Abstract
Suspension culture of Ajuga lobata D. Don cells provides a method of synthesis of the phytoecdysteroid 20-hydroxyecdysone (20E) which can regulate the molting process of larvae. We characterized the culture conditions to optimize 20E production. Growth of A. lobata D. Don cells fits the logistic equation curve with a growth cycle of 19 days. Medium conductivity was negatively correlated with dry cell weight and 20E accumulation, thus could be used to determine the optimal time for cell harvest. Continuous subculture reduced 20E synthesis, but supplementing medium with 20E precursors mevalonic (MVA), α-Pinene, and nitric oxide (NO) can significantly promote cell growth and influence 20E accumulation. Combination of α-Pinene, MVA, and SNP significantly elevated 20E accumulation, thus may synergistically enhance 20E synthesis in A. lobata D. Don. The optimal concentrations of α-Pinene, MVA, and NO donor SNP in suspension culture were 50 μL L−1, 10 mg L−1, and 80 μmol L−1.
Graphical abstract
Continuous culture of A. lobata D. Don will reduce the β-EC harvest. Supplemented with MVA, α-Pinene, and SNP to improve the content for saving cost.
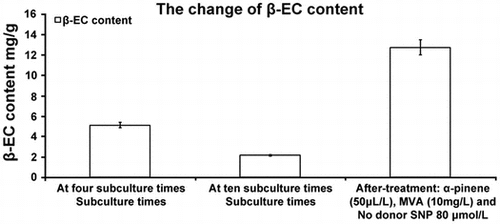
Ecdysterone is a naturally occurring steroid hormone secreted by arthropods, including insects, to regulate the molting process of larvae. Ecdysterone also has many pharmacological functions including promoting cell growth and inducing human epidermal cell differentiation.Citation1) Some plants produce phytoecdysteroids which share structural similarity with insect-derived ecdysterone,Citation2) thus can influence insect development, metamorphosis, reproduction, and embryogenesis.Citation3) There are many more pharmacological effects of phytoecdysteroids on mammals even humans,Citation4,5) they are apparently non-toxic to mammals and a wide range of beneficial pharmacological effects, such as adaptogenic, anabolic,Citation6) anti-diabetic,Citation7) hepatoprotective,Citation8,9) wound-healing,Citation10) and perhaps even anti-tumor.Citation6,11) These plant steroids can be applied for the purpose of pest control.Citation12,13) Phytoecdysteroids can be obtained from a wide variety of plant sources, and the concentration of phytoecdysteroids in plants is higher than the concentration of zooecdysteroids in insects.Citation14–19) The most prevalent phytoecdysteroids in Ajuga lobata D. Don is 20-hydroxyecdysone (20E), which can accumulate to concentrations as high as 4.57 mg g−1, highlighting the value of this plant as a source for phytoecdysteroids.Citation20)
A. lobata D. Don (Labiatae, Ajuga) is a perennial herb widely distributed in southern China.Citation21–23) However, artificial cultivation of A. lobata D. Don requires a long growth cycle, and the yield of 20E isolation from wild A. lobata D. Don is low. As an alternative, plant tissue and cell culture requires shorter growth periods and is not be influenced by environmental or seasonal factors. Since 20E is concentrated in the root of A. lobata D. Don, cell engineering techniques have been applied to A. lobata D. Don culture, and methods for simple, fast, and convenient 20E extraction have been developed.Citation20) A. lobata D. Don cell culture not only provides adequate raw materials for 20E production, but also lowers production costs and limits the impact on wild A. lobata D. Don.
In 1987, Klaus Richter et al.’s study contacted toxicity of different solvent extracts from Ajuga reptans against Periplaneta americana.Citation24) Then, Lev. et al. (1990) studied the possibility of obtaining ecdysteroids—ecdysterone and turkesterone—with the aid of a culture of the tissues and cells of the plant Ajuga turkestanica.Citation25) In the post-studies, the researchers focused on the 20E production associated with the hairy roots (especially induced hairy roots) or callus cultures.Citation26–29) And some studies demonstrated that this plant tissue culture is an excellent experimental system for the study of “ecdysteroid biosynthesis”Citation30, 31) and there are extensive research studies about the activities of some related enzymes.Citation32) Recently, there are some articles about the effect of secondary metabolites on cell growth and the ecdysteroid biosynthesis.Citation14,33)
A. lobata D. Don was transplanted by the Blue Sky Garden Company in 2009. Effective propagation of A. lobata D. Don was established by the Insect Laboratory of the Department of Forest Bioengineering, and in 2013, we established a preliminary suspension culture system for A. lobata D. Don.Citation20) The suspension culture system conditions have since been adapted to optimize the production of secondary metabolite 20E, and facilitate efficient 20E extraction. On this basis, we analyzed the relationship between nutrient consumption, electric conductivity, cell growth, and biomass accumulation, and aimed to design a culture system optimized to produce 20E.
The biosynthesis of 20E is closely associated with terpenoid biosynthesis; these compounds are synthesized by the isoprenoid biosynthesis pathway, consisting of the mevalonate (MVA) pathway and the 5-phosphate-D-deoxyxylulose/2-C-methy–D-erythritol-4-phosphate (DOXP/MEP) pathway.Citation34–37) The MVA pathway is a major plant synthetic pathway that begins with acetyl CoA condensation, reduction to mevalonate, and results in production of steroid ketones and terpenes. In contrast, the DOXP/MEP pathway utilizes pyruvic acid and GA-3P as the starting substrates to produce terpenes. Although isopentenyl pyrophosphate (IPP) and its isomer dimethylallyl pyrophosphate (DMAPP) are intermediate products of both pathways, the mechanisms by which DMAPP and IPP are synthesized differ, as does the intracellular location of metabolic end products.
