Abstract
In the brewing of high-quality sake such as Daiginjo-shu, the cerulenin-resistant sake yeast strains with high producing ability to the flavor component ethyl caproate have been used widely. Genetic stability of sake yeast would be important for the maintenance of both fermentation properties of yeast and quality of sake. In eukaryotes, checkpoint mechanisms ensure genetic stability. However, the integrity of these mechanisms in sake yeast has not been examined yet. Here, we investigated the checkpoint integrity of sake yeasts, and the results suggested that a currently used cerulenin-resistant sake yeast had a defect in spindle assembly checkpoint (SAC). We also isolated a spontaneous cerulenin-resistant sake yeast FAS2-G1250S mutant, G9CR, which showed both high ethyl caproate-producing ability and integrity/intactness of the checkpoint mechanisms. Further, morphological phenotypic robustness analysis by use of CalMorph supported the genetic stability of G9CR. Finally, we confirmed the high quality of sake from G9CR in an industrial sake brewing setting.
Graphical Abstract
Cerulenin (lower structure)-resistant sake yeast G9CR (green/cell wall, red/actin, blue/nucleus) with both high productivity of ethyl caproate (upper structure) and normal checkpoint integrity.

The Japanese alcoholic beverage sake has achieved popularity among many consumers around the world. To further elevate the popularity of sake, various processes in sake brewing have been improved. The high-quality sake Daiginjo-shu, which is produced from highly polished rice (less than a 50% polishing ratio, which is the weight ratio of polished rice to brown rice), has contributed to increased popularity of sake, as evidenced by new sake consumers.
Ethyl caproate, one of the major flavor components in Daiginjo-shu, is synthesized from ethanol and caproic acid (C6 medium-chain fatty acid) by budding yeast (sake yeast). In yeast, caproic acid is synthesized from acetyl-CoA, malonyl-CoA, and NADPH in the fatty acid synthesis pathway. Budding yeast fatty acid synthetase, one of the key enzymes for the synthesis of fatty acids, has the subunit composition of α6β6, consisting of 2 kinds of multifunctional polypeptides, an α subunit (encoded by FAS2) and a β subunit (FAS1).Citation1) Previously, sake yeast mutants with high ethyl caproate-producing ability were isolated as yeast resistant to cerulenin, an inhibitor of fatty acid synthesis.Citation2) It was also reported that in the cerulenin-resistant mutant with high productivity for ethyl caproate, glycine-1250 (GGT: the underlined G-3748th nucleotide is changed to A) in the α subunit (FAS2) of fatty acid synthetase is mutated to serine (AGT) and that this mutation leads to an increase in the amount of caproic acid, a precursor of ethyl caproate.Citation2,3) The high production of ethyl caproate by the FAS2-G1250S mutation has been confirmed by self-cloning GM (genetically modified) method.Citation3) Currently, the cerulenin-resistant sake yeast strain K1801 (bred by mating of K9 MATα with the cerulenin-resistant K1601 MATa in the Brewing Society of Japan) with high ability to produce ethyl caproateCitation4) have been used widely in high-quality sake brewing, such as Daiginjo-shu.
In the cell cycle of eukaryotes, a checkpoint mechanism ensures the chromosomal stability. The DNA-integrity checkpoint (DIC) and spindle assembly checkpoint (SAC) are essential for the maintenance of genome integrity in the event of perturbed DNA replication and spindle assembly abnormality (such as monopolar attachment between spindle and kinetochore), respectively.Citation5,6) A defect in either checkpoint mechanism causes abnormalities in chromosome segregation and genome ploidy, inducing cancerization. In sake brewing, genetic stability (integrity/intactness of the checkpoint mechanisms) of sake yeast would be important for the maintenance of both fermentation properties of the yeast and quality of the final product, sake. However, this integrity in sake yeast has not been examined yet.
In fission yeast, the relationship between a defect in fatty acid synthesis and an abnormality in nuclear division was reported.Citation7) Two temperature-sensitive mutants, cut6 and lsd1, showed a defect in nuclear division, with lsd1 producing daughter nuclei of different size (lsd, large and small daughter). The cut6+ and lsd1+ genes encode, respectively, acetyl-CoA carboxylase and fatty acid synthetase (α subunit, Fas2), the key enzymes for fatty acid synthesis. Further, cerulenin caused the lsd phenotype in wild-type (WT) cells. This report indicated that fatty acid synthesis is important for normal nuclear division in mitosis in fission yeast.Citation7) However, in budding yeast, the effect of the cerulenin-resistant FAS2-G1250S mutation on nuclear division has not been reported yet.
