the amount in surface water. However, collecting the leaf dew during the paddy shoot stage was difficult. Therefore, the calculated
and
deposition in leaf dew was less than the actual value. presents the amounts of
,
, TN, and P in paddy leaf dew deposited during the experimental period. The input of
and
constituted 66.7, 62.9, and 69.2% of the TN in leaf dew in the 2008, 2009, and 2010, respectively. The annual input of
,
, and TN were about 0.15, 2.0, and 0.6 g m−2 in Sede Boqer (Kidron & Starinsky, Citation2012). The N input was much higher than in the Sanjiang Plain. The dewfall in Sede Boqer was 38 mm, which approximates that in the paddy fields in the Sanjiang Plain. However, the concentrations of
and
in Sede Boqer were higher, approaching 15.0 and 150.0 mg L−1, respectively. This finding indicates that the N input in Sede Boqer was considerably higher.
Table III. Concentration and deposition (calculated with Formula 6) of
,
, TN, and P of leaf dew and foliar fertilizer after each application in 2008, 2009, and 2010.
Figure 5. Variations in dewfall, leaf area index (LAI), and condensed dew intensity with time in the paddy fields in 2008, 2009, and 2010 in the Sanjiang Plain. The number under the date represents the growth stage scale of the rice during which the LAI was measured.
Note: 2 is the tillering stage; 3 is the stem elongation stage; 4 is panicle initiation to booting stage; 5 is the heading stage; 6 is flowering stage; 7 is the milk grain stage; 8 is the dough grain stage; 9 is the mature grain stage. For example, LAI was measured in 13 June of 2008. The number under the date is ‘2’ and it indicated LAI measured for this time was in the ‘tillering stage’ of the rice.
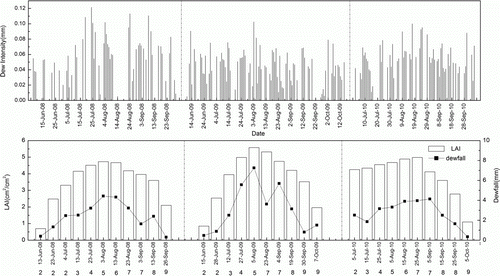
Although the average ,
, and P concentrations were lower in leaf dew than in the fertilizer, the deposition in leaf dew was obviously greater than that in the foliar fertilizer. Dew condensed on the rice leaves during the whole growth period, and dew occurred for about 80–90 days. The dewfall was 26.2, 31.4, and 27.3 mm in the paddies in 2008, 2009, and 2010, respectively. The leaf dewfall was 37.4, 44.5, and 39.0 mm during the experimental period. The volume of leaf dewfall was much higher than that of foliar fertilizer. Therefore, although leaf dew contained lower nutrient concentrations, it supplied nutrients on a long-term basis. Leaf dew has temporality, and the foliar fertilizer evaporates. Thus, leaf dew contributes more nutrients to paddies and is consequently a significant resource for rice growth.
The nutrient deposition by leaf dew and the foliar fertilizer was calculated. However, because both leaf dew and foliar fertilizer evaporate easily, the nutrients they contain are not completely absorbed. The amount of N and P in leaf dew and foliar fertilizer that is actually used by rice should be discussed in further research.
Acknowledgements
Funding support is gratefully acknowledged from the Special S&T Project on Treatment and Control of Water Pollution (2012ZX07201004) and National Nature Science Foundation of China (41101470). We express our gratitude to the Sanjiang Mire Wetland Experimental Station of Chinese Academy of Sciences for providing meteorological data. The authors also thank Liu Jinhui for her help during dew collection. We are indebted to the seniors in our team for their critical reading, kind remarks, and relevant suggestions.