A small molecule monocyclic monoterpene product of the MVA and MEP pathways, α-Pinene, can inhibit terpene synthesis, altering the synthesis pathway to increase the yield of steroid ketones. Terpineol, a product of α-Pinene metabolism, is also a monocyclic terpene alcohol that exerts an inhibitory function on terpene biosynthesis.Citation38) We sought to investigate the influence of molecules that interfere with these pathways on the production of 20E. As a key substrate of the MVA pathway, MVA could be considered to be a precursor for sterone synthesis, and the essential amino acid L-phenylalanine (L-Phe) is recognized to be a universal precursor for various secondary metabolic pathways.Citation39) As nitric oxide (NO) has been used as an elicitor of plant secondary metabolism widely,Citation40) thus, we added these substrates into the culture media of the A. lobata D. Don suspension culture system and evaluated their influence on the secondary metabolite 20E production.
Materials and methods
Basic culture
The roots of A. lobata D. Don were taken as the explants for callus induction (MS + 6-BA 1 mg L−1 + 2,4-D 0.4 mg L−1). The callus tissues were taken for continuous cultivation (MS + 2,4-D 0.4 mg L−1) after 15 days. Then, at passage 2, the cells were harvested for the suspension culture.Citation20) The basic liquid medium used in this study consisted of MS media, 0.4 mg L−1 of 2, 4-D, and 0.5 mg L−1 of 6-BA. The pH value was adjusted to 5.8, the sucrose concentration was 2%, and the inoculation ratio was 10% (5 g cells in 50 mL medium). Cells were cultured under a 16/8-h light/dark cycle with 2000-lx light intensity at 25 °C and 70% humidity. Culture flasks were shaken at 120–130 rpm.
Measurement was obtained for four individual experiments, each with 10 replicates.
Kinetics of suspension cell culture
Cells at passage 4 were inoculated. After 24 h (1 day), then at day 3, 5, 7, 9, 11, 13, 15, 17, 19 post inoculation, three flasks were taken, and the cell fresh weight (FW), dry weight (DW), and 20E content was recorded. The culture medium pH, electric conductivity, soluble sugar, phosphate, nitrate, and ammonium salt were measured.
Measurement of cell weight and establishment of kinetic model
The cell suspension was passed through a 300-mesh sieve and dried with filter paper, and the FW was recorded. Then, cells were dried at 60 °C to measure the DW.
Cell growth kinetics were calculated according to the following formula
wherein, represents the highest cell concentration (
dt represents the parameter obtained from data fitting; K represents the scaling factor.
Measurement of culture medium pH
The culture medium pH was measured using pH S-25 pH meter (Shanghai Leici Instrument Factory).
Measurement of culture medium electric conductivity
The culture medium conductivity was measured using a BEC microprocessor-based conductivity meter (BEC-11AW, Bell Analysis Instrument Co., Ltd.).
Measurement of nutrient consumption and sugar consumption
The molybdenum blue method was used to measure culture medium phosphate content; salicylic acid spectrophotometry was used to determine nitrate content; the ninhydrin assay was used to measure ammonium salt content; and anthrone colorimetry was used to determine the total content of soluble sugar.Citation41)
The kinetics of sugar consumption were calculated according to the following formula
wherein, represents theoretical yield; m represents cell maintenance factor;
represents macro-yield;
represents initial substrate concentration (mg L−1 DW; cs represents substrate concentration (mg L−1); t represents the culture time (d).
Measurement of cell suspension 20E content
Dried A. lobata D. Don cells (DW 0.2 g) were soaked in 5 mL methanol for 24 h, treated with ultrasound(YH-200DH, produced by YUHAO in China) for 1 h at 40 kHz, and digested with microwave (WT-8000 microwave digestion system, conditions: T = 50 °C, p = 2 P0, T = 10 min, W = 300 × 2). The resultant suspension was filtered through an organic membrane, and the 20E content of the filtrate was assessed by HPLC. A MS C18 column (4.6 × 250 mm, 5 μm particle size) (American, Waters Company) and a UV–vis detector (detection range: 190–800 nm) were used to detect 20E at 242 nm wavelength. The mobile phase (methanol: water = 50: 50) had a flow rate of 0.8 mL min−1, and the sample loading volume was 20 μL.
20E standards with a concentration of 0, 0.4, 0.32, 0.24, 0.16, and 0.08 mg mL-1 were prepared and loaded at a volume of 20 μL. Detection of each standard was repeated 3 times. Using the average peak area as the vertical axis and the concentration as abscissa, the regression equation of 20E was obtained (y = 2 × 10−8 × −0.0029, R2 = 0.9986). The 20E content of each sample was calculated according to the standard curve. The final concentration of 20E = the value (we obtained from HLPC) × 0.2 g (DW)/5 mL (volume of the liquid). The kinetic model of 20E production fits the reference logistic equation: , let
then we obtain the formula:
.
wherein, Pmax represents the maximum product concentration (mg g−1); P0 represents the initial product concentration (mg g−1); Pp represents product concentration (mg g−1); k represents the coefficient of product formation; and t represents the culture time (d).
Measuring influence of passaging on 20E content
20E content of A. lobata D. Don cells was measured at initial inoculation (passage 1), then immediately prior to each passage, which was carried out by inoculating 5 g of cells into 50 mL of liquid medium every seven days for fifteen passages.
