Graphical abstract
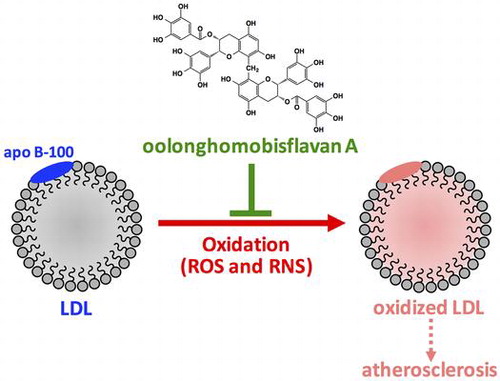
Abstract
Oxidation of low-density lipoprotein (LDL) by reactive oxygen species (ROS) and reactive nitrogen species (RNS) has been suggested to be involved in the onset of atherosclerosis. Oolong tea contains unique polyphenols including oolonghomobisflavan A (OFA). In this study, the effects of OFA on LDL oxidation by ROS and RNS were investigated in vitro. OFA suppressed formation of cholesterol ester hydroperoxides in LDL oxidized by peroxyl radical and peroxynitrite, and formation of thiobarbituric acid reactive substances in LDL oxidized by Cu2+. In addition, OFA inhibited fragmentation, carbonylation, and nitration of apolipoprotein B-100 (apo B-100) in the oxidized LDL, in which heparin-binding activity of apo B-100 was protected by OFA. Our results suggest that OFA exhibits antioxidant activity against both lipid peroxidation and oxidative modification of apo B-100 in LDL oxidized by ROS and RNS. Polyphenols in oolong tea may prevent atherosclerosis by reducing oxidative stress.
It has been suggested that high level of low-density lipoprotein (LDL) in blood and oxidation of LDL are positively associated with the onset of cardiovascular diseases.Citation1) The core of LDL is composed of cholesterol esters and triacylglycerols and is covered by phospholipid monolayer containing unesterified cholesterol and apolipoprotein B-100 (apo B-100).Citation2) Unsaturated fatty acid moieties of lipids in LDL can be oxidized by reactive oxygen species (ROS), such as hydroxyl radical, and reactive nitrogen species (RNS), such as peroxynitrite, generating lipid hydroperoxides.Citation3) Cysteine, lysine, histidine, and tyrosine residues of apo B-100 are also targets of ROSCitation4) and RNS.Citation5) Oxidative modification of apo B-100 may deteriorate the function of apo B-100 as a ligand to LDL receptors. In atherosclerosis, oxidized LDL (oxLDL) is phagocytosed by monocyte-derived macrophages through scavenger receptor in the intima.Citation6) Lipid-loaded macrophages transform into foam cells and accumulate to form fatty streak and fibrous plaque.
Oolong is a semifermented tea prepared from leaves of Camellia sinensis, while green tea is an unfermented tea. The fermentation of oolong tea is not mediated by microbes, but instead, it is mediated by oxidative enzymes such as polyphenol oxidase and peroxidase in the leaves.Citation7) The fermentation process induces browning and generates unique flavors and tastes. Depending on the method of preparation including degree of oxidation, various oolong teas are available in Asian countries. Several studies have shown that oolong tea has many beneficial health effects, such as antihyperglycemic, antiobesity, and mitigation of the risk of cardiovascular disease.Citation8) The major components of oolong tea are alkaloids, saponins, polysaccharides, l-theanines, and polyphenols including polymerized polyphenols derived from the oxidative polymerization of catechins.Citation8) Thearubigins, theaflavins, and dimeric catechins, such as oolonghomobisflavans, are the most abundant polymerized polyphenols of oolong tea.Citation9) Oolonghomobisflavan A (OFA, Fig. ) has a unique structure as a dimer of epigallocatechin gallate (EGCG).Citation10) Although the mechanisms remain unclear, the biological effects of oolong tea polyphenols have attracted considerable attention over the past few years. In the present study, we investigated the antioxidant effects of OFA on LDL oxidation by ROS and RNS in vitro.
Materials and methods
Materials
OFA was purchased from Nagara Science Co. (Gifu, Japan). 2,2-Diphenyl-1-picrylhydrazyl (DPPH), 2,2′-azobis (2-amidinopropane) dihydrochloride (AAPH), and 2,4-dinitrophenylhydrazine (DNPH) were obtained from Wako Pure Chemical Industries (Osaka, Japan). 3-(4-Morpholinyl) sydnonimine (SIN-1) was supplied from Dojindo Molecular Technologies Inc. (Kumamoto, Japan). All other chemicals were of reagent grade.
Radical-scavenging assay
Radical-scavenging capacity of OFA in solution was determined by DPPH method.Citation11) Methanol solutions (400 μL) containing various concentrations of OFA (2–5 μM) were mixed with 400 μL of 200 μM DPPH in methanol. The absorbance of the reaction mixture was measured at 517 nm after incubation at room temperature for 30 min in the dark. 6-Hydroxy-2,5,7,8-tetramethylchroman-2-carboxylic acid (Trolox, EMD Chemicals, Inc., San Diego, CA, USA) was used as a reference antioxidant.
