Abstract
Sterol regulatory element-binding proteins (SREBPs) are a family of transcription factors that regulate lipid homeostasis by controlling the expression of genes involved in fatty acid and cholesterol synthesis. In this study, we used a stable cell line that expresses a luciferase reporter gene driven by an SRE-containing fatty acid synthase promoter to identify allyl isothiocyanate (AITC), one of the major isothiocyanates in cruciferous vegetables, as a novel SREBP inactivator. We found that AITC downregulated the proteolytic processing of SREBPs and the expression of their target genes in human hepatoma Huh-7 cells. Furthermore, AITC reduced the de novo synthesis of both fatty acids and cholesterol. Our results indicate a novel physiological function of AITC in lipid metabolism regulation.
Graphical abstract
Allyl isothiocyanate suppresses the processing of SREBP-1 and SREBP-2.
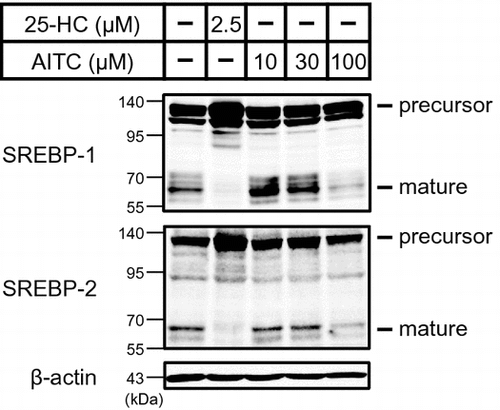
Obesity is a chronic metabolic disorder caused by energy imbalance due to long-term excessive food intake and insufficient physical activity and is associated with type II diabetes, insulin resistance, and cardiovascular disease.Citation1) The increasing prevalence of obesity is a rapidly growing health-related problem throughout the world.Citation2) The prevention and amelioration of obesity should lower the risks of these diseases.
Sterol regulatory element-binding proteins (SREBPs) are a family of transcription factors that regulate lipid biosynthesis.Citation3) The SREBP family comprises three isoforms: SREBP-1c, which mainly enhances the transcription of genes encoding enzymes of fatty acid synthesis; SREBP-2, which predominantly stimulates the expression of genes involved in cholesterol synthesis; and SREBP-1a, which is a potent activator of genes involved in both fatty acid and cholesterol synthetic pathways.Citation4) SREBPs are synthesized as inactive precursors located on the endoplasmic reticulum (ER) membrane. In cells with low sterol levels, these precursors are processed to liberate the N-terminal halves that function as transcription factors in the nucleus. The synthesized sterols then suppress the proteolytic cleavage of SREBPs, and therefore, lipid homeostasis is controlled by a sterol feedback system. The expression and proteolytic processing of SREBP-1c are markedly enhanced in the livers of obese mice.Citation5,6) Moreover, the overexpression of SREBP-1c in transgenic mouse livers leads to the development of fatty liver, hypertriglyceridemia, and insulin resistance.Citation7) Thus, the aberrant regulation of SREBP-1c may play an important role in the pathogenesis of obesity-associated diseases.
To identify new SREBP inactivators, we previously established a human hepatoma Huh-7 cell line that stably expresses a luciferase reporter gene under the control of SRE-containing fatty acid synthase (FAS) promoter (Huh-7/FAS-luc).Citation8) Using this cell line, we found that 4′-hydroxyflavanone (4′-HF), a synthetic analog of flavanone, and xanthohumol (XN), a prenylated flavonoid from hops, attenuated the activities of SREBPs.Citation8,9) In this study, we identified allyl isothiocyanate (AITC), one of the major isothiocyanates in cruciferous vegetables, such as mustard, wasabi, and cabbage, as a natural food component that reduced the activities of SREBPs. Furthermore, AITC suppressed the proteolytic processing of SREBPs and the de novo synthesis of fatty acids and cholesterol.
Materials and methods
Reagents
25-Hydroxycholesterol (25-HC), fluvastatin, and lipoprotein-deficient serum (LPDS) were purchased from Sigma. AITC and Dulbecco’s modified Eagle’s medium (DMEM) were purchased from Wako.
