Abstract
Conditions for the optimal production of polyhydroxyalkanoate (PHA) by Pseudomonas mendocina PSU using a biodiesel liquid waste (BLW) were determined by response surface methodology. These were an initial carbon to nitrogen ratio (C/N) of 40 (mole/mole), an initial pH of 7.0, and a temperature of 35 °C. A biomass and PHA concentration of 3.65 g/L and about 2.6 g/L (77% DCW), respectively, were achieved in a growth associated process using 20 g/L glycerol in the BLW after 36 h of exponential growth. The PHA monomer compositions were 3HB (3-hydroxybutyrate), a short-chain-length-PHA, and the medium-chain-length-PHA e.g. 3-hydroxyoctanoate and 3-hydroxydecanoate. Both the phbC and phaC genes were characterized. The phbC enzyme had not been previously detected in a Pseudomonas mendocina species. A 2.15 g/L of an exopolysaccharide, alginate, was also produced with a similar composition to that of other Pseudomonas species.
Graphical abstract
PHA biosynthesis from biodiesel liquid waste (BLW) by Pseudomonas mendocina PSU.
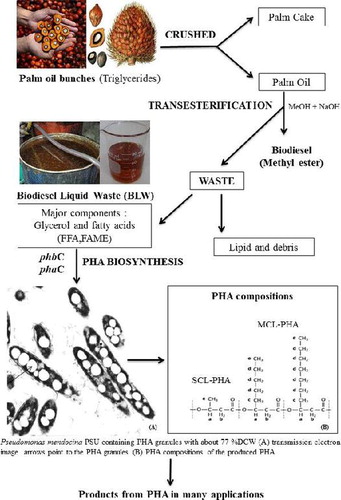
Polyhydroxyalkanoates (PHAs) are a family of aliphatic biopolyesters produced by many bacterial species as intracellular storage compounds for carbon and energy normally when grown with nutrient-limited conditions such as a limitation by nitrogen, phosphorous, oxygen, or magnesium but in the presence of an excess carbon source.Citation1,Citation2 Approximately 150 different PHA subunits have now been identified.Citation3,Citation4 PHA can be divided into two general groups: short-chain-length (SCL), with monomeric subunits of 3–5 carbons in length, and medium-chain-length (MCL) PHA consisting of monomers of 6–14 carbons in length. These differences in PHA monomer composition can dramatically affect the properties and qualities of the polymers.Citation3−6 Although most PHA-synthesizing bacteria accumulate either only short-chain-length-PHA (SCL-PHA) or medium-chain-length-PHA (MCL-PHA), both accumulation of SCL-PHA and MCL-PHA have been reported in some bacterial strains e.g. Pseudomonas sp. DSY-82, Pseudomonas stutzeri A1501, Pseudomonas sp. 61-3, and Pseudomonas sp. A33.Citation2,7 Due to their ease of biodegradation, biocompatibility and thermoprocessibility, PHA has found many uses such as for packaging materials, biomedical implant materials, drug delivery carriers, and biofuels.Citation8 However, the main obstacle for using PHA as a commercial product is its high production cost when compared with other conventional plastics so its use is limited to special circumstances. The major cost in PHA production is associated with the carbon source that can account for more than 50% of the overall production cost.Citation9 There is therefore considerable interest in identifying a readily available, cheap, and renewable carbon source that can be efficiently converted into PHA.
Biodiesel is now recognized as being an alternative fuel to petroleum diesel. It is produced from renewable sources such as animal fats and vegetable oils, but this process generates about 10% (w/w) crude glycerol as the main waste byproduct.Citation10,Citation11 These generated biodiesel liquid waste (BLW) can become an environmental problem. One possible application for the excess BLW would be to use it as a carbon and energy source for supporting microbial growth that in turn would produce some value-added products such as PHA.Citation10−13 This approach could reduce the overall costs to make PHA economically competitive and at the same time would minimize any waste disposal problems. Several studies have shown that SCL-PHA and MCL-PHA can be produced from BLW by various Pseudomonas species including Pseudomonas oleovorans and Pseudomonas corrugata,Citation10 Pseudomonas mediterranea,Citation14, and Pseudomonas putida.Citation13,15 However, under unbalanced growth conditions such as a situation of nitrogen limitation but a sufficiency of carbon, apart from the intracellular accumulation of PHA, some bacteria especially some Pseudomonads also produce alginate as a commercially useful exopolysaccharide (EPS) and this can restrict the use of the available carbon substrate for PHA.Citation16,Citation17 Alginate is an EPS that consists of variable amounts of β-d-mannuronic acid and a C5-epimer α-l-guluronic acid linked via β-1,4-glycosidic bonds. Bacterial alginates are synthesized by only two bacterial genera e.g. Pseudomonas sp. and Azotobacter sp. However, at present commercial alginate is currently extracted from marine algae such as Laminaria and Macrocystis. Alginates produced by bacteria have the same physiochemical characteristics to those produced by algae but the alginate produced from bacteria is acetylated at the hydroxyl groups at position C2 and/or C3 of the mannuronic acid residue. The alginate biosynthesis pathway in Pseudomonas species has been well characterized.Citation16−18
Recently, we isolated a new strain of Pseudomonas mendocina PSU that accumulated PHA at up to 77% of its dry cell weight (DCW) within a 36-h growth period from BLW as the sole carbon source; however, it also produced alginate. In order to maximize PHA synthesis, we have optimized the culture conditions in shake flask experiments using response surface methodology (RSM) statistical analysisCitation19 to obtain a high PHA accumulation and biomass concentration. In addition, the PHA synthase genes of P. mendocina PSU have been amplified and investigated. The composition of the PHA was determined by gas chromatography–mass spectrometry (GC–MS) and 1H-nuclear magnetic resonance (NMR). This is perhaps the first report to study the use of BLW from biodiesel production for PHA production and to efficiently produce SCL-PHA from a P. mendocina species.
Materials and methods
Identification and characterization of the isolate
The PHA-producing bacteria were isolated from a biodiesel-contaminated sediment at Biodiesel Ethyl Ester Pilot Plant, the Specialized R & D Center for Alternative Energy from Palm Oil and Oil Crops, Faculty of Engineering, Prince of Songkla University, Thailand according to standard isolation methods.Citation20 Identification was achieved based on biochemical characteristics (VITEK®2 compact, bioMérieux S.A., France) and full-length 16S rDNA analysis. Amplification of the 16S rRNA gene by PCR used the universal primers: 20F (5′-GAG TTT GAT CCT GGC TCA G-3′) and 1500R (5′-GTT ACC TTG TTA CGA CTT-3′) and the DNA Engine Dyad® Thermal Cycler (Bio-Rad Laboratories). Conditions for the PCR were: 94 °C for 3 min, 25 cycles of denaturation at 94 °C for 1 min, annealing at 50 °C for 1 min and elongation at 72 °C for 2 min, followed by a final amplification step at 72 °C for 3 min and then products were purified using the QIAquick® PCR purification kit (QIAGEN, Germany). The 16S rRNA gene sequence was then determined using the ABI Prism® 3730XL DNA sequencer (Applied Biosystems Co., USA). Sequences were compared using the GenBank database and the nucleotide–nucleotide BLAST command at the National Centre for Biotechnology Information (NCBI). Comparisons of the DNA sequences were made by the CLUSTAL X (version 1.8) in the BioEdit Program. A phylogenetic tree of the 16S rRNA genes was constructed by the neighbor joining method of Saitou and Nei.Citation21 The robustness for individual branches was evaluated by 1000 replication bootstrapping with the MEGA 5.0 program.
