Abstract
Fluvirucins are 14-membered macrolactam polyketides that show antifungal and antivirus activities. Fluvirucins have the β-alanine starter unit at their polyketide skeletons. To understand the construction mechanism of the β-alanine moiety in fluvirucin biosyntheses, we have identified the biosynthetic cluster of fluvirucin B2 produced from Actinomadura fulva subsp. indica ATCC 53714. The identified gene cluster contains three polyketide synthases, four characteristic β-amino acid-carrying enzymes, one decarboxylase, and one amidohydrolase. We next investigated the activity of the adenylation enzyme FlvN, which is a key enzyme for the selective incorporation of a β-amino acid substrate. FlvN showed strong preference for l-aspartate over other amino acids such as β-alanine. Based on these results, we propose a biosynthetic pathway for fluvirucin B2.
Graphical abstract
Proposed biosynthetic pathway for fluvirucin B2
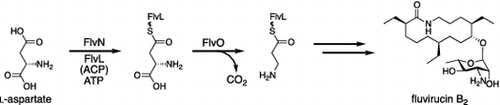
Fluvirucins are potent antifungal and antivirus 14-membered macrolactam antibiotics (Fig. ).Citation1–6) One of the structural features of fluvirucins is the β-alanine starter unit of the polyketide skeleton, which is almost fully reduced and has a single hydroxy group. Currently, a variety of fluvirucin derivatives with several combinations of alkyl side chains and deoxyaminosugars have been isolated from several actinomycetes. For example, fluvirucin B1 has ethyl side chains at the C2 and C10 positions and a methyl side chain at the C6 position and contains 3-amino-3,6-dideoxy-l-talopyranose through the C9 hydroxy group. Fluvirucin B2 (Sch38518) has ethyl side chains at all of the C2, C6, and C10 positions and contains l-mycosamine. Because of their attractive biological activities, organic syntheses of these fluvirucin compounds have been reported.Citation7–9)
The biosynthetic pathway of vicenistatin, which is a related macrolactam antibiotic, has been elucidated.Citation10–12) In the vicenistatin biosynthetic pathway, (2S,3S)-3-methylaspartate (3-MeAsp) is first activated by the adenylation enzyme VinN and ligated with the standalone acyl carrier protein (ACP) VinL to give 3-MeAsp-ACP. Then, the PLP-dependent decarboxylase VinO removes the α-carboxy group of the 3-MeAsp-ACP. The resulting β-aminoisobutyryl-ACP is further aminoacylated with l-alanine by another adenylation enzyme VinM to give a dipeptidyl-ACP, which is then transferred to the loading ACP domain of polyketide synthase (PKS) VinP1 by acyltransferase VinK. After polyketide chain elongation, the terminal alanyl group is removed from the polyketide chain by the amidohydrolase VinJ prior to macrocyclization. In addition to the vicenistatin biosynthetic gene cluster, various related macrolactam biosynthetic gene clusters have been identified.Citation12–18) Homologous genes for four β-amino acid-carrying enzymes VinN, VinL, VinM, and VinK and one amidohydrolase VinJ are fully conserved in these gene clusters. Therefore, the same scenario, except for the decarboxylation step catalyzed by VinO, is proposed for the incorporation step of the β-amino acid starter unit in these macrolactam biosynthetic pathways. Among these starter-related enzymes, VinN-type adenylation enzyme is relatively diverse. The recognition of a specific β-amino acid by the VinN-type adenylation enzyme appears to be crucial for the selective incorporation of a β-amino acid into the polyketide skeleton in macrolactam biosynthesis. Therefore, mechanistic insight into this family of enzymes has been investigated to uncover its substrate specificity and thus aid future swapping and engineering of enzymes.