Cell culture
Huh-7 cells were maintained in medium A (DMEM supplemented with 100 units/mL penicillin, 100 μg/mL streptomycin, and 10% fetal bovine serum). Huh-7/FAS-luc (a stable cell line of Huh-7 that expresses a luciferase reporter driven by an SRE-containing FAS promoter) cellsCitation8) were maintained in medium A containing 2 μg/mL blasticidin S. The cells were incubated at 37 °C under a 5% CO2 atmosphere.
Luciferase assays
Huh-7/FAS-luc cells were plated in 12-well plates at a density of 1.0 × 105 cells/well, cultured with medium A for 24 h, and the cells were then switched to medium B (medium A supplemented with 12.5 μM fluvastatin and 50 μM sodium mevalonate) for 16 h. After incubation for another 24 h in the absence or presence of 10, 30, or 100 μM AITC, the luciferase activity and protein contents of the cell extracts were measured as described previously.Citation10) The normalized luciferase values were determined by dividing the luciferase activity by the protein content in the cell extracts quantified using the BCA protein assay (Pierce).
Real-time quantitative PCR
Huh-7 cells were plated in six-well plates at a density of 5.0 × 105 cells/well and cultured with medium A for 24 h. The cells were then switched to medium C (DMEM supplemented with 100 units/mL penicillin, 100 μg/mL streptomycin, 5% LPDS, 12.5 μM fluvastatin, and 50 μM sodium mevalonate) for 16 h. After incubation for another 24 h in the absence or presence of 100 μM AITC, total RNA was extracted from the cells using Isogen (Nippon Gene) according to the manufacturer’s instructions. RNA was reverse-transcribed using a high capacity cDNA reverse transcription kit (Applied Biosystems). Real-time quantitative PCR (TaqMan probe and SYBR Green) analysis was performed using an Applied Biosystems StepOnePlus instrument. The expression levels were normalized against those of the glyceraldehyde-3-phosphate dehydrogenase (GAPDH) control. The TaqMan ID numbers for the analyzed genes are as follows: human (h) FAS, Hs00188012_m1; h stearoyl-CoA desaturase 1 (SCD1), Hs00748952_s1; hGAPDH, 4352934. The sequences of the primer sets used were as follows: h acetyl-CoA carboxylase 1 (ACC1), 5′-TGGGCCTCAAGAGGATTTGT-3′ and 5′-TCCACTGTTGGCTGATACATAGATG-3′; h HMG-CoA synthase (HMGCS), 5′-GACTTGTGCATTCAAACATAGCAA-3′ and 5′-GCTGTAGCAGGGAGTCTTGGTACT-3′; h HMG-CoA reductase (HMGCR), 5′-TACCATGTCAGGGGTACGTC-3′ and 5′-CAAGCCTAGAGACATAATCATC-3′; h squalene synthase (SQS), 5′-ATGACCATCAGTGTGGAAAAGAAG-3′.
Antibodies
Monoclonal anti-SREBP-1 (2A4) antibody was purchased from Santa Cruz Biotechnology. Monoclonal anti-β-actin (AC-15) antibody was purchased from Sigma. Polyclonal anti-SREBP-2 (RS004) antibody has been previously described.Citation11) Peroxidase-conjugated affinity-purified donkey anti-mouse IgG and donkey anti-rabbit IgG were purchased from Jackson ImmunoResearch.
Immunoblotting
Huh-7 cells were plated in six-well plates at a density of 5.0 × 105 cells/well and cultured with medium A for 24 h. The cells were then switched to medium C for 16 h. After incubation for another 3 h in the absence or presence of 10, 30, or 100 μM AITC, the cells were lysed in RIPA buffer (50 mM Tris–HCl, pH 8.0, 150 mM NaCl, 1% Triton X-100, 0.5% deoxycholate, and 0.1% sodium dodecyl sulfate) supplemented with a protease inhibitor mixture. The lysates were subjected to sodium dodecyl sulfate-polyacrylamide gel electrophoresis, transferred to a polyvinylidene difluoride membrane, and probed with the antibodies indicated in the figure legends. The immunoreactive proteins were visualized using ECL (GE Healthcare) or Immobilon (Millipore) immunoblotting detection reagents. The signals on the membrane were detected by an ImageQuant LAS 4000 mini (GE Healthcare) system.
