Abstract
The aim of the present study is to investigate the effects of salinity and the influence of supplemental K+ and Ca2+ on the alleviation of the deleterious effects of salinity stress in spinach plants. Spinach cultivars used were Green Gold, Larissa, Mikado, Ohio and Matador. In addition to four NaCl levels of 50, 100, 150 and 200 mM, the treatments were repeated with 10 mM K and 10 mM Ca at each NaCl level. The results revealed that Na+ and Cl− concentrations were significantly increased and the K+, Ca2+ and NO3 − concentrations were decreased with increasing NaCl concentrations in the growth medium. Being very important to the physiology and biochemistry of plants decreases in K+, Ca2+ and NO3 − reduced both growth and the yield. The additions of K+ and Ca2+ reduced the Na+ and Cl− concentrations in the plant tissues, increased the K+, Ca2+ and NO3 − concentrations, resulting in an improvement in the marketable yield of the spinach plants. Even though the effects of supplemental K+ and Ca2+ were similar in some plants at different salt concentrations, the effect of additional Ca2+ alone was higher overall. Thus, the results of this study confirmed the principal effects of K+ and Ca2+ supplies in reducing the detrimental effects of salinity.
Introduction
The salt tolerance of vegetables species is important due to the cash value of the product. Indeed, the yield and quality traits of vegetable crops are adversely affected by environmental factors, such as high salinity in the root zone (Goyal et al., Citation2003). Salinity stress results in a notable stunting of plant growth, which results in a considerable decrease in the fresh and dry weights of the leaves, stems and roots (Parida & Das, Citation2005; Hajer et al., Citation2006). Spinach is a vegetable for which the leaves and stems are used fresh or processed (Rubatzky & Yamaguchi, Citation1997) and is moderately salt sensitive (Shannon & Grieve, Citation1999).
Potassium is an essential element for plant growth and has important functions (Mahajan & Tuteja, Citation2005). Because of its importance, salt-tolerant plants must maintain a high level of K+ in their cells (Volkmar et al., Citation1998). Under salt stress, potassium deficiency occurs due to the similar structures of potassium and sodium, which impedes discrimination between the two ions (Maathuis & Amtmann, Citation1999). Na+ toxicity mainly results from this lack of discrimination: The salinity-induced inhibition of shoot growth at a low level of K+ in the root medium was attributed to the effect of K+ deficiency and/or Na+ toxicity in the plants. Similar responses have been found in spinach plants (Chow et al., Citation1990). The addition of K+ to a saline culture solution was found to increase the plant dry weight and K+ concentration, with a corresponding decrease in the Na+ concentration, in rice and bean plants (Benlloch et al., Citation1994). Under saline conditions, an increased supply of K+ reduced the fruit fresh weight and marketable yield (MY) of pepper, while promoting the vegetative growth (Rubio et al., Citation2009). In tomato, lettuce, Chinese cabbage and sweet corn, the application of potassium nitrate was a very efficient method of combating the aforementioned stresses and enhancing crop performance under saline conditions and resulted in increased yields (Achilea, Citation2002).
Most of the research on Ca2+ and salinity interactions has focused on agricultural crop species (Renault et al., Citation2001). Salinity impairs the uptake of Ca2+ ions by plants due to ionic interactions and a reduction in the Ca2+ activity in the soil solution, thus limiting their availability to the plant (Suarez & Grieve, Citation1988). The addition of Ca2+ ameliorates the adverse effects of salinity on plants (Martinez-Ballesta et al., Citation2000). Rubio et al. (Citation2009) stated that pepper fruit quality parameters were affected by K+ and Ca2+ treatments and that the MY was increased with additional Ca2+ in the nutrient solution. Lauchli and Epstein (Citation1990) also demonstrated that supplemental Ca2+ could maintain membrane integrity in both roots and shoots by limiting Ca2+ displacement from the membrane by Na+ ions.
Salinity can directly affect nutrient uptake, with Cl− reducing the NO3 − uptake; apparently, the counter-cation may influence the Cl− antagonism of NO3 − uptake (Grattan & Grieve, Citation1999). Kafkafi et al. (Citation1992) found that Cl− inhibited NO3 − uptake in melon and tomato in a range at which the plants would likely be exposed under field conditions. Because Na+ in most cases of natural salinity is accompanied by chloride, competition with nitrate was suggested as a practical agricultural method to prevent salt damage in tomato (Kafkafi et al., Citation1982). Additions of nutrients improved the growth and/or yield of spinach (Langdale et al., Citation1971) when the degree of salinity was not severe.
