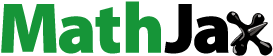
ABSTRACT
Under the current context of reducing chemical N input in agriculture, it is important to investigate better N allocation to different growth stages of crops. The plants were subjected to sufficient and reduced soil water regimes in interaction with two N application proportions applied at the vegetative and reproductive growth stages of tomato plants, respectively. In terms of the soil water impact, across the N proportion treatments, the reduced water treatments significantly decreased leaf and biomass growth by 33% and meanwhile remarkably reduced stomatal conductance of leaves, which significantly decreased water consumption by 41%. Consequently, plant WUE markedly decreased by 10.4%. The N uptake and fresh yield were considerably reduced by 37.5% and 39.3%, respectively. Regarding the N proportion effect across the soil water treatments, the lower N application of 30% at the vegetative growth stage significantly enhanced photosynthesis products allocated to fruits, which substantially improved the fresh yield by 32.9%. Furthermore, it significantly improved N accumulation by 9.0% compared to the higher N application of 70% at this stage. Conclusively, when given the certain amount of N supply, the N allocation should be reduced at the vegetative growth stage to achieve high yield and N uptake in tomato production .
Introduction
Soil water and nitrogen (N) are two main limiting factors for plant growth, development and photosynthetic capacity (Wang et al. Citation2012). The rising demand for food caused by the growth of the world population requires improved efficiency in water use and nutrient resources (Quemada and Gabriel Citation2016). However, the excessive application of N fertiliser beyond the crop demand causes undesirable consequences such as soil acidification, leaching of N into groundwater and emissions of nitrous oxide (N2O) (Harmanto et al. Citation2005; Reina-Sánchez et al. Citation2005; Levidow et al. Citation2014). Therefore, it is important to use water and N fertiliser resources efficiently, not only to optimise plants’ physiological processes and exploit their yields to the maximum, but also to reduce the negative impact of overuse of N fertiliser on the environment (Badr et al. Citation2016; Geisseler et al. Citation2019).
Previous studies have reported the effect of different N rates and application methods on tomato growth, yield and N uptake during different growth stages (Zhang et al. Citation2011; Ayankojo et al. Citation2020). It was observed that the reduction in soil water regimes under N fertigation caused the partial closure of stomata due to the decrease in plant water status, the intensification of root-to-shoot ABA signalling, and the predominant regulation of water use efficiency (WUE) by stomatal conductance rather than N in plants (Wang et al. Citation2018). Zhou et al. (Citation2020) reported that the application of N partially alleviated the reduction in transpiration (T) associated with reduced irrigation. They also observed that at the early growth stage of fruit expansion, the application of N aggravated the reduction of the plant scale WUE (WUEp) due to the reduced water availability. In contrast, at the medium and late growth stages of tomato fruits, the application of N improved the WUEp of tomatoes under reduced irrigation (Zhou et al. Citation2020). Liu et al. (Citation2013) investigated N application by drip irrigation in ten equal splits at 8-day intervals and found that N application applied in two splits saved 20–40% N compared to furrow irrigation. At the late stage of fruit growth, it was argued that a balance is needed between irrigation and N application rate (Yurtseven et al. Citation2005; Elia et al. Citation2007), as high N application can improve T and intrinsic WUE with more available irrigation water at this stage. It was found that N assimilation was higher at fruit growth than at early development stages of tomato plants (Tahi et al. Citation2007). The effect of different N application doses of 3, 7 and 14 mM showed that reduced N dose at early growth stages only affected the performance in the most severe treatment (3 mM). A decrease in N application at the anthesis stage did not affect the yield, indicating the feasibility of reducing the N dose in tomato growth without compromising performance. But it is of major importance to choose the most appropriate stage to impose this reduction without affecting, or even improving the nutritional quality of fruits (Hernández et al. Citation2020). Zotarelli et al. (Citation2009) noted that N application rate higher than 220 kg ha−1 did not improve the growth and N accumulation in fruits and shoots but substantially increased the leaching of in low water holding capacity soils. Zheng et al. (Citation2013) investigated the processing tomato grown in a clay loam soil and found that the highest yields were observed under full irrigation, whereas the highest water productivity was achieved under a moderate soil water regime. The fertigation rate of 180 kg N ha−1 at medium soil water regime produced the highest values of commercial yield, fruit size, the total yield of soluble solids, and the total protein content of tomatoes (Kuscu et al. Citation2014; Wang et al. Citation2019a).
Previous studies have shown the effect of N quantities on growth, yield and efficiency of N utilisation in conjunction with irrigation. However, less is known about the consequence of applied soil water and N application proportions during different growth stages when given the certain amount of N fertiliser. Therefore, the current study examined the interaction effect of water regime and N application proportions at the vegetative and reproductive growth stages on cherry tomato plant growth, yield, WUE and N uptake.