Measurement of de novo fatty acid and cholesterol synthesis
Huh-7 cells were plated in 12-well plates at a density of 2.0 × 105 cells/well and cultured with medium A for 24 h. The cells were then switched to medium D (DMEM supplemented with 100 units/mL penicillin, 100 μg/mL streptomycin, and 5% LPDS) for 16 h. After incubation for another 18 h in the absence or presence of 100 μM AITC, the cells were placed in fresh medium supplemented with 1.6 μCi/mL [14C]-acetate and incubated for 6 h. Next, the cells were washed with phosphate-buffered saline, scraped, and collected by centrifugation. The cell pellets were mixed with 1 mL of 8 N potassium hydrate and 1 mL of ethanol. The mixture was heated at 100 °C for 2 h and extracted two times with 2 mL of petroleum ether (cholesterol extract). In addition, 2 mL of the lower aqueous layer was initially mixed with 1 mL of 12 N hydrochloric acid and then extracted two times with 3 mL of petroleum ether (fatty acid extract). The lipid extracts were then resolved by thin-layer chromatography on Silica Gel 60 (Merck) and quantified with a BAS2000 image analysis system (Fujifilm). The normalized fatty acid and cholesterol synthesis rates were determined by dividing the signals for [14C]-fatty acid and [14C]-cholesterol by the amounts of the total cellular protein quantified using the BCA protein assay.
Statistical analysis
All data are presented as mean ± S.E. Statistical analysis was performed using Ekuseru-Toukei Version 2.0 (Social Survey Research Information). The Student’s t-test was used to compare pairs of groups. Significance was assumed at p < 0.05 and p < 0.01. One-way analysis of variance followed by the Bonferroni procedure was used to compare more than two groups. Differences were considered significant at p < 0.05.
Results
AITC reduces the promoter activity of the FAS gene
To identify SREBP inactivators, we used a human hepatoma Huh-7 cell line that stably expressed a luciferase reporter gene under the control of an SRE-containing FAS promoter (Huh-7/FAS-luc).Citation8) Here, we used Huh-7 cells because it is easy to detect SREBP activation in these cells owing to their high sensitivity toward changes in sterol levels. To promote SREBP activation, Huh-7/FAS-luc cells were initially incubated in sterol-depleted medium containing LPDS and an HMGCR inhibitor (fluvastatin). The cells were then treated with approximately 100 naturally occurring food constituents, and their effects on SREBP activity were evaluated. As a positive control, we confirmed that 25-HC, a potent inhibitor of SREBP processing, significantly lowered the luciferase activity of Huh-7/FAS-luc cells (Fig. (A)). Using this assay system, we identified AITC as a potent SREBP inactivator (Fig. (A) and (B)).
Fig. 1. AITC reduces the activity of the SRE-containing FAS promoter.
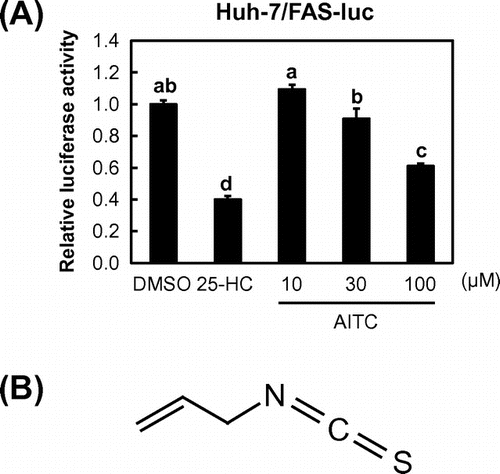
AITC suppresses the proteolytic processing of SREBPs
Next, we investigated whether AITC affects the proteolytic activation of SREBPs. To stimulate SREBP processing and detect the mature forms of SREBPs, sterols in Huh-7 cells were depleted by the incubation of these cells in a medium containing fluvastatin and LPDS. Huh-7 cells were then treated using AITC for 3 h under sterol-depleted conditions. The whole-cell lysates were subjected to immunoblotting using anti-SREBP-1 and anti-SREBP-2 antibodies. Similar to 25-HC, 100 μM AITC suppressed SREBP-1 and SREBP-2 processing, according to the decreases in the mature forms (Fig. ).