PHA biosynthesis from BLW
A BLW, obtained from Biodiesel Ethyl Ester Pilot Plant at Prince of Songkla University, was used as the sole carbon source for the PHA production. The BLW composition was analyzed by a CHNS-O analyzer (see analytical procedures). This BLW was a suitable carbon substrate for PHA biosynthesis because of its high carbon and glycerol content and low nitrogen content. The initial carbon to nitrogen ratio (C/N) was roughly 800–850 (see Table S1).
The inoculum for each experiment was prepared using 50 mL of nutrient rich (NR) mediumCitation22 in a 250 mL Erlenmeyer flask. The medium composition in g/L was: peptone 10, meat extract 10, and yeast extract 2 adjusted to an initial pH of 7.0. The culture was incubated in a rotary incubator shaker at 200 rpm and 35 °C until mid-log phase was reached and then a 4.0% (v/v) inoculum suspension (OD600 = 0.5) was added to the experimental medium a nitrogen-limiting mineral salts medium (MSM) containing in g/L: total glycerol in the BLW, 20 as the sole carbon source; (NH4)2SO4, 1.0; KH2PO4, 2.0; Na2HPO4, 0.6; MgSO4·7H2O, 1.0; and a trace element solution 1 mL containing in g/L: CaCl2·2H2O, 20; ZnSO4·7H2O, 1.30; FeSO4·7H2O, 2.0; (NH4)2MoO4, 0.6; and boric acid, 0.6.Citation23 Aerobic conditions were maintained by shaking as described above and samples were taken every 12 h until 72 h of cultivation. For each sample, the cell mass, the PHA content and its composition, and substrate utilization from the cell free liquid culture were analyzed (see analytical procedures).
Effect of glycerol concentration in BLW on cell growth and PHA production
The effect of different amounts of BLW on cell growth and PHA production by Pseudomonas sp. PSU was investigated by growth in MSM production medium supplemented with various concentrations of total glycerol in the BLW e.g. 5, 10, 20, 30, 40, and 50 g/L of glycerol. The initial pH of the culture medium was adjusted to 7.0 and incubated under the same conditions as described above.
Effect of different nitrogen sources and their concentration on cell growth and PHA production
Different nitrogen sources e.g. (NH4)2SO4, NH4NO3, and (NH2)2CO (urea) in the MSM containing 20 g/L of total glycerol in the BLW as the carbon source were tested for their effects on the biomass and PHA accumulation. The amount of each nitrogen compound added to provide an equimolar nitrogen content. The amount of the best nitrogen source was then adjusted to find the optimal concentration and the suitable C/N for PHA production during the RSM experiment as described below.
Optimization of cell growth and PHA production using RSM
Optimization of cell growth and PHA production was based on the single-factor test, three factors e.g. factor (A): ratio of carbon to nitrogen (mole/mole) was performed by fixing the carbon (total glycerol in the BLW) concentration at 20 g/L while varying the nitrogen concentration, factor (B): initial pH, and factor (C): incubation temperature (°C) was all selected to optimize the production conditions to achieve the maximum cell growth and PHA content. The selected factors were subjected to RSM with a three-factor three-coded levels Box–Behnken design (BBD). The range and the levels of the experimental variables that were investigated in this study are presented in Table S2. In addition, several verification experiments were carried out according to the RSM determined optimal conditions.
PCR analysis of the SCL-PHA synthase and MCL-PHA synthase genes from the isolate
The phbC gene encoding for SCL-PHA synthase and phaC gene encoding for MCL-PHA synthase were detected by PCR amplification using two sets of primers. These primers were designed based on two highly conserved sequences deduced from multiple alignment analysis of the Pseudomonads phbC and phaC genes. For phbC amplification, phbC-F (5′-GCCTTCTACCTGCTGAATTCCG-3′) and phbC-R (5′-GGTGTGGCGCAGATACCA-3′) were usedCitation24 whereas I-179-L (5′-ACAGATCAACAAGTTCTACATCTTCGAC-3′) and I-179-R (5′-GGTGTTGTCGTTGTTCCAGTAGAGGATGTC-3′) were used for phaC amplification.Citation25,Citation26 The PCR parameters used were: 95 °C hold for 5 min, followed by 30 cycles of 1 min at 95 °C, 1 min at 50 °C, 1 min at 72 °C, and a final extension for 7 min at 72 °C. The nucleotide sequences of PHA synthase genes from Pseudomonas sp. PSU were compared with those similar genes in other PHA-accumulating bacteria by a BLAST search at NCBI then aligned with 20 representative classes of PHA synthase genes obtained from GenBank to generate a phylogenetic tree according to the method as previously described.
Production of EPS
According to an observations made during PHA production, it was interesting that in the cell-free culture medium there was obviously a high viscosity. It was thought that this could be due to some secondary metabolites e.g. an EPS that was being produced. Therefore, in this study, the supernatant was used to extract the EPS and its composition was determined using liquid chromatography–mass spectrometry (LC–MS) (see analytical procedures).
Analytical procedures.
Biomass concentration
The cells were harvested by centrifugation (7155×g, 15 min at 4 °C). The cell pellets were washed once with hexane to remove some residual oil and finally with distilled water, followed by lyophilization to obtain a constant cell weight. The biomass concentration was recorded as DCW in g/L.
Quantification of PHA by gas chromatography–flame ionization detector
Approximately 20 mg of lyophilized cells were added to a mixture of 2 mL of chloroform and 2 mL of acidified methanol (3.0% (v/v) H2SO4) then heated at 100 °C for 3.5 h. After cooling to room temperature, 2 mL of distilled water was added followed by vigorous shaking and centrifugation (1006×g for 10 min). The chloroform portion containing the PHA methyl esters was transferred to a vial for analysis by GC-FID using an HP-INNOWax 19091 N-133 GC column (30 m × 0.25 mm × 0.25 μm, Agilent Technologies, USA). PHA was determined by the method described by Sun et al.Citation27 Benzoic acid was used as the internal standard.
Identification of the monomer composition of PHA by GC–MS
The methanolyzed sample was injected into a TR-WaxMS GC column (30 m × 0.25 mm × 0.25 μm, Thermo Fisher Scientific Inc., USA). Helium was used as the carrier gas. The ion trap analyzer was operated in the electron ionization mode. Mass spectra were in the range from m/z 35 to 500. Standard monomers of methyl hydroxyalkanoates (Larodan, Sweden) were used as the reference for the peak retention times and the ionization mass determination (method used in this study was modified from Gumel et al.Citation28 and Shahid et al.Citation29
PHA extraction
PHA was extracted by stirring approximately 1.0 g of lyophilized cells in 100 mL of chloroform at room temperature (26 °C) for 5 days. After filtration, the chloroform extract was concentrated and the dissolved PHA was purified by precipitation in chilled methanol. The purified PHA was then dried at room temperature for 24 h to remove residual solvent.Citation22
1H NMR analysis
A Fourier Transform NMR spectrometer (Unity Inova, Varian, Germany) was used to record the 1H NMR at 500 MHz and 25 °C against tetramethylsilane (TMS) as the internal reference standard. Approximately 5 mg of the extracted PHA sample was dissolved in 2 mL deuterated chloroform (CDCl3) for 1H NMR analysis. Chemical shifts were reported in ppm relative to the signal of the TMS.