In this study, we investigated the integrity/intactness of the checkpoint mechanisms in sake yeasts (budding yeast), and the results suggested that a currently used cerulenin-resistant sake yeast had a defect in SAC function. We also isolated a spontaneous cerulenin-resistant FAS2-G1250S sake yeast with both high productivity for ethyl caproate and integrity/intactness of checkpoint functions and confirmed the high quality of sake fermented from the isolated strain in an industrial sake brewing setting.
Materials and methods
Strains
The laboratorial and industrial budding yeast Saccharomyces cerevisiae strains were used in this study. The laboratorial strains were the following: WT, temperature-sensitive rad53 (DIC defective),Citation8,9) bub1-deletion mutant (Δbub1; SAC defective),Citation10,11) and rim15-deletion mutant (Δrim15; stress sensitive). The gene deletion library of the BY4741 strain background (Invitrogen) was used in this study. The industrial strains used were as follows: K7,Citation12) K1801 (bred by mating of K9 MATα with the cerulenin-resistant K1601 MATa in the Brewing Society of Japan),Citation4) and G9 (original strain of Niigata Prefectural Sake Research Institute).Citation13)
Media and drugs
The following yeast media were used in this study: YPD medium (for general pre-culture/culture), koji-extract (Be 5/sake meter (Sm) -50) medium (for pre-culture of fermentation test), and the YNB(Yeast Nitrogen Base w/o amino acids, DifcoTM)solid medium containing 2% glucose (for the screening for cerulenin-resistant mutants). The following drugs were used in this study: the fatty acid synthesis inhibitor cerulenin (WAKO No.031-18181, for mutant screening),Citation14) the microtubule-destabilizing drug benomyl (SIGMA No.1001328433, for investigating the SAC function),Citation10,11) and the DNA synthesis inhibitor hydroxyurea (HU) (SIGMA No.1001429575, for investigating the DIC function).Citation8,9)
Drug sensitivity
After the pre-culture in YPD medium at 28 °C for 12 h, the number of yeast cells was adjusted to 1.0 × 107 cells/mL. The cell suspension was diluted serially and inoculated onto YPD plates, each containing one of various drugs: 0.1–2.0 μg/mL cerulenin, 10–30 μg/mL benomyl, or 100–200 mM HU. After incubation at 28 °C for 3–5 days, the cell growth (drug sensitivity) on the plate was observed.
Investigation of checkpoint function
Yeast cells were cultured in YPD medium at 28 °C for 12 h, and then, the cells were inoculated freshly into YPD medium at 5.0 × 105 cells/mL. After incubation at 28 °C for 4 h, each drug (final concentration 15 μg/mL benomy or 200 mM HU) was added to the cell culture at early log phase (1.0–2.0 × 106 cells/mL). Then, during incubation at 28 °C, at various times (0, 2, 4, 6 h), the cell number was counted by use of a Sysmex F820 Hematology Analyzer, and the cells were sampled and spread on a YPD plate. After incubation at 28 °C for 3 days, the number of developed colonies was counted for measurement of cell viability.
Isolation of spontaneous cerulenin-resistant yeast
The cerulenin-resistant mutant (FAS2-G1250S) was isolated by performing the following 3 steps (Fig. (A)): As the first screening, 105 cells of the freshly cultured sake yeast strain G9 were spread on a YNB (w/o amino acids) plate (total 164 plates) containing 0.4 μg/mL cerulenin and incubated at 30 °C for 5–6 days. The growing colonies were isolated as cerulenin-resistant mutants. As the second screening, the production of free fatty acids (FFA) by 3056 isolated mutants was examined by use of the previously reported FFA assay method (Patent No. P2012-231751A).Citation15) It has been reported that 2 fatty acids, caproic and caprylic acids, were the major FFAs in the sake produced by the cerulenin-resistant sake yeast with high productivity of ethyl caproate and that the content ratio of caproic acid to total FFAs in the sake was about 83% and the sum of the content ratios of caproic acid and caprylic acid to total FFAs was about 98%.Citation15) Measurement of the total FFAs concentration by an enzymatic method proved useful for estimating the ethyl caproate concentration in sake.Citation15) Therefore, we used the FFA assay method for the second screening: The isolated cerulenin-resistant mutants were inoculated into wells of a 96-well plate and incubated at 30 °C for 2–3 days. The FFA concentration of each culture in 96-well plate was examined using a NEFA C-test kit (Wako), and the mutants with high production of FFA were selected. As the third screening, to isolate the FAS2-G1250S mutant, a DNA fragment of 503 nucleotides containing the 3748th nucleotide of the FAS2 gene was amplified by PCR using 2 primers (P1 and P2 in Fig. (B)), cleaved with the restriction enzyme Bfa I, and analyzed by electrophoresis. The desired FAS2-G1250S mutation contains one base change from G (the 3748th nucleotide) to A, and this mutation generates one new recognition site by Bfa I. The FAS2-G1250S heterozygous mutant was detected by examining the electrophoresis pattern (Fig. (C), compare lanes 2 and 4). Further, the FAS2-G1250S mutation (change from 3748th G to A) was confirmed by DNA sequencing analysis of the amplified 503-bp (from the 3543rd to 4046th nucleotide) fragment for Bfa I-digestion.