Materials and methods
Experimental site and materials
The experiment was carried out from April to July 2020 in the glasshouse located at the Chinese Academy of Agricultural Sciences (CAAS), Beijing, China (39.97°N, 116.33°E). During the experimental period, the glasshouse’s average day and night temperatures were 27°C and 22°C, respectively. The average air humidity was 70% and the photon flux density ranged from 450–800 μmol m−2 s−1.
The tomato (Lycopersicon esculentum L. var. Qianxi) seedlings with five leaves were obtained from the specialised cultivation house, which guaranteed their optimal state of physiological development and the absence of pests and diseases. The pots had a volume of 10.6 L with 27.5 cm in diameter at the top edge, 20 cm in diameter at the bottom and 24 cm in depth. The bottoms of the pots had small holes that allowed free drainage, but there was no water leaching from the pots during the experimental period.
The soil was collected from the plough layer (0–30 cm) at the experimental station of CAAS and was classified as sandy loam. The soil was characterised by the pH of 7.6, total C content of 9.0 g kg−1, total N content of 1.0 g kg−1, soil water holding capacity at 35% and the permanent wilting point at 9%. Before filling the pots, the soil was sieved through a 5 mm mesh. The pots were filled with 12.8 kg of air-dried soil with a bulk density of 1.20 g of dry soil cm–3.
Irrigation and N fertilisation treatments
The soil water content in the pots was controlled by irrigating the pots to predefined weights during the experimental period. Plant water consumption was computed from the water balance method (Li et al. Citation2020). All the seedlings were well watered up to 90% of the soil water holding capacity (SWHC) before the commencement of the irrigation treatments on 11 May 2020. After this, the soil water contents were irrigated to the designed soil water contents in the treatments until the end of the experiment. The treatments comprised two soil water regimes and N proportion levels. The soil water treatments (W) consisted of sufficient (90% of SWHC, designated as W90) and reduced (60% of SWHC, designated as W60) water regimes during the whole treatment period.
NH4NO3 was used as the N fertiliser. It was applied at the rate of 3 g N pot−1 with irrigation water into the soil as fertigation. The N proportion levels included 70% or 30% of the total N amount during the vegetative growth stage and 30% or 70% of the total N amount during the reproductive growth stage. The detail of the experimental treatments was shown in . The phosphorus and potassium fertilisers were mixed homogeneously at the rate of 5.75 g of KH2PO4 and 1.87 g of K2SO4 per pot to meet the macronutrient demand (Li et al. Citation2020). The experiment was carried out in a completely randomised design with eight replicates in each treatment, yielding 32 pots in total.
Table 1. The detail of the experimental treatments in the study.
Gas exchange and leaf area measurements
The leaf gas exchange parameters, including photosynthetic rate (An), stomatal conductance (gs) and transpiration, were measured weekly between 9:00 and 11:00 during the treatment period with a portable photosynthesis system (LI-6400, Lincoln, Nebraska, USA). Measurements were made at the light intensity (PAR) of 1200 μmol m−2 s−1 and a CO2 concentration of 400 ppm. The intrinsic water use efficiency (WUEint) was calculated as An/gs (Papacek et al. Citation2019). At the end of the experiment, after all the fruits were ripe on the 16th of July, the plants were harvested. The leaf area for all the leaves of each replicated plant was measured using a leaf area metre (model 3050A, Li-Cor Biosciences, NE, USA) (Li et al. Citation2020).
Root and leaf water potential
The leaves measured for leaf gas exchange were sampled and sealed in a plastic cup for the determination of leaf water potential (Ψl). The water potential of plant roots (Ψr) and leaves (Ψl) was determined by a Dewpoint Potentia Meter (METER Group, Pullman, USA) during the daytime at around 25°C room temperature. Some root segments (about 0.5 g) were excised from the root system in each pot. The root segments were tapped to remove adhering soil particles, briefly blotted with absorbent paper, and immediately placed and sealed inside a plastic sample cup (<10 s) for root water potential (Ψr) measurement.
Biomass, nitrogen concentration and WUE
At the end of the experiment, plants were divided into leaves, stems, fruits and roots. They were weighed on a laboratory balance (ME104, Mettler Toledo Co., Ltd., Shanghai, China) with an accuracy of 0.001 g. Subsequently, they were subjected to a drying process at 70°C in an oven (WGL-230B, Taiste Instrument Co., Ltd., Tianjin, China) to determine the dry biomass. After grinding the plant material into fine powder, the plant samples were analysed for total N concentration using an Elemental Analyser System (vario PYRO cube, Elementar Analysensysteme GmbH, Germany) (Wang et al. Citation2018). The N accumulation was calculated by the N concentration and dry biomass. The plant water use efficiency (WUE) was computed as the ratio between the above-ground dry biomass and the water consumption during the treatment period.
Statistical analyses
Data were analysed by one-way and two-way ANOVA using SPSS version 22.0 (IBM Corporation, New York, USA). The general linear model was used in the ANOVA. Duncan’s multiple range test was applied to evaluate the differences between the treatments at a significance level of 5%.