Determination of organic carbon and nitrogen
Total organic carbon and nitrogen were determined based on the dynamic flash combustion method with an elemental analyzer (thermal scientific flash EA1112 CHNS-O analyzer, Thermo Quest, Italy). The sample was analyzed according to the procedures described by Gustafsson et al.Citation30
Glycerol utilization
The glycerol concentration was measured by the free glycerol determination method as described by Bondioli and Della Bella.Citation31
Extraction, identification and characterization of the produced EPS
EPS extraction
Cold 96% ethanol was added to the collected supernatant obtained by centrifugation (10,000×g, 4 °C, 30 min) at a 1:3 (v/v) supernatant:ethanol ratio to precipitate the crude EPS. The precipitated product was centrifuged and then lyophilized until it maintained a constant weight. The EPS obtained was quantified in g/L.Citation32
Liquid chromatography–mass spectrometry
Approximately 5.0 mg of crude EPS sample was dissolved in 2.3 mL of H2O in the presence of 1.7 mL H2SO4 at 100 °C for 140 min in order to obtain the corresponding monomers. Sodium alginate (Sigma–Aldrich, USA) was used as a standard. The LC–MS was used to elucidate the structure and monomer compositions of the produced EPS. The electrospray ionization (ESI) tandem mass spectrometry was carried out in the negative ion mode with the time-of-flight analysis by liquid chromatography–mass spectrometer, 2690 LCT (Waters/Micromass, UK). Nitrogen was used as the desolvation gas. The mass range was m/z 100–600 (method used in this study was modified from Chaki et al.Citation33
Statistical analysis
The data from the optimization of cell growth and PHA production were analyzed by Design Expert 6.0 software and the second-order polynomial equation (ANOVA of the quadratic regression model). The Duncan and Tukey’s multiple range tests were used to determine the difference among the groups. p-values of less than 0.05 were considered to be statistically significant.
Results and discussion
Bacterial identification
The DNA sequence of the 16S rRNA gene of Pseudomonas sp. PSU was aligned with the 16S rRNA gene of the similar genes in the NCBI database and the closest similarity was to P. mendocina S100E (99% identity) followed by Pseudomonas sp. USM 4–55 and P. mendocina NS2 as shown in the phylogenetic tree in Fig. . In addition, the biochemical test results also indicated that Pseudomonas sp. PSU had the highest similarity to P. mendocina (98% probability). The distinctive characteristics of P. mendocina compared to other Pseudomonas species were that it was unable to use either starch or maltose as a carbon source. P. mendocina is an aerobic gram-negative rod-shaped bacterium with a polar monotrichous flagellum. Colonies were flat, smooth, and butyrous with a brownish-yellow color due to the presence of carotenoid pigmentsCitation34 (see Table S3). The 16S rRNA gene sequence of the PSU isolate has been deposited at GenBank under the code name P. mendocina PSU (KC414580).
Fig. 1. Neighbour-joining phylogenetic tree constructed on the basis of the 16S rRNA gene sequence showing the phylogenetic relationships between Pseudomonas sp. PSU and its close relationship to other Pseudomonas species. The percentages of replicate trees in which the associated taxa were clustered together in the bootstrap test (1000 replicates) are shown next to the branches.
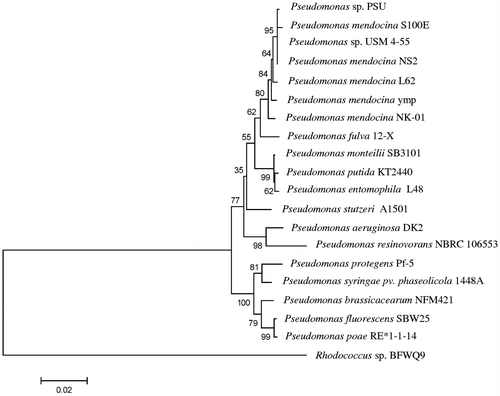
Effect of the initial glycerol concentration, free fatty acids, and fatty acid methyl esters in BLW on the bacterial growth and PHA production
A maximum DCW of 3.65 g/L with a PHA concentration of 2.59 g/L (approximately 77% DCW) and a PHA productivity of 0.072 g/L/h were produced by P. mendocina PSU when grown with 20 g/L of glycerol added as part of the BLW (Table ). Higher concentrations of glycerol e.g. from 30 to 50 g/L caused a significant reduction of the biomass and PHA production. Zhu et al. also reported that an increase in glycerol concentration in biodiesel–glycerol waste resulted in a decrease of microbial biomass in Burkhloderia cepacia ATCC 17759 by almost 50%. For example, the biomass reached 2.8 g/L at 3.0% (v/v) glycerol but when 9.0% (v/v) glycerol was added, the amount of biomass decreased to 1.3 g/L.Citation35 Palmeri et al. examined the effect of various glycerol concentrations in crude glycerol on P. mediterranea 9.1 and P. corrugata 388. Again the highest biomass and PHA concentration were obtained at 2.0% (v/v) glycerol in both strains, whereas any further increase in glycerol concentration up to 5.0% (v/v) reduced both cell growth and PHA accumulation.Citation14 A similar effect was also observed with Cupriavidus necator DSM 545, at glycerol concentrations that exceeded 3.0% (v/v), the specific growth rate decreased when using either pure glycerol or biodiesel–glycerol.Citation11 Ibrahim and Steinbüchel also reported that the biomass and P(3HB) accumulation in Zobellella denitrificans MW1 were inhibited at high concentrations of glycerol. The highest DCW and P(3HB) concentration decreased significantly from 5.04 and 4.27 g/L at 15 g/L of glycerol to only 2.72 and 1.52 g/L, respectively, when 50 g/L of glycerol was used.Citation36 These may due to the high glycerol concentrations used causing osmotic stress that modified cellular metabolism e.g. a decrease in enzyme efficiency and slower growth rate as indicated by the reduction of biomass that also decreased the PHA yield.Citation20 In addition, the effect of the concentration of pure glycerol on P. mendocina PSU was then investigated. For example, the DCW and PHA content in P. mendocina PSU decreased from 3.8 to 1.85 g/L and from 80.5 to 43.2%DCW, respectively, when the pure glycerol concentration was increased from 20 to 50 g/L. Kalaiyezhini and Ramachandran also showed a significant inhibitory effect on the accumulation of P(3HB) and growth in Paracoccus denitrificans DSMZ 413. When an initial pure glycerol concentration was increased from 30 to 40 g/L, the P(3HB) content and the specific rate of product formation rate (qp) were decreased from 50.7 wt% and 0.037 g/g cell/h to 48.6 wt% and 0.017 g/g cell/h, respectively.Citation37 Also with Alcaligenes eutrophus, an increase in the pure glycerol concentration from 10 to 20 g/L markedly decreased the P(3HB) content from 64 to 30 wt%.Citation38 This results indicated that when the same amount of glycerol was supplied, from either BLW or in a pure form, at a concentration of higher than 20 g/L the same inhibitory effect on both cell growth and PHA accumulation occurred. It was of interest that P. mendocina PSU used very little of the other carbon substrates present in BLW (Tables S4 and S5) e.g. some free fatty acids (FFA) and some residual fatty acid methyl esters (FAME) to support its growth and PHA production. The consumption of some of these alternative substrates was then determined. After 48 h of cultivation, when virtually all growth had ceased, only 30% of the FFA had been consumed whereas glycerol was almost completely depleted. Then, when six fatty acids e.g. butyric acid, octanoic acid, decanoic acid, lauric acid, palmitic acid, and oleic acid were supplemented into the MSM to observe PHA production, both the DCW and PHA content were very low compared with the use of pure glycerol. The highest cell growth and PHA production from fatty acids were obtained with oleic acid; however, the produced DCW and PHA content were only half of that produced with pure glycerol. This result clearly showed that P. mendocina PSU utilized mainly glycerol rather than any fatty acids as potential substrates for PHA production. In addition, according to the biochemical analysis (see Table S3), there was no lipase activity in P. mendocina PSU; therefore, any FFA and FAME were probably not readily utilized by this strain.