Robustness analysis
Yeast cells at log phase (4.0–10.0 × 107 cells/mL) grown in YPD medium were fixed in a fixation solution (37% formaldehyde: 1 M potassium phosphate buffer, pH 6.5 = 1:1). The fixed cells were stained with fluorescein isothiocyanate-concanavalin A (FITC-ConA), rhodamine-phalloidin (Rh-ph), and 4′,6′-diamidino-2-phenylindole (DAPI) to visualize the cell wall, actin, and nucleus, respectively. The stained cells were transferred to a μclear cell culture microplate (Greiner 781091; 384 well, black, f-bottom, with lid), and images were captured by a high-throughput microscope (GE Healthcare IN Cell Analyzer 2000; Objective lens Nikon 100x). The images of yeast cells were processed by CalMorph (ver. 1.3) image processing software to obtain cell morphological parameters.Citation16,17) These parameters were subjected to morphological robustness analysis as described previously.Citation18,19) In this analysis, we performed 5 independent experiments.
Sake brewing test
To investigate the fermentation properties of the isolated yeast strain G9CR, we performed the sake brewing test using K1801 and the parental strain G9 as the control. First, we examined the production of ethyl caproate and fermentation properties of G9CR by performing a small-scale brewing test (total rice of 1 kg, 200 g of rice with 58% polishing ratio as koji, and 800 g of rice with 60% polishing ratio added directly to the sake mash). General properties (Sm, sake meter; Alc, alcohol; TA, total acidity; AA, amino acidity) and flavor components (iBuOH, isobutyl Alc; iAmOH, isoamyl Alc; iAmOAc, isoamyl acetate; EtOCap, ethyl caproate) of the sake were analyzed by the standard method established by the National Tax Agency of Japan. Then, we performed the sake brewing test on an industrial scale with the K1801, G9, and G9CR strains, using 600 kg of the polished sake rice Gohyakumangoku (130 kg of 50% polishing ratio as koji, and 470 kg of rice with 55% polishing ratio added directly to the sake mash). The sake mash during fermentation was periodically sampled and subjected to general analysis. The general properties and flavor components of the sake were analyzed by standard methods established by the National Tax Agency of Japan. Analysis of FFA was performed using the NEFA C-test kit according to the reported method.Citation15) The amount of medium-chain fatty acids, C6 and C8, was measured using gas chromatography, as previously reported.Citation20)
Results and discussion
Investigation of checkpoint integrity of cerulenin-resistant sake yeasts
As a relationship between a defect in fatty acid synthesis and an abnormality in nuclear division in fission yeast was reported earlier,Citation7) we examined the genetic stability of cerulenin-resistant mutants in budding yeast (sake yeast). In general, genetic stability (integrity of checkpoint mechanism) of yeast cells is evaluated by the sensitivity to drugs activating the checkpoint mechanism and the cell viability in the presence of the drug.Citation8–11) To investigate the genetic stability of sake yeast, we examined the sensitivity to the drugs benomyl and HU of the representative sake yeast strain K7,Citation12) the cerulenin-resistant K1801 with high productivity for ethyl caproate,Citation4) and the original strain G9 of Niigata Prefectural Sake Research Institute.Citation13)
Benomyl inhibits microtubule polymerization and activates the SAC,Citation10,11) whereas HU inhibits DNA replication and activates the DIC.Citation8,9) The BUB1 gene is essential for SAC function, and the deletion mutant (Δbub1), a SAC defective, showed sensitivity to benomyl but not to HU (Fig. ). On the other hand, the RAD53 gene is essential for both cell growth and the DIC, and the temperature-sensitive rad53 mutant showed sensitivity to HU but not to benomyl (Fig. ). To investigate the drug sensitivity of sake yeast, we used laboratorial Δbub1 and rad53 mutant cells as controls.
Fig. 1. Drug sensitivity of the yeast strains.