It is well known fact that an additional supply of Ca2+ or K+ to salt-stressed plants improves their tolerance to salinity. In the present study, five commercial spinach cultivars, Green Gold, Larissa, Mikado, Ohio and Matador, were exposed to different NaCl concentrations with additional potassium and calcium. The main goal of this study is to examine the effects of salinity and to ascertain the ameliorating effects of calcium and potassium supplementations on the adverse effects of salinity in the five different spinach varieties by evaluating the MY and accumulation of ions.
Materials and methods
The experiment was conducted in a greenhouse at the Mustafakemalpasa Vocational School (40°01′N, 28°22′E, 25m a.s.l.), Department of Soil Sciences (laboratory), Uludag University, Turkey, during the autumn season of 2010.
Plant material
Five spinach cultivars (Spinacia olerecea L.) Green Gold, Larissa, Mikado, Ohio (Pop Vriend Seeds BV Company, Andijk, the Netherlands) and Matador (Kucuk Ciftlik Seeds Company, Balikesir, Turkey) were used in the experiments.
Growth conditions and salinity treatments
The seeds were sown in plastic containers (15 cm apart in rows of 15 cm in width) in early September of 2010. The containers were filled with sandy loam soil (71% sand, 19% loam, 10% clay, 1.3% organic matter and 0.09% total N, pH 6.5). All of the containers were randomized on benches in a greenhouse (unheated). The plants were grown in greenhouse, with a day/night average temperature of 21°C and an average relative humidity of 70%.
Salinity treatments were imposed when the first true leaves appeared by adding NaCl to the nutrient solutions. The plants were irrigated 1–2 times a day. It was necessary to maintain the quantity of the drainage water at 30–40% of the amount of nutrient solution applied; the excess water drained through the holes in the base of the container. For these experiments, solutions of varying NaCl concentrations were supplied to the plants growing in a soil medium. The treatments initiated for the seedling stage were as follows: (1) Complete nutrient solution alone control (C); (2) C+50 mM (C+S50); (3) C+100 mM (C+S100); (4) C+150 mM NaCl (C+S150); (5) C+200 mM NaCl (C+S200); (6) C+50 mM NaCl+supplementary 10 mM K (in the form of KNO3) (C+S50+K10); (7) C+50 mM NaCl+supplementary 10 mM Ca (in the form of Ca(NO3)2) (C+S50+Ca10); (8) C+100 mM NaCl+supplementary 10 mM K (C+S100+K10); (9) C+100 mM NaCl+supplementary 10 mM Ca (C+S100+Ca10); (10) C+150 mM NaCl+supplementary 10 mM K (C+S150+K10); (11) C+150 mM NaCl+supplementary 10 mM Ca (C+S150+Ca10); (12) C+200 mM NaCl+supplementary 10 mM K (C+S200+K10) and (13) C+200 mM NaCl+supplementary 10 mM Ca (C+S200+Ca10). The composition of the nutrient solution was, as described by Robinson et al. (Citation1983), as follows: 4 mM Ca(NO3)2; 6 mM KNO3; 1 mM KH2PO4; 2 mM MgSO4; 50 mM FeEDTA; 50 mM H3BO3; 10 µM MnCl2; 0.5 mM CuSO4; 0.1 µM Na2MoO4 and 1 µM ZnS04. The pH of the solution was maintained at ~6.5 by adding H2SO4 as required. The salt levels were gradually increased over 4 days to avoid osmotic shock.
Experimental design
The experiment was arranged separately in a completely randomized block design, with four replications. There were 12 plants in each container, with one container in each replicate.
Growth parameter analysis
All of the plants in each container were harvested at 60 days after the seeds were sown (45 days after the initiation of the salinization). The net MY was calculated as described by Leskovar and Piccinni (Citation2005). The spinach plants were cut at approximately 5 cm above the soil surface; after removing the foreign material and dry and yellow leaves, the tissue was weighed and expressed as the MY kg m−2.
Mineral composition analysis
The harvested plants were first washed with tap water and twice with distilled water to remove the medium and nutrient solution and then dried at 70°C for 48 h. The dried samples were milled through a 1 mm screen and stored in plastic vials. The total cations (K+ ‘potassium’, Ca2+ ‘calcium’ and Na+ ‘sodium’) were determined after the digestion of the samples with 2 mL HNO3 and 3 mL H2O2 using a microwave oven and measured using the Eppendorf Elex 6361 model Flame photometer (Kacar & Inal, Citation2008). NO3 − (nitrate) was determined by the methods of Cataldo et al. (Citation1975). The NO3 − concentration was measured in using a Shimadzu UV-1208 spectrophotometer at a wavelength of 410 nm. Cl− (chloride) was determined by titration with silver nitrate using potassium chromate indicator (Chapman & Pratt, Citation1961). The K+, Ca2+, Na+, NO3 − and Cl− concentrations in dry matter were expressed as percentage.