Table 1. Maximum cell growth, PHA production and PHA productivity produced from Pseudomonas mendocina PSU at various concentration of total glycerol in BLW.
Effect of NaCl in BLW on the bacterial growth and PHA production
Another possible reason for the inhibitory effect of higher concentrations of BLW on the growth and PHA production by most bacteria could be the presence of a high concentration of NaCl (3–5% (w/w)) from the alkaline-catalyzed trans-esterification process used to produce biodiesel. NaCl normally has a significant negative effect on microbial growth and PHA yield.Citation11,13,36 However, the BLW used in this study contained only 0.3% of sodium (data from ICP-OES analysis) and this had no inhibitory effect on the biomass and PHA content of P. mendocina PSU.
Effect of different nitrogen sources on the bacterial growth and PHA production
The best nitrogen source tested for the growth PHA production and PHA productivity by P. mendocina PSU was ammonium sulfate (Table ). The reason for ammonium sulfate being the preferred nitrogen substrate could be explained by a higher rate of ammonium uptake in the presence of the sulfate anion.Citation39 Other workers have also shown the importance of ammonium ions for growth that led to the accumulation of PHA.Citation15,40,41 Some bacterial strains e.g. Azohydromonas lata MTCC 2311 can efficiently utilize urea for P(3HB) productionCitation42 but P. mendocina PSU showed no urease activity (see Table S3). Although some growth and PHA were produced in a small amount when supplemented with urea, it was probably due to the presence of some other nitrogen source in the BLW.
Table 2. Maximum cell growth, PHA production and PHA productivity produced from Pseudomonas mendocina PSU from three different nitrogen sources.
Optimization of the bacterial growth and PHA production using RSM
Statistical analysis and model fitting of the optimization of bacterial growth and PHA production were performed using RSM. There were 17 experimental runs for optimizing three individual BBDs and the experimental conditions according to the factorial designs (see Table S6). The DCW ranged from 2.10 to 3.56 g/L and the PHA content was in the range from 18.59 to 76.30% of DCW whereas the carbon consumed was in the range from 2.83 to 9.60 g/L. The maximum DCW (3.56 g/L) and PHA content (76.30%DCW) as well as the carbon reduction (9.6 g/L) were found in the optimal conditions e.g. C/N = 40 (mole/mole), the initial pH 7.0, and the incubation temperature = 35 °C. The statistical significance of the regression model was checked by the F-test and the p-value. The analysis of variance (ANOVA) for the response surface quadratic model was determined by the coefficient (R2) to indicate that the model was highly significant and adequate for prediction within the range of the experimental variables.Citation19 In this study, the predicted R2 was in reasonable agreement with the adjusted R2 and the plotted data exhibited normal behavior (see Tables S7–S9 and Fig. S1).
To ensure that the predicted result was not biased toward the practical value, experimental re-checking was performed by comparing between the modified and the optimal conditions. The mean DCW of 3.58 ± 0.09 g/L and a mean PHA content of 77.27 ± 2.08% DCW occurred simultaneously with the highest utilization of carbon substrate of 9.60 ± 0.20 g/L (Table ). This indicated that the model was adequate for achieving the optimal cell growth and PHA production because the obtained data from the modified and the optimal conditions showed almost similar results. The time profile for cell growth, PHA production, and the substrate utilization by P. mendocina PSU grown with MSM supplemented with 20 g/L of total glycerol in the BLW are shown in Fig. . During the period of exponential growth, accumulation of PHA increased continuously and reached a maximum DCW of 3.65 g/L and a PHA concentration of 2.59 g/L (account for about 77% DCW of PHA content) at 36 h of cultivation simultaneously with the reduction in both the carbon and nitrogen supply. After 36 h, the accumulation of PHA and cell growth significantly decreased to 1.83 and 3.25 g/L, respectively, due presumably to the reduction of the preferred metabolizable carbon source and the complete consumption of the nitrogen source therefore cells started to degrade the storage PHA for cell maintenance purposes. It was of interest that the PHA accumulation increased continuously as the biomass increased. This indicated that PHA biosynthesis in this bacterium was a growth associated process. This is in contrast to many other Pseudomonas species in which the recognized time for the highest PHA accumulation was when the specific growth rate of the organism was low under nutrient limitation but require an excess of carbon substrate.Citation28 However, there have been reports in some Pseudomonas species that their cell growth and PHA accumulation increased synchronously, for example, P. putida Bet001,Citation28 P. putida PGACitation1,15,43, and Pseudomonas fluorescens BM07.Citation44 These growth-associated PHA producing organisms do not require any nutrient limitation for initiation of the accumulation. PHA accumulation and growth occurred simultaneously and the PHA production process can usually be performed in one stage Citation28,45 although nutrient limitation can be applied to optimize PHA production. Fig. S2 shows the accumulated PHA in P. mendocina PSU. Cells accumulate large amounts of PHA that is visible as highly refractile granules by phase contrast microscopy (Fig. S2(A)) in addition, the transmission electron micrograph showed that the PHA granules were hydrophobic materials stored in water insoluble granules in the cell cytoplasm (Fig. S2(B)).
Table 3. Predicted and experimental values of the responses at optimal conditions.
Fig. 2. Time profile of cell growth, PHA accumulation, alginate production and substrate utilization by Psuedomonas mendocina PSU grown with MSM supplemented with 20 g/L of total glycerol in BLW with a C/N of 40 at 35 °C and initial pH 7.0 (optimal conditions for PHA production obtained from RSM). Each data point represents a mean value of three independent experiments and a vertical bar represents the standard deviation.