Isolation and purification of LDL
Human LDL was prepared by discontinuous density gradient ultracentrifugation using KBr.Citation12) Human blood (200 mL) was collected from a healthy volunteer with informed consent after fasting overnight using 0.4% citric acid as an anticoagulant, and blood plasma was separated by centrifugation at 1,200 × g at 4 °C for 20 min. KBr solution (d = 1.019, 8 mL) was layered on 16 mL of the plasma and centrifuged at 100,000 × g at 16 °C for 16 h. The top lipid layer was removed and density of the lower layer was adjusted to 1.063 by adding KBr. KBr solution (d = 1.063, 3.5 mL) was added to 21 mL of the lower fraction and centrifuged at 100,000 × g at 16 °C for 20 h. The top layer including LDL fraction (d = 1.019–1.063) was then ultrafiltered and dialyzed against phosphate-buffered saline (PBS, pH 7.4) treated with chelating resin (Sigma-Aldrich Co., St. Louis, MO, USA) at 4 °C. Protein concentration of LDL was measured by Lowry’s method.Citation13) LDL fraction was kept at 4 °C under nitrogen gas.
Peroxyl radical-mediated oxidation of LDL
LDL (200 μg protein/mL) was preincubated with OFA (0.5, 1, and 2 μM) in PBS containing 10 mM diethylene triamine pentaacetic acid (DTPA) at 37 °C for 5 min. Oxidation of LDL was induced by 5 mM of AAPH, an aqueous peroxyl radical generating reagent, at 37 °C. Aliquots (50 μL) of the reaction mixture were mixed with 0.9 mL of 2 mM butyl hydroxyl anisole in methanol at intervals. n-Hexane (1.5 mL) was added to oxLDL in methanol to extract neutral lipids. After centrifugation at 1,200 × g at 4 °C for 5 min, the upper layer was collected and extraction with n-hexane from the bottom layer was repeated again. The upper layer extracts were pooled and evaporated, and the residue was dissolved in 100 μL of 2-propanol. Cholesteryl ester hydroperoxide (CE-OOH) in the sample was analyzed by reversed-phase high-performance liquid chromatography (HPLC) using an InertSustain C8 column at 40 °C (150 × 4.6 mm i.d.; GL Science Inc., Tokyo, Japan) with methanol as an eluent at a flow rate of 1.0 mL/min.Citation14) The concentration of CE-OOH was detected at 235 nm and calculated using cholesteryl linoleate hydroperoxide (Cayman Chemical Company, Ann Arbor, MI, USA) as an authentic standard.
Metal ion-catalyzed oxidation of LDL
LDL (200 μg protein/mL) was preincubated with OFA (0.5, 1, and 2 μM) in PBS at 37 °C for 5 min. LDL oxidation was initiated by adding 5 μM CuCl2 at 37 °C. Aliquots (50 μL) of the reaction mixture were mixed with 450 μL of 0.2% 2-thiobarbituric acid and heated at 95 °C for 60 min. After centrifugation at 20,000 × g at 4 °C for 5 min, 2-thiobarbituric acid reactive substances (TBARS) in the supernatant were determined by reversed-phase HPLC with fluorescence detection (excitation at 515 nm, emission at 553 nm).Citation15) The samples were eluted with acetonitrile/water (30:70, v/v) at 1.0 mL/min on InertSustain C18 column (150 × 4.6 mm i.d.; GL Science Inc.) at 40 °C. 1,1,3,3-Tetramethoxypropane was used as a standard.
Apo B-100 analysis in oxLDL
LDL (200 μg protein/mL) was oxidized by 5 μM CuCl2 at 37 °C for 3 h in the presence of 2 μM OFA as described above. OxLDL was analyzed by sodium dodecyl sulfate-polyacrylamide gel electrophoresis (SDS-PAGE) using an acrylamide slab gel system (AnykD TGX gel; Bio-Rad Laboratories, Hercules, CA, USA) according to Laemmli’s method.Citation16) The gel was stained with Coomassie Brilliant Blue (CBB). Proteins in the other gel were transferred to polyvinylidene difluoride membrane for Western blotting. The membrane was incubated with serum-free blocking buffer (Thermo Fisher Scientific Inc., Rockford, IL, USA) and washed with 0.05% Tween-Tris-buffered saline (TBS). The membrane was incubated with anti-human apo B-100 monoclonal (6H12) antibody (MP Biomedicals, Santa Ana, CA, USA) for 1 h at room temperature. After washing with 0.05% Tween-TBS, the membrane was incubated with goat anti-mouse IgG-Fc conjugated with horseradish peroxidase (Bethyl Laboratories, Inc., Montgomery, TX, USA) for 1 h at room temperature. The immunoreactive substances were detected with the enhanced chemiluminescence (ECL) reagent (GE Healthcare, Little Chalfont, UK).