Measuring influence of exogenous substrates on cell growth and 20E content
Influence of exogenous precursors. To assess the influence of L-Phe, MVA, α-Pinene, or terpineol on cell growth and 20E content, A. lobata D. Don cells at passage 10 were inoculated (5 g) into 50 mL of liquid medium supplemented with the indicated supplements either along or in combination, and cultured for seven days. Cell growth and 20E content were measured. During measurement, each condition had 4 repeats in the same experiment.
L-Phe (0.1652 g) was diluted in sterilized ddH2O (10 mL) to a concentration of 0.1 mmol mL−1, which was added to culture medium to yield a final concentration of 0, 0.1, 0.2, or 0.4 mmol L−1. MVA was diluted in KOH (0.1 N) to a concentration of 50 g L−1, which was added to culture medium to yield a final concentration of 0, 5, 10, 20, or 80 mg L−1. α-Pinene was diluted in 100% ethanol (α-Pinene: ethanol = 8: 2 in volume), which was added to culture medium to yield a final concentration of 0, 25, 50, or 100 μL L−1. Terpineol (8.4 mL) was diluted in 1.6 mL of 100% ethanol which was added to culture medium to yield a final concentration of 0, 100, or 400 μL L−1. After seven days, cell growth, activity, and 20E content were measured.
To assess the influence of NO on cell growth and 20E content, A. lobata D. Don cells at passage 10 were inoculated (5 g) into 50 mL of liquid medium and cultured for six days. Sodium nitroprusside (SNP, 50 mmol L−1, 0.0298 g SNP in 2 mL ddH2O) was added to the culture media to yield a final concentration of 0, 10, 20, 40, 80, or 160 μmol L−1 (0.5 mmol L−1 of SNP can release 2.0 mmol L−1 of NO).Citation42,43) After 24-h cell growth, activity and 20E content were measured.
Cell activity was assessed using the tetrazolium chloride (TTC) assay. Fresh cells (0.2 g) were incubated with 0.4% TTC (2.5 mL) and PBS (2.5 mL, pH 7) in the dark for 14 h. Then, the suspension was centrifuged, the supernatant discarded, and cells were washed with ddH2O three times. 95% ethanol (5 mL) was added to the washed pellet and cells were decolorized in a 60 °C water bath for 30 min (flask shaken for every 5 min). Absorbance at 485 nm was measured using a spectrophotometer.
Statistical analysis
Data were analyzed by Microsoft Excel 2007. Kinetic fitting was performed using the software Origin 8.6. ANOVA was performed using the software DPSv3.1.
Results
Kinetics of A. lobata D. Don cell growth
Growth curve of A. lobata D Don cells
The growth curve suggested that cell growth fitted the logistic equation. The growth cycle of A. lobata D. Don cells lasted 19 days, and the growth curve was S-shaped. In the lag phase (days one to three), cell growth and biomass accumulation were slow. The logarithmic growth phase occurred between day three and 11, and after day 11, cell growth gradually slowed, and cells entered the stationary phase where biomass accumulation (FW) peaked at 34.74 ± 0.140 g /flask. The stationary phase lasted until day 17, after which cells entered the decline phase and biomass accumulation decreased.
Cell culture pH variation during A. lobata D. Don cell culture
The medium pH varied throughout the growth cycle, declining immediately after passage, then gradually increasing within the range of pH 4.5–6.0. At day 19, the pH had fallen to 5.8 ± 0.34.
Cell culture electric conductivity, cell DW, and 20E content
During A. lobata D. Don culture, the conductivity of the culture suspension decreased while cell DW increased (Fig. ). At day nine, cell DW peaked at 0.63 ± 0.021 g and the corresponding electric conductivity was 2.78 ± 0.318 mS cm−1. After cells entered the stationary phase, the electric conductivity decreased to less than 2 ± 0.411 mS cm−1. The change in DW and cell growth was fitted to the equation: (Fig. ).
Fig. 1. Cell dry weight in A. lobata D. Don culture (Spots indicate the cell dry weight at different culture times, curve is cell dry weight kinetics).
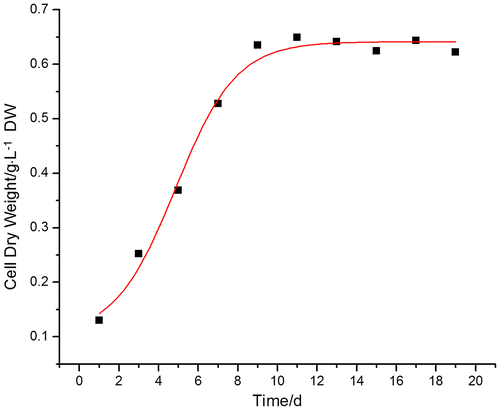
Parameters obtained from data fitting were:
cx0 = 0.10285, t0 = 4.84955 d, dt = 1.53538 and the correlation coefficient of dt = 0.98296. This indicated the model satisfactorily reflected the kinetics of A. lobata D. Don cell growth during suspension culture.
The 20E content was negatively correlated with the medium electric conductivity (Fig. ). When the electric conductivity reached to 2.78 ± 0.318 mS cm−1, the 20E content peaked at 0.246 ± 0.0113 mS cm−1. In the stationary phase, the electric conductivity continued to decrease until the decline phase, potentially as a result of outflow of intracellular ions after cell membrane rapture.
Fig. 2. Dynamic changes in cell suspension electric conductivity and 20E content.