Notes: Drug sensitivity of laboratorial and industrial yeast strains. The laboratorial yeast strains, WT or Δbub1 (defective in SAC) and rad53 (defective in DIC), were used as the control strains, positive or negative, respectively. Drug sensitivities of a laboratorial yeast strain, Δrim15, and those of 4 industrial yeast strains, K7 (Brewing Society of Japan, Kyokai No.7), K1801 (Brewing Society of Japan, Kyokai No.1801), G9 (Niigata Prefectural Sake Research Institute), and the isolated G9CR, were examined on YPD plates containing cerulenin, benomyl, or HU at the indicated concentrations. The upper (WT, Δbub1, rad53, Δrim15, and K7) and lower panels (K1801, G9, and G9CR) are the left and right sides, respectively, on the same plate.
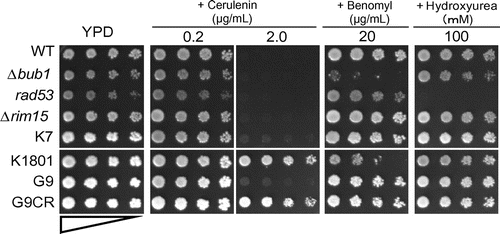
As shown in Fig. , the cerulenin-resistant strain K1801, but not K7 or G9, showed sensitivity to benomyl, as did the Δbub1 mutant. Further, K1801 was also sensitive to other inhibitors of microtubule polymerization, such as carbendazim and thiabendazole (data not shown). On the other hand, unlike the rad53 mutant, K1801, K7, and G9 sake yeasts showed no sensitivity to HU. These results suggest that K1801 was defective in SAC function but normal in DIC function.
To further investigate the checkpoint integrity of sake yeasts, we examined cell viability in the presence of benomyl or HU. Cells at early log phase were cultured in YPD liquid medium containing 15 μg/mL benomyl, and cell viability was examined. As a result (Fig. (A)), after incubation for 2 h, the viability of Δbub1 begun to decrease and dropped to 16.2% after 6 h of incubation. The viability of K1801, but not that of K7 and G9, showed similar behavior as the Δbub1 mutant and dropped to 12.2% after the 6-h incubation (Fig. (A)). On the other hand, in the presence of HU, the cell viability of the DIC-defective rad53 mutant, but not that of the 3 sake yeasts (K7, K1801, and G9), decreased significantly to 0.1% after the 6-h incubation (Fig. (B)). These results established that the cerulenin-resistant sake yeast K1801 strain had a defect in SAC function.
Fig. 2. Checkpoint integrity of the yeast strains.
Notes: (A, B) Cell viability of the indicated yeast strains in YPD liquid medium containing 15 μg/mL benomyl (A) or 200 mM HU (B) at the indicated times (h).
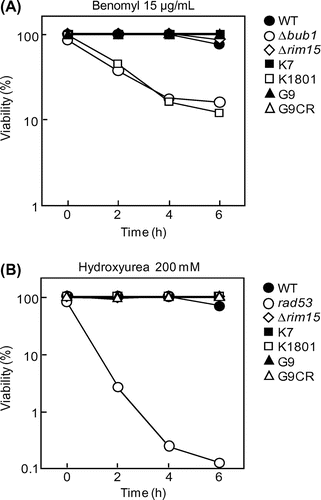
Previously, it was reported that sake yeast has a mutation in the RIM15 gene, a gene is important for stress responses, and this mutation causes sensitivity to various stresses.Citation21) To investigate whether the RIM15 gene was related to the checkpoint function, we evaluated the checkpoint integrity/intactness of the RIM15 deletion mutant (Δrim15) by examining its drug sensitivity and viability in the presence of the drugs activating checkpoint. As a result (Fig. ), Δrim15 cells showed no sensitivity to benomyl or HU. Further, the viability of Δrim15 cells was maintained normally in the presence of benomyl or HU (Fig. ). These results indicated that the RIM15 gene was not related to the checkpoint function.
Isolation of spontaneous cerulenin-resistant mutant with normal checkpoint integrity
Currently, treatment with a mutagen such as EMS has been used widely for breeding of industrial yeast strains. However, this method has a risk for inducing a mutation in a number of genes other than the target one. To avoid this risk, we sought to isolate a spontaneous cerulenin-resistant mutant with both high productivity for ethyl caproate and integrity/intactness of the checkpoint mechanisms from the sake yeast G9, which is the original strain of Niigata Prefecture,Citation13) and showed intact checkpoint integrity (Figs. and ). Further, in Niigata Prefecture, a spontaneous cerulenin-resistant sake yeast producing a high level of ethyl caproate had not been developed yet.