Statistical analysis
The data were analyzed using MSTAT-C v. 2.1 and Minitab 14.0 software. An analysis of variance (ANOVA) was performed, and the significance of differences among the treatments was tested using the least significant difference (LSD) method. The differences were judged significant at P<0.05, according to the F-test. The F-protected LSD values were calculated at 0.05 probability levels.
Results
The results revealed that the MYs of the spinach cultivars were strongly affected by all of the salt treatments, with an increased salt concentration causing a decrease in the MY. The MY versus NaCl concentration function was obtained as MY=−0.0093 [NaCl]+2.6856; R 2=0.94 (). The function shows that, in this study, a concentration of approximately 289 mM NaCl was required for the spinach cultivars to cease producing a MY. This number can be derived from the equation that assumes a MY=0 kg m−2 [NaCl]=2.6856/0.0093=289 mM. The slope of the line indicates that the MY decreased with the NaCl concentration at a rate of 0.0093 kg m−2 mM−1. For the salt-stressed spinach plants, the highest MY was found with the treatments including the supplementation of K+ and Ca2+. That is, the supplementation of NaCl concentrations with 10 mM K+ and 10 mM Ca2+ significantly increased the MY for the spinach plants when compared to the plants treated only with NaCl (excluding Green Gold and Mikado at 50 mM NaCl and Larissa and Mikado at 200 mM NaCl). The lowest MY was achieved without any supplementation. The ability of supplemental K+ and Ca2+ to ameliorate the negative effects of NaCl on the yield was clearly shown at the increased NaCl concentrations. Comparing the addition of K+ to the addition of Ca2+, the MY values of the plants treated with supplemental Ca2+ were higher than those treated with supplemental K+. In some cases, increases in the yield were slight with the addition of Ca2+ (Ohio at 50 and 100 mM NaCl) but were very significant in many cases (Larissa and Mikado at 100 mM NaCl, Green Gold, Larissa and Ohio at 150 mM and Green Gold, Ohio and Matador at 200 mM NaCl) ().
Figure 1. Relationship between NaCl and MY, and relationships of NaCl and ions concentrations (K+, Ca2+, Na+, Cl− and NO3 −) in five spinach cultivars.
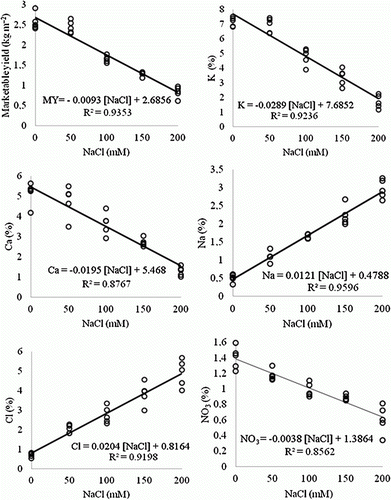
Table I. Effects of NaCl, and K+ and Ca2+ supplementation on MY (kg fw m−2)a in spinach plants grown under saline conditions.
In this study, the K+ concentration in the spinach plants decreased with increased salinity. A negative linear relationship was found between the NaCl concentration and K+ concentration, with the equation for the relationship being K+=−0.0289 [NaCl]+7.6852 with R 2=0.92 (). The K+ concentration of the NaCl-treated spinach plants were compared to those supplemented with K+ and Ca2+, and the results showed that the additional K+ and Ca2+ had positive effects on the K+ concentration of the spinach plants. The supplementation of 10 mM K+ and Ca2+ at a 50 mM NaCl concentration drastically increased the K+ concentrations for Green Gold, Mikado and Matador as compared to the plants treated with NaCl only. K+ and Ca2+ supplementations did not result in a significant change in the concentration of K+ in Larissa and Ohio plants at a 50 mM level of NaCl. However, the supplementation of 10 mM K+ and Ca2+ increased the K+ concentrations at 100 mM NaCl concentration in Mikado, Ohio and Matador at 150 mM NaCl in Green Gold, Mikado and Matador and at 200 mM NaCl in Green Gold, Larissa and Matador. We examined whether K+ and Ca2+ could influence the K+ concentration of spinach grown under saline conditions. The highest K+ concentrations were obtained in the spinach plants treated only with additional Ca2+, yet the K+ concentrations were not different between the supplemental K+ and Ca2+ treatments for some of the cultivars. Increases in the K+ concentrations in the spinach plants treated only with Ca2+ were small in some cases (Mikado and Matador at 50 mM, Mikado, Ohio and Matador at 100 mM and Larissa at 200 mM) yet were quite significant at the 50 mM level for Green Gold ().
Table II. Effects of NaCl, and K+ and Ca2+ supplementation on K+, Ca2+, Na+, Cl− and NO3 − concentrations in spinach plants grown under saline conditions.