Results
Plant water status
The leaf water potential was not significantly affected either by soil water regimes or N proportion levels (), though the leaf water potential decreased under the reduced water treatments. In contrast, the analysis across the N proportion treatments showed that the root water potential was significantly lower under the reduced water treatments than the well-watered treatments. Nevertheless, the leaf and root water potential were similar between the N proportion levels.
Table 2. Effect of soil water regimes and N application proportions on leaf and root water potential (at harvest), averaged photosynthetic rate, stomatal conductance and intrinsic water use efficiency (WUE) of tomato plants during the treatment period (ANOVA results included).
Leaf gas exchange
The photosynthetic rate, stomatal conductance and intrinsic water use efficiency (WUEint) were almost not affected significantly by soil water or N proportion treatments () except for the significant reduction in stomatal conductance under the reduced water treatments compared with the well-watered treatments across the N proportion levels. The reduced water treatments slightly increased WUEint compared with the well-watered treatments.
Leaf area, dry biomass, water consumption, WUE and fresh yield
The leaf area was significantly affected by the soil water treatments; however, it was similar between the two N proportion levels (). When analsed cross the N proportions, the total leaf area was significantly increased by more than 49.6% under the well-watered treatments (W90) compared to the reduced water treatments (W60). Under the well-watered treatment, a reduced proportion of N application during the vegetative growth stage reduced the leaf area by 12.3%. The leaf area was lowest in the treatment with reduced water regime and a high proportion of N application at the vegetative growth stage.
Table 3. Effect of soil water regimes and proportions of nitrogen (N) application on leaf area, dry biomass, water consumption, plant water use efficiency (WUE) and fresh yield of tomato plants at harvest (ANOVA results included).
The dry biomass, water consumption and plant WUE were decreased by 30.3%, 38.9%, and 30.0%, respectively, for the high N proportion treatment at the vegetative growth stage under the reduced water regime condition, and by 36.1%, 42.7% and 35.9%, respectively, for the low N proportion treatment at the vegetative growth stage under water stress ().
The fresh yield was significantly decreased by the reduced water treatments (). Under the well-watered treatment, a reduced proportion of N application at the vegetative growth stage significantly improved the fresh yield, which was 66.9% higher than the increased proportion of N application at the early growth stage. There was a significant interaction between soil water regimes and N proportion levels on the fresh yield.
The allocation of dry biomass was significantly affected by soil water treatments (). Under the well-watered condition, significantly higher dry biomass was transformed to fruits when the N application proportion was reduced at the vegetative growth stage, while considerably greater dry biomass was allocated to stems for plants under the high N application proportion level at the vegetative growth stage. Under the reduced water treatment, the plants gave more biomass to leaves when the reduced proportion of N was applied during the vegetative growth stage.
Nitrogen concentration and accumulation
The N concentrations in the stem, leaf and fruit were significantly affected by the N proportions and there was an interaction between N and water treatments (). The N concentrations in the stems, leaves and fruits were significantly reduced and were the lowest in the treatment with reduced water and a high proportion of N application at the vegetative growth stage.
Figure 2. Effect of soil water regimes and N application proportions on nitrogen concentration in the stem, leaf and fruit and nitrogen accumulation in tomato plants at harvest. The different letters above the columns indicate significant differences among the treatments at p < 0.05. The detail of the treatments refers to .
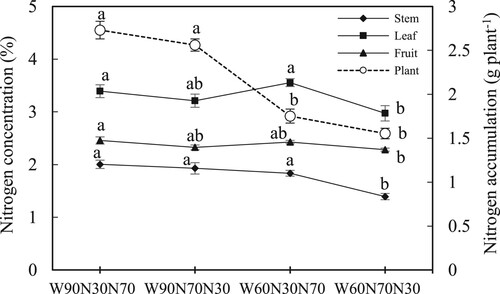
The N uptake was significantly affected by both soil water and N proportion treatments (). Across the N proportion levels, the reduced water treatment significantly reduced N uptake by 37.5% compared with the well-watered treatments. The analysis across the soil water levels showed that the reduced proportion of N application increased N accumulation by 9.0% compared to the higher proportion of N application at the early growth stage.