Fig. 2. AITC suppresses the processing of SREBP-1 and SREBP-2. Sterols in Huh-7 cells were depleted by incubating these cells in medium C for 16 h.
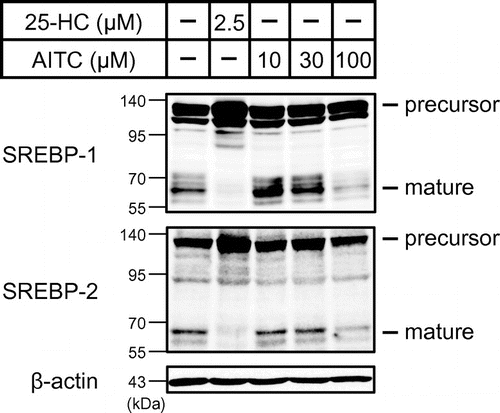
AITC inhibits the expression levels of SREBP target genes as well as the de novo synthesis of fatty acids and cholesterol
To determine whether AITC regulates SREBP target gene expression, Huh-7 cells were treated with AITC for 24 h. Total RNA was prepared and subjected to quantitative real-time PCR analysis. The mRNA analysis demonstrated that the gene expression levels of three SREBP-1 target genes involved in fatty acid synthesis, i.e., ACC1, FAS, and SCD1, were downregulated significantly by 100 μM of AITC (Fig. (A)). Similarly, AITC treatment reduced the mRNA levels of three SREBP-2 target genes involved in cholesterol synthesis, i.e., HMGCS, HMGCR, and SQS (Fig. A). We also examined the effect of AITC on lipogenesis, which showed that the de novo synthesis of fatty acids and cholesterol was reduced by AITC (Fig. B).
Fig. 3. AITC suppresses SREBP target gene expression as well as the de novo synthesis of fatty acids and cholesterol.
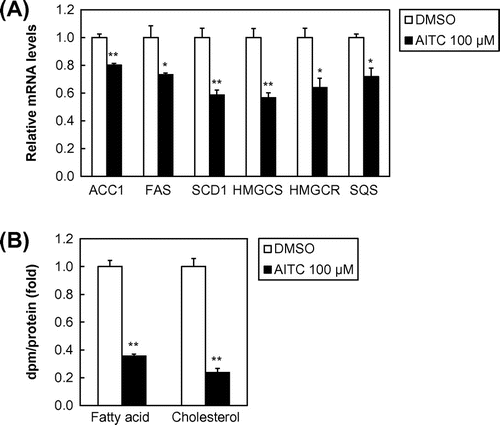
Discussion
The results of this study demonstrated that AITC reduced the SRE-containing FAS promoter activity in Huh-7/FAS-luc cells. Moreover, we showed that AITC suppressed the proteolytic processing of SREBPs. The reduction in the SREBP activity caused by AITC treatment suppressed SREBP target gene expression as well as de novo fatty acid and cholesterol synthesis in Huh-7 cells.
AITC is an organosulfur phytochemical, which is abundant in common cruciferous vegetables such as mustard, wasabi, and cabbage.Citation12–14) AITC has many desirable attributes as a cancer chemopreventive agent, including microbicidal activity,Citation15–17) apoptosis-inducing ability in cancer cells,Citation18,19) activation of nuclear factor erythroid 2-related factor 2 signaling,Citation20,21) and inhibition of cancer growth.Citation22,23) Moreover, it has been reported that the oral administration of AITC caused a decrease in body weight and blood glucose level in rats.Citation24) A recent study also demonstrated that AITC reduces obesity and insulin resistance by correcting high-fat diet-induced mitochondrial dysfunction.Citation25) However, little is known about the effects of AITC on lipid metabolism. In this study, we demonstrated that AITC lowers the activity of SREBP transcription factors, which are the master regulators of lipid homeostasis.