Protein carbonyl analysis of oxLDL
LDL (200 μg protein/mL) was oxidized by 5 μM CuCl2 at 37 °C for 3 h in the presence of OFA (0.5, 1, and 2 μM). Protein carbonyls in oxLDL was derivatized with 2,4-dinitrophenylhydrazine and analyzed by SDS-PAGE and Western blotting as previously described.Citation17) Briefly, rabbit anti-dinitrophenyl antisera (Dako Cytomation, Glostrup, Denmark) and goat anti-rabbit IgG-Fc conjugated with horseradish peroxidase (Bethyl Laboratories Inc.) were used as primary and secondary antibodies, respectively.
Heparin-binding activity of apo B-100 in oxLDL
LDL (200 μg protein/mL) was oxidized by 5 μM CuCl2 at 37 °C for 3 h in the presence of 2 μM OFA. OxLDL (20 μg protein/100 μL) was incubated with 100 μL of heparin Sepharose 6 Fast Flow (GE Healthcare) for 3 h at 4 °C. The heparin Sepharose beads were washed 10 times with 1 mL of 0.05% Tween-TBS and mixed with 100 μL of SDS-PAGE sample buffer. After heating at 95 °C for 5 min, proteins in the supernatant were analyzed by SDS-PAGE and CBB staining.
Peroxynitrite-mediated oxidation of LDL and nitrotyrosine analysis
LDL (200 μg protein/mL) was preincubated with OFA (2, 4, and 8 μM) in PBS containing 10 mM DTPA at 37 °C for 5 min. LDL was oxidized by 1 mM SIN-1, a water-soluble peroxynitrite generator, at 37 °C. Determination of CE-OOH in oxLDL was performed as previously described. Proteins in oxLDL were analyzed by SDS-PAGE and CBB staining. Nitrotyrosine in oxLDL was analyzed by SDS-PAGE and Western blotting using primary mouse anti-nitrotyrosine monoclonal (CC.22.8C7.3) antibody (Cayman Chemical Company) and secondary goat anti-mouse IgG-Fc conjugated with horseradish peroxidase (Bethyl Laboratories Inc.). The immunoreactive substances were detected with ECL reagent.
Results and discussion
Several studies have reported the antioxidant effects of oolong tea. Xie et al.Citation18) reported that oolong tea extract exerts higher antioxidative activity than black tea in vitro. Yen et al.Citation19) showed that oolong tea extract exhibits stronger radical-scavenging activity than that of green and black teas. However, the active compounds in oolong tea have not been identified. Oolong tea contains various polymerized polyphenols.Citation20) During the semifermentation process in making oolong tea, monomeric polyphenols, especially catechins, are converted to oligomeric flavan-3-ols by polyphenol oxidases. The contents of polymerized polyphenols in oolong tea leaves depend on the producing procedures such as fermentation. Komatsu et al.Citation21) indicated that contents of polymerized polyphenols and EGCG in oolong tea prepared from 15 g of leaves are 68 mg/300 mL and 81 mg/300 mL, respectively. On the other hand, Toyoda-Ono et al.Citation22) showed that contents of polymerized polyphenols and EGCG in oolong tea extract are 114 mg/g and 14 mg/g, respectively. Zhu et al.Citation23) reported that the strong free radical-scavenging activity of oolong tea water extracts is attributed to several lower-molecular-weight polymerized polyphenols. Hashimoto et al.Citation24) reported that polyphenols such as monomeric and dimeric flavan-3-ols and hydrolyzed tannins in black, oolong, and green teas contribute to their scavenging activities of free radicals. These suggest that EGCG and epicatechin gallate are not the only major antioxidant components in teas. Hashimoto et al.Citation25) isolated 53 mg of OFA from 5 kg of oolong tea as a kind of polymerized polyphenol that has dimeric structure of EGCG (Fig. ). Several studies have shown that EGCG in green tea has many health benefits including antioxidant effects.Citation24,26) Therefore, we hypothesized that OFA exhibits antioxidant activity and might decrease oxidative stress in human body. A high level of ROS in human body can inflict damage to the structure and function of LDL, which in turn may lead to atherosclerosis.Citation27) LDL oxidation involves lipid peroxidation and modification of apolipoproteins.Citation28) In the present study, we evaluated the antioxidant effects of OFA on LDL oxidation induced by ROS and RNS in vitro; both lipid peroxidation and oxidative modification of apo B-100 were analyzed as the oxidative markers.
Radical-scavenging capacity of OFA in solution
Fig. shows DPPH radical-scavenging capacity of OFA in solution (methanol/water). OFA dose-dependently scavenged DPPH radicals. Half maximal inhibitory concentration (IC50) of OFA was 2.99 μM. IC50 of Trolox, an analog of α-tocopherol, was 23.7 μM. These results indicate that OFA has a higher radical-scavenging capacity as compared to Trolox.
Effect of OFA on LDL oxidation by ROS
Lipid peroxidation of polyunsaturated fatty acid moieties in LDL is mediated by radical chain reaction, resulting in the generation of various highly reactive products including lipid hydroperoxides.Citation29) We measured CE-OOH as the major product of lipid peroxidation of LDL induced by AAPH.Citation12) As shown in Fig. , CE-OOH concentration in the control increased with time and attained 8.7 μM at 4 h. OFA dose-dependently suppressed CE-OOH formation; 2 μM of OFA exhibited the strongest inhibition. OFA may act as a chain-breaking antioxidant against peroxyl radical-mediated lipid peroxidation of LDL.