At the first screening (Fig. (A)), we selected cerulenin-resistant mutants from G9 using the YNB solid medium (2% glucose) containing 0.4 μg/mL cerulenin and obtained 3056 cerulenin-resistant mutants. At the second screening (Fig. (A)), we measured the content of FFA in the culture medium using the previously reported FFA assay methodCitation15) and thereby obtained 14 mutants with high production of FFA. At the third screening (Fig. (A) and (B)), to identify the mutation site of FAS2-G1250S, we amplified a 503-bp DNA fragment of the FAS2 gene by PCR, cleaved the amplified DNA fragment with the restriction enzyme Bfa I, and analyzed the products by electrophoresis. The desired FAS2-G1250S mutation contains one nucleotide change from G (the 3748th nucleotide) to A, and this mutation generates a new Bfa I recognition site (Fig. (B)). The FAS2-G1250S heterozygous mutant was detected based on its electrophoresis pattern (Fig. (C), compare lanes 2 and 4). Finally, we isolated 1 cerulenin-resistant FAS2-G1250S heterozygous mutant and named this mutant G9CR (G9 cerulenin-resistant).
Fig. 3. Screening scheme for the spontaneous FAS2-G1250S yeast mutant with high productivity of ethyl caproate.
Notes: (A) Isolation of the FAS2-G1250S mutant with high productivity of ethyl caproate. At the first screening, cerulenin-resistant mutants were selected. At the second screening, the free fatty acid (FFA) high-producing mutants were selected by performing the FFA assay. At the third screening, the FAS2 mutation site in the selected mutants was examined by digestion with the restrictive enzyme Bfa I and DNA sequencing. (B) Confirmation of the FAS2 mutation site. The FAS2 gene in the mutant was amplified by PCR using 2 primers, P1 and P2. The amplified 503-bp fragment was digested by Bfa I. Putative cleavage DNA fragments of WT FAS2 gene and mutated FAS2-G1250S gene are 2 bands (430 and 73 bp) and 3 bands (205, 225, and 73 bp), respectively. (C) Cleavage pattern of the DNA sample after Bfa I-mediated digestion of the PCR-amplified fragment. Bfa I-digested (+) and non-digested DNA fragments (−) were loaded onto an agarose gel. M indicates the Gene Ladder Wide 1 (NIPPON GENE), which used as a size marker.
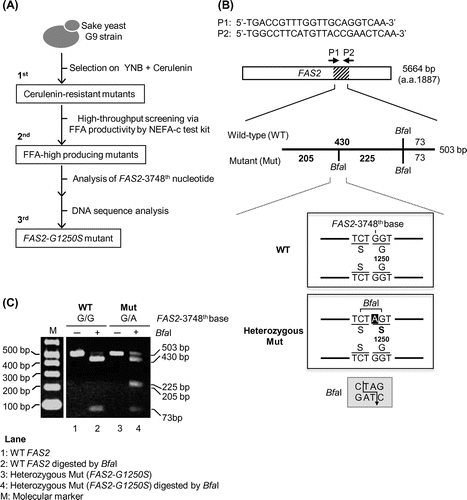
Checkpoint integrity of the spontaneous cerulenin-resistant G9CR
To investigate the genetic stability of G9CR, we examined its sensitivity to the drugs activating the checkpoints and viability in the presence of the drugs. The isolated G9CR strain was not sensitive to benomyl and HU, as in the case of the K7 and parental strain G9 (Fig. ); the viability of G9CR was maintained in the presence of either benomyl or HU (Fig. ). These results indicated that the spontaneously isolated G9CR strain had normal integrity of both DIC and SAC checkpoints.
The question arose as to the cause of the SAC-defective phenotype (sensitivity and viability) when K1801 was treated with benomyl. Two cerulenin-resistant strains (G9CR and K1801) having the FAS2-G1250S mutation (K1801, confirmed by Bfa I-digestion of PCR product) showed distinct phenotypes (sensitivity and viability) when exposed to benomyl, indicating that the phenotypic difference between these 2 strains was not caused by the cerulenin-resistant FAS2-G1250S mutation. A defect in SAC integrity of K1801 was not related to the cerulenin-resistant FAS2-G1250S mutation. Further analysis is necessary to clarify this question.
As mentioned above, in fission yeast, a defect in fatty acid synthesis causes an abnormality in nuclear division.Citation7) However, our result indicates that in budding yeast, the FAS2-G1250S mutation does not affect the checkpoint integrity in both DIC and SAC. The symmetrical cell division in fission yeast might require the intactness of fatty acid synthesis. Further analysis will need to clarify the relationship between nuclear division and fatty acid synthesis in budding yeast.