Discussion
Water availability is a critical factor that directly influences leaf area development and biomass accumulation of plants (Hatfield and Dold Citation2019). Not to mention that leaves as essential organs in maintaining nutrition and growth of plants are susceptible to environmental conditions and more particularly to the reduced soil water conditions, affecting their respiration and photosynthesis efficiency and synthesis of organic matters (Shao et al. Citation2008). It was found that plants under the well-watered treatments presented significantly greater leaf area development and dry biomass than under the reduced water treatments (). Medyouni et al. (Citation2021) reported that water deprivation mitigated the number, widths and length of tomato leaves. The reduction in leaf area under the reduced water regime conditions was attributed to an appreciable decline in the mitotic activity of epidermal cells (or the total number of leaf cells) and the size of cells as well as early leaf senescence (Granier and Tardieu Citation1999; Farooq et al. Citation2009; Wang et al. Citation2019b). Among them, however, reduction in the total number of leaf cells has been introduced as the leading cause of water stress-induced leaf area reduction in Sesbania aculeate, Phaseolus vulgaris (Ashraf and Iram Citation2005), Sesamum indicum (Hassanzadeh et al. Citation2009), Pennisctum glaucum L (Kusaka et al. Citation2005) and Solanum lycopersicum (Medyouni et al. Citation2021).
In the present study, the reduced N proportion during vegetative growth also decreased the leaf area compared with the increased supply of N proportion under the well-watered treatment (). Nonetheless, under the reduced water treatment, the leaf area was similar between the N application levels. Our result was analogous to the findings of Ullah et al. (Citation2021) with regards to significant mitigation in the leaf area of tomato plants suffering from decreased water supply and N application. This indicates that maintaining high water and N supply is essential to improve plant vegetative growth (Ellsworth et al. Citation2017). Both the growth of the foliar area and the roots of plants determine the water consumption, taking into account the availability of water in the soil (Buttaro et al. Citation2015). In the current study, the reduced water treatments (W60) caused a reduction in leaf area and this significantly decreased water consumption (). This reduction might be correlated to the partial decrease in stomatal conductance (or transpiration rate) under the reduced water regime conditions (). Transpiration rate in crops has been known as the main factor of water consumption (Bernacchi and VanLoocke Citation2015). Notably, stomatal area index (SAI), which corresponds to parameters, such as stomatal density, size and conductance, was coined as a critical index for estimation of drought tolerance index in plant species (Hennig et al. Citation2015). This result confirmed the conception that water deprivation affected overall foliage structure and water consumption, as indicated earlier by Merrien et al. (Citation1981) and recently by Papacek et al. (Citation2019).
The fresh yield was significantly decreased by the reduced water treatments (). Similarly, Cantero-Navarro et al. (Citation2016) reported that reduced water treatment significantly decreased the yield of fresh tomatoes, indicating that water availability is crucial for the development of both plant vegetative growth and fruit formation. Cantore et al. (Citation2016) noted that water scarcity significantly affected the yield of tomato plants, and a decrease in the commercial yield of 52.7% was observed when the water supply was reduced by 50% due to the reduction of both the weight and the number of fruits. It is noteworthy that under the well-watered condition, the reduced proportion of N fertiliser at the vegetative growth stage considerably improved the fresh yield of fruits compared with the larger proportion of N application at the early growth stage, indicating that N application should be reduced during the vegetative growth period to achieve high fresh yields for tomato production (). This was ascribed to the significantly enhanced photosynthate allocated to fruits instead of biomass growth when the reduced N proportion was applied during the early growth stage (). On the contrary, higher application of N proportion during the vegetative growth stage significantly decreased fresh fruit weight while considerably increased the allocation of biomass into stems despite sufficient water supply. In agreement with this, Zhou et al. (Citation2020) suggested abundant water supply without N excess at the early growth stage, however, the amount of N fertiliser and soil water contents could be increased at later growth stages to promote tomato yield and WUE. Thus, the fresh fruit yield was regulated by the interactions of soil water content and N application quantity during different growth stages.
Several previous studies and reports have shown that reducing water application could improve plant WUE (Martìnez et al. Citation2003; Evans and Sadler Citation2008; Fan et al. Citation2018; Hatfield and Dold Citation2019; Ahmad et al. Citation2021; Ullah et al. Citation2021). The present study revealed that the reduced water treatments significantly decreased stomatal conductance across the N application proportion levels (). Consequently, plant water use dropped by 40.8% under the water deficit condition and plant dry matter production was reduced by 33.2%. As a result, the reduced water treatments significantly decreased plant WUE by 10.4% compared with the well-watered treatments (). Similarly, previous studies have noted that the reduced soil water availability and decreased fertilisation reduced the growth, yield and WUE (Gebremariam and Tesfay Citation2019; Ullah et al. Citation2021). Additionally, a combination of 100% NPK fertilisation rate and slightly deficient irrigation (80% of crop evapotranspiration) resulted in the highest WUE and fruit quality compared with other water and fertilisation treatments (Abdelhady et al. Citation2017). Du et al. (Citation2017) investigated the effects of three doses of irrigation at 50%, 75% and 100% of field capacity and N application rates at 150, 250 and 350 kg N ha−1 on tomato growth in the greenhouse. They observed that the treatment at 75% of field capacity and with 250 kg N ha−1 resulted in optimal tomato yield, fruit quality and WUE. Wang and Xing (Citation2016) showed that the fruit yield and WUE increased with elevating fertilisation rate, and an irrigation quantity of 151–208 mm and a fertiliser quantity of 454–461 kg/ha−1 was recommended for drip fertigation of tomatoes in the greenhouse. Nonetheless, in terms of N proportions during the growth stages, the results of the present study indicated that the biomass production, water consumption and plant WUE were mainly affected by the water regimes without significant impact by the N proportions ().