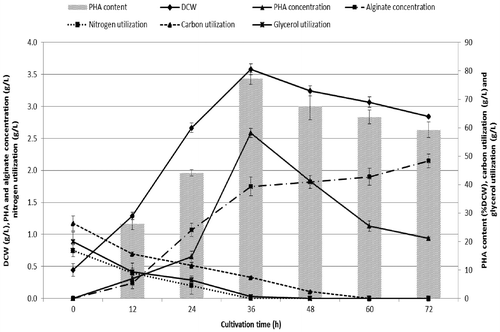
It is well known that the biomass and PHA content can be increased by changing the C/N molar ratio, initial pH, and incubation temperature. In this study, the maximum biomass (3.65 g/L) and PHA content (approximately 77% DCW) were obtained at C/N of 40, an initial pH of 7.0 and 35 °C. These can be explained that at a C/N ratio of 20, carbon becomes the limiting factor of growth whereas any additional increase in C/N up to 60 did not result in a further increase in the amount of biomass because the nitrogen concentration was no longer sufficient to support growth.Citation28,46 In general, nitrogen limitation has been largely used to promote the biosynthesis of PHA. The C/N ratio is important in the biosynthesis of PHA, especially in a one-stage culture because the accumulation of acetyl-CoA is determined by the amount of available nitrogen in the culture medium. C/N ratios of between 20 and 40 are widely used for optimal PHA biosynthesis.Citation24,46 Typically, metabolic processes are highly susceptible to even slight changes in pH. The initial pH value may also affect the bioavailability of some of the required trace elements. Most experimental data have indicated that pH values other than 7.0 reduce PHA production.Citation40,46 In addition, the incubation temperature was another crucial parameter for achieving a high biomass and PHA content. The optimal temperature for growth of most mesophilic organisms is between 30 and 37 °C. For instance, a temperature of 30 °C is the optimal temperature for P. putida CA-3 to grow and produce PHACitation47, whereas Pseudomonas guezennei sp. nov. has an optimal temperature of between 33 and 37 °C.Citation48 Any deviations above and below this temperature will contribute to an increase in stress and may thus challenge its ability to accumulate PHA.Citation49 However, according to the data obtained from the previous experiment before and after optimization by RSM, the optimal conditions were similar. The maximum DCW and PHA content of P. mendocina PSU were approximately 3.6 g/L and 77% DCW, respectively, produced at 36 h of cultivation using 20 g/L of total glycerol in the BLW and 1.0 g/L of ammonium sulfate (shown in Tables and ) with the same C/N ratio of 40 as determined in the RSM experiment. This is the highest PHA content that has been reported and the obtained DCW was higher compared with all previous work that has used P. mendocina species.Citation18,50,51
PCR analysis of SCL-PHA synthase and MCL-PHA synthase
Some Pseudomonas strains including Pseudomonas sp. 61-3,Citation2,52 Pseudomonas sp. LDC-5,Citation26 and Pseudomonas sp. USM 4-55Citation53 can accumulate two types of PHA, SCL-PHA e.g. P(3HB) and MCL-PHA. This may require two separate systems for the accumulation of the monomers i.e. the presence of two different PHA synthases such as the SCL-PHA and MCL-PHA synthase. Molecular studies have shown that these strains possessed two PHA synthetic pathways, one responsible for P(3HB) production and the other for MCL-PHA accumulation each requiring its own specific PHA synthase.Citation52 In this study, the phbC and phaC genes of the expected lengths of 978 and 540 bp, respectively, were amplified from P. mendocina PSU (Fig. ). The phbC and phaC of P. mendocina PSU exhibited 100 and 99% identity to the phbC and phaC of Pseudomonas sp. USM 4-55, respectively. A phylogenetic tree (see Fig. S3) was generated from the 21 bacteria possessing the PHA synthases. The phbC of Pseudomonas sp. PSU was located in the same group as the phbC of Pseudomonas sp. USM 4-55 and P. stutzeri A1501 with a strong bootstrap support of 100%, whereas the phaC of this strain showed the closest relationship to Pseudomonas sp. USM 4-55 with a 98% bootstrap. Therefore, the PHA synthase genes of P. mendocina PSU appear to be similar to the PHA synthase genes of Pseudomonas sp. USM 4-55. According to Tan et al., the PHA synthase genes of Pseudomonas sp. USM 4-55 have been cloned and characterized. The result showed that this strain was capable of producing both SCL-PHA and MCL-PHA simultaneously and its genetic arrangement of the PHA biosynthesis operon was similar to Pseudomonas sp. 61-3 that has well-studied PHA synthases and an ability to incorporate both SCL and MCL monomers into a PHA copolymer chain. The phbC locus of Pseudomonas sp. USM 4-55 was clustered in the phbBAC operon which may be a unique property of the Pseudomonads that can produce both SCL-PHA and MCL-PHA whereas in the earliest operon model investigated, C. necator, its operon was arranged as phbCAB.Citation53 However, the genetic organization in P. mendocina PSU will be further studied. To corroborate that this strain can produce two types of PHA granules, GC–MS and 1H NMR were used to determine the monomer compositions of the produced PHA.
Fig. 3. PCR analysis of the SCL-PHA synthase gene (phbC) and MCL-PHA synthase gene (phaC) in Pseudomonas sp. 100 pb ladder (lane 1, left) lane 2–4 primers for phbC amplification were used24 whereas lane 5–7 primers for phaC amplification were used25,26 lane 2 and lane 5; Pseudomonas mendocina PSU against control e.g. Pseudomonas sp. USM 4-55 (lane 3 and lane 6) and negative control e.g. Burkholderia sp. USM (lane 4 and lane 7).
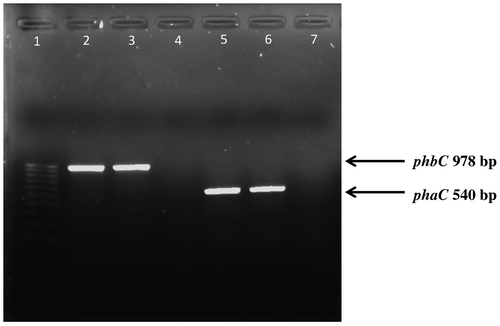
PHA monomeric compositions
The PHA produced from P. mendocina PSU when grown for 36 h with BLW was composed of three types of monomers: 3-hydroxybutyrate (3HB), 3-hydroxyoctanoate (3HO), and 3-hydroxydecanoate (3HD) by comparing their mass spectrogram with that of the standards in the GC–MS analysis (see Fig. S4). To confirm the polymer structure of the type of PHA produced from P. mendocina PSU, 1H NMR was performed. The resulting spectrum exhibited typical chemical shift patterns of the already published spectra for this polyester by Shahid et al.Citation29 At 5.2 ppm (a), the spectrum showed a specific signal of the methine protons attached to an ester function. The polymer structure was in addition verified by the absence of a signal at 4.1 ppm that is characteristic of the CH groups attached to an alcohol function. The methylene protons of C2 had a set of signals between 2.5 and 2.6 ppm (b). The terminal methyl group was shown at 0.9 ppm (e). The methylene protons of C4 gave a signal at 1.6 ppm (c), whereas all other methylene hydrogens of the saturated side chains generated a signal at 1.3 (d) (Fig. ).
Fig. 4. 1H-NMR spectrum of PHA produced from Pseudomonas mendocina PSU when grown with BLW and a schematic structure of the PHA.