Recently, the PKS genes responsible for the formation of fluvirucin B1 aglycone were identified and characterized.Citation19) However, key β-amino acid-carrying enzymes including the VinN-type adenylation enzyme have not been identified in the reported fluvirucin B1 biosynthetic gene cluster. The mechanism of the construction of the β-alanine moiety in fluvirucin thus remains elusive. Although the previous feeding experiment with a fluvirucin producer strain showed that the β-alanine moiety of fluvirucin originates from l-aspartate presumably via decarboxylation of its α-carboxyl group,Citation20) the timing of decarboxylation of the α-carboxyl group is still unclear. One possibility is that l-aspartate is loaded to a standalone ACP by an adenylation enzyme before the decarboxylation reaction, as reported in the vicenistatin biosynthetic pathway. Alternatively, l-aspartate is first decarboxylated and the resulting β-alanine is loaded to a standalone ACP by an adenylation enzyme. In this study, we identified the whole biosynthetic cluster of fluvirucin B2 produced from Actinomadura fulva subsp. indica ATCC 53714 and functionally characterized the β-amino acid specific adenylation enzyme. This study expands our knowledge of the β-amino acid biosynthetic machinery and the selective incorporation mechanism in macrolactam biosynthesis.
Materials and methods
Bacterial strain and growth conditions
A. fulva subsp. indica ATCC 53714 was used as the fluvirucin B2 producer strain and source of the fluvirucin B2 biosynthetic (flv) genes. A. fulva subsp. indica ATCC 53714 was maintained on ISP2 agar medium (0.4% yeast extract, 1.0% malt extract, 0.4% glucose, and 2.0% agar) at 28 °C, and the spores were used for a seed culture in the same medium without agar. Escherichia coli DH5α was routinely used for plasmid preparation. E. coli BL21(DE3) was used for the expression of Flv proteins.
Genome sequence analysis
Genome DNA sequencing was performed on a PacBio RS II (Pacific Biosciences, Menlo Park, CA, USA). The obtained sequence data were assembled using HGAP2 (Pacific Biosciences). Automated gene annotation was performed with the MiGAP server (http://www.migap.org/index.php/en), and the annotated open frames were revised by FramePlot analysis (http://www0.nih.go.jp/~jun/cgi-bin/frameplot.pl). The DNA sequence including the putative fluvirucin B2 biosynthetic gene cluster was deposited in the DDBJ under the accession number LC095592.
Preparation of recombinant proteins
flvN was amplified from chromosomal DNA with the primers 5′-CCATATGCGTCCCGGCTGCCGGAG-3′ and 5′-GGAATTCTCACGGGCTCTCGTCGAG-3′. Similarly, flvL was amplified from chromosomal DNA with the primers 5′-ACATATGTGGGATAATCGTTTCGAAG-3′ and 5′-AGGATCCTCAACCGTCCGTCTCGG-3′. These PCR fragments were ligated into the vector pMD19 (Takara Biochemicals, Ohtsu, Japan). After sequence confirmation, flvN and flvL were excised from these plasmids and inserted between NdeI and EcoRI sites of the expression vector pCold I (Takara Biochemicals) and between NdeI and BamHI sites of the expression vector pET28 (Takara Biochemicals), respectively. For expression of the FlvN protein, E. coli BL21(DE3) cells harboring the pCold I-flvN were grown at 37 °C in Luria Bertani (LB) broth containing ampicillin (50 μg/mL). After the optical density at 600 nm reached 0.6, protein expression was induced by the addition of isopropyl β-d-1-thiogalactopyranoside to a final concentration of 0.2 mM. The cells were then cultured for an additional 18 h at 15 °C. For expression of the FlvL protein, the FlvL protein was co-expressed with the sfp gene to obtain holo-ACP, as described previously.Citation11) E. coli BL21(DE3) cells harboring the pET28-flvL and pET21-sfp were grown at 28 °C in LB broth containing kanamycin (50 μg/mL) and ampicillin (50 μg/mL). After the optical density at 600 nm reached 0.6, protein expression was induced by the addition of isopropyl β-d-1-thiogalactopyranoside to a final concentration of 0.2 mM and the cells were cultured for an additional 18 h at 28 °C. The recombinant protein, which was collected from cell-free extracts prepared by sonication, was purified on a His60 Ni Superflow affinity column (Clontech, Mountain View, CA, USA). The protein was then desalted using a PD-10 column (GE Healthcare, Buckinghamshire, UK).