Wang et al. (Citation2019c) found that increased irrigation and fertilisation improved leaf nutrient content and accumulation as well as nutrient transfer to fruits. Soil water stress can decrease soil nutrient availability due to mineralisation (Fierer and Schimel Citation2002; Sanaullah et al. Citation2012). It can also restrict the uptake ability of the root system for nutrients (Bassirirad Citation2000; Christophe et al. Citation2011). Furthermore, water consumption directly influences the amount of nutrients that plants can incorporate into their system (Bista et al. Citation2018). In addition, by presenting a poorly developed foliar system (), the processing capacity of the nutrients assimilated by the root system is reduced and insufficient. As a result, it was observed that N uptake by plants under the reduced water treatments was significantly reduced (). In agreement with our findings, recent studies on tomato and wheat (Triticum aestivum L.) plants have shown that drought stress significantly reduced the growth and biomass accumulation as well as the mineral uptake (Ahanger and Agarwal Citation2017; Ahanger et al. Citation2021). The reduced N proportions at early vegetative growth significantly increased N accumulation by 9.0% compared to the higher N proportions applied at this stage across the soil water treatments.
Author contribution statement
YW designed the experiment. EE, TM and CW performed the experiment. AS, EE, TM and YW analysed the data and drafted the manuscript. PZ, EB, BBK and AD participated in the interpretation of results. YW, EB, BBK, PZ, AD, AS, EE and TM reviewed and edited the manuscript All authors approved the final submitted version of the manuscript.
Data availability statement
The data presented in this study are available on request from the corresponding author.
Disclosure statements
We confirm that there are no known conflicts of interest associated with this publication and there has been no significant financial support for this work that could have influenced its outcome. We confirm that the manuscript has been read and approved by all named authors and that there are no other persons who satisfied the criteria for authorship but are not listed.
Additional information
Funding
Notes on contributors
Elio Enrique Trujillo Marín
Elio Enrique Trujillo Marín B.Sc. He is currently working on water and nitrogen interaction for horticultural and cereal crops.
Chao Wang
Chao Wang Ph.D. His research is focusing on improving water and nutrient use efficiencies under different agronomic practices.
Ashutus Singha
Ashutus Singha Ph.D. He is presently researching on the physiological responses of crops to multi-abiotic stresses.
Elke Bloem
Elke Bloem Ph.D. She is a senior scientist responsible for research on plant stress metabolism, soil biology and plant nutrition.
Peiman Zandi
Peiman Zandi Ph.D. He is currently an assistant professor and is specialized in stress physiology, biotic/abiotic stresses and agroecology.
Beata Barabasz-Krasny
Beata Barabasz-Krasny Ph.D. She is an associate professor with main scientific interests in anthropogenic changes in vegetation and the dynamics of plant communities.
Aminu Darma
Aminu Darma Ph.D. He is currently researching on sorption mechanisms, biotransformation and mobilisation of heavy metals
Yaosheng Wang
Yaosheng Wang Ph.D. He is a full professor with the expertise in plant ecophysiology at different levels under biotic and abiotic stresses, plant stress biology and precision and smart agriculture.
References
- Abdelhady SA, El-Azm NAI, El-Kafafi ESH. 2017. Effect of deficit irrigation levels and NPK fertilization rates on tomato growth, yield and fruits quality. Middle East J Agric Res. 6(3):587–604.
- Ahanger MA, Agarwal RM. 2017. Potassium up-regulates antioxidant metabolism and alleviates growth inhibition under water and osmotic stress in wheat (Triticum aestivum L). Protoplasma. 254(4):1471–1486. https://doi.org/10.1007/s00709-016-1037-0.
- Ahanger MA, Qi M, Huang Z, Xu X, Begum N, Qin C, et al. 2021. Improving growth and photosynthetic performance of drought stressed tomato by application of nano-organic fertilizer involves up-regulation of nitrogen, antioxidant and osmolyte metabolism. Ecotoxicol Environ Saf. 216:112195. https://doi.org/10.1016/j.ecoenv.2021.112195.
- Ahmad HS, Imran M, Ahmad F, Rukh S, Ikram RM, Rafique HM, et al. 2021. Improving water use efficiency through reduced irrigation for sustainable cotton production. Sustainability. 13:4044. https://doi.org/10.3390/su13074044.
- Ashraf M, Iram AI. 2005. Drought stress induced changes in some organic substances in nodules and other plant parts of two potential legumes differing in salt tolerance. Flora. 200(6):535–546. https://doi.org/10.1016/j.flora.2005.06.005.