Morphological phenotypic robustness analysis of spontaneous cerulenin-resistant G9CR
Phenotypic robustness is defined as the ability of the system to continue to function despite disturbances. Phenotypic robustness in yeast morphology has been analyzed by image processing program, CalMorphCitation16,17) which enables us to obtain high-dimensional and quantitative data of cell, actin, and nuclear DNA morphology at single-cell resolution. Phenotypic robustness of 4718 nonessential gene deletion mutants was investigated, revealing that more than 300 genes contribute to the phenotypic robustness.Citation18) Strikingly, approximately one-fourth of identified genes are annotated to be involved in maintaining the chromosome stability and DNA integrity, suggesting the linkage between the phenotypic robustness and genetic stability.
To analyze phenotypic robustness of G9CR and K1801, we quantified their phenotypic variance of the morphology of cell, actin cytoskeleton, and nuclear DNA at single-cell resolution (Fig. (A)). We observed that G9CR exhibited increased phenotypic robustness, that is, more homogeneous morphology in its cell population; in other words, K1801 cells showed more heterogeneous morphology such as cell size and cell roundness (Fig. (A)). This was supported by the fact that G9CR significantly showed a lower score in phenotypic potential (p < 0.05 from t-test, Fig. (B)) which was the indicator of overall phenotypic variation in cell population.Citation18,19) We found that more than half of the morphological traits (142/220 parameters, 65%) of G9CR showed smaller variation. For example, means of the parameter C117_A1B (ratio of outline length of bud to mother cell in S/G2 cells) were comparable in G9CR and K1801 (Fig. (C), left), but noise scores (normalized variation) of G9CR were significantly smaller than those of K1801 (Fig. (C), right). These results indicated that G9CR was more robust in morphology than K1801. The reason for the increased phenotypic robustness in G9CR was unknown, but one of the possibilities was due to the intact checkpoint integrity of G9CR.
Fig. 4. Morphological phenotypic robustness of K1801 and G9CR.
Notes: (A) Morphology of K1801 and G9CR. The cells was examined after having been stained with FITC-ConA (green), Rh-ph (red), or DAPI (blue) to visualize the cell wall, actin, and nucleus, respectively. (B) Phenotypic potential of sake yeast was scored from each experiment data (n = 5). Asterisk indicates a significant difference (p < 0.05 by Student’s t-test) between the bracketed values. (C) Mean and noise score (normalized variance, [Citation19]) of the morphological parameter C117_A1B (cell size ratio) were obtained from K1801 and G9CR morphometric data.
![Fig. 4. Morphological phenotypic robustness of K1801 and G9CR.Notes: (A) Morphology of K1801 and G9CR. The cells was examined after having been stained with FITC-ConA (green), Rh-ph (red), or DAPI (blue) to visualize the cell wall, actin, and nucleus, respectively. (B) Phenotypic potential of sake yeast was scored from each experiment data (n = 5). Asterisk indicates a significant difference (p < 0.05 by Student’s t-test) between the bracketed values. (C) Mean and noise score (normalized variance, [Citation19]) of the morphological parameter C117_A1B (cell size ratio) were obtained from K1801 and G9CR morphometric data.](/cms/asset/26d1f6d6-440e-44d1-81c8-f42ac1d259e4/tbbb_a_1020756_f0004_oc.gif)
Our result together with previous study raised the possibility that monitoring of yeast morphology can be used for quality control management of sake yeasts as was applied to Saccharomyces pastorianus during beer fermentation.Citation22) Sake yeasts themselves showed a higher bud index and a smaller mother cell size than the laboratory strains,Citation23) providing their common morphological properties. In light of high correlation of phenotypic robustness and chromosome/DNA integrity,Citation18) the morphological homogeneity is a preferable trait for risk-free sake yeast. Since intraspecies robustness of S. cerevisiae morphology is highly variable in nature,Citation19) breeding yeasts with homogeneous morphology would be a practical and immediate aim for domestication.
Confirmation of high producing ability of ethyl caproate by spontaneous cerulenin-resistant G9CR by industrial sake brewing
To investigate the fermentation properties of G9CR, we performed a sake brewing test on a small scale (1 kg of total rice) and an industrial one (600 kg of total rice), and compared its fermentation properties with those of the parental strain G9 and K1801.
In the small-scale sake brewing test, the fermentation profile of G9CR was similar to that of parental strain G9 (data not shown). The amount of ethyl caproate produced by G9CR was 3.5 times that from G9 and about 80% of that from K1801 (Table ). In terms of sensory evaluation, sake fermented by G9CR received a high sensory score (Table ).
Table 1. Properties of sake made by G9, G9CR, and K1801 in small-scale sake brewing (1 kg of total rice).
Further, in the sake brewing test conducted on an industrial scale (600 kg of total rice), G9CR showed a fermentation profile similar to that of G9 (Alc and Sm in Fig. (A)). The amount of ethyl caproate produced by G9CR was 2.5 times that from G9 and about a half of that from K1801 (Fig. (B)). In the sensory evaluation, sake fermented by G9CR received the highest sensory score among sakes from the 3 strains (Table ). These results indicate that G9CR had fermentation ability similar to that of the parental strain G9 and higher ethyl caproate-producing ability than G9.