The highest Ca2+ concentrations were obtained in the control treatment, and the Ca2+ concentrations decreased with increasing salt concentrations. The NaCl concentration was well correlated with the Ca2+ concentration, resulting in R 2=0.88 (). The lowest Ca2+ concentrations were found at 200 mM NaCl, but additions of K+ and Ca2+ positively altered the Ca2+ concentrations. The ability of supplemental K+ and Ca2+ to ameliorate the negative effects of NaCl was clearly observed at concentrations of 150 and 200 mM NaCl. In all of the spinach plants, with the exception of Mikado (150 mM NaCl) and Matador (200 mM NaCl), the Ca2+ concentrations were significantly increased with supplemental K+ and Ca2+ treatments. At concentrations of 50 and 100 mM NaCl, the additional K+ and Ca2+ treatments increased the Ca2+ concentration more significantly in Green Gold, Matador (50 mM NaCl) and Green Gold (100 mM NaCl). As compared with the additional K+, a small increase in the total K+ concentration was observed when the plants were grown with NaCl and additional Ca2+ (Green Gold and Matador at 50 mM NaCl, Green Gold, Ohio and Matador at 100 mM NaCl, Larissa and Ohio at 150 mM NaCl and Larissa and Mikado at 200 mM NaCl). Increases in the Ca2+ concentration resulting from Ca2+ supplementation were also more significant for Matador (150 mM NaCl) and Green Gold (200 mM NaCl) than additional K+ ().
In the present study, when the salinity was increased, the plant Na+ concentrations increased markedly under saline conditions. The Na+ concentration versus NaCl concentration function was obtained as Na+=0.0121 [NaCl]+0.4788, with R 2=0.96 (). The data for the Na+ concentration of the spinach plants in relation to salinity and supplementation are shown in . In general, the supplemental K+ and Ca2+ treatments tended to decrease the Na+ concentrations for the spinach plants under 100, 150 and 200 mM NaCl. Additions of 10 mM K+ and Ca2+ to the nutrient solutions at concentrations of 100, 150, and 200 mM NaCl significantly decreased the Na+ concentration in all of the spinach plants (except for Larissa at 100 and 150 mM). Interestingly, the supplemental K+ and Ca2+ treatments at 50 mM NaCl were not as effective as at the other levels, and decreases in the Na+ concentrations in Mikado and Ohio were important only with the K+ supplementation. Compared to the Ca2+ supplemented plants, reduced Na+ concentrations in the plant tissues were observed with the supplemental K+ treatments.
As expected, increasing the NaCl concentration in the growth medium significantly increased the Cl− concentrations in the spinach plants, as shown in . The Cl− concentration versus NaCl concentration function was obtained as Cl−=0.0204 [NaCl]+0.8164, with R 2=0.92 (). In the salt-treated spinach plants, the lowest Cl− concentrations were found under the supplemental Ca2+ treatment. The supplementation of 10 mM Ca2+ to NaCl significantly decreased the Cl− accumulation in the spinach plants compared with the plants treated with NaCl without added Ca2+, indicating the essential role of Ca2+ in decreasing the toxic effects of Cl− in stressed spinach plants. In contrast, we found that a Ca2+ supply was ineffective only in Green Gold (50 and 100 mM NaCl) and Larissa (50 mM NaCl). A similar trend was found with supplementation of K+ compared to addition of Ca2+, the reduction in the Cl− concentration with the addition of K+ was less pronounced. For instance, no significant differences were detected between the NaCl and NaCl+10 mM K+ treatments in Green Gold and Larissa (50, 100 and 150 mM NaCl) and Matador (150 mM NaCl).
The nitrate concentrations of spinach plants at various concentrations of salinity as a function of the K+ and Ca2+ concentrations are given in . In this study, the nitrate concentrations decreased markedly with increasing salinity. indicates that there was a good negative linear relationship between the NO3 − concentration and NaCl concentration (R 2=0.86). In general, the additions of K+ (10 mM) and Ca2+ (10 mM) to the nutrient solutions resulted in increased concentrations of nitrate in the spinach plants (except for Mikado at 100 mM NaCl) and might, therefore, account for the alleviation of the salt stress-induced toxic effects. However, the addition of Ca2+ caused more significant increases in the nitrate concentrations in all of the cultivars than the addition of K+ alone, which can be clearly observed at 50 mM NaCl (Green Gold, Mikado and Ohio), 100 mM NaCl (Green Gold and Ohio), 150 mM NaCl (Ohio and Matador) and 200 mM NaCl (in all of the plants).