- Ayankojo IT, Morgan KT, Kadyampakeni DM, Liu GD. 2020. Tomato growth, yield, and root development, soil nitrogen and water distribution as affected by nitrogen and irrigation rates on a Florida sandy soil. J Am Soc Hortic Sci. 55(11):1744–1755. https://doi.org/10.21273/HORTSCI15177-20.
- Badr MA, Abou-Hussein SD, El-Tohamy WA. 2016. Tomato yield, nitrogen uptake and water use efficiency as affected by planting geometry and level of nitrogen in an arid region. Agric Water Manag. 169:90–97. https://doi.org/10.1016/j.agwat.2016.02.012.
- Bassirirad H. 2000. Kinetics of nutrient uptake by roots: responses to global change. New Phytol. 147:155–169. https://doi.org/10.1046/j.1469-8137.2000.00682.x.
- Bernacchi CJ, VanLoocke A. 2015. Terrestrial ecosystems in a changing environment: a dominant role for water. Annu Rev Plant Biol. 66:599–622. https://doi.org/10.1146/annurev-arplant-043014-114834.
- Bista DR, Heckathorn SA, Jayawardena DM, Mishra S, Boldt JK. 2018. Effects of drought on nutrient uptake and the levels of nutrient-uptake proteins in roots of drought-sensitive and -tolerant grasses. Plants. 7(2):28. https://doi.org/10.3390/plants7020028.
- Buttaro D, Santamaria P, Signore A, Cantore V, Boari F, Montesano FF, et al. 2015. Irrigation management of greenhouse tomato and cucumber using tensiometer: Effects on yield, quality and water use. Agric Agric Sci Procedia. 4:440–444. https://doi.org/10.1016/j.aaspro.2015.03.050.
- Cantero-Navarro E, Romero-Aranda R, Fernández-Muñoz R, Martínez-Andújar C, Pérez-Alfocea F, Albacete A. 2016. Improving agronomic water use efficiency in tomato by rootstock-mediated hormonal regulation of leaf biomass. Plant Sci. 251:90–100. https://doi.org/10.1016/j.plantsci.2016.03.001.
- Cantore V, Lechkar O, Karabulut E, Sellami MH, Albrizio R, Boari F, et al. 2016. Combined effect of deficit irrigation and strobilurin application on yield, fruit quality and water use efficiency of “cherry” tomato (Solanum lycopersicum L.). Agric Water Manag. 167:53–61. https://doi.org/10.1016/j.agwat.2015.12.024.
- Christophe S, Jean-Christophe A, Annabelle L, Alain O, Marion P, Anne-Sophie V. 2011. Plant N fluxes and modulation by nitrogen, heat and water stresses: A review based on comparison of legumes and non-legume plants. In: A. Shanker, B. Venkateswarlu, editor. Abiotic stress in plants—mechanisms and adaptations. Rijeka, Croatia: In Tech; p. 79–118.
- Du YD, Cao HX, Liu SQ, Gu XB, Cao YX. 2017. Response of yield, quality, water and nitrogen use efficiency of tomato to different levels of water and nitrogen under drip irrigation in Northwestern China. J Integr Agric. 16(5):1153–1161. https://doi.org/10.1016/S2095-3119(16)61371-0.
- Elia A, Conversa G, Rotonda PL, Montemurro F. 2007. Nitrogen level effect on yield and quality of fertigated processing tomato in Southern Italy. Acta Hortic. 758:235–240. https://doi.org/10.17660/ActaHortic.2007.758.27.
- Ellsworth PZ, Ellsworth PV, Cousins AB. 2017. Relationship of leaf oxygen and carbon isotopic composition with transpiration efficiency in the C4 grasses Setaria viridis and Setaria italica. J Exp Bot. 68(13):3513–3528. https://doi.org/10.1093/jxb/erx185.
- Evans RG, Sadler EJ. 2008. Methods and technologies to improve efficiency of water use. Water Resour Res. 44(7):W00E04. https://doi.org/10.1029/2007WR006200.
- Fan Y, Wang C, Nan Z. 2018. Determining water use efficiency of wheat and cotton: a meta-regression analysis. Agric Water Manag. 199:48–60. https://doi.org/10.1016/j.agwat.2017.12.006.
- Farooq M, Wahid A, Kobayashi N, Fujita D, Basra SMA. 2009. Plant drought stress: effects, mechanisms and management. Agron Sustainable Dev. 29:185–212. https://doi.org/10.1051/agro:2008021.
- Fierer N, Schimel JP. 2002. Effects of drying–rewetting frequency on soil carbon and nitrogen transformations. Soil Biol Biochem. 34:777–787. https://doi.org/10.1016/S0038-0717(02)00007-X.
- Gebremariam M, Tesfay T. 2019. Optimizing irrigation water and N levels for higher yield and reduced blossom end rot incidence on tomato. Int J Agron. 2019:8125063. https://doi.org/10.1155/2019/8125063.