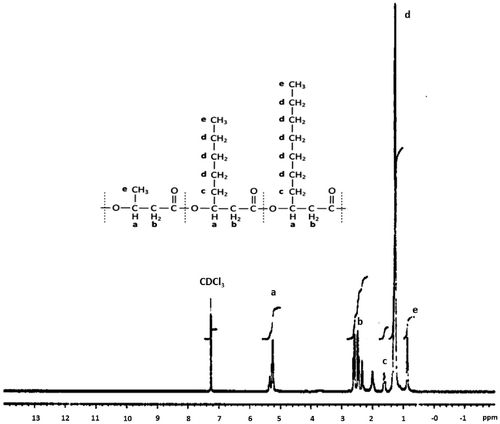
Due to P. mendocina PSU possessing two PHA synthases (i.e. the SCL-PHA and MCL-PHA synthase) and the glycerol and fatty acids which are the major components in the BLW were used as the carbon substrates, SCL-PHA e.g. 3HB and MCL-PHA e.g. 3HO and 3HD could be accumulated. The 3HB unit would be synthesized from glycerol through the glycolysis pathway and from fatty acids through a β-oxidation pathway that generated the 3-hydroxybutyryl CoA, an intermediate precursor for P(3HB) biosynthesis. Then, P(3HB) will be polymerized by the action of the SCL-PHA synthase (phbC). In contrast, the precursors for the MCL-PHA could occur via a de novo fatty acid biosynthetic pathway and β-oxidation pathway when glycerol (structurally unrelated carbon source) and fatty acid (structurally related carbon source) were utilized, respectively. The intermediate R-3-hydroxyacyl-CoA was then polymerized to MCL-PHA by the action of MCL-PHA synthase (phaC).Citation3,5,26,49 In this study, there was evidence that P. mendocina PSU preferred to utilize glycerol prior to any fatty acid components; however, after 48 h the glycerol carbon supply was completely depleted then fatty acids were metabolized (see Fig. ). This result was very interesting because until now there has been no report that P. mendocina species can produce 3HB. According to the production of PHA among P. mendocina species in the previous studies, only MCL-PHA was produced due to their lack of phbC. For example, P. mendocina NK-01 produced 3HO and 3HDCitation18 whereas 3HHx, 3HO, and 3HD were synthesized by P. mendocina 0806 when they were grown with glucose.Citation50 P. mendocina CH50 could produce the homopolymer 3HO when sodium octanoate was used as a carbon source.Citation51 In general, the composition of the produced PHA was primarily controlled by three factors e.g. the specificity of the PHA synthase, the availability of suitable carbon sources, and their specific metabolic pathways.Citation6 Hence the newly isolate P. mendocina PSU was considered to be the most likely strain to use for the synthesis of new PHA products by manipulation of the PHA synthase genes and substrate modifications. For example, the production of copolymers of SCL-PHA and MCL-PHA with various PHA compositions that will show a wide range of physical and mechanical properties and may provide similar properties to petroleum-based plastics such as a low density polyethylene.Citation8 In addition, this is the first time that PHA has been produced from a BLW byproduct from biodiesel production as the sole carbon source by a P. mendocina species. However, the amounts of the monomer components of PHA did vary slightly during the cultivation time as the proportion of 3HB was decreased whereas the 3HO and 3HD increased (see Fig. S5). As a result of P. mendocina PSU having the ability to utilize various carbon substrates through different metabolic pathways, the expression of each PHA synthase and its substrate specificity with different carbon sources will be further investigated.
Production of the EPS alginate
Depending upon the species and environmental conditions, the amount and types of EPS produced can vary extensively. It has become increasingly clear that alginate is one of the many EPS polymers that can be produced by a number of Pseudomonas species. Alginate production probably plays a fundamentally important role in the ecological success of these microorganisms under environmental stress.Citation16,Citation17 For example, Rehm and Valla found that under unbalanced growth conditions in addition to the intracellular accumulation of PHA, some bacteria mostly from the genus Pseudomonas produced alginate as an EPS.Citation16 The biosynthetic pathway for alginate and PHA in Pseudomonas species has been well characterized according to recent reviews.Citation17,Citation18 It would be expected that acetyl-CoA was a common substrate utilized for PHA and alginate synthesis in P. mendocina.
In this study, during the bacterial production of PHA, the production of alginate was also investigated. Fig. shows the amount of PHA and alginate produced over the 72 h period of cultivation. PHA production reached a maximum about 2.6 g/L at 36 h and then decreased to 1.8 g/L at 48 h. Due to the carbon supply being rapidly diminished, an intracellularly stored PHA started to degrade in contrast to the production of alginate that gradually increased and continued to the value of 2.15 g/L at the end of the fermentation process even after the depletion of the carbon source. This situation can be explained by the PHA being degraded by the cells to not only maintain their growth but also for alginate production.
The extracted alginate from P. mendocina PSU was further analyzed by LC–MS to determine its monomer compositions compared with the standard. According to ESI-MS data, the major product ions at m/z 177.1 were from 1-deoxy-β-d-mannuronic acid and/or 1-deoxy-α-l-guluronic acid. After these products were hydrolyzed by the acid and high temperature conditions, 1-deoxy-β-d-mannuronic acid and/or 1-deoxy-α-l-guluronic acid were then converted to d-gluconic acid and/or l-gluconic acid as shown by the ions at m/z 195.1 (see Table S10 and Fig. S6). However, the ions at m/z 217.0 were from acetylated 1-deoxy-4-enoyl-β-d-mannuronic acid and/or acetylated 1-deoxy-4-enoyl-α-l-guluronic acid have been found only in the alginate produced from P. mendocina PSU. This result is a further confirmation that acetylation of alginate is a normal occurrence in the production of bacterial alginate.
Recently, the relationships between the PHA and alginate biosynthetic pathway in P. mendocina NK-01 have been identified. Acetyl-CoA has been indicated as the common substrate utilized for the synthesis of both PHA and alginateCitation16,18,54 hence if one pathway was disrupted, this could promote the synthesis of the other pathway. It was hypothesized that blocking the alginate synthetic pathways could lead to a better production of PHA because most of the excess carbon would be used for PHA biosynthesis; however, this assumption will require further investigations.
In conclusion, P. mendocina PSU, a new isolate from a site contaminated with a biodiesel waste product produced a high amount of PHA (approximately 77% DCW in a 3.65 g/L of the DCW) when grown with BLW. In addition, an alginate was also produced during the PHA production and reached 2.15 g/L at the end of cultivation. This is the first report for the use of an inexpensive BLW for PHA production from a P. mendocina species. Moreover, the SCL-PHA synthase that was responsible for P(3HB) biosynthesis was for the first time discovered in this species. Further work is now being carried out to limit the production of alginate and to determine the cost effectiveness for the industrial production of this PHA and its potential use.
Author contribution
WC conducted the experimental works, analyzed the results, and prepared the first draft. BH, KS and KU designed the experiments, analyzed the results, and prepared the final manuscript for submission.
Funding
This work was financially supported by the Development and Promotion of Science and Technology Talents Projec (DPST) Scholarship and Prince of Songkla University (SCI560117S), Thailand.
Supplemental materials
The supplemental material for this paper is available at http://dx.doi.org/10.1080/09168451.2016.1158628.
TBBB_1158628_Supplemental.zip
Download Zip (3.1 MB)Acknowledgment
We gratefully acknowledge Biodiesel Ethyl Ester Pilot Plant, the Specialized R & D Center for Alternative Energy from Palm Oil and Oil Crops, Faculty of Engineering, Prince of Songkla University for the supply of the biodiesel liquid waste.