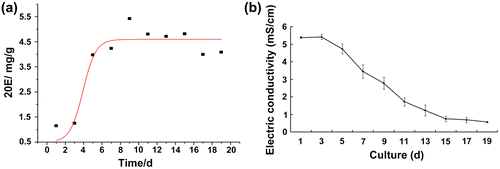
Parameters describing the accumulation of 20E in suspension culture obtained from data fitting were the correlation coefficient R2 = 0.87492, Pmax = 0.20449, and k = 1.42409.
Substrate consumption during A. lobata D. Don suspension culture
Comparison of the cell growth curve and substrate consumption curve revealed that these two indicators were significantly associated, as the culture sugar, phosphate, nitrate, and ammonium salt content gradually decreased with cell growth, and phases of culture substrate use corresponded to the lag, logarithmic, and stationary phases of cell growth. However, at day 19, 11.96% of medium nitrates (Fig. (c)) remained unabsorbed, while 7.59% of medium ammonium salts were unabsorbed (Fig. (d)). Consumption of total sugar fitted the kinetic model of sugar consumption, and the = 7.931, m = 0.0958 (Fig. (a)). The correlation coefficient of fitting, R2 = 0. 99747, indicated that the curve could satisfactorily reflect the rate of sugar consumption during suspension culture.
Influence of cell passaging on 20E content
The 20E content of A. lobata D. Don increased with the first three subcultures, peaking at 5.11 ± 0.256 mg g−1 at passage four. However, from passage five to passage 15, 20E content declined (p < 0.05) (Fig. ). At passage 10, the 20E content was lower than at passage 2. At passage 15, the 20E content was 23.5% of that at passage 2, and 14.4% of that at passage 4.
Influence of exogenous substrates on A. lobata D. Don activity and 20E content
In order to measure the capacity of exogenous culture supplements to influence A. lobata D. Don culture, we added the indicated supplements to passage 10 A. lobata D. Don. At this passage, 20E production was observed to be less than in younger cells, and thus the influence of exogenous supplements on 20E accumulation could be clearly measured.
Influence of L-Phe on A. lobata D Don cell activity and 20E accumulation
Supplementation of passage 10 A. lobata D. Don cell culture with 0.1 to 0.4 mmol L−1 L-Phe significantly increased the cell activity (p < 0.01) (Table ). However, L-Phe did not significantly impact 20E content. Considering the influence of subculture on cell activity, 0.2 mmol L−1 L-Phe could be used as an activator of A. lobata D. Don cells at passage 10 of suspension culture.
Table 1. Effect of L-PAL on A. lobata D. Don cell suspension culture.
Influence of MVA on A. lobata D Don cell activity and 20E accumulation
Supplementation of A. lobata D. Don cell culture with 10 to 20 mg L−1MVA increased cell activity (p < 0.01) (Table ), and 5 or 10 mg L−1MVA stimulated synthesis of 20E, enhancing culture content of 20E by127% and 394.8%, respectively (p < 0.05 and p < 0.01, respectively). However, 5–80 mg L−1MVA inhibited cell growth in a dose-dependent manner. Cells incubated with 5 mg L−1 MVA exhibited ordinary growth and the medium was gray-colored, but cells incubated with 20 mg L−1 MVA exhibited browning and death, and 20E accumulation was significantly decreased.
Table 2. Effect of MVA on A. lobata D. Don cell suspension culture.
Influence of α-Pinene on A. lobata D. Don cell activity and 20E accumulation
Supplementation of A. lobata D. Don cell culture with 25–100 μL L−1 α-Pinene reduced the growth of A. lobata D. Don cells (Table ), but 50–100 μL L−1 α-Pinene enhanced cell activity (p < 0.01). Also, the accumulation of 20E was elevated in the presence of 25–100 μL L−1 α-Pinene (p < 0.01). 20E accumulation peaked at 3.50 ± 0.006 mg g−1, 60% higher than control levels in the presence of 50 μL L−1 α-Pinene (p < 0.01).
Table 3. Effect of α-Pinene on A. lobata cell suspension culture.
Influence of terpineol on A. lobata D. Don cell activity and 20E content
Supplementation of A. lobata D. Don cell culture with 100 μL L−1 terpineol significantly stimulated 20E synthesis, elevating the content to 145.5% higher than the control (p < 0.01) (Table ). However, at this concentration, and above, terpineol reduced cell growth and activity.
Table 4. Effect of terpineol on A. lobata cell suspension culture.
Influence of NO on A. lobata D. Don cell activity and 20E accumulation
There are no obvious differences of the cell FW between different concentrations of SNP (Fig. ). Supplementation of A. lobata D. Don cell culture with NO in the form of 10–40 μmol L−1 SNP, slightly reduced 20E accumulation, while higher levels of SNP enhanced 20E accumulation, which peaked at 2.84 ± 0.030 mg g−1 in the presence of 80 μmol L−1 SNP, and was slightly reduced in the presence of 160 μmol L−1 SNP (p < 0.01).
Influence of exogenous substrate combinations on A. lobata D. Don
Don cell growth and 20E content
In order to assess the affect of supplementation of culture medium with multiple medium compounds, the supplements that were found to enhance 20E content without significantly influencing cell growth rate were added together to culture medium. On the first day of culture, 50 μL L−1 α-Pinene and/or 10 mg L−1 MVA were added to medium, and 80 mmol L−1 SNP was added on the seventh day. On the eighth day, culture was harvested and the FW of cells and cellular 20E content was assessed (Table ).
Table 5. Effect of different combination of exogenous substrates on A. lobata cells.