SREBP activity is regulated primarily by proteolytic processing. When cells are depleted of sterols, SREBP cleavage-activating protein (SCAP) binds to Sec23/24, which clusters the SCAP/SREBP complex into common coated protein II (COP II) vesicles.Citation26) These vesicles then transport the SCAP/SREBP complex from the ER to the Golgi, where the SREBPs are processed proteolytically by two proteases, i.e., site-1 protease (S1P) and site-2 protease (S2P), to release mature forms.Citation27,28) When the cellular sterol levels are sufficient, SCAP associates with insulin-induced genes (Insigs), which facilitate the retention of the SCAP/SREBP complex in the ER, thereby suppressing SREBP processing.Citation29) Several studies have investigated small molecules that inhibit the proteolytic activation of SREBPs. Betulin, a pentacyclic triterpene isolated from birch bark, and fatostatin, a synthetic diarylthiazole molecule, impair the ER-to-Golgi translocation of SREBPs by binding to their escort protein SCAP.Citation30,31) Recently, we reported that XN, a prenylated flavonoid from hops, interacts with Sec23/24 and blocks the sorting of the SCAP/SREBP complex into COP II vesicles.Citation9) In this study, we showed that AITC inhibits the proteolytic processing of SREBPs. It is possible that AITC suppresses the transport of SREBPs from the ER to the Golgi by acting on key factors involved in this transport such as SCAP, Insigs, and COP II components. Alternatively, AITC may reduce the cleavage of SREBPs by inhibiting the activities of S1P and S2P. It is also possible that AITC regulates specific protein kinases involved in SREBP processing, such as AMP-activated kinase (AMPK) and Akt. Previously, it was demonstrated that AMPK phosphorylates SREBPs and suppresses SREBP maturationCitation32) and that Akt promotes the ER-to-Golgi transport of the SCAP/SREBP complex.Citation33) At present, the molecular mechanism that allows AITC to attenuate SREBP processing is poorly understood, and thus further investigations are required to determine the detailed functions of AITC.
Using Huh-7/FAS-luc cells, we previously identified 4′-HF, a synthetic analog of flavanone, and XN, a prenylated flavonoid from hops, as SREBP inactivators.Citation8,9) In this study, we showed that AITC inhibits SREBP activation. AITC has a different chemical structure from flavonoids, including 4′-HF and XN. AITC belongs to the isothiocyanate family with members that contain an –N=C=S reactive group, such as benzyl isothiocyanate, phenethyl isothiocyanate, and sulforaphane. Various isothiocyanates, including these compounds, have been shown to exert chemopreventive effects in numerous studies, and it has been proposed that at least some of their activities are initiated via the direct reaction of the carbon atom in the –N=C=S reactive group with the cysteine sulfhydryl groups of target proteins, thereby causing conformational changes in these proteins.Citation34) Thus, it is possible that the –N=C=S group of AITC reacts with cysteine residues in specific proteins involved in the regulation of SREBP processing such as those mentioned above. During the proteolytic processing of SREBPs, cholesterol binds to SCAP and induces conformational changes, which promote the association of SCAP with Insigs, and thus the conformational states of SCAP are crucial for regulating the activity of SREBP.Citation35) Structural and biochemical analyses of SCAP in fission yeast showed that substitutions of two cysteine residues, C618S/C680S, led to a decrease in the binding affinity with SREBP, thereby suggesting that specific cysteine residues in SCAP are important for the interaction between SCAP and SREBP.Citation36) Therefore, it is conceivable that the –N=C=S group of AITC binds to SCAP via cysteine residues and that it stimulates SCAP conformation changes, thereby attenuating its association with SREBP. Thus, the –N=C=S group may be essential for suppressing the proteolytic activation of SREBPs, so the effects of isothiocyanates other than AITC on the activity of SREBPs must also be investigated.