Fig. 5. Sake brewing test using K1801, G9, and G9CR.
Notes: (A) Fermentation profiles (upper, Alc; lower, Sm) in the sake mash (Shubo/pre-culture and Moromi/main culture) produced with K1801 (closed circle), G9 (closed triangle), or G9CR (open triangle). The values were measured by standard methods established by the National Tax Agency of Japan. (B) Contents of ethyl caproate (upper panel) and free fatty acids (lower panel) in the sake mash (Shubo/pre-culture and Moromi/main culture) produced with K1801, G9, or G9CR. Sake brewing test on an industrial scale was performed using 600 kg of the polished sake rice (Gohyakumangoku, polishing ratios of 50% for koji and 55% for rice added directly to the sake mash).
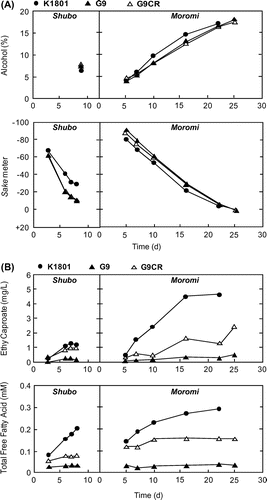
Table 2. Properties of sake made by G9CR in industrial-scale sake brewing (600 kg of total rice).
In conclusion, we investigated the checkpoint integrity of sake yeast, and the results suggest that a currently used sake yeast K1801 with high productivity for ethyl caproate has a defect in SAC function, although a cause of the SAC-defective phenotype of K1801 remains elusive. We also isolated the spontaneous cerulenin-resistant sake yeast G9CR having high producing ability of ethyl caproate and confirmed the intactness of checkpoint functions of the strain. The genetic stability of sake yeast is thought to be important for the maintenance of both the fermentation properties of sake yeast and quality of the final product, sake. However, it should be noted that instability nature of the fermentation properties of K1801 on industrial scale has not been detected (communication from the Brewing Society of Japan). Although it is necessary to clarify the relationship between the checkpoint integrity of sake yeast and the quality of sake, the investigation of checkpoint integrity/intactness of yeast will be an important screening step for breeding of risk-free industrial yeasts.
Acknowledgments
We thank T. Kuribayashi (Niigata Prefectural Sake Research Institute; NPSRI) for support of the experiments (checkpoint investigation, G9CR isolation, and sake brewing test); all members of NPSRI for sensory evaluation of sake; and the Brewing Society of Japan (T. Ishikawa, N. Okazaki, and M. Inahashi), NPSRI, and Niigata Sake Brewers Association (S. Ohdaira and S. Yamashita) for helpful discussion, advice, and communication. We also thank all members of Asahi Sake Brewing Co. Ltd. for their support.
References
- Stoops JK, Wakil SJ. The yeast fatty acid synthetase. J. Biol. Chem. 1981;256:8364–8370.
- Ichikawa E, Hosokawa N, Hata Y, Abe Y, Suginami K, Imayasu S. Breeding of sake yeast with improved ethyl caproate productivity. Agric. Biol. Chem. 1991;55:2153–2154.10.1271/bbb1961.55.2153
- Aritomo K, Hirosawa I, Hoshida H, Shiigi M, Nishizawa Y, Kashiwagi S, Akada R. Self-cloning yeast strains containing novel FAS2 mutations produce a higher amount of ethyl caproate in Japanese sake. Biosci. Biotechnol. Biochem. 2004;68:206–214.10.1271/bbb.68.206
- Yoshida K. Kyokai sake yeast 1801. Nippon Jozo Kyokaishi. 2006;101:910–922. Japanese.