Enzymatic assays and determination of kinetic parameters
A continuous spectroscopic assay that measures the release of inorganic pyrophosphate with the adenylating reaction was carried out according to the Webb’s method.Citation21) The assay solution (150 μL total volume) contained 20 mM HEPES-Na buffer (pH 7.8), 10% glycerol, 1 mM MgCl2, 1 mM ATP, 0.1 mM 2-amino-6-mercapto-7-methylpurine ribonucleoside (MESG), 1 U/mL inorganic pyrophosphatase, 1 U/mL purine nucleotide phosphorylase, 50 μM FlvL, and 0.5 mM of either l-aspartate, d-aspartate, β-alanine, dl-threo-3-MeAsp, or l-glutamate. For the kinetic assay, the l-aspartate concentration was varied between 0.05 and 5 mM. The reaction was initiated by the addition of FlvN (2 μM) to the mixture and incubated at 25 °C. The increase in absorbance at 360 nm, attributable to the formation of 2-amino-6-mercapto-7-methylpurine, per second was monitored using a UV-2450 spectrophotometer (Shimadzu, Tokyo, Japan). The initial velocity was determined from the linear portion of the optical density profile (ε360 nm = 11,000 M−1cm−1). Steady-state parameters were determined by the Michaelis–Menten equation.
Results and discussion
Identification of the gene cluster
We used a genome-scanning technique to identify the fluvirucin B2 biosynthetic gene cluster in the producer strain A. fluva ATCC 53714. Genome sequence analysis revealed a contiguous biosynthetic gene cluster containing genes for four characteristic β-amino acid-forming enzymes, one amidohydrolase and three PKSs (Table and Supplemental Fig. S1). No other candidate gene cluster with these features was found in the genome sequence.
Table 1. Fluvirucin B2 biosynthetic genes from Actinomadula fulva ATCC 53714 and putative functions.
The identified β-amino acid-carrying enzymes such as FlvN, FlvL, FlvM, and FlvK and the aminohydrolase FlvJ share high sequence identity with the vicenistatin biosynthetic enzymes (Table ). Notably, a putative adenylation enzyme FlvN, which appears to be a key enzyme for the selective incorporation of a β-amino acid substrate, has 63% sequence identity with VinN. In addition, the identified gene cluster encodes a putative PLP-dependent decarboxylase FlvO, which is highly homologous (identity 61%) to VinO. Judging from the high sequence identities of these starter-related enzymes with Vin-type enzymes, fluvirucin B2 is likely to be biosynthesized through a vicenistatin-like strategy that involves l-aspartate being loaded to a standalone ACP FlvL by FlvN before the FlvO reaction.
The presence of three PKS genes, flvP1, flvP2, and flvP3, in the gene cluster is consistent with that of fluA, fluB, and fluC in the biosynthetic gene cluster of fluvirucin B1.Citation19) The module 1 of FlvP1, the modules 3 and 4 of FlvP2, and the module 5 of FlvP3 have a full set of PKS domains consisting β-ketosynthase (KS), acyltransferase (AT), dehydratase (DH), β-ketoreductase (KR), and enoylreductase (ER) domains, indicating that these PKS modules are responsible for constructing the fully reduced polyketide chain. The module 2 of FlvP1 would be responsible for constructing the C9–C10 moiety with a hydroxy group, because this module does not contain the DH domain. The terminal thioesterase (TE) domain in FlvP3 is responsible for the macrocyclization. The prediction of the substrate specificity of the AT domains agrees well with the fluvirucin B2 aglycon structure (Supplemental Fig. S2).
In addition, putative ethylmalonyl-CoA biosynthetic enzymesCitation22,23) are encoded in the gene cluster. A putative β-ketoacyl synthase III type enzyme FlvE2 may be responsible for the formation of acetoacetyl-CoA from acetyl-CoA and malonyl-CoA (Supplemental Fig. S3A), and function as the initiation of fatty acid biosynthesis. Then, a putative 3-hydroxybutyryl-CoA dehydrogenase FlvE3 or short-chain dehydrogenase FlvE4 could be responsible for the formation of crotonyl-CoA. Dehydratase gene is not found in the flv gene cluster as observed in the indanomycin biosynthetic gene cluster.Citation23) Dehydratase encoded outside of the gene cluster might be involved in the dehydration reaction for the formation of crotonyl-CoA. A putative crotonyl-CoA decarboxylase FlvE5 could function by attaching the carboxylate group to crotonyl-CoA to afford ethylmalonyl-CoA. Alternatively, a putative propionyl-CoA carboxylase β-subunit FlvE1 might cooperatively be involved in ethylmalonyl-CoA formation.