Analysis of variance indicated that all supplements enhanced 20E accumulation (Table ). The 20E content of cells cultured with MVA, MVA + SNP, and MVA + α-Pinene was increased to 540, 578, and 425% of control levels, respectively, and addition of all three substrates further elevated the 20E content to 586% higher than the control cultures. The 20E content of MVA-, MVA + SNP-, and MVA + α-Pinene + SNP-treated cells did not differ significantly, but cells treated with MVA + α-Pinene was less significantly elevated (p < 0.01), at only 425% of control levels. The 20E content of cells cultured with α-Pinene, α-Pinene + SNP, and α-Pinene + MVA was 160, 425, and 426% of control levels, respectively (p < 0.01). The 20E content of cells cultured with SNP, α-Pinene + SNP, and SNP + MVA was 238, 425, and 578% of control levels, respectively, and addition of all three substrates significantly elevated the 20E content to 586% of control levels (p < 0.01). In summary, the combination of MVA + SNP + α-Pinene most significantly elevated 20E accumulation, followed – in order of potency – by MVA + SNP; MVA; MVA + α-Pinene; SNP + α-Pinene; α-Pinene; SNP; and CK.
Discussion
Changes in the pH of cell culture medium reflect changes in the composition of the medium, cell membrane permeability, and enzyme activity. In this study of A. lobata D. Don suspension cell culture, the pH of culture medium decreased rapidly from 5.8 to 5.2 with high temperature steam, and the reduction in the pH during the lag phase of cell growth might be a result of adaptation of cells to the suspension culture environment. Under suitable culture conditions, after a short lag period, cells will absorb ammonium ions, resulting in acidification of the culture medium.Citation44) Cells can also respond to ambient pH changes to maintain a relatively stable environment that is conductive to their growth and metabolism.Citation45)
Electric conductivity directly reflects the concentration of ions in the culture system, and indirectly reflects nutritional content of the medium as many nutrients are present as ions. Because cell growth and secondary metabolism are directly associated with the cell culture nutritional content,Citation46) we observed that electric conductivity reflected cell growth and 20E accumulated in A. lobata D. Don cells during cell suspension culture.
We also analyzed the relationship between nutrient consumption, cell growth, and biomass accumulation, and observed that the nutrient consumption curve corresponded to the cell growth curve. However, it should be noted that the consumption of ammonium salt and nitrate was not synchronized, as the rate of nitrate use was slower than the rate of ammonium salt use during the lag phase, and faster in the logarithm and stationary growth periods. After cells entered the decline phase, consumption of these substrates ceased. At the end of the culture, there is significant difference between the change of the nitrates and the ammonium salts. This phenomenon could be explained by the mechanisms of A. lobata D. Don cell growth requiring ammonium salts, while the accumulation of 20E was significantly associated with nitrate. Cusidó et al.Citation44) suggested that the NH4﹢ absorbed by cells or generated from NO3− will be assimilated immediately as high concentrations of NH4+ exert toxic effects including inhibition of ATP synthesis and hydrolysis during photoreaction. Understanding the kinetics of cell growth can inform optimization of cell culture conditions.Citation47) For example, the FW, DW, and growth curve form the basis for growth simulation of Rowan cells under conventional culture. The growth curve indicated Rowan cell growth fits the Logistic equation, and this equation is often used to represent the kinetics of cell growth in an enclosed system.Citation48–50) The Logistic equation was also used in this study to simulate cell growth; however, due to the complex biochemical reactions during cell growth, no model can incorporate all influencing factors. Thus, this study used sucrose as the only limiting factor, presuming biomass accumulation was synchronized with 20E synthesis. However, during in vitro culture, cell growth and biomass synthesis might not occur at an equivalent rate, and synthesis may only take place when cell proliferation decreased. The preliminary kinetic model was established to inform design of a cell culture reactor.
Aside from the influence of environmental factors and hormones on in vitro-cultured plant cells, cell groups or tissues, cell culture can also exert a significant effect on cell metabolism, resulting in genomic instability and loss of metabolites during cell passage.Citation51,52) Our results indicated that subculture weakened 20E production in A. lobata D. Don cells. Thus, alternations in environmental conditions, such as temperature, precursor concentration, nutrient and hormone supplementation, will be required to stimulate secondary metabolism, or new callus tissue should be routinely obtained from the plant to establish new suspension culture cycle. In order to measure the capacity of exogenous culture supplements to influence the culture of A. lobata D. Don cells, we added the indicated supplements to passage 10 A. lobata D. Don. At this passage, 20E production was observed to be less than in younger cells, and thus the influence of exogenous supplements on 20E accumulation could be clearly measured.
The boundary between primary and secondary metabolisms is blurred, and many amino acids, sterols, and terpenes are considered to be primary metabolites. Primary metabolism produced many small molecules that participate in secondary metabolism. Precursors refer to those primary metabolites that are directly associated with the synthesis of target secondary metabolites. Song et al.Citation53) suggested L-proline and L-ornithine, precursors of human stachydrine hydrochloric, enhanced accumulation of hydrochloric acid stachydrine in hydroponic culture of Leonurus japonicus by 52% and 13%, respectively. Supplementation of cultures with multiple precursors could also enhance the production of secondary metabolites. Lv et al.Citation54) studied a suspension culture of Cistanche deserticola and found L-Phe and L-tyrosine collaboratively exerted the most significant enhancement of secondary metabolism. As a key product in the MVA pathway, MVA could directly enhance the accumulation of plant secondary metabolites. In this study, when MVA was added to the culture system, cell growth was inhibited and cell activity was enhanced in a dose-dependent manner, and 20E accumulation was significantly enhanced. The toxicity of MVA may be attributed to MVA’s macromolecular structure, which prevents direct absorption and MVA might also influence the synthesis of metabolites involved in the early MVA pathway, thereby impeding normal cell metabolism and causing rapid cell browning and death.