Fig. 3. Effect of OFA on peroxyl radical-mediated lipid peroxidation of LDL.
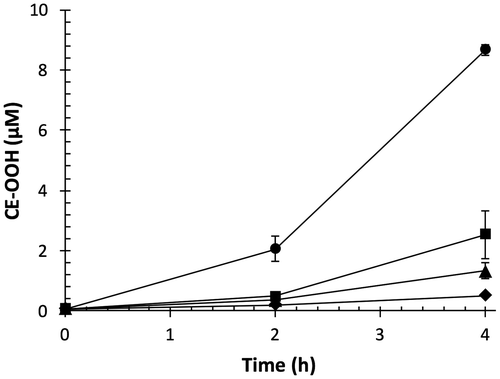
Transition metal ions can generate ROS and cause oxidative stress in human body.Citation17) Cu2+ stimulates lipid peroxidation and catalyzes decomposition of lipid hydroperoxides to lipid alkoxyl and peroxyl radicals.Citation30) During the termination process of lipid peroxidation, secondary oxidation products, such as oxysterols, lipofuscins, and aldehydes, are generated.Citation1) TBARS is a widely-used indicator to detect secondary oxidation products in transition metal ion-catalyzed lipid peroxidation. Several reports have shown that tea polyphenols such as flavan-3-ols inhibit oxidation of LDL catalyzed by Cu2+.Citation31) As shown in Fig. , TBARS time-dependently accumulated up to 12.5 μM at 4 h in control. OFA dose-dependently inhibited TBARS formation; in particular, 2 μM of OFA strongly inhibited TBARS accumulation. These data suggest that OFA is a potent inhibitor of transition metal ion-catalyzed LDL oxidation, due to breaking of the chain reaction by scavenging radicals.
Fig. 4. Effect of OFA on transition metal ion-catalyzed lipid peroxidation of LDL.
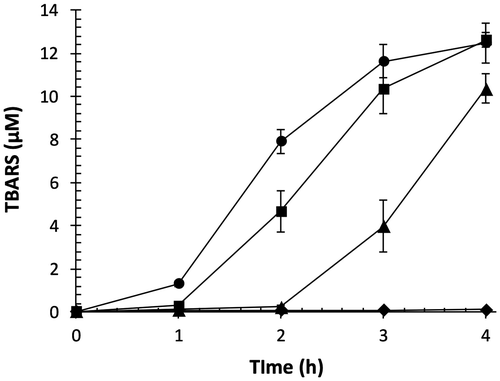
LDL oxidation is characterized by apolipoprotein oxidation and lipid peroxidation.Citation1) Several processes are involved in LDL oxidation, such as chemical modification of amino acid residues, cleavage of peptide chains, and cross linking of polypeptides.Citation32) Fig. shows the effect of OFA on oxidative modification of apo B-100 in LDL oxidized by Cu2+. SDS-PAGE analysis with CBB staining (Fig. (A)) and Western blot analysis with primary anti-human apo B-100 antibody (Fig. (B)) are shown. A single band with molecular weight greater than 250 kDa was observed in native LDL (lane 1, Fig. (A)). However, the band was not detected in oxLDL (lane 2), whereas a weak smear band was observed. The single band remained in oxLDL with 2 μM OFA (lane 3). As in Fig. (A), apo B-100 in Fig. (B) was observed as a single band with molecular weight greater than 250 kDa in native LDL (lane 1). Apo B-100 in LDL incubated with 2 μM OFA for 3 h without oxidation was detected as a single band at the same position of apo B-100 in native LDL (data not shown). A smear band of apo B-100 was detected in oxLDL (lane 2). The oxidative modification of apo B-100 in oxLDL was suppressed by 2 μM OFA (lane 3). These results indicate that OFA can protect oxidation of apo B-100 in LDL oxidized by transition metal ions.
Fig. 5. Effect of OFA on apo B-100 modification in oxLDL.