Disclosure statement
No potential conflict of interest was reported by the authors.
References
- Anderson AJ, Dawes EA. Occurrence, metabolism, metabolic role, and industrial uses of bacterial polyhydroxyalkanoates. Microbiol. Rev. 1990;54:450–472.
- Hokamura A, Fujino K, Isoda Y, et al. Characterization and identification of the proteins bound to two types of polyhydroxyalkanoate granules in Pseudomonas sp. 61-3. Biosci. Biotechnol. Biochem. 2015;79:1369–1377.10.1080/09168451.2015.1023250
- Sudesh K, Abe H, Doi Y. Synthesis, structure and properties of polyhydroxyalkanoates: biological polyesters. Prog. Polym. Sci. 2000;25:1503–1555.10.1016/S0079-6700(00)00035-6
- Rehm BHA. Polyester synthases: natural catalysts for plastics. Biochem. J. 2003;376:15–33.10.1042/bj20031254
- Nomura CT, Taguchi K, Taguchi S, Doi Y. Coexpression of genetically engineered 3-ketoacyl-ACP synthase III (fabH) and polyhydroxyalkanoate synthase (phaC) genes leads to short-chain-length-medium-chain-length polyhydroxyalkanoate copolymer production from glucose in Escherichia coli JM109. Appl. Environ. Microbiol. 2004;70:999–100710.1128/AEM.70.2.999-1007.2004
- Kek YK, Chang CW, Amirul AA, et al. Heterologous expression of Cupriavidus sp. USMAA2-4 PHA synthase gene in PHB−4 mutant for the production of poly(3-hydroxybutyrate) and its copolymers. World J. Microbiol. Biotechnol. 2010;26:1595–1603.10.1007/s11274-010-0335-5
- Rai R, Keshavarz T, Roether JA, et al. Medium chain length polyhydroxyalkanoates, promising new biomedical materials for the future. Mat. Sci. Eng. R. 2011;72:29–47.10.1016/j.mser.2010.11.002
- Chen GQ. Plastics completely synthesized by bacteria: polyhydroxyalkanoates. In: Chen GQ, editor. Plastics from bacteria: natural functions and application. Berlin: Spinger-Verlag; 2010. p. 1–450.10.1007/978-3-642-03287-5
- Choi J, Lee SY. Process analysis and economic evaluation for poly(3-hydroxybutyrate) production by fermentation. Bioprocess. Eng. 1997;17:335–342.10.1007/s004490050394
- Ashby RD, Solaiman DK, Foglia TA. Bacterial poly(hydroxyalkanoate) polymer production from the biodiesel co-product stream. J. Polym. Environ. 2004;12:105–112.10.1023/B:JOOE.0000038541.54263.d9
- Cavalheiro JMBT, de Almeida MCMD, Grandfils C, et al. Poly(3-hydroxybutyrate) production by Cupriavidus necator using waste glycerol. Process. Biochem. 2009;44:509–515.10.1016/j.procbio.2009.01.008
- Yang F, Hanna MA, Sun R. Value-added uses for crude glycerol – a byproduct of biodiesel production. Biotechnol. Biofuels. 2012;5:13–23.10.1186/1754-6834-5-13
- Fu J, Sharma U, Sparling R, et al. Evaluation of medium-chain-length polyhydroxyalkanoate production by Pseudomonas putida LS46 using biodiesel by-product streams. Can. J. Microbiol. 2014;60:461–468.10.1139/cjm-2014-0108
- Palmeri R, Pappalardo F, Fragalà M, et al. Polyhydroxyalkanoates (PHAs) production through conversion of glycerol by selected strains of Pseudomonas mediterranea and Pseudomonas corrugata. Chem. Eng. Trans. 2012;27:121–126.
- Annuar MSM, Tan IKP, Ramachandran KB. Evaluation of nitrogen sources for growth and production of medium-chain-length poly-(3-hydroxyalkanoates) from palm kernel oil by Pseudomonas putida PGA1. AsPac. J. Mol. Biol. Biotechnol. 2008;16:11–15.
- Rehm BH, Valla S. Bacterial alginates: biosynthesis and applications. Appl. Microbiol. Biotechnol. 1997;48:281–288.10.1007/s002530051051
- Remminghorst U, Rehm BHA. Bacterial alginates: from biosynthesis to applications. Biotechnol. Lett. 2006;28:1701–1712.10.1007/s10529-006-9156-x
- Guo W, Song C, Kong M, et al. Simultaneous production and characterization of medium-chain-length polyhydroxyalkanoates and alginate oligosaccharides by Pseudomonas mendocina NK-01. Appl. Microbiol. Biotechnol. 2011;92:791–801.10.1007/s00253-011-3333-0
- Zhao X, Liu J, Hu Y, et al. Optimization on condition of glycyrrhetinic acid liposome by RSM and the research of its immunological activity. Int. J. Biol. Macromol. 2012;51:299–304.10.1016/j.ijbiomac.2012.05.005
- Chanasit W, Sueree L, Hodgson B, et al. The production of poly(3-hydroxybutyrate) [P(3HB)] by a newly isolated Bacillus sp. ST1C using liquid waste from biodiesel production. Ann. Microbiol. 2014;64:1157–1166.10.1007/s13213-013-0755-1
- Saitou N, Nei M. The neighbor-joining method: a new method for reconstructing phylogenetic trees. Mol. Biol. Evol. 1987;4:406–425.
- Lau NS, Tsuge T, Sudesh K. Formation of new polyhydroxyalkanoate containing 3-hydroxy-4-methylvalerate monomer in Burkholderia sp. Appl. Microbiol. Biotechnol. 2011;89:1599–1609.10.1007/s00253-011-3097-6
- Kulpreecha S, Boonruangthavorn A, Meksiriporn B, et al. Inexpensive fed-batch cultivation for high poly(3-hydroxybutyrate) production by a new isolate of Bacillus megaterium. J. Biosci. Bioeng. 2009;107:240–245.10.1016/j.jbiosc.2008.10.006
- Lee SH, Kim JH, Mishra D, et al. Production of medium-chain-length polyhydroxyalkanoates by activated sludge enriched under periodic feeding with nonanoic acid. Bioresour. Technol. 2011;102:6159–6166.10.1016/j.biortech.2011.03.025
- Solaiman DK, Ashby RD, Foglia TA. Rapid and specific identification of medium-chain-length polyhydroxyalkanoate synthase gene by polymerase chain reaction. Appl. Microbiol. Biotechnol. 2000;53:690–694.10.1007/s002530000332
- Sujatha K, Mahalakshmi A, Shenbagarathai R. Molecular characterization of Pseudomonas sp. LDC-5 involved in accumulation of poly 3-hydroxybutyrate and medium-chain-length poly 3-hydroxyalkanoates. Arch. Microbiol. 2007;188:451–462.10.1007/s00203-007-0265-2
- Sun Z, Ramsay JA, Guay M, et al. Carbon-limited fed-batch production of medium-chain-length polyhydroxyalkanoates from nonanoic acid by Pseudomonas putida KT2440. Appl. Microbiol. Biotechnol. 2007;74:69–77.10.1007/s00253-006-0655-4
- Gumel AM, Annuar MSM, Heidelberg T. Biosynthesis and characterization of polyhydroxyalkanoates copolymers produced by Pseudomonas putida Bet001 isolated from palm oil mill effluent. PLoS One. 2012;7:e45214. doi:10.1371/journal.pone.0045214.