Some substances could inhibit the activity of key enzymes involved in metabolism, thus promoting synthesis of a specific compound. Thus, adding inhibitors of alternative pathways is another way to stimulate secondary metabolism. For example, Gong et al.Citation55) added inhibitors to hydroponic culture of Festuca arundinacea which significantly reduced the activity of root protective enzymes, while the phenanthrene content was significantly increased, indicating that the inhibitors promoted phenanthrene synthesis by regulating the activity of enzymes involved in root metabolism. Chen et al.Citation56) suggested that metabolic inhibitors of polyamine reduced plant stress resistance by inhibiting the conversion of polyamines. We used monoterpenes α-Pinene and derivative terpineol as inhibitors of terpene synthesis, in order to redirect the MAV and DOXP/MEP pathways to increase the production of sterone.
Elicitors are substances or environmental factors that can induce plant defense responses. NO is widely recognized as an elicitor of secondary metabolism during plant culture, and the role of NO in synthetic regulation of various secondary metabolites has been a research focus in recent years. As a central factor in plant secondary metabolism transduction networks, NO plays the role of a molecular switch.Citation57–59). Xu et al.Citation60) suggested that in wide type Pueraria thomsonii Benth cells, a fungal elicitor could inhibit the synthesis of JA (jasmonic acid) by inducing synthesis of SA(salicylic acid), while NO promoted puerarin production via the SA pathway. However, in NahG cells, which were inhibited with SA accumulation, NO activated JA biosynthesis and promoted puerarin production in a JA pathway-dependent manner. Our results confirmed their findings, but higher concentration of NO was not more effective. A. lobata D. Don cells treated with 160 μmol L−1 SNP had a lower secondary metabolite level and 20E content than cells treated with 80 μmol L−1 SNP.
Conditions promoting cell growth and biomass accumulation may not necessarily increase secondary metabolite production, and sometimes more active secondary metabolism occurs when growth is inhibited. Based on the previous studies of terpenoid metabolism, we investigated the influence of α-Pinene, terpineol, L-Phe, and MVA on A. lobata D. Don activity and 20E synthesis. Supplementation of cultures with L-Phe and MVA significantly elevated cell activity and 20E synthesis, consistent with many studies of plant culture and secondary metabolite extraction. However, the addition of both α-Pinene and MVA in combination reduced the effect of MVA on 20E accumulation, an observation that requires further investigation.
The influence of α-Pinene, terpineol, L-Phe, and MVA on plant secondary metabolism has been extensively reported; however, the effect of these supplements in combination has not previously been reported. These substances influence plant secondary metabolism by different mechanisms, therefore a cumulative effect is possible. We observed that the combination of precursor + elicitor + metabolic inhibitor (MVA + SNP + α-Pinene) significantly increased 20E content, allowing the development of a system of A. lobata D. Don suspension culture with improved efficiency of large-scale industrial production of 20E. These findings also provide a concrete experimental basis for the mass production of a safe and pollution-free biological pesticide.
Authors contribution
Defu Chi and Jingjing Qian carried out the studies, participated in collecting data, and drafted the manuscript. Yueyue Yang and Xin Li performed the statistical analysis and helped to draft the manuscript. All authors read and approved the final manuscript.
Funding
This work was supported by the National Natural Science Foundation of China (NSFC) [grant number 31370649].
Disclosure statement
No potential conflict of interest was reported by the authors.
References
- Wang HM, Chen W, Liu DJ, et al. Determination of ecdysterone in Radix achyranthis bidentatae and Juejin Granula by HPLC. Chin. J. Exp. Traditional Med. Formulae. 2012;18:122–125.
- Lafont R, Horn DHS. Phytoecdysteroids: structures and occurrence. In: Koolman J, editor. Ecdysone from chemistry to mode of action. Stuttgart: Thieme Verlag; 1989. p. 39–64.
- Smagghe G. Ecdysone: structures and functions. Dordrecht: Springer Science. 2009; 3–45.
- Bathori M, Toth N, Hunyadi A, et al. Phytoecdysteroids and anabolic-androgenic steroids – structure and effects on humans. Curr. Med. Chem. 2008;15:75–91.10.2174/092986708783330674
- Dinan L. The Karlson Lecture. Phytoecdysteroids: what use are they? Arch. Insect Biochem. Physiol. 2009;72:126–141.
- Lagova ND, Valueva IM. Effect of ecdysterone isolated from Rhaponticum carthamoides on the growth of experimental tumors. Eksperimental’naya Onkologiya. 1981;3:69–71.
- Yoshida T, Otaka T, Uchiyama M, et al. Effect of ecdysterone on hyperglycemia in experimental animals. Biochem. Pharmacol. 1971;20:3263–3268.10.1016/0006-2952(71)90431-X
- Okui S, Otaka T, Uchiyama M, et al. Stimulation of protein synthesis in mouse liver by insect-moulting steroids. Chem. Pharm. Bull. (Tokyo). 1968;16:384–387.10.1248/cpb.16.384
- Otaka T, Okui S, Uchiyama M. Stimulation of protein synthesis in mouse liver by ecdysterone. Chem. Pharm. Bull. 1969;17:75–81.10.1248/cpb.17.75
- Detmar M, Dumas M, Bonte F. Effects of ecdysterone on the differentiation of normal human keratinocytes in vitro. Eur. J. Dermatol. 1994;4:558–562.