- Boddy MN, Russell P. DNA replication checkpoint. Curr. Biol. 2001;11:R953–R956.10.1016/S0960-9822(01)00572-3
- Musacchino A, Salmon ED. The spindle-assembly checkpoint in space and time. Nat. Rev. Mol. Cell. Biol. 2007;8:379–393.10.1038/nrm2163
- Saitoh S, Takahashi K, Nabeshima K, Yamashita Y, Nakaseko Y, Hirata A, Yanagida M. Aberrant mitosis in fission yeast mutants defective in fatty acid synthetase and acetyl CoA carboxylase. J. Cell. Biol. 1996;134:949–961.10.1083/jcb.134.4.949
- Weinert TA, Kiser GL, Hartwell LH. Mitotic checkpoint genes in budding yeast and the dependence of mitosis on DNA replication and repair. Genes Dev. 1994;8:652–665.10.1101/gad.8.6.652
- Allen JB, Zhou Z, Side W, Friedberg EC, Elledge SJ. The SAD1/RAD53 protein kinase controls multiple checkpoints and DNA damage-induced transcription in yeast. Genes Dev. 1994;8:2401–2415.10.1101/gad.8.20.2401
- Hoyt MA, Totis L, Roberts BT. S. cerevisiae genes required for cell cycle arrest in response to loss of microtubule function. Cell. 1991;66:507–517.10.1016/0092-8674(81)90014-3
- Li R, Murray AW. Feedback control of mitosis in budding yeast. Cell. 1991;66:519–531.10.1016/0092-8674(81)90015-5
- Akao T, Yashiro I, Hosoyama A, Kitagaki H, Horikawa H, Watanabe D, Akada R, Ando Y, Harashima S, Inoue T, Inoue Y, Kajiwara S, Kitamoto K, Kitamoto N, Kobayashi O, Kuhara S, Masubuchi T, Mizoguchi H, Nakao Y, Nakazato A, Namise M, Oba T, Ogata T, Ohta A, Sato M, Shibasaki S, Takatsume Y, Tanimoto S, Tsuboi H, Nishimura A, Yoda K, Ishikawa T, Iwashita K, Fujita N, Shimoi H. Whole-genome sequencing of sake yeast S. cerevisiae Kyokai no. 7. DNA Res. 2011;18:423–434.10.1093/dnares/dsr029
- Sato K, Kaneoke M, Aoki T, Nabekura Y, Watanabe K. A new medium for distinguishing between sake yeast and wild yeast. Nippon Jozo Kyokaishi. 2005;100:209–213. Japanese.
- Omura S. The antibiotic cerulenin, a novel tool for biochemistry as an inhibitor of fatty acid synthesis. Bacteriol. Rev. 1976;40:681–697.
- Kuribayashi T, Kaneoke M, Hirata D, Watanabe K. Analysis of free fatty acids in sake by an enzymatic method and its application for estimating ethyl caproate and selecting yeast with high productivity of the ester. Biosci. Biotechnol. Biochem. 2012;76:391–394.10.1271/bbb.110698
- Ohtani M, Saka A, Sano F, Ohya Y, Morishita S. Development of image processing program for yeast cell morphology. J. Bioinform. Comput. Biol. 2004;1:695–709.10.1142/S0219720004000363
- Ohya Y, Sese J, Yukawa M, Sano F, Nakatani Y, Saito TL, Saka A, Fukuda T, Ishihara S, Oka S, Suzuki G, Watanabe M, Hirata A, Ohtani M, Sawai H, Fraysse N, Latgé JP, François JM, Aebi M, Tanaka S, Muramatsu S, Araki H, Sonoike K, Nogami S, Morishita S. High-dimensional and large-scale phenotyping of yeast mutants. Proc. Natl. Acad. Sci. USA. 2005;102:19015–19020.10.1073/pnas.0509436102
- Levy SF, Siegal ML. Network hubs buffer environmental variation in S. cerevisiae. PLoS Biol. 2008;6:2588–2604.
- Yvert G, Ohnuki S, Nogami S, Imanaga Y, Fehrmann S, Schacherer J, Ohya Y. Single-cell phenomics reveals intra-species variation of phenotypic noise in yeast. BMC Syst. Biol. 2013;7:54.10.1186/1752-0509-7-54
- de Jong C, Badings HT. Determination of free fatty acids in milk and cheese: procedures for extraction, clean up, and capillary gas chromatographic analysis. J. High Resolut. Chromatogr. 1990;13:94–98.10.1002/(ISSN)1521-4168
- Watanabe D, Araki Y, Zhou Y, Maeya N, Akao T, Shimoi H. A loss-of-function mutation in the PAS kinase Rim15p is related to defective quiescence entry and high fermentation rate of Saccharomyces cervisiae sake yeast strains. Appl. Environ. Microbiol. 2012;78:4008–4016.10.1128/AEM.00165-12
- Ohnuki S, Enomoto K, Yoshimoto H, Ohya Y. Dynamic changes in brewing yeast cells in culture revealed by statistical analyses of yeast morphological data. J. Biosci. Bioeng. 2014;117:278–284.10.1016/j.jbiosc.2013.08.005
- Watanabe D, Nogami S, Ohya Y, Kanno Y, Zhou Y, Akao T, Shimoi H. Ethanol fermentation driven by elevated expression of the G1 cyclin gene CLN3 in sake yeast. J. Biosci. Bioeng. 2011;112:577–582.10.1016/j.jbiosc.2011.08.010