Discussion
Our findings revealed that increasing the salt concentration caused a decrease in the MY. In agreement with our results, several authors using different spinach cultivars, including Matador (Kaya et al., Citation2001), Space (Suleiman et al., Citation2002) and water spinach (Yousif et al., Citation2010), have also found significant reductions in growth as the salinity increased in the irrigation water. Maas and Hoffman (Citation1977) also reported that a high salt concentration in the growth media affects the growth and economic yield of many important crops.
The excess foliar accumulation of Na+ and Cl− inhibits plant growth and development (Munns Citation2002; Ashraf & Harris, Citation2004). Salam et al. (Citation1992) found a highly significant negative correlation between Na+ and Cl− and the yield. In the present study, when the salinity increased, the Na+ and Cl− concentrations in the spinach plants markedly increased under saline conditions. According to Chookhampaeng et al. (Citation2007), the accumulation of harmful Na+ and Cl− and retardation of the uptake of macronutrients, especially K+ and Ca2+, are regarded as some of the most important consequences of salinity stress and cause a reduction in plant growth. The leaf K+ of salt-treated spinach plants was decreased, but Na+ and Cl− were greatly increased (Robinson et al., Citation1983). Inal (Citation2002) reported on salinity-induced Ca2+ deficiency. In our research, the K+ and Ca2+ concentrations in the spinach plants significantly decreased with increasing salinity. Na+-induced K+ deficiency has been implicated in abnormal growth and reduced yield in several crops, including spinach (Chow et al., Citation1990). However, increasing the amount of salt in the growth media affected the NO3 − uptake of spinach plants; the nitrate concentration was significantly decreased in salt-stressed spinach plant tissue. Our results were in agreement with Gouia et al. (Citation1994), Parida and Das (Citation2005) who found that salt stress reduces N+ uptake in many plants. Hussain et al. (Citation1990) also showed that the source of nitrogen could play an important role in increasing plant tolerance to salinity. Our results indicated a negative relationship between the nitrate and chloride concentrations and increased NaCl concentrations. Studying different plants, several authors have reported the same relationship: Grattan and Grieve (Citation1999) stated that salinity could directly affect nutrient uptake, with Cl− reducing NO3 − uptake. Chow et al. (Citation1990) found that Cl− induced NO3 − deficiency was responsible for the growth retardation in plants exposed to high levels of NaCl stress.
Modifying the nutrient supply is one of the ways of alleviating the opposing effects of salinity on plant growth; the adverse effects of salinity on plant growth and the tolerance to salinity can be altered by mineral nutrition (Tabatabaei & Fakhrzad, Citation2008). In the present study, it was considered that the deleterious effects of salinity on the MY can be largely mitigated by supplementary K+ and Ca2+, and separate additions of K+ and Ca2+ significantly increased the MY values in some cultivars. These results are similar to those of Benlloch et al. (Citation1994), Muhammad et al. (Citation1987) who reported increases in the plant dry weight and K+ concentration and a corresponding decrease in the Na+ concentration in rice and bean plants with the addition of K+ to a saline growth medium. When the addition of K+ was compared to the addition of Ca2+, we found that the treatments with Ca2+ significantly increased the MY.
Our results suggest that supplementary K+ can reduce the adverse effects of increased salinity in spinach plants. Supplementary 10 mM K+ treatment in spinach plants played a significant role in mitigating the deleterious effects of salinity, decreasing the amounts of Na+ and Cl−, while increasing the concentrations of K+, Ca2+ and NO3 −, which are important in growth and development. These results were in agreement with Kaya et al. (Citation2001) who found that supplementary K+ can reduce the adverse effects of high salinity on plant growth and physiological development in spinach plants (cv. Matador). These results were supported by Satti and Al-Yahyai (Citation1995) who showed that deficiencies in tomato growth were corrected by the addition of P and K+. Achilea (Citation2002) also indicated that, in lettuce, Chinese cabbage and sweet corn, the application of potassium nitrate was a very efficient method of enhancing crop performance under saline conditions and increasing yields. The findings of Tzortzakis (Citation2010) also suggest that the proper management of the salt concentration of the nutrient solution plus external elemental enrichment may provide an efficient tool to improve the quality of leafy vegetables, with little effect on the yield.