Based on the comparison with the dTDP-l-rhamnose biosynthetic pathway,Citation24,25) putative NDP-deoxyaminosugar biosynthetic genes were also identified in the gene cluster. dTDP-glucose synthase FlvS1, dTDP-glucose-4,6-dehydratase FlvS2, dTDP-sugar 3,5-epimerase FlvS3, and dTDP-sugar aminotransferase FlvS4 could be responsible for the preparation of dTDP-l-mycosamine (Supplemental Fig. S3B). A gene responsible for 3,4-ketoisomerization is not contained in the gene cluster. It was reported that the polyene antibiotic biosynthetic gene clusters also lack a 3,4-isomerase gene responsible for the GDP-d-mycosamine synthesis.Citation26) This isomerization reaction could occur nonenzymatically. A putative glycosyltransferse FlvS5 would catalyze the glycosylation of the aglycon of fluvirucin B2 with dTDP-l-mycosamine. Putative transcriptional regulators and transporters were also identified. Thus, the identified gene cluster appears to be responsible for fluvirucin B2 biosynthesis.
Substrate specificity of the adenylation enzyme FlvN
The substrate specificity of the β-amino acid selective adenylation enzyme is a key determinant of the starter moiety of the macrolactam polyketide. Since our genome sequence analysis suggested that FlvN is the β-amino acid selective adenylation enzyme in the fluvirucin B2 biosynthetic pathway, we expressed a recombinant FlvN protein in E. coli (Supplemental Fig. S4) and examined its adenylating activity in vitro. We first attempted to analyze the activity by the previously described coupling assay with inorganic pyrophosphatase, purine nucleoside phosphorylase, and MESG.Citation21) However, we were unable to detect significant release of inorganic pyrophosphate, which is concomitant with the formation of an acyl adenylate intermediate. It has been reported that the activity of nonribosomal peptide synthetase-independent siderophore (NIS) synthetases fails to be detected by the normal ATP–pyrophosphate exchange assay.Citation27,28) In this case, the enzyme is unable to release pyrophosphate from the active site after formation of the acyl adenylate intermediate because the intermediate remains bound to the active site. Because we postulate that FlvN may also be unable to release the pyrophosphate from the active site, we added the standalone ACP FlvL into the FlvN reaction for the decomposition of the acyl adenylate intermediate. As a result, we succeeded in detecting FlvN activity against l-aspartate (Fig. ). The kcat and Km values for l-aspartate at 25 °C were determined to be 6.8 ± 0.2 min−1 and 0.15 ± 0.01 mM, respectively, which are comparable with those of the known adenylation enzymes.Citation29–34) In contrast, FlvN showed very weak or no activity toward other amino acids including d-aspartate, β-alanine, dl-threo-3-MeAsp, and l-glutamate (Fig. ). Thus, FlvN exhibits different substrate specificity when compared with VinN, which has a clear preference for (2S,3S)-3-MeAsp over l-aspartate,Citation34) despite their high sequence identity (63%).
Fig. 2. Amino acid selectivity of FlvN. FlvN was reacted with 0.5 mM amino acid. The bar diagram represents the relative adenylation activities. The specific activity for l-aspartate (5.4 min−1) is defined as 100% relative activity. Error bars represent SEM (n = 3).
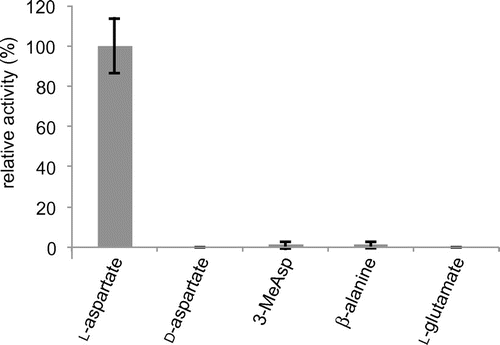
Because the crystal structure of VinN complexed with (2S,3S)-3-MeAsp has already been determined,Citation34) we compared the amino acid sequence between FlvN and VinN to gain insight into the substrate recognition mechanism of FlvN. The sequence alignment analysis revealed that FlvN shares most of the residues in the substrate-binding pocket with VinN (Supplemental Fig. S5). FlvN has a conserved Asp230 residue for recognition of the amino group of the substrate. In addition, FlvN has a set of basic residues, Lys330 and Arg331, which are equivalent to Lys330 and Arg331 of VinN for recognition of the C1 carboxyl group of (2S,3S)-3-MeAsp (Supplemental Fig. S6). This suggests that l-aspartate is the preferred substrate for FlvN due to the interaction of its α-carboxyl group with Lys330 and Arg331 of FlvN. In contrast, Phe231 and Ser299 of VinN, which are located close to the methyl group of (2S,3S)-3-MeAsp in the substrate-binding pocket of VinN, are replaced by Tyr231 and Thr299 in FlvN, respectively. These two bulkier residues might cause steric hindrance to the methyl group of 3-MeAsp in the substrate-binding pocket of FlvN. Thus, the findings from sequence comparison are consistent with the kinetic result that FlvN selectively recognizes l-aspartate as a substrate.