- Konovalova NR, Mitrokhin YI, Volkova LM, et al. Ecdysterone modulates antitumor activity of cytostatics and biosynthesis of macromolecules in umorbearing mice. Biol. Bull. 2002;29:530–536.10.1023/A:1021755622981
- Li JF. Introduction of botanical pesticides. Anhui Agric. Sci. Bull. 2009;15:160–162.
- He L, Wang LB, Jia L. The study on the mechanism of plant-insecticide. Pestic. Sci. Admin. 2013;34:16–19.
- Cheng DM, Yousef GG, Grace MH, et al. In vitro production of metabolism enhancing phytoecdysteroids from Ajuga turkestanica. Plant Cell Tiss. Org. 2008;93:73–83.10.1007/s11240-008-9345-5
- Baltaev UA. Phytoecdysteroids: structure, sources and biosynthetic pathways in plants. Bioorg Khim. 2000;26:892–925.
- Adler JH, Grebenok RJ. Biosynthesis and distribution of insect-molting hormones in plants – A review. Lipids. 1995;30:257–262.10.1007/BF02537830
- Benderoth M, Textor S, Windsor AJ, et al. Positive selection driving diversification in plant secondary metabolism. Proc. Nat. Acad. Sci. USA. 2006;103:9118–9123.10.1073/pnas.0601738103
- Catalan RE, Aragones MD, Godoy JE, et al. Ecdysterone induces acetylcholinesterase in mammalian brain. Comp. Biochem. Physiol. Part C: Pharmacol. 1984;78:193–195.10.1016/0742-8413(84)90068-9
- Catalán RE, Martinez AM, Aragones MD, et al. Alterations in rat lipid metabolism following ecdysterone treatment. Comp. Biochem. Physiol. Part B: Biochem. 1985;81:771–775.10.1016/0305-0491(85)90403-1
- Li X, Qian JJ,, Li XC, et al. A Study on edysterone production of Ajuga lobata D. Don cell lines in suspension culture. Chin. Agr. Sci. Bull. 2013;29: 127–133.
- Xiong Y, Qu W, Liang JY. Progress on chemical constituents and biological activities of the genus Ajuga. Strait Pharm. J. 2012;24:1–7.
- Li WW, Wu WL, Liu SJ, et al. The Chemical components of the genus Ajuga. Anhui. Med. Pharm. J. 2009;13:329–337.
- Chen F, Li XD, Wu FH. Ajuga decumbens Thunb and its dose-effect relation. J. Fujian Univ. of Traditional Chin. Med. 2009;19:27–29.
- Richter K, Birkenbeil H. The effect of extracts of Ajuga reptans on moult regulation in Periplaneta americana. J. Insect Physiol. 1987;33:933–939.10.1016/0022-1910(87)90005-9
- Lev SV, Zakirova RP, Saatov Z, et al. Ecdysteroids from tissue and cell cultures of Ajuga turkestanica. Khimiya Prirodnykh Soedinenii. 1990;1:51–52.
- Tomás J, Camps F, Claveria E, et al. Composition and location of phytoecdysteroids in Ajuga reptans in vivo and in vitro cultures. Phytochemistry. 1992;31:1585–1591.10.1016/0031-9422(92)83112-C
- Tanaka N, Matsumoto T. Regenerants of Ajuga hairy roots with high productivity of 20-hydroxyecdysone. Plant Cell Rep. 1993;13:87–90.10.1007/BF00235296
- Calcagno MP, Camps F, Coll J, et al. New phytoecdysteroids from roots of Ajuga reptans varieties. Tetrahedron. 1996;52:10137–10146.10.1016/0040-4020(96)00536-4
- Nagakari M, Kushiro T, Matsumoto T, et al. Incorporation of acetate and cholesterol into 20-hydroxyecdysone by hairy root clone of Ajuga reptans var. Atropurpurea. Phytochemistry. 1994;36:907–910.10.1016/S0031-9422(00)90461-8
- Fujimoto Y, Ohyama K, Nomura K, et al. Biosynthesis of sterols and ecdysteroids in Ajuga hairy roots. Lipids. 2000;35:279–288.10.1007/s11745-000-0524-z
- Hyodo R, Fujimoto Y. Biosynthesis of 20-hydroxyecdysone in Ajuga hairy roots: the possibility of 7-ene introduction at a late stage. Phytochemistry. 2000;53:733–737.10.1016/S0031-9422(00)00018-2
- Alekseeva LI. Ecdysone 20-monooxygenase activity of cytochrome P450 in Ajuga reptans L. plants and cell culture. Appl. Biochem. Micro. 2004;40:135–139.
- Uozumi N, Makino S, Kobayashi T. 20-Hydroxyecdysone production in Ajuga hairy root controlling intracellular phosphate content based on kinetic model. J. Ferment. Bioeng. 1995;80:362–368.10.1016/0922-338X(95)94205-6
- Rohmer M, Knani M, Simonin P, et al. Isoprenoid biosynthesis in bacteria: a novel pathway for the early steps leading to isopentenyl diphosphate. Biochem. J. 1993;295:517–524.10.1042/bj2950517
- Liao ZH, Chen M, Gong YF, et al. Isoprenoid biosynthesis in plants: pathways, genes, regulation and metabolic engineering. J. Biol. Sci. 2006;6:209–219.