Most of the research on Ca2+ and salinity interactions has focused on agricultural crop species (Renault & Afifi, Citation2009). In tomato (Caines & Shennan, Citation1999), pepper (Cabanero et al., Citation2004) and endive (Tzortzakis, Citation2010), the negative impact of salinity on plant growth was reversed when the salinity was combined with Ca2+ enrichment. In our study, the supplementation of Ca2+ with salt positively affected the growth of the plants. Increases in the amount of NaCl in the growth medium induces nutritional disorders, which may result from the decrease in the uptake of such nutrients as K+, Ca2+ and NO3 −, and these adverse effects were mostly eliminated by the addition of 10 mM of Ca 2+ to the nutrient solution. In most of the treatments, the presence of Ca2+ with NaCl lowered the Na+ and Cl− concentrations of the spinach plants, while increasing the K+, Ca2+ and NO3 − concentrations. These results were in agreement with Bliss et al. (Citation1986), Girija et al. (Citation2002), Qadir et al. (Citation2002) who reported that supplemental Ca2+ alleviates deleterious effects, probably by mitigating the toxic effect of NaCl. Al-Khateeb (Citation2006) reported that Ca2+ plays a significantly role in diminishing the harmful effects of salinity, probably by controlling Cl− absorption. Colmer et al. (Citation1995) found that supplemented Ca2+ reduced Na+ accumulation. In spinach plants (cv. Space), the shoot Ca2+ increased significantly, as the salinity and substrate Ca2+ increased (Suleiman et al., Citation2002). Furthermore, external Ca2+ facilitates K+ and minimizes Na+ uptake (Taiz & Zeiger Citation2006).
The practical implication of this study is that the principal effects of K+ and Ca2+ supplies in reducing the detrimental effects of salinity. Future research should be conducted in the field, where salinity is an issue, using other spinach cultivars to validate these findings.
References
- Achilea , O. 2002 . Alleviation of salinity–induced stress in cash crops by multi-K (potassium nitrate), five cases typifying the underlying pattern . Acta Horticulturae , 573 : 43 – 48 .
- Al-Khateeb , S. A. 2006 . Effect of calcium/sodium ratio on growth and ion relations of Alfalfa (Medicago sativa L.) seedling grown under saline conditions . Journal of Agronomy , 5 : 175 – 181 . doi: 10.3923/ja.2006.175.181
- Ashraf , M. and Harris , P. J. C. 2004 . Potential indicators of salinity tolerance in plants . Plant Science , 166 : 13 – 16 . doi: 10.1016/j.plantsci.2003.10.024
- Benlloch , M. , Ojeda , M. A. , Ramos , J. and Rodriguez-Navarro , A. 1994 . Salt sensitivity and low discrimination between potassium and sodium in bean plants . Plant Soil , 166 : 117 – 123 . doi: 10.1007/BF02185488
- Bliss , R. D. , Platt-Aloia , A. and Thomson , W. W. 1986 . Osmotic sensitivity in relation to salt sensitivity in germination barley seed . Plant Cell Environment , 9 : 721 – 725 . doi: 10.1111/j.1365-3040.1986.tb02104.x
- Cabanero , F. J. , Martinez , V. and Carvajal , M. 2004 . Does calcium determine water uptake under saline conditions in pepper plants, or is it water flux that determines calcium uptake? . Plant Science , 166 : 443 – 450 . doi: 10.1016/j.plantsci.2003.10.010
- Caines , A. M. and Shennan , C. 1999 . Interactive effects of Ca2+ and NaCl salinity on the growth of two tomato genotypes differing in Ca2+ use efficiency . Plant Physiology and Biochemistry , 37 : 569 – 576 .
- Cataldo , D. A. , Haroon , L. E. , Schrader , L. and Young , V. L. 1975 . Rapid colorimetric determination of nitrate in plant tissue by nitration of salicylic acid . Communications of Soil Science and Plant Analysis , 6 : 71 – 80 . doi: 10.1080/00103627509366547
- Chapman , H. D. & Pratt , P. F. 1961 . Methods of Analysis for Soils Plants and Waters Berkeley : University of California Division of Agricultural Science .
- Chookhampaeng , S. , Pattanagul , W. and Theerakulpisut , P. 2007 . Screening of some tomato commercial cultivars from Thailand for salinity tolerance . Asian Journal of Plant Sciences , 6 : 788 – 794 . doi: 10.3923/ajps.2007.788.794
- Chow , W. S. , Ball , M. C. and Anderson , J. M. 1990 . Growth and photosynthetic responses of spinach to salinity: Implications of K+ nutrition for salt tolerance . Australian Journal of Plant Physiology , 17 : 563 – 578 . doi: 10.1071/PP9900563
- Colmer , T. D. , Epstein , E. and Dvora , K. J. 1995 . Differential solute regulation in leaf blades of various ages in salt-sensitive wheat and a salt-tolerant wheat Lophopyrum elongatum (Host.) A Love amphiploid . Plant Physiology , 108 : 1715 – 1724 .