As mentioned above, we needed to add the ACP FlvL to detect FlvN activity. Unlike FlvN, we were able to detect VinN activity even in the absence of the ACP VinL. This ACP dependency and independency might be explained by their slight structural differences, which would affect solvent accessibility in the active site. VinN might allow a water molecule accessible to the acyl adenylate intermediate in the active site, resulting in the degradation of intermediate. In contrast, FlvN might not have such a water molecule in the active site.
Proposed fluvirucin B2 biosynthetic pathway
Given the clarified substrate specificity that FlvN strongly prefers l-aspartate over β-alanine, we propose the following biosynthetic pathway for fluvirucin B2 (Fig. ). At first, the adenylation enzyme FlvN selectively recognizes l-aspartate and ligates it with the standalone ACP FlvL to generate l-aspartyl-FlvL. The PLP-dependent enzyme FlvO then catalyzes the decarboxylation to give β-alanyl-FlvL. Another adenylation enzyme FlvM catalyzes the aminoacylation with relatively small amino acids such as glycine and alanine to form dipeptidyl-FlvL. The acyltransferase FlvK transfers the dipeptide group from FlvL to the loading ACP domain of the initial PKS FlvP1. Following polyketide chain elongation by the reaction of FlvP1, FlvP2, and FlvP3, the amidohydrolase FlvJ removes the terminal amino acyl group from the polyketide chain. The TE domain of FlvP3 then catalyzes macrocyclization to form the aglycon of fluvirucin B2. Finally, the glycosyltransferse FlvS5 glycosylates the aglycon with dTDP-l-mycosamine to complete the biosynthesis.
In summary, we have identified the biosynthetic cluster of fluvirucin B2 and functionally characterized the l-aspartate selective adenylation enzyme FlvN. Although FlvN has high sequence identity to VinN, it shows different substrate specificity. Thus, our study provides additional information about substrate specificity of β-amino acid selective adenylation enzymes.
Author contributions
A. Miyanaga, F. Kudo, and T. Eguchi designed the research plan with assistance from K. Shin-ya. A. Miyanaga, Y. Hayakawa, M. Numakura, J. Hashimoto, K. Teruya, and T. Hirano performed experiment and analyzed data. A. Miyanaga, F. Kudo, and T. Eguchi wrote the manuscript. All authors reviewed and approved the final manuscript.
Supplemental material
The supplementary material for this paper is available at http://dx.doi.org/10.1080/09168451.2015.1132155.
SI_1202.doc
Download MS Word (1.1 MB)Disclosure statement
No potential conflict of interest was reported by the authors.
Funding
This work was supported in part by Grants-in-Aid for Young Scientists (B) from the Japan Society for the Promotion of Science [grant number 25850050] (to A.M.), Grants-in-Aid for Scientific Research in Innovative Areas from the Ministry of Education, Culture, Sports, Science and Technology [grant number 22108003] (to T.E.), Nagase Science and Technology Foundation (to F.K.), and Takeda Science Foundation (to F.K.).
Notes
Abbreviations: 3-MeAsp, 3-methylaspartate; ACP, acyl carrier protein; PKS, polyketide synthase; MESG, 2-amino-6-mercapto-7-methylpurine ribonucleoside; dTDP, thymidine diphosphate.