In summary, our study demonstrated that AITC is a novel SREBP inactivator, which reduces the de novo synthesis of fatty acids and cholesterol. SREBPs function as principal regulators of lipid biosynthesis and excess SREBP activation is linked to metabolic disease states. Thus, the inhibition of SREBP activation could be a promising strategy for ameliorating metabolic disorders. A few studies have focused on the relationship between AITC and metabolic diseases, but our results may partly explain the anti-obesity effect of AITC. Moreover, the activity of SREBPs is probably required for tumor growth because cancer cells need to maintain a lipid supply to continue their rapid proliferation, and the SREBP pathway has been highlighted as a potential target for anti-cancer therapy.Citation37) Our results also indicate that suppressing the activity of SREBPs may partly contribute to the well-known anti-cancer effect of AITC. Further studies are needed to elucidate the detailed mechanism and the in vivo efficacy of AITC. Overall, this study indicates that AITC has the potential to become a functional food or pharmacological agent for treating obesity and related diseases.
Author contributions
JI and RS conceived the project. SM and JI designed the study. SM performed the experiments. SM, JI, MS, and RS analyzed the data. SM and JI wrote the manuscript. All authors read and approved the manuscript.
Funding
This work was supported by the JSPS KAKENHI [grant numbers 15H05781, 24580175, 25126703].
Disclosure statement
No potential conflict of interest was reported by the authors.
Acknowledgments
We would like to thank Enago (www.enago.jp) for the English language review.
Notes
Abbreviations: ACC1, acetyl-CoA carboxylase 1; AITC, allyl isothiocyanate; AMPK, AMP-activated kinase; COP II, coated protein II; DMEM, Dulbecco’s modified Eagle’s medium; ER, endoplasmic reticulum; FAS, fatty acid synthase; GAPDH, glyceraldehyde-3-phosphate dehydrogenase; 25-HC, 25-Hydroxycholesterol; 4′-HF, 4′-hydroxyflavanone; HMGCR, HMG-CoA reductase; HMGCS, HMG-CoA synthase; Insigs, insulin-induced genes; LPDS, lipoprotein-deficient serum; SCAP, SREBP cleavage-activating protein; SCD1, stearoyl-CoA desaturase 1; S1P, site-1 protease; S2P, site-2 protease; SQS, squalene synthase; SREBPs, sterol regulatory element-binding proteins; XN, xanthohumol.
References
- Isganaitis E, Lustig RH. Fast food, central nervous system insulin resistance, and obesity. Arterioscler Thromb. Vasc. Biol. 2005;25:2451–2462.10.1161/01.ATV.0000186208.06964.91
- Mokdad AH, Bowman BA, Ford ES, et al. The continuing epidemics of obesity and diabetes in the United States. JAMA. 2001;286:1195–1200.10.1001/jama.286.10.1195
- Brown MS, Goldstein JL. A proteolytic pathway that controls the cholesterol content of membranes, cells, and blood. Proc. Natl. Acad. Sci. USA. 1999;96:11041–11048.10.1073/pnas.96.20.11041
- Horton JD, Goldstein JL, Brown MS. SREBPs: activators of the complete program of cholesterol and fatty acid synthesis in the liver. J. Clin. Invest. 2002;109:1125–1131.10.1172/JCI0215593
- Shimomura I, Bashmakov Y, Horton JD. Increased levels of nuclear SREBP-1c associated with fatty livers in two mouse models of diabetes mellitus. J. Biol. Chem. 1999;274:30028–30032.10.1074/jbc.274.42.30028
- Kammoun HL, Chabanon H, Hainault I, et al. GRP78 expression inhibits insulin and ER stress-induced SREBP-1c activation and reduces hepatic steatosis in mice. J. Clin. Invest. 2009;119:1201–1215.10.1172/JCI37007
- Knebel B, Haas J, Hartwig S, et al. Liver-specific expression of transcriptionally active SREBP-1c is associated with fatty liver and increased visceral fat mass. PLoS One. 2012;7:e31812.10.1371/journal.pone.0031812
- Miyata S, Inoue J, Shimizu M, et al. 4′-Hydroxyflavanone suppresses activation of sterol regulatory element-binding proteins and de novo lipid synthesis. FEBS Lett. 2012;586:1778–1782.10.1016/j.febslet.2012.04.060
- Miyata S, Inoue J, Shimizu M, et al. Xanthohumol improves diet-induced obesity and fatty liver by suppressing sterol regulatory element-binding protein (SREBP) activation. J. Biol. Chem. 2015;290:20565–20579.10.1074/jbc.M115.656975
- Sato R, Inoue J, Kawabe Y, et al. Sterol-dependent transcriptional regulation of sterol regulatory element-binding protein-2. J. Biol. Chem. 1996;271:26461–26464.