- Girija , C. , Smith , B. N. and Swamy , P. M. 2002 . Interactive effects of sodium chloride and calcium chloride on the accumulation of proline and glycinebetaine in peanut (Arachis hypogaea L.) . Environmental and Experiment Botany , 47 : 1 – 10 . doi: 10.1016/S0098-8472(01)00096-X
- Gouia , H. , Chorbal , H. M. and Touraine , B. 1994 . Effects of NaCl on flows of N and mineral ions and on NO3-reduction rate within whole plants of salt-sensitive bean and salt-tolerant cotton . Plant Physiology , 105 : 1409 – 1418 .
- Goyal , S. S. , Sharma , S. K. and Rains , D. W. 2003 . Crop Production in Saline Environments: Global and Integrative Perspectives , 427 New York : Haworth Press .
- Grattan , S. R. and Grieve , C. M. 1999 . Salinity mineral nutrient relations in horticultural crops . Scientia Horticulturae , 78 : 127 – 157 . doi: 10.1016/S0304-4238(98)00192-7
- Hajer , A. S. , Malibari , A. A. , Al Zahrani , H. S. and Almaghrabi , O. A. 2006 . Responses of three tomato cultivars to seawater salinity 1. Effect of salinity on the seedling growth . African Journal of Biotechnology , 5 : 855 – 861 .
- Hussain , M. , Sharaf , M. S. & Aslam , F. 1990 . Growth, nodulation nutrient uptake and nitrogen fixation of Dalbergia sissoo as affected by different levels of nitrogen . C.F. Nitrogen fixing Tree Research Report , 8 . Faisalabad , , Pakistan : Department of Soil Science, University of Agricultural .
- Inal , A. 2002 . Growth, proline accumulation and ionic relations of tomato (Lycopersicon esculentum L.) as influenced by NaCl and Na2SO4 salinity . Turk Journal of Botany , 26 : 285 – 290 .
- Kacar , B. & Inal , A. 2008 . Plant Analysis Ankara : Nobel Publication , 892 ; ISBN: 978-605-395-036-3.
- Kafkafi , U. , Siddiqi , M. Y. , Ritchie , R. J. , Glass , A. D. M. and Ruth , T. J. 1992 . Reduction of nitrate (13NO3) influx and nitrogen (13N) translocation by tomato and melon varieties after short exposure to calcium and potassium chloride salts . Journal of Plant Nutrition , 15 : 959 – 975 . doi: 10.1080/01904169209364374
- Kafkafi , U. , Valoras , N. and Letey , J. 1982 . Chloride interaction with nitrate and phosphate nutrition in tomato (Lycopersicon esculentum L.) . Journal of Plant Nutrition , 5 : 1369 – 1385 . doi: 10.1080/01904168209363070
- Kaya , C. , Higgs , D. and Kirnak , H. 2001 . The effects of high salinity (NaCl) and supplementary phosphorus and potassium on physiology and nutrition development of spinach . Bulgarian Journal of Plant Physiology , 27 : 47 – 59 .
- Langdale , G. W. , Thomas , J. R. and Littleton , T. G. 1971 . Influence of soil salinity and nitrogen fertilizer on spinach growth . Journal of the Rio Grande Valley Horticultural Society , 25 : 61 – 66 .
- Lauchli , A. & Epstein , E. 1990 . Plant responses to saline and sodic conditions . In K.K. Tanji Agricultural Salinity Assessment and Management Manual . Manual No: 71 . Reston , , USA : ASCE Press , 113 – 137 .
- Leskovar , D. I. and Piccinni , G. 2005 . Yield and leaf quality of processing spinach under deficit irrigation . Horticultural Science , 40 : 1868 – 1870 .
- Maas , E. V. and Hoffman , G. J. 1977 . Crop salt tolerance-current assessment . Journal of the Irrigation and Drainage Division, American Society of Civil Engineers , 103 : 115 – 119 .
- Maathuis , F. J. and Amtmann , A. 1999 . K+ nutrition and Na+ toxicity: The basis of cellular K+/Na+ ratios . Annals of Botany , 84 : 123 – 133 . doi: 10.1006/anbo.1999.0912
- Mahajan , S. and Tuteja , N. 2005 . Cold, salinity and drought stress: An overview . Archives of Biochemical and Biophysics , 444 : 139 – 158 . doi: 10.1016/j.abb.2005.10.018
- Martinez-Ballesta , M. , Martinez , V. and Carvajal , M. 2000 . Regulation of water channel activity in whole roots of melon plants grown under saline conditions . Australian Journal of Plant Physiology , 27 : 685 – 691 .