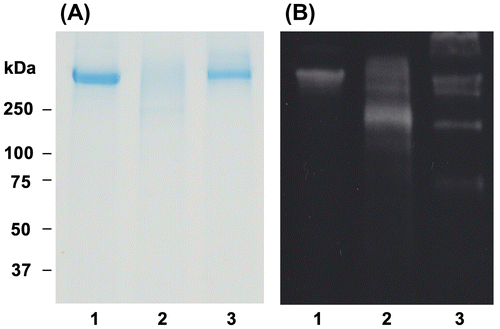
The decomposed products of lipid hydroperoxides generated by transition metal ions can react with the lysine, histidine, threonine, and proline side chain of proteins to form carbonyl compounds.Citation33) Carbonylated protein has been used as a marker of ROS-mediated protein oxidation. DNPH-derivatized protein carbonyls can be detected by Western blotting using anti-dinitrophenyl antibody.Citation34) During LDL oxidation catalyzed by Cu2+, aldehydes including malondialdehyde and 4-hydroxy-2-nonenal can covalently react with lysine residue.Citation35) This process generates Schiff’s bases or Michael adducts and renders LDL particles more electronegative.Citation36) In addition, carbonylated proteins are generated by the reaction of proteins with aldehydes that is produced during lipid peroxidation of LDL oxidation catalyzed by transition metal ions.Citation17) Fig. illustrates the effect of OFA on protein carbonyl formation in LDL oxidized by Cu2+. Protein carbonyls were not observed in native LDL (lane 1). Protein carbonyls were observed in oxLDL as a smear band at high molecular weight (lane 2). OFA dose-dependently inhibited protein carbonyl formation (lane 3, 4, and 5) in oxLDL, and 2 μM OFA strongly suppressed protein carbonyl formation. These results suggest that OFA protects apo B-100 from carbonylation in transition metal ion-catalyzed LDL oxidation, which may be due to preventing lipid peroxidation and its decomposition.
Fig. 6. Effect of OFA on protein carbonyl formation in oxLDL.
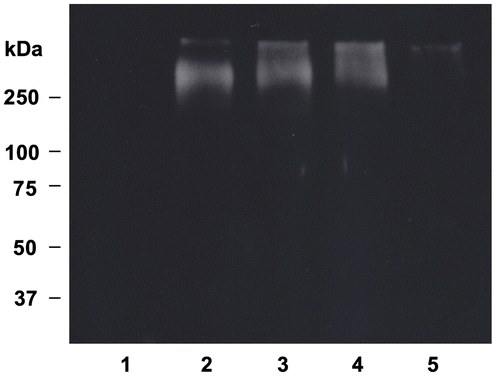
The interaction of apolipoproteins with glycosaminoglycans such as heparin plays an important role in LDL metabolism and cholesterol homeostasis in the circulatory system.Citation37) LDL receptor-binding regions in apo B-100 partly correspond to the heparin-binding regions.Citation38) Peptide analysis of apo B-100 demonstrated that the positively charged amino acid residues, such as lysine and arginine, are responsible for specific binding to heparin.Citation39) Oorni et al.Citation40) suggested that the reduction of the positive charge in lysine and arginine side chains by ROS results in the loss of the heparin-binding activity of apo B-100. The decomposed substances of lipid hydroperoxides induced by Cu2+ can modify lysine residues. Here, the effect of OFA on heparin-binding activity of apo B-100 in LDL oxidized by Cu2+ was examined by SDS-PAGE and CBB staining (Fig. ). A single band greater than 250 kDa was detected in native LDL (lane 1). A single band was found in LDL incubated with 2 μM OFA for 3 h without oxidation at the same position of native LDL (data not shown). However, no protein band was observed in oxLDL (lane 2). The single band was remained in oxLDL with 2 μM OFA (lane 3), indicating that OFA inhibits the loss of heparin-binding activity of apo B-100 in LDL oxidation. These data suggest that OFA may protect the oxidative modification of the lysine residues of apo B-100 in LDL oxidized by transition metal ions.
Fig. 7. Effect of OFA on heparin-binding activity of apo B-100 in oxLDL.
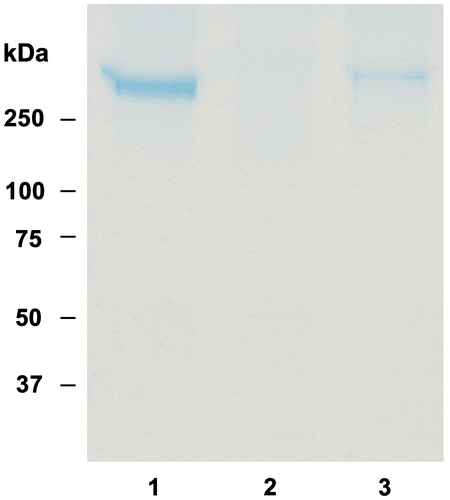
Effect of OFA on LDL oxidation by RNS
RNS such as peroxynitrite is also involved in inducing oxidative stress in human body.Citation41) Peroxynitrite is a strong oxidizing and nitrating speciesCitation42) that is generated by the reaction between nitric oxide and superoxide anion.Citation41) Peroxynitrite can efficiently induce lipid oxidation in cell membranes and lipoproteins.Citation42) LDL oxidized by peroxynitrite binds with high affinity to scavenger receptors of macrophage, leading to the accumulation of cholesteryl esters involved in the production of fatty streak and atherosclerotic lesion.Citation43) We, therefore, examined the effect of OFA against peroxynitrite-mediated oxidation of LDL. Fig. represents the effect of OFA on peroxynitrite-mediated lipid peroxidation induced by SIN-1. SIN-1 generates peroxynitrite by simultaneously releasing nitric oxide and superoxide.Citation44) Concentration of CE-OOH time-dependently increased in the control. OFA dose-dependently suppressed CE-OOH formation in LDL oxidized by peroxynitrite, especially 8 μM of OFA. Lipid–protein adducts are produced during LDL oxidation induced by peroxynitrite.Citation45) Nitrotyrosine is prominently formed by radical reactions through tyrosyl radical intermediate.Citation46) Therefore, nitrotyrosine can be measured as an oxidative stress marker. Fig. shows the effect of OFA on oxidative modification of apo B-100 and nitrotyrosine formation in LDL oxidized by SIN-1. SDS-PAGE analysis with CBB staining and Western blot analysis with primary anti-nitrotyrosine antibody are exhibited in panel A and B, respectively. A single band with molecular weight greater than 250 kDa was observed in native LDL (lane 1, panel A), although smear band was detected in oxLDL induced by SIN-1 (lane 2). A weak single band was observed in oxLDL with 8 μM OFA (lane 3). As in panel B, nitrotyrosine was not detected in native LDL (lane 1). However, a single band of nitrotyrosine greater than 250 kDa was detected in oxLDL (lane 2), although it was not a smear band. The nitrotyrosine formation in oxLDL was inhibited by 8 μM OFA (lane 3). Our data indicate that OFA prevents nitrotyrosine formation of apo B-100 as well as lipid peroxidation in LDL oxidized by peroxynitrite.