References
- Beysens , D. , Ohayon , C. , Muselli , M. and Clus , O. 2006 . Chemical and biological characteristics of dew and rain water in an urban coastal area (Bordeaux, France) . Atmospheric Environment , 40 : 3710 – 3723 . doi: 10.1016/j.atmosenv.2006.03.007
- Bloom , A. J. 1985 . Wild and cultivated barleys show similar affinities for mineral nitrogen . Oecologia , 65 : 555 – 557 . doi: 10.1007/BF00379672
- Cai , G. X. , Chen , D. L. , Ding , H. , Pacholske , A. , Fan , X. H. and Zhu , Z. L. 2002 . Nitrogen losses from fertilizers applied to maize, wheat and rice in the North China Plain . Nutrient Cycling in Agroecosystems , 63 : 187 – 195 . doi: 10.1023/A:1021198724250
- Chen , C. T. , Chou , I. T. and Kao , C. H. 1990 . Senescence of rice leaves. XX. changes of proton secretion during senescence . Plant Science , 66 : 29 – 34 . doi: 10.1016/0168-9452(90)90165-K
- Chiwa , M. , Oshiro , N. , Miyake , T. , Nakatani , N. , Kimura , N. , Yuhara , T. , Hashimoto , N. and Sakugawa , H. 2003 . Dry deposition washoff and dew on the surfaces of pine foliage on the urban-and mountain-facing sides on Mt. Gokurakuji, western Japan . Atmospheric Environment , 37 : 327 – 337 . doi: 10.1016/S1352-2310(02)00889-0
- Chung , S. O. , Kim , H. S. and Kim , J. S. 2003 . Model development for nutrient loading from paddy rice fields . Agricultural Water Management , 62 : 1 – 17 . doi: 10.1016/S0378-3774(03)00078-7
- Datta , S. K. D. , Samson , M. I. , Wang , K. R. and Buresh , R. J. 1988 . Nitrogen Use Efficiency and Nitrogen-15 Balances in Broadcast-seeded Flooded and Transplanted Rice . Soil Science Society of America Journal , 52 : 849 – 855 . doi: 10.2136/sssaj1988.03615995005200030045x
- Foster , J. R. , Pribush , R. A. and Carter , B. H. 1990 . The chemistry of dews and frosts in Indianapolis, Indiana . Atmospheric Environment , 24 : 2229 – 2236 . doi: 10.1016/0960-1686(90)90254-K
- Franco , A. A. and Munns , D. N. 1982 . Plant assimilation and nitrogen cycling . Plant Soil , 67 : 1 – 13 . doi: 10.1007/BF02182751
- Grammatikopoulos , G. and Manetas , Y. 1994 . Direct absorption of water by hairy leaves of Phlomis fruticosa and its contribution to drought avoidance . Canadian Journal of Botany , 72 : 1805 – 1811 . doi: 10.1139/b94-222
- Han , X. Z. , Wang , S. Y. , Song , C. Y. & Qiao , Y. F. 2003 . Fate of fertilizer nitrogen in paddy field of black soil region . Chinese Journal of Applied Ecology 14 , 1859 – 1862 . [in Chinese]
- Janjit , I. , Su , W. Y. and Jae , S. R. 2007 . Nutrient removals by 21 aquatic plants for vertical free surface-flow (VFS) constructed wetland . Ecological Engineering , 29 : 287 – 293 . doi: 10.1016/j.ecoleng.2006.09.010
- Jia , Z. J. , Song , C. C. and Sun , L. 2006 . The study on latent and sensible heat flux over mire in the Sanjiang Plain . Wetland Science , 4 : 13 – 20 .
- Jiries , A. 2001 . Chemical composition of dew in Amman, Jordan . Atmospheric Research , 57 : 261 – 268 . doi: 10.1016/S0169-8095(01)00079-5
- Kahrl , F. , Li , Y. J. , Su , Y. F. , Tennigkeit , T. , Wilkes , A. and Xu , J. C. 2010 . Greenhouse gas emissions from nitrogen fertilizer use in China . Environmental Science & Policy , 13 : 688 – 694 . doi: 10.1016/j.envsci.2010.07.006
- Karthikeyan , S. , Balasubramanian , R. and He , J. 2009 . Inter-laboratory study to improve the quality of the analysis of nutrients in rainwater chemistry . Atmospheric Environment , 43 : 3424 – 3430 . doi: 10.1016/j.atmosenv.2009.03.025
- Kidron , G. J. and Starinsky , A. 2012 . Chemical composition of dew and rain in an extreme desert (Negev): Cobbles serve as sink for nutrients . Journal of Hydrology , 420–421 : 284 – 291 . doi: 10.1016/j.jhydrol.2011.12.014
- Krupa , S. V. 2003 . Effects of atmospheric ammonia (NH3) on terrestrial vegetation: A review . Environmental Pollution , 124 : 179 – 221 . doi: 10.1016/S0269-7491(02)00434-7
- Le , Y. Q. , Dai , X. Z. & Zhou , B. B. 1998 . Experiment on the effect of simulated dew on plant . Shanghai Environmental Sciences 17 , 33 – 34 . [In Chinese] .