- Sato R, Miyamoto W, Inoue J, et al. Sterol regulatory element-binding protein negatively regulates microsomal triglyceride transfer protein gene transcription. J. Biol. Chem. 1999;274:24714–24720.10.1074/jbc.274.35.24714
- Uematsu Y, Hirata K, Suzuki K. Determination of isothiocyanates and related compounds in mustard extract and horseradish extract used as natural food additives. Shokuhin Eiseigaku Zasshi. 2002;43:10–17.10.3358/shokueishi.43.10
- Sultana T, Savage GP, McNeil DL, et al. Effects of fertilisation on the allyl isothiocyanate profile of above-ground tissues of New Zealand-grown wasabi. J. Sci. Food Agric. 2002;82:1477–1482.10.1002/(ISSN)1097-0010
- Rungapamestry V, Duncan AJ, Fuller Z, et al. Changes in glucosinolate concentrations, myrosinase activity, and production of metabolites of glucosinolates in cabbage (Brassica oleracea Var. capitata) cooked for different durations. J. Agric. Food Chem. 2006;54:7628–7634.10.1021/jf0607314
- Shin IS, Masuda H, Naohide K. Bactericidal activity of wasabi (Wasabia japonica) against Helicobacter pylori. Int. J. Food Microbiol. 2004;94:255–261.10.1016/S0168-1605(03)00297-6
- Tunc S, Chollet E, Chalier P, et al. Combined effect of volatile antimicrobial agents on the growth of Penicillium notatum. Int. J. Food Microbiol. 2007;113:263–270.10.1016/j.ijfoodmicro.2006.07.004
- Luciano FB, Holley RA. Enzymatic inhibition by allyl isothiocyanate and factors affecting its antimicrobial action against Escherichia coli O157:H7. Int. J. Food Microbiol. 2009;131:240–245.10.1016/j.ijfoodmicro.2009.03.005
- Xiao D, Srivastava SK, Lew KL, et al. Allyl isothiocyanate, a constituent of cruciferous vegetables, inhibits proliferation of human prostate cancer cells by causing G2/M arrest and inducing apoptosis. Carcinogenesis. 2003;24:891–897.10.1093/carcin/bgg023
- Xu C, Shen G, Yuan X, et al. ERK and JNK signaling pathways are involved in the regulation of activator protein 1 and cell death elicited by three isothiocyanates in human prostate cancer PC-3 cells. Carcinogenesis. 2006;27:437–445.10.1093/carcin/bgi251
- Ye L, Zhang Y. Total intracellular accumulation levels of dietary isothiocyanates determine their activity in elevation of cellular glutathione and induction of Phase 2 detoxification enzymes. Carcinogenesis. 2001;22:1987–1992.10.1093/carcin/22.12.1987
- Jeong WS, Keum YS, Chen C, et al. Differential expression and stability of endogenous nuclear factor E2-related factor 2 (Nrf2) by natural chemopreventive compounds in HepG2 human hepatoma cells. J. Biochem. Mol. Biol. 2005;38:167–176.10.5483/BMBRep.2005.38.2.167
- Smith T, Musk SR, Johnson IT. Allyl isothiocyanate selectively kills undifferentiated HT29 cells in vitro and suppresses aberrant crypt foci in the colonic mucosa of rats. Biochem. Soc. Trans. 1996;24:381s.10.1042/bst024381s
- Srivastava SK, Xiao D, Lew KL, et al. Allyl isothiocyanate, a constituent of cruciferous vegetables, inhibits growth of PC-3 human prostate cancer xenografts in vivo. Carcinogenesis. 2003;24:1665–1670.10.1093/carcin/bgg123