- Geisseler D, Aegerter BJ, Miyao EM, Turini T, Cahn MD. 2019. Nitrogen in soil and subsurface drip-irrigated processing tomato plants (Solanum lycopersicum L.) as affected by fertilization level. Sci Hortic. 261:108999. https://doi.org/10.1016/j.scienta.2019.108999.
- Granier C, Tardieu F. 1999. Water deficit and spatial pattern of leaf development. variability in responses can be simulated using a simple model of leaf development. Plant Physiol. 119(2):609–619. https://doi.org/10.1104/pp.119.2.609.
- Harmanto, Salokhe VM, Babel MS, Tantau HJ. 2005. Water requirement of drip irrigated tomatoes grown in greenhouse in tropical environment. Agric Water Manag. 71(3):225–242. https://doi.org/10.1016/j.agwat.2004.09.003.
- Hassanzadeh M, Asghari A, Jamaati-E-Somarin SH, Saeidi M, Zabihi-E-Mahmoodabad R, Hokmalipour S. 2009. Effects of water deficit on drought tolerance indices of sesame (Sesamum indicum L.) genotypes in Moghan region. J Environ Sci. 3(1):116–121. https://doi.org/10.3923/rjes.2009.116.121.
- Hatfield JL, Dold C. 2019. Water-use efficiency: advances and challenges in a changing climate. Front Plant Sci. 10:103. https://doi.org/10.3389/fpls.2019.00103.
- Hennig A, Kleinschmit JRG, Schoneberg S, Löffler S, Janßen A, Polle A. 2015. Water consumption and biomass production of protoplast fusion lines of poplar hybrids under drought stress. Front Plant Sci. 6:330. https://doi.org/10.3389/fpls.2015.00330.
- Hernández V, Hellín P, Fenoll J, Flores P. 2020. Impact of nitrogen supply limitation on tomato fruit composition. Sci Hortic. 264:109173. https://doi.org/10.1016/j.scienta.2020.109173.
- Kusaka M, Lalusin AG, Fujimura T. 2005. The maintenance of growth and turgor in pearl millet (Pennisetum glaucum [L.] Leeke) cultivars with different root structures and osmo-regulation under drought stress. Plant Sci. 168:1–14. https://doi.org/10.1016/j.plantsci.2004.06.021.
- Kuscu H, Turhan A, Ozmen N, Aydinol P, Demir AO. 2014. Optimizing levels of water and nitrogen applied through drip irrigation for yield, quality, and water productivity of processing tomato (Lycopersicon esculentum Mill.). Hortic Environ Biotechnol. 55:103–114. https://doi.org/10.1007/s13580-014-0180-9.
- Levidow L, Zaccaria D, Maia R, Vivas E, Todorovic M, Scardigno A. 2014. Improving water-efficient irrigation: prospects and difficulties of innovative practices. Agric Water Manag. 146:84–94. https://doi.org/10.1016/j.agwat.2014.07.012.
- Li L, Ma HY, Xing JY, Liu FL, Wang YS. 2020. Effects of water deficit and nitrogen application on leaf gas exchange, phytohormone signaling, biomass and water use efficiency of oat plants. J Plant Nutr Soil Sci. 183:695–704. https://doi.org/10.1002/jpln.202000183.
- Liu X, Fan Y, Long J, Wei R, Kjelgren R, Gong C, et al. 2013. Effects of soil water and nitrogen availability on photosynthesis and water use efficiency of Robinia pseudoacacia seedlings. J Environ Sci. 25(3):585–595. https://doi.org/10.1016/S1001-0742(12)60081-3.
- Martìnez JP, Ledent JF, Bajji M, Kinet JM, Lutts S. 2003. Effect of water stress on growth, Na+ and K+ accumulation and water use efficiency in relation to osmotic adjustment in two populations of Atriplex halimus L. Plant Growth Regul. 41:63–73. https://doi.org/10.1023/A:1027359613325.
- Medyouni I, Zouaoui R, Rubio E, Serino S, Ahmed HB, Bertin N. 2021. Effects of water deficit on leaves and fruit quality during the development period in tomato plant. Food Sci Nutr. 9(4):1949–1960. https://doi.org/10.1002/fsn3.2160.
- Merrien A, Blanchet R, Gelfi N. 1981. Relationships between water supply, leaf area development and survival, and production in sunflower (Helianthus annuus. L.). Agronomie. 10(1):917–922.
- Papacek M, Christmann A, Grill E. 2019. Increased water use efficiency and water productivity of arabidopsis by abscisic acid receptors from Populus canescens. Ann Bot. 124(4):581–589. https://doi.org/10.1093/aob/mcy225.
- Quemada M, Gabriel JL. 2016. Approaches for increasing nitrogen and water use efficiency simultaneously. Glob Food Sec. 9:29–35. https://doi.org/10.1016/j.gfs.2016.05.004.