- Shahid S, Mosrati R, Ledauphin J, et al. Impact of carbon source and variable nitrogen conditions on bacterial biosynthesis of polyhydroxyalkanoates: evidence of an atypical metabolism in Bacillus megaterium DSM 509. J. Biosci. Bioeng. 2013;116:302–308.10.1016/j.jbiosc.2013.02.017
- Gustafsson Ö, Haghseta F, Chan C, et al. Quantification of the dilute sedimentary soot phase: implications for PAH speciation and bioavailability. Environ. Sci. Technol. 1997;31:203–209.10.1021/es960317s
- Bondioli P, Della Bella L. An alternative spectrophotometric method for the determination of free glycerol in biodiesel. Eur. J. Lipid Sci. Technol. 2005;107:153–157.10.1002/(ISSN)1438-9312
- Castellane TCL, Lemos MVF. Lemosa EGdM. Evaluation of the biotechnological potential of Rhizobium tropici strains for exopolysaccharide production. Carbohydr. Polym. 2014;111:191–197.10.1016/j.carbpol.2014.04.066
- Chaki T, Kakimi H, Shibata A, et al. Detection of Alginate Oligosaccharides from Mollusks. Biosci. Biotechnol. Biochem. 2006;70:2793–2796.10.1271/bbb.60313
- Aragone MR, Maurizi DM, Clara LO, et al. Pseudomonas mendocina, an environmental bacterium isolated from a patient with human infective endocarditis. J. Clin. Microbiol. 1992;30:1583–1584.
- Zhu C, Nomura CT, Perrotta JA, et al. Production and characterization of poly-3-hydroxybutyrate from biodiesel-glycerol by Burkholderia cepacia ATCC 17759. Biotechnol. Prog. 2010;26:424–430.
- Ibrahim MHA, Steinbüchel A. Zobellella denitrificans strain MW1, a newly isolated bacterium suitable for poly(3-hydroxybutyrate) production from glycerol. J. Appl. Microbiol. 2010;108:214–225.10.1111/jam.2009.108.issue-1
- Kalaiyezhini D, Ramachandran KB. Biosynthesis of poly-3-hydroxybutyrate (PHB) from glycerol by Paracoccus denitrificans in a batch bioreactor: effect of process variables. Prep. Biochem. Biotechnol. 2015;45:69–83.10.1080/10826068.2014.887582
- Taidi B, Anderson AJ, Dawes EA, et al. Effect of carbon source and concentration on the molecular mass of poly(3-hydroxybutyrate) produced by Methylobacterium extorquens and Alcaligenes eutrophus. Appl. Microbiol. Biotechnol. 1994;40:786–790.10.1007/BF00173975
- Beaulieu M, Beaulieu Y, Melinard J, et al. Influence of ammonium salts and cane molasses on growth of Alcaligenes eutrophus and production of polyhydroxybutyrate. Appl. Environ. Microbiol. 1995;61:165–169.
- Grothe E, Moo-Young M, Chisti Y. Fermentation optimization for the production of poly(β-hydroxybutyric acid) microbial thermoplastic. Enzyme Microb. Technol. 1999;25:132–141.10.1016/S0141-0229(99)00023-X
- Belal EB. Production of poly-β-hydroxybutyric acid (PHB) by Rhizobium elti and Pseudomonas stutzeri. Curr. Res. J. Biol. Sci. 2013;5:273–284.
- Zafar M, Kumar S, Kumar S, et al. Optimization of polyhydroxybutyrate (PHB) production by Azohydromonas lata MTCC 2311 by using genetic algorithm based on artificial neural network and response surface methodology. Biocatal. Agric. Biotechnol. 2012;1:70–79.
- Marsudi S, Tan IKP, Gan SN, et al. Production of medium chain length polyhydroxyalkanoates from oleic acid using Pseudomonas putida PGA1 by fed batch culture. Makara Teknologi. 2007;11:1–4.
- Lee HJ, Choi MH, Kim TU, et al. Accumulation of polyhydroxyalkanoic acid containing large amounts of unsaturated monomers in Pseudomonas fluorescens BM07 utilizing saccharides and its inhibition by 2-bromooctanoic acid. Appl. Environ. Microbiol. 2001;67:4963–4974.10.1128/AEM.67.11.4963-4974.2001
- Chee JY, Yoga SS, Lau NS, et al. Bacterially produced polyhydroxyalkanoate (PHA): converting renewable resources into bioplastics. In: Mendez-Vilas A, editor. Current research, technology and education topics in Applied Microbiology and Microbial Biotechnology. Vol. 2. Badajoz: Formatex Research Center Publishers; 2010. p. 789–1620.
- Lee WH, Loo CY, Nomura CT, et al. Biosynthesis of polyhydroxyalkanoate copolymers from mixtures of plant oils and 3-hydroxyvalerate precursors. Bioresour. Technol. 2008;99:6844–6851.10.1016/j.biortech.2008.01.051
- Goff M, Nikodinovic-Runic J, O’Connor KE. Characterization of temperature-sensitive and lipopolysaccharide overproducing transposon mutants of Pseudomonas putida CA-3 affected in PHA accumulation. FEMS Microbiol. Lett. 2009;292:297–305.10.1111/fml.2009.292.issue-2
- Simon-Colin C, Alain K, Colin S, et al. A novel mcl-PHA-producing bacterium, Pseudomonas guezennei sp. nov., isolated from a ‘kopara’ mat located in Rangiroa, an atoll of French Polynesia. J. Appl. Microbiol. 2008;104:581–586
- Madison LL, Huisman GW. Metabolic engineering of poly (3-hydroxyalkanoates): from DNA to plastic. Microbiol. Mol. Biol. Rev. 1999;63:21–53.
- Tian W, Hong K, Chen GQ, et al. Production of polyesters consisting of medium chain length 3- hydroxyalkanoic acids by Pseudomonas mendocina 0806 from various carbon sources. Anton. van. Leeuwenhoek. 2000;77:31–36.10.1023/A:1002099023046
- Rai R, Yunos DM, Boccaccini AR, et al. Poly-3-hydroxyoctanoate P(3HO), a medium chain length polyhydroxyalkanoate homopolymer from Pseudomonas mendocina. Biomacromolecules. 2011;12:2126–2136.10.1021/bm2001999
- Matsusaki H, Manji S, Taguchi K, et al. Cloning and molecular analysis of the poly(3-hydroxybutyrate) and poly(3-hydroxybutyrate-co-3-hydroxyalkanoate) biosynthesis genes in Pseudomonas sp. strain 61-3. J. Bacteriol. 1998;180:6459-6467.
- Tan Y, Neo PC, Najimudin N, et al. Cloning and characterization of poly(3-hydroxybutyrate) biosynthesis genes from Pseudomonas sp. USM 4-55. J. Basic Microbiol. 2010;50:179–189.
- Guo W, Feng J, Geng W, et al. Augmented production of alginate oligosaccharides by the Pseudomonas mendocina NK-01 mutant. Carbohydr. Res. 2012;352:109–116.10.1016/j.carres.2012.02.024