- Lekouch , I. , Mileta , M. , Muselli , M. , Milimouk-Melnytchouk , I. , Šojat , V. , Kabbachi , B. and Beysens , D. 2010 . Comparative chemical analysis of dew and rain water . Atmospheric Research , 95 : 224 – 234 . doi: 10.1016/j.atmosres.2009.10.002
- Lekouch , I. , Muselli , M. , Kabbachi , B. , Ouazzani , J. , Milimouk , M. I. and Beysens , D. 2011 . Dew, fog, and rain as supplementary sources of water in south-western Morocco . Energy , 36 : 2257 – 2265 . doi: 10.1016/j.energy.2010.03.017
- Liang , X. Q. , Li , H. , Chen , Y. X. , He , M. M. , Tian , G. M. and Zhang , Z. J. 2008 . Nitrogen loss through lateral seepage in near-trench paddy fields . Journal of Environmental Quality , 37 : 712 – 717 . doi: 10.2134/jeq2007.0073
- Luo , W. H. and Goudriaan , J. 2000 . Dew formation on rice under varying durations of nocturnal radiative loss . Agricultural and Forest Meteorology , 104 : 303 – 313 . doi: 10.1016/S0168-1923(00)00168-4
- Muselli , M. , Beysens , D. , Marcillat , J. , Milimouk , I. , Nilsson , T. and Louche , A. 2002 . Dew water collector for potable water in Ajaccio (Corsica Island, France) . Atmospheric Research , 64 : 297 – 312 . doi: 10.1016/S0169-8095(02)00100-X
- Peuke , A. D. , Jeschke , W. D. , Dietz , K. J. , Scheriber , L. and Hartung , W. 1998 . Foliar application of nitrate or ammonium as sole nitrogen supply in Ricinus communis I. Carbon and nitrogen uptake and inflows . New Phytologist , 138 : 675 – 687 . doi: 10.1046/j.1469-8137.1998.00158.x
- Raun , W. R. and Johnson , G. V. 1999 . Improving nitrogen use efficiency for cereal production . Agronomy Journal , 91 : 357 – 636 . doi: 10.2134/agronj1999.00021962009100030001x
- Rubio , M. A. , Lissi , E. and Villena , G. 2002 . Nitrite in rain and dew in Santiago city, Chile. Its possible impact on the early morning start of the photochemical smog . Atmospheric Environment , 36 : 293 – 297 . doi: 10.1016/S1352-2310(01)00356-9
- Rubio , M. A. , Lissi , E. and Villena , G. 2008 . Factors determining the concentration of nitrite in dew form Santiago, Chile . Atmospheric Environment , 42 : 7651 – 7656 . doi: 10.1016/j.atmosenv.2008.05.055
- Sergi , M. B. , Salvador , N. and Leonor , A. 1999 . Diurnal variations of photosynthesis and dew absorption by leaves in two evergreen shrubs growing in mediterranean field conditions . New Phytologist , 144 : 109 – 119 . doi: 10.1046/j.1469-8137.1999.00490.x
- Singh , S. P. , Khare , P. , Maharaj , K. K. and Srivastava , S. S. 2006 . Chemical characterization of dew at a regional representative site of North-Central India . Atmospheric Research , 80 : 239 – 249 . doi: 10.1016/j.atmosres.2005.09.003
- Sun , C. F. , Xiao , K. , Han , S. F. , Zhao , F. H. & Bao , J. X. 2011 . Advances in the molecular mechanism of phosphorus uptake and transportation in plants . Journal of Agricultural Science and Technology 13 , 17 – 24 . [In Chinese] .
- Takenaka , N. , Soda , H. , Sato , K. , Terada , H. , Suzue , T. , Bandow , H. and Maeda , Y. 2003 . Difference in amounts and composition of dew from different types of dew collectors . Water, Air & Soil Pollution , 147 : 51 – 60 . doi: 10.1023/A:1024573405792
- Valenta , P. , Nguyen , V. D. and Nornberg , H. W. 1986 . Acid and heavy metal pollution by wet deposition . Science of the Total Environment , 55 : 311 – 320 . doi: 10.1016/0048-9697(86)90188-9
- Wagner , G. H. , Steele , K. F. and Peden , M. E. 1992 . Dew and frost chemistry at a midcontinent site, United States . Journal of Geophysical Research , 97 : 20591 – 20597 . doi: 10.1029/92JD02385
- Xu , Y. Y. , Yan , B. X. & Wang , L. X. ( 2011a ). A research of dewfall in paddy . Scientia Agricultura Sinica 44 , 524 – 530 . [In Chinese] .
- Xu , Y. Y. , Yan , B. X. & Wang , L. X. ( 2011b ). Discrimination of vapour sources of dew in Sanjiang Plain by stable isotopic technique . Environmental Science 32 , 1550 – 1556 . [In Chinese] .
- Yan , B. X. and Xu , Y. Y. 2010 . Method exploring on dew condensation monitoring in wetland ecosystem . Procedia Environmental Sciences , 2 : 123 – 133 . doi: 10.1016/j.proenv.2010.10.016
- Yuan , J. , Chen , Y. , Li , Q. , Yuan , H. Y. & Sun , L. P. 2010 . Impact of pH on release of nitrogen and phosphorus from excess sludge . China Water Wastewater 26 , 96 – 98 . [In Chinese] .
- Zhang , Z. J. , Zhang , J. Y. , He , R. , Wang , Z. D. and Zhu , Y. M. 2007 . Phosphorus interception in floodwater of paddy field during the rice-growing season in TaiHu lack basin . Environmental Pollution , 145 : 425 – 433 . doi: 10.1016/j.envpol.2006.05.031
- Zhu , H. & Yan , B. X. 2010 . Export of phosphorus from paddy field and the transport process in Sanjiang Plain . Wetland Science 8 , 266 – 272 . [In Chinese] .