- Reina-Sánchez A, Romero-Aranda R, Cuartero J. 2005. Plant water uptake and water use efficiency of greenhouse tomato cultivars irrigated with saline water. Agric Water Manag. 78(1–2):54–66. https://doi.org/10.1016/j.agwat.2005.04.021.
- Sanaullah M, Rumpel C, Charrier X, Chabbi A. 2012. How does drought stress influence the decomposition of plant litter with contrasting quality in a grassland ecosystem? Plant Soil. 352:277–288. https://doi.org/10.1007/s11104-011-0995-4.
- Shao HB, Chu LY, Jaleel CA, Zhao CX. 2008. Water-deficit stress-induced anatomical changes in higher plants. C R Biol. 331(3):215–225. https://doi.org/10.1016/j.crvi.2008.01.002.
- Tahi H, Wahbi S, Wakrim R, Aganchich B, Serraj R, Centritto M. 2007. Water relations, photosynthesis, growth and water-use efficiency in tomato plants subjected to partial rootzone drying and regulated deficit irrigation. Plant Biosyst. 141(2):265–274. https://doi.org/10.1080/11263500701401927.
- Ullah I, Mao H, Rasool G, Gao H, Javed Q, Sarwar A, et al. 2021. Effect of deficit irrigation and reduced N fertilization on plant growth, root morphology and water use efficiency of tomato grown in soilless culture. Agronomy. 11:228. https://doi.org/10.3390/agronomy11020228.
- Wang C, He J, Zhao TH, Cao Y, Wang G, Sun B, et al. 2019b. The smaller the leaf is, the faster the leaf water loses in a temperate forest. Front Plant Sci. 10:58. https://doi.org/10.3389/fpls.2019.00058.
- Wang X, Yun J, Shi P, Li Z, Li P, Xing Y. 2019a. Root growth, fruit yield and water use efficiency of greenhouse grown tomato under different irrigation regimes and nitrogen levels. Plant Growth Regul. 38(2):400–415. https://doi.org/10.1007/s00344-018-9850-7.
- Wang C, Wu S, Tankari M, Zhang X, Li L, Gong D, et al. 2018. Stomatal aperture rather than nitrogen nutrition determined water use efficiency of tomato plants under nitrogen fertigation. Agric Water Manag. 209:94–101. https://doi.org/10.1016/j.agwat.2018.07.020.
- Wang HB, Cao HX, Hao SX, Pan XY. 2019c. Responses of plant nutrient and photosynthesis in greenhouse tomato to water-fertilizer coupling and their relationship with yield. Sci Agric Sin. 52(10):1761–1771 (in Chinese).
- Wang M, Shi S, Lin F, Hao Z, Jiang P, Dai G. 2012. Effects of soil water and nitrogen on growth and photosynthetic response of Manchurian ash (Fraxinus mandshurica L.) seedlings in northeastern China. PLoS One. 7(2):e30754. https://doi.org/10.1371/journal.pone.0030754.
- Wang XH, Xing YY. 2016. Evaluation of the effect of irrigation and fertilization by drip fertigation on tomato yield and water use efficiency in greenhouse. Int J Agron. 2016:3961903. https://doi.org/10.1155/2016/3961903.
- Yurtseven E, Kesmez GD, Ünlükara A. 2005. The effects of water salinity and potassium levels on yield, fruit quality and water consumption of a native central Anatolian tomato species (Lycopersicon esculantum). Agric Water Manag. 78(1-2):128–135. https://doi.org/10.1016/j.agwat.2005.04.018.
- Zhang TQ, Liu K, Tan CS, Warner J, Wang YT. 2011. Processing tomato nitrogen and soil residual nitrogen as influenced by nitrogen and phosphorus addition with drip-fertigation. Soil Sci Soc Am J. 75(2):738–745. https://doi.org/10.2136/sssaj2009.0365.
- Zheng J, Huang G, Jia D, Wang J, Mota M, Pereira LS, et al. 2013. Responses of drip-irrigated tomato (Solanum lycopersicum L.) yield, quality and water productivity to various soil matric potential thresholds in an arid region of Northwest China. Agric Water Manag. 129:181–193. https://doi.org/10.1016/j.agwat.2013.08.001.
- Zhou H, Kang S, Li F, Du T, Shukla MK, Li X. 2020. Nitrogen application modified the effect of deficit irrigation on tomato transpiration, and water use efficiency in different growth stages. Sci Hortic. 263:109112. https://doi.org/10.1016/j.scienta.2019.109112.
- Zotarelli L, Dukes MD, Scholberg JMS, Muñoz-Carpena R, Icerman J. 2009. Tomato nitrogen accumulation and fertilizer use efficiency on a sandy soil, as affected by nitrogen rate and irrigation scheduling. Agric Water Manag. 96(8):1247–1258. https://doi.org/10.1016/j.agwat.2009.03.019.