References
- Hegde VR, Patel MG, Gullo VP, et al. Macrolactams: a new class of antifungal agents. J. Am. Chem. Soc. 1990;112:6403–6405.10.1021/ja00173a042
- Hegde VR, Patel MG, Gullo VP, et al. Sch 38518 and Sch 39185: two novel macrolactam antifungals. J. Chem. Soc., Chem. Commun. 1991;12:810–812.10.1039/c39910000810
- Naruse N, Tenmyo O, Kawano K, et al. Fluvirucins A1, A2, B1, B2, B3, B4 and B5, new antibiotics active against influenza a virus. I. production, isolation, chemical properties and biological activities. J. Antibiot. 1991;44:733–740.10.7164/antibiotics.44.733
- Naruse N, Tsuno T, Sawada Y, et al. Fluvirucins A1, A2, B1, B2, B3, B4 and B5, new antibiotics active against influenza A virus II. structure determination. J. Antibiot. 1991;44:741–755.
- Naruse N, Konishi M, Oki T. Fluvirucins A1, A2, B1, B2, B3, B4 and B5, new antibiotics active against influenza a virus. III. The stereochemistry and absolute configuration of fluvirucin A1. J. Antibiot. 1991;44:756–761.10.7164/antibiotics.44.756
- Ui H, Imoto M, Umezawa K. Inhibition of phosphatidylinositol-specific phospholipase C activity by Fluvirucin B2. J. Antibiot. 1995;48:387–390.10.7164/antibiotics.48.387
- Xu Z, Johannes CW, Houri AF, et al. Applications of Zr-catalyzed carbomagnesation and Mo-catalyzed macrocyclic ring closing metathesis in asymmetric synthesis, enantioselective total synthesis of Sch 38516 (fluvirucin B1). J. Am. Chem. Soc. 1997;119:10302–10316.10.1021/ja972191k
- Llàcer E, Urpí F, Vilarrasa J. Efficient approach to fluvirucins B2–B5, Sch 38518, and Sch 39185. first synthesis of their aglycon, via CM and RCM reactions. Org. Lett. 2009;11:3198–3201.10.1021/ol901030f
- Nebot J, Pedro Romea P, Urpí F. Stereoselective synthesis of protected 3-amino-3,6-dideoxyaminosugars. Org. Biomol. Chem. 2012;10:6395–6403.10.1039/c2ob25793a
- Ogasawara Y, Katayama K, Minami A, et al. Cloning, sequencing, and functional analysis of the biosynthetic gene cluster of macrolactam antibiotic vicenistatin in Streptomyces halstedii. Chem. Biol. 2004;11:79–86.
- Shinohara Y, Kudo F, Eguchi T. A natural protecting group strategy to carry an amino acid starter. J. Am. Chem. Soc. 2011;133:18134–18137.10.1021/ja208927r
- Kudo F, Miyanaga A, Eguchi T. Biosynthesis of natural products containing β-amino acids. Nat. Prod. Rep. 2014;31:1056–1073.10.1039/C4NP00007B
- Takaishi M, Kudo F, Eguchi T. Identification of incednine biosynthetic gene cluster: characterization of novel β-glutamate-β-decarboxylase IdnL3. J. Antibiot. 2013;66:691–699.10.1038/ja.2013.76
- Amagai K, Takaku R, Kudo F, et al. A unique amino transfer mechanism for constructing the β-amino fatty acid starter unit in the biosynthesis of the macrolactam antibiotic cremimycin. ChemBioChem. 2013;14:1998–2006.10.1002/cbic.v14.15
- Jørgensen H, Degnes KF, Sletta H, et al. Biosynthesis of macrolactam BE-14106 involves two distinct PKS systems and amino acid processing enzymes for generation of the aminoacyl starter unit. Chem. Biol. 2009;16:1109–1121.10.1016/j.chembiol.2009.09.014
- Udwary DW, Zeigler L, Asolkar RN, et al. Genome sequencing reveals complex secondary metabolome in the marine actinomycete Salinispora tropica. Proc. Natl. Acad. Sci. USA. 2007;104:10376–10381.10.1073/pnas.0700962104
- Kudo F, Kawamura K, Uchino A, et al. Genome mining of the hitachimycin biosynthetic gene cluster: involvement of a phenylalanine-2,3-aminomutase in biosynthesis. ChemBioChem. 2015;16:909–914.10.1002/cbic.201500040