- Muhammad , S. M. , Akbar , M. and Neue , U. H. 1987 . Effect of Na/Ca and Na/K ratios in saline culture solution on the growth and mineral nutrition of rice (Oryza sativa L.) . Plant and Soil , 104 : 57 – 62 . doi: 10.1007/BF02370625
- Munns , R. 2002 . Comparative physiology of salt and water stress . Plant, Cell and Environment , 25 : 239 – 250 . doi: 10.1046/j.0016-8025.2001.00808.x
- Parida , A. K. and Das , A. B. 2005 . Salt tolerance and salinity effects on plants: A review . Exotoxicol Environment , 60 : 324 – 349 . doi: 10.1016/j.ecoenv.2004.06.010
- Qadir , M. , Qureshi , R. H. and Ahmad , N. 2002 . Amelioration of calcareous saline sodic soils through phytoremediation and chemical strategies . Soil Use and Management , 18 : 381 – 385 . doi: 10.1111/j.1475-2743.2002.tb00256.x
- Renault , S. and Afifi , M. 2009 . Improving NaCl resistance of red-osier dogwood: Role of CaCl2 and CaSO4 . Plant Soil , 315 : 123 – 133 . doi: 10.1007/s11104-008-9737-7
- Renault , S. , Croser , C. , Franklin , J. A. and Zwiazek , J. J. 2001 . Effects of NaCl and Na2SO4 on red-osier dogwood (Cornus stolonifera Michx) seedlings . Plant Soil , 233 : 261 – 268 . doi: 10.1023/A:1010512021353
- Robinson , S. P. , Downton , W. J. S. , John , S. and Millhouse , A. 1983 . Photosynthesis and ion content of leaves and isolated chloroplasts of salt-stressed spinach . Plant Physiology , 73 : 238 – 242 . doi: 10.1104/pp.73.2.238
- Rubatzky , V. E. & Yamaguchi , M. 1997 . World Vegetable, Principle, Production and Nutritive Values New York Champan Hall , 843 .
- Rubio , J. S. , Garcia-Sanchez , F. , Rubio , F. and Martinez , V. 2009 . Yield, blossom-end rot incidence, and fruit quality in pepper plants under moderate salinity are affected by K and Ca fertilization . Scientia Horticulturae , 119 : 79 – 87 . doi: 10.1016/j.scienta.2008.07.009
- Salam , A. , Wyn Jhones , R. G. & Gliddon , C. J. 1992 . Genetic control of salt tolerance in Wheat (Triticum aestivum L.) . Proceeding Second Congress of the European Society for Agronomy , August 23–28 , Warwick University , UK . 132 – 133 .
- Satti , S. M. E. and Al-Yahyai , C. J. 1995 . Salinity tolerance in tomato: Implications of potassium, calcium and phosphorus . Communication in Soil Science and Plant Analysis , 26 : 2749 – 2760 . doi: 10.1080/00103629509369484
- Shannon , M. C. and Grieve , C. M. 1999 . Tolerance of vegetable crops to salinity . Scientia Horticulturae , 78 : 5 – 38 . doi: 10.1016/S0304-4238(98)00189-7
- Suarez , D. L. and Grieve , C. M. 1988 . Predicting cation ratios in corn from saline solution composition . Journal of Experimental Botany , 39 : 605 – 612 . doi: 10.1093/jxb/39.5.605
- Suleiman , S. , Wilson , C. and Grieve , C. M. 2002 . Effect of salinity and exogenously applied polyamines on growth and ion relations in spinach . Journal of Plant Nutrition , 12 : 2705 – 2717 . doi: 10.1081/PLN-120015533
- Tabatabaei , S. J. and Fakhrzad , F. 2008 . Foliar and soil application of potassium nitrate affects the tolerance of salinity and canopy growth of perennial ryegrass (Lolium perenne var. Boulevard) . American Journal of Agricultural and Biological Sciences , 3 : 544 – 550 . doi: 10.3844/ajabssp.2008.544.550
- Taiz , L. & Zeiger , Z. 2006 . Cell walls: Structure, biogenesis, and expansion . L. Taiz Z. Zeiger Plant Physiology Sunderland, MA , , USA : Sinauer Associates, Inc., Publishers , 349 – 375 .
- Tzortzakis , N. G. 2010 . Potassium and calcium enrichment alleviate salinity-induced stress in hydroponically grown endives . Horticultural Science (Prague) , 37 : 155 – 162 .
- Volkmar , K. M. , Hu , Y. and Steppuhn , H. 1998 . Physiological responses of plants to salinity: A review . Canadian Journal of Plant Science , 78 : 19 – 27 .
- Yousif , B. S. , Nguyen , N. T. , Fukuda , Y. , Hakata , H. , Okamato , Y. , Masaoka , Y. and Saneoka , H. 2010 . Effect of salinity on growth, mineral composition, photosynthesis and water relations of two vegetable crops; New Zealand Spinach (Tetragonia tetragonioides) and Water Spinach (Ipomoea aquatica) . International Journal of Agriculture and Biology , 12 : 211 – 216 .