- Reuter K, Sanderbrand S, Jomaa H, et al. Crystal structure of 1-deoxy-D-xylulose-5-phosphate Reductoisomerase, a crucial enzyme in the non-mevalonate pathway of isoprenoid biosynthesis. J. Biol. Chem. 2002;277:5378–5384.10.1074/jbc.M109500200
- Jomaa H, Wiesner J, Sanderbrand S, et al. Inhibitors of the nonmevalonate pathway of isoprenoid biosynthesis as antimalarial drugs. Science. 1999;285:1573–1576.10.1126/science.285.5433.1573
- Luo YM, Liu AH, Li Q, et al. Study development on the biosynthesis path of plant terpenoids and its key enzyme. J. Jiangxi College Traditional Chin. Med. 2003;15:45–51.
- Cao XB, Wang Z, Peng S, et al. Effects of lanthanum nitrate and L-phenylalanine on callus growth and total alkaloid accumulation of Pinellia ternata. Shandong Agric. Sci. 2012;44:26–28.
- Qiao M, Sun JW, Chen Y, et al. Wheat suspension cell replication elicitor stimulation of Ca2+ and NO allergic reaction dynamics and its interactions. Chin. Bull. Bot. 2015;50:1–11.
- Yang JC. Cell engineering. Beijing: Chemical industry press; 2008.
- Zhang CY, Liu J, Duan QQ, et al. Photo-induced NO relase in SNP aqueous solution and its photobiological appications. J. Shanxi Univ. (Nat. Sci. Ed.). 2014;37:609–613.
- Lou YJ, Chen Y, Yang J. Nitric Oxide releasing pathway and kinetics from sodium nitroprusside in Ringer-Locke’s solution. J. Zhenjiang Univ. 2000;06:241–244.
- Cusidó RM, Palazón J, Navia-Osorio A, et al. Production of Taxol® and baccatin III by a selected Taxus baccata callus line and its derived cell suspension culture. Plant Sci. 1999;146:101–107.10.1016/S0168-9452(99)00093-X
- Oh K, Kato T, Xu H. Transport of nitrogen assimilation in xylem vessels of green tea plants fed with NH4-N and NO3-N. Pedosphere. 2008;18:222–226.10.1016/S1002-0160(08)60010-7
- Li ZL, Rao QR, Li GX, et al. Monitor of cell growth and taxol accumulation in cell suspension culture of Taxus chinensis by conductivity of medium. Nat. Prod. Res. Dev. 2008;20:346–348.
- Xiao WJ, Yang G, Guo LP, et al. Study on the gowth kinetics of Sorbus sucuparia L. in suspension cultured cells. Mod. Chin. Med. 2013;15:569–573.
- Monod J. The Growth of Bacterial Cultures. Annu. Rev. Microbiol. 1949;3:371–394.10.1146/annurev.mi.03.100149.002103
- Gabriel JP, Saucy F, Bersier LF. Paradoxes in the logistic equation? Ecol. Model. 2005;185:147–151.10.1016/j.ecolmodel.2004.10.009
- Yue GJ, Liu WX, Liu JS, et al. Product kinetics of Logistic model to simulate ethanol fermentation. T. Chin. Soc. Agric. Eng. 2015;31:280–285.
- Hu F, Shi Q, Huang LJ. Induction of adventitious roots during tissue culture of Acacia mangium and A. auriculiformis elite trees. J. Nanjing For. Univ. (Nat. Sci. Edit.). 2015;39:57–62.
- Su YL, Tian P, Wei WD. Induction and proliferation culture of adventitious shoots from Pyrus communis leaves. Nonwood For. Res. 2015;33:103–106.
- Song T, Peng F, Tan CY. Effect of precursors on the content of stachydrine hydrochloride in the hydroponic Herba leonuri. Cent. S. Pharm. 2012;10:814–817.
- Lv JJ, Hu GS, Li JK, et al. Effects of precursor feeding and fungal elicitors on secondary metabolits in cell suspension culture of Cistanche deserticola. J. Chin. Med. Mater. 2009;32:171–173.
- Gong SS, Han J, Gao YZ, et al. Effects of inhibitor and safener on enzyme activity and phenanthrene metabolism in root of tall fescue. Acta Ecol. Sin. 2011;31:4027–4033.
- Chen XW, Ma YP, Xu CX, et al. Effects of exogenous polyamines and polyamine metabolism inhibitors on and chloroplastsionic homeostasis and thylakoid membrane conjugated H+-ATPase activity of Ziziphus jujuba Mill. under salt stress. J. Cent. S. Univ. For. Tech. 2012;32:1–8
- Wang XJ, Li Z, Peng Y. The antioxidant enzyme activities and gene expression induced by spermidine in leaves of white clover. Acta Pratac. Sin. 2015;24:140–147.
- Liu JX, Hu HB, Wang X, et al. Effect of nitric oxide on proline accumulation in ryegrass seedlings subjected to salt stress. Agrestia Sin. 2010;18:786–791.
- Wang F, Chang PP, Chen YP, et al. Effects of exogenous nitric oxide on seedling growth and physiological characteristics of maize seedlings under cadmium stress. Acta Pratac. Sin. 2013;22:178–186.
- Xu MJ, Dong JF, Zhu MY. Nitric oxide mediates the fungal elicitor-induced puerarin biosynthesis in Pueraria thomsonii Benth. suspension cells through a salicylic acid (SA)-dependent and a jasmonic acid (JA)-dependent signal pathway. Sci. Chin. Ser. C: Life Sci. 2006; 2006, 49: 379–389.10.1007/s11427-006-2010-5