Fig. 8. Effect of OFA on peroxynitrite-mediated lipid peroxidation of LDL.
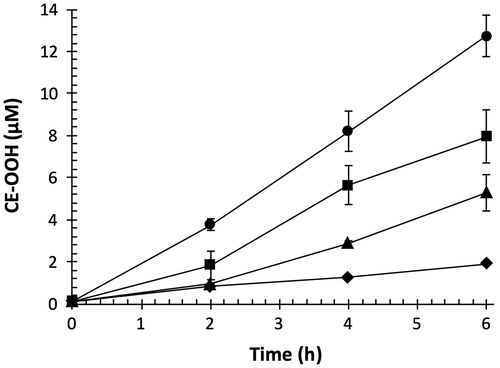
Fig. 9. Effect of OFA on nitrotyrosine formation in oxLDL.
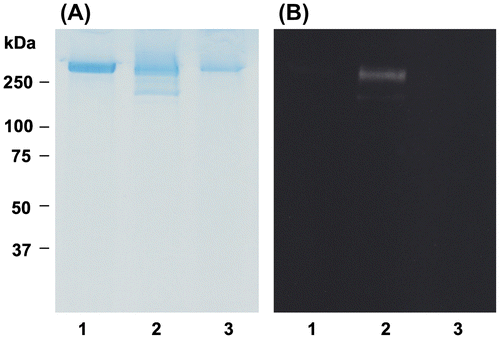
Conclusions
Our data suggest that OFA, an oolong tea-polymerized polyphenol, is a strong antioxidant that suppresses both lipid peroxidation and oxidative modification of apo B-100 in LDL oxidized by ROS, such as peroxyl radicals and transition metal ions, and RNS, such as peroxynitrite. The antioxidant effects of OFA are attributable to its radical-scavenging activity to reduce ROS and RNS. Oolong tea polyphenols may protect against atherosclerosis by reducing oxidative stress.
OFA is a unique polymerized polyphenol that has dimeric structure of EGCG, although information about the content of OFA in oolong tea is not enough so far. Therefore, the role of OFA in total antioxidant activity of oolong tea and comparison of antioxidant activity of OFA with EGCG would be important to elucidate. In addition, further studies using cells and animals are required to determine bioavailability of OFA including the absorption and metabolism because antioxidant activity of OFA may depend on their bioavailability in vivo.
Author contributions
H. A. designed this study. H. A. and R. H. prepared the materials. E. S., S. S., E. W., A. Y., and L. Y. performed the experiment. H. A., E. S., M. T., K. Y., and K. F. analyzed the data and discussed the results. E. S. and H. A. wrote the manuscript.
Disclosure statement
No potential conflict of interest was reported by the authors.
Notes
Abbreviations: Apo B-100, apolipoprotein B-100; AAPH, 2,2′-azobis(2-amidinopropane) dihydrochloride; CBB, Coomassie Brilliant Blue; CE-OOH, cholesteryl ester hydroperoxide; DNPH, 2,4-dinitrophenylhydrazine; DPPH, 2,2-diphenyl-1-picrylhydrazyl; DTPA, diethylene triamine pentaacetic acid; ECL, enhanced chemiluminescence; EGCG, epigallocatechin gallate; HPLC, high-performance liquid chromatography; LDL, low-density lipoprotein; OFA, oolonghomobisflavan A; oxLDL, oxidized LDL; PBS, phosphate-buffered saline; RNS, reactive nitrogen species; ROS, reactive oxygen species; SIN-1, 3-(4-morpholinyl)sydnonimine; SDS-PAGE, sodium dodecyl sulfate polyacrylamide gel electrophoresis; TBARS, 2-thiobarbituric acid reactive substances; TBS, Tris-buffered saline.
References
- Salvayre R, Auge N, Benoist H, et al. Oxidized low-density lipoprotein-induced apoptosis. Biochem Biophys Acta. 2002;1585:213–221.