- Zhu Y, Zhang W, Chen Y, et al. Characterization of heronamides biosynthesis reveals a tailoring hydroxylase and indicates migrated double bonds. ChemBioChem. 2015;16:2086–2093.10.1002/cbic.201500281
- Lin TY, Borketey LS, Prasad G, et al. Sequence, cloning, and analysis of the fluvirucin B1 polyketide synthase from Actinomadura vulgaris. ACS Synth. Biol. 2013;2:635–642.10.1021/sb4000355
- Puar MS, Gullo V, Gunnarsson I, et al. Biosynthesis of macrolactam antifungal agents. Bioorg. Med. Chem. Lett. 1992;2:575–578.10.1016/S0960-894X(01)81200-2
- Webb MR. A continuous spectrophotometric assay for inorganic phosphate and for measuring phosphate release kinetics in biological systems. Proc. Natl. Acad. Sci. USA. 1992;89:4884–4887.10.1073/pnas.89.11.4884
- Erb TJ, Berg IA, Brecht V, et al. Synthesis of C5-dicarboxylic acids from C2-units involving crotonyl-CoA carboxylase/reductase: the ethylmalonyl-CoA pathway. Proc. Natl. Acad. Sci. USA. 2007;104:10631–10636.10.1073/pnas.0702791104
- Li C, Roege KE, Kelly WL. Analysis of the indanomycin biosynthetic gene cluster from Streptomyces antibioticus NRRL 8167. ChemBioChem. 2009;10:1064–1072.10.1002/cbic.v10:6
- Jiang XM, Neal B, Santiago F, et al. Structure and sequence of the rfb (O antigen) gene cluster of Salmonella serovar typhimurium (strain LT2). Mol. Microbiol. 1991;5:695–713.10.1111/mmi.1991.5.issue-3
- Graninger M, Nidetzky B, Heinrichs DE, et al. Characterization of dTDP-4-dehydrorhamnose 3,5-epimerase and dTDP-4-dehydrorhamnose reductase, required for dTDP-L-rhamnose biosynthesis in Salmonella enterica serovar Typhimurium LT2. J. Biol. Chem. 1999;274:25069–25077.10.1074/jbc.274.35.25069
- Aparicio JF, Caffrey P, Gil JA, et al. Polyene antibiotic biosynthesis gene clusters. Appl. Microbiol. Biotechnol. 2003;61:179–188.10.1007/s00253-002-1183-5
- Schmelz S, Kadi N, McMahon SA, et al. AcsD catalyzes enantioselective citrate desymmetrization in siderophore biosynthesis. Nat. Chem. Biol. 2009;5:174–182.10.1038/nchembio.145
- Kadi N, Challis GL. Chapter 17, Siderophore biosynthesis a substrate specificity assay for nonribosomal peptide synthetase-independent siderophore synthetases involving trapping of acyl-adenylate intermediates with hydroxylamine. Methods Enzymol. 2009;458:431–457.10.1016/S0076-6879(09)04817-4
- Mootz HD, Marahiel MA. The tyrocidine biosynthesis operon of Bacillus brevis: complete nucleotide sequence and biochemical characterization of functional internal adenylation domains. J. Bacteriol. 1997;179:6843–6850.
- Van Lanen SG, Lin S, Dorrestein PC, et al. Substrate specificity of the adenylation enzyme SgcC1 involved in the biosynthesis of the enediyne antitumor antibiotic C-1027. J. Biol. Chem. 2006;281:29633–29640.10.1074/jbc.M605887200
- Rachid S, Krug D, Weissman kJ, et al. Biosynthesis of (R)-β-tyrosine and its incorporation into the highly cytotoxic chondramides produced by Chondromyces crocatus. J. Biol. Chem. 2007;282:21810–21817.10.1074/jbc.M703439200
- Du L, He Y, Luo Y. Crystal structure and enantiomer selection by d-alanyl carrier protein ligase DltA from Bacillus cereus. Biochemistry. 2008;47:11473–11480.10.1021/bi801363b
- Villiers BR, Hollfelder F. Mapping the limits of substrate specificity of the adenylation domain of TycA. ChemBioChem. 2009;10:671–682.10.1002/cbic.200800553
- Miyanaga A, Cieślak J, Shinohara Y, et al. The crystal structure of adenylation enzyme VinN reveals a unique β-amino acid recognition mechanism. J. Biol. Chem. 2014;289:31448–31457.10.1074/jbc.M114.602326