- Lewerenz HJ, Plass R, Bleyl DW, et al. Short-term toxicity study of allyl isothiocyanate in rats. Nahrung. 1988;32:723–728.10.1002/(ISSN)1521-3803
- Ahn J, Lee H, Im SW, et al. Allyl isothiocyanate ameliorates insulin resistance through the regulation of mitochondrial function. J. Nutr. Biochem. 2014;25:1026–1034.10.1016/j.jnutbio.2014.05.006
- Espenshade PJ, Li WP, Yabe D. Sterols block binding of COPII proteins to SCAP, thereby controlling SCAP sorting in ER. Proc. Natl. Acad. Sci. USA. 2002;99:11694–11699.10.1073/pnas.182412799
- Duncan EA, Brown MS, Goldstein JL, et al. Cleavage site for sterol-regulated protease localized to a leu-Ser bond in the lumenal loop of sterol regulatory element-binding protein-2. J. Biol. Chem. 1997;272:12778–12785.10.1074/jbc.272.19.12778
- Rawson RB, Zelenski NG, Nijhawan D, et al. Complementation cloning of S2P, a gene encoding a putative metalloprotease required for intramembrane cleavage of SREBPs. Mol. Cell. 1997;1:47–57.10.1016/S1097-2765(00)80006-4
- Yabe D, Brown MS, Goldstein JL. Insig-2, a second endoplasmic reticulum protein that binds SCAP and blocks export of sterol regulatory element-binding proteins. Proc. Natl. Acad. Sci. USA. 2002;99:12753–12758.10.1073/pnas.162488899
- Tang JJ, Li JG, Qi W, et al. Inhibition of SREBP by a small molecule, betulin, improves hyperlipidemia and insulin resistance and reduces atherosclerotic plaques. Cell Metab. 2011;13:44–56.10.1016/j.cmet.2010.12.004
- Kamisuki S, Mao Q, Abu-Elheiga L, et al. A small molecule that blocks fat synthesis by inhibiting the activation of SREBP. Chem. Biol. 2009;16:882–892.10.1016/j.chembiol.2009.07.007
- Li Y, Xu S, Mihaylova MM, et al. AMPK phosphorylates and inhibits SREBP activity to attenuate hepatic steatosis and atherosclerosis in diet-induced insulin-resistant mice. Cell Metab. 2011;13:376–388.10.1016/j.cmet.2011.03.009
- Du X, Kristiana I, Wong J, et al. Involvement of Akt in ER-to-Golgi transport of SCAP/SREBP: a link between a key cell proliferative pathway and membrane synthesis. Mol. Biol. Cell. 2006;17:2735–2745.10.1091/mbc.E05-11-1094
- Zhang Y. The 1,2-benzenedithiole-based cyclocondensation assay: a valuable tool for the measurement of chemopreventive isothiocyanates. Crit. Rev. Food Sci. Nutr. 2012;52:525–532.10.1080/10408398.2010.503288
- Sun LP, Seemann J, Goldstein JL, et al. Sterol-regulated transport of SREBPs from endoplasmic reticulum to Golgi: Insig renders sorting signal in Scap inaccessible to COPII proteins. Proc. Natl. Acad. Sci. USA. 2007;104:6519–6526.10.1073/pnas.0700907104
- Gong X, Li J, Shao W, et al. Structure of the WD40 domain of SCAP from fission yeast reveals the molecular basis for SREBP recognition. Cell Res. 2015;25:401–411.10.1038/cr.2015.32
- Guo D, Bell EH, Mischel P, et al. Targeting SREBP-1-driven lipid metabolism to treat cancer. Curr. Pharm. Des. 2014;20:2619–2626.10.2174/13816128113199990486