- Morita SY. Metabolism and modification of apolipoprotein B-containing lipoproteins involved in dyslipidemia and atherosclerosis. Biol Pharm Bull. 2016;39:1–24.10.1248/bpb.b15-00716
- Stocker R, Keaney JF. Role of oxidative modifications in atherosclerosis. Physiol Rev. 2004;84:1381–1478.10.1152/physrev.00047.2003
- Obama T, Kato R, Masuda Y, et al. Analysis of modified apolipoprotein B-100 structures formed in oxidized low-density lipoprotein using LC-MS/MS. Proteomics. 2007;7:2132–2141.10.1002/(ISSN)1615-9861
- Frijhoff J, Winyard PG, Zarkovic N, et al. Clinical relevance of biomarkers of oxidative stress. Antioxid Redox Signal. 2015;23:1144–1170.10.1089/ars.2015.6317
- Moore KJ, Tabas I. Macrophages in the pathogenesis of atherosclerosis. Cell. 2011;145:341–355.10.1016/j.cell.2011.04.005
- Sheibani E, Duncan SE, Kuhn DD, et al. Changes in flavor volatile composition of oolong tea after panning during tea processing. Food Sci Nutr. 2016;4:456–468.10.1002/fsn3.2016.4.issue-3
- Sae-tan S. Systematic review: hypolipidemic activity of oolong tea polymerized polyphenols. J Health Res. 2016;30:451–459.
- Yi D, Tan X, Zhao Z, et al. Reduced risk of dyslipidaemia with oolong tea consumption: a population-based study in southern China. Br J Nutr. 2014;111:1421–1429.10.1017/S0007114513003644
- Nakai M, Fukui Y, Asami S, et al. Inhibitory effects of oolong tea polyphenols on pancreatic lipase in vitro. J Agric Food Chem. 2005;53:4593–4598.10.1021/jf047814+
- Blois MS. Antioxidant determinations by the use of a stable free radical. Nature. 1958;181:1199–1200.10.1038/1811199a0
- Yang L, Kirikoshi J, Seikomoto S, et al. Effect of bean extract of yabumame (Amphicarpaea bracteata (L.) Fernald subsp. edgeworthii (Benth.) H.Ohashi) on low-density lipoprotein oxidation in vitro. Food Sci Technol Res. 2015;21:589–596.10.3136/fstr.21.589
- Lowry OH, Rosebrough NJ, Farr AL, et al. Protein measurement with the Folin phenol reagent. J Biol Chem. 1951;193:265–275.
- Arai H, Terao J, Abdalla DS, et al. Coulometric detection in high performance liquid chromatographic analysis of cholesteryl ester hydroperoxides. Free Radic Biol Med. 1996;20:365–371.10.1016/0891-5849(96)02062-X
- Fukunaga K, Yoshida M, Nakazono N. A simple, rapid, highly sensitive and reproducible quantification method for plasma malondialdehyde by high-performance liquid chromatography. Biomed Chromatogr. 1998;12:300–303.10.1002/(ISSN)1099-0801
- Laemmli UK. Cleavage of structural proteins during the assembly of the head of bacteriophage T4. Nature. 1970;227:680–685.10.1038/227680a0
- Arai H, Berlett BS, Chock PB, et al. Effect of bicarbonate on iron-mediated oxidation of low-density lipoprotein. Proc Natl Acad Sci USA. 2005;102:10472–10477.10.1073/pnas.0504685102
- Xie B, Shi H, Chen Q, et al. Antioxidant properties of fractions and polyphenol constituents from green, oolong and black teas. Proc Natl Sci Counc Repub China B. 1993;17:77–84.
- Yen GC, Chen HY. Antioxidant activity of various tea extracts in relation to their antimutagenicity. J Agric Food Chem. 1995;43:27–32.10.1021/jf00049a007
- Hsu TF, Kusumoto A, Abe K, et al. Polyphenol-enriched oolong tea increases fecal lipid excretion. Eur J Clin Nutr. 2006;60:1330–1336.10.1038/sj.ejcn.1602464
- Komatsu T, Nakamori M, Komatsu K, et al. Oolong tea increases energy metabolism in Japanese females. J Med Invest. 2003;50:170–175.
- Toyoda-Ono Y, Yoshimura M, Nakai M, et al. Suppression of postprandial hypertriglyceridemia in rats and mice by oolong tea polymerized polyphenols. Biosci Biotechnol Biochem. 2007;71:971–976.10.1271/bbb.60635
- Zhu QY, Hackman RM, Ensunsa JL, et al. Antioxidative activities of oolong tea. J Agric Food Chem. 2002;50:6929–6934.10.1021/jf0206163
- Hashimoto F, Ono M, Masuoka C, et al. Evaluation of the anti-oxidative effect (in vitro) of tea polyphenols. Biosci Biotechnol Biochem. 2003;67:396–401.10.1271/bbb.67.396
- Hashimoto F, Nonaka G, Nishioka I. Tannins and related compounds. XC. 8-C-ascorbyl (-)-epigallocatechin 3-O-gallate and novel dimeric flavan-3-ols, oolonghomobisflavans A and B, from oolong tea.(3). Chem Pharm Bull. 1989;37:3255–3263.10.1248/cpb.37.3255
- Singh BN, Shankar S, Srivastava RK. Green tea catechin, epigallocatechin-3-gallate (EGCG): mechanisms, perspectives and clinical applications. Biochem Pharmacol. 2011;82:1807–1821.10.1016/j.bcp.2011.07.093
- Vogiatzi G, Tousoulis D, Stefanadis C. The role of oxidative stress in atherosclerosis. Hellenic J Cardiol. 2009;50:402–409.
- Frei B, Gaziano JM. Content of antioxidants, preformed lipid hydroperoxides, and cholesterol as predictors of the susceptibility of human LDL to metal ion-dependent and -independent oxidation. J Lipid Res. 1993;34:2135–2145.
- Hevonoja T, Pentikäinen MO, Hyvönen MT, et al. Structure of low density lipoprotein (LDL) particles: basis for understanding molecular changes in modified LDL. Biochim Biophys Acta. 2000;1488:189–210.10.1016/S1388-1981(00)00123-2
- Gutteridge JM. Lipid peroxidation and antioxidants as biomarkers of tissue damage. Clin Chem. 1995;41:1819–1828.
- Miura S, Watanabe J, Tomita T, et al. The inhibitory effects of tea polyphenols (flavan-3-ol derivatives) on Cu2+ mediated oxidative modification of low density lipoprotein. Biol Pharm Bull. 1994;17:1567–1572.10.1248/bpb.17.1567
- Stadtman ER, Levine RL. Free radical-mediated oxidation of free amino acids and amino acid residues in proteins. Amino Acids. 2003;25:207–218.10.1007/s00726-003-0011-2
- Gieseg SP, Pearson J, Firth CA. Protein hydroperoxides are a major product of low density lipoprotein oxidation during copper, peroxyl radical and macrophage-mediated oxidation. Free Radic Res. 2003;37:983–991.10.1080/10715760310001603612
- Requena JR, Levine RL, Stadtman ER. Recent advances in the analysis of oxidized proteins. Amino Acids. 2003;25:221–226.10.1007/s00726-003-0012-1
- Uchida K, Toyokuni S, Nishikawa K, et al. Michael addition-type 4-hydroxy-2-nonenal adducts in modified low-density lipoproteins: markers for atherosclerosis. Biochemistry. 1994;33:12487–12494.10.1021/bi00207a016
- Itakura K, Oya-Ito T, Osawa T, et al. Detection of lipofuscin-like fluorophore in oxidized human low-density lipoprotein. 4-hydroxy-2-nonenal as a potential source of fluorescent chromophore. FEBS Lett. 2000;473:249–253.10.1016/S0014-5793(00)01539-8
- Weisgraber KH, Rall SC. Human apolipoprotein B-100 heparin-binding sites. J Biol Chem. 1987;262:11097–11103.
- Cardin AD, Weintraub HJ. Molecular modeling of protein-glycosaminoglycan interactions. Arterioscler Thromb Vasc Biol. 1989;9:21–32.10.1161/01.ATV.9.1.21
- Krisko A, Piantanida I, Kveder M, et al. The effect of heparin on structural and functional properties of low density lipoproteins. Biophys Chem. 2006;119:234–239.10.1016/j.bpc.2005.09.011
- Oorni K, Pentikainen MO, Annila A, et al. Oxidation of low density lipoprotein particles decreases their ability to bind to human aortic proteoglycans: dependence on oxidative modification of the lysine residues. J Biol Chem. 1997;272:21303–21311.10.1074/jbc.272.34.21303
- Beckman JS, Beckman TW, Chen J, et al. Apparent hydroxyl radical production by peroxynitrite: implications for endothelial injury from nitric oxide and superoxide. Proc Natl Acad Sci USA. 1990;87:1620–1624.10.1073/pnas.87.4.1620
- Rubbo H, O’Donnell V. Nitric oxide, peroxynitrite and lipoxygenase in atherogenesis: mechanistic insights. Toxicology. 2005;208:305–317.10.1016/j.tox.2004.11.019
- Guy RA, Maguire GF, Crandall I, et al. Characterization of peroxynitrite-oxidized low density lipoprotein binding to human CD36. Atherosclerosis. 2001;155:19–28.10.1016/S0021-9150(00)00524-4
- Lomonosova EE, Kirsch M, Rauen U, et al. The critical role of Hepes in SIN-1 cytotoxicity, peroxynitrite versus hydrogen peroxide. Free Radic Biol Med. 1998;24:522–528.10.1016/S0891-5849(97)00295-5
- Trostchansky A, Batthyany C, Botti H, et al. Formation of lipid-protein adducts in low-density lipoprotein by fluxes of peroxynitrite and its inhibition by nitric oxide. Arch Biochem Biophys. 2001;395:225–232.10.1006/abbi.2001.2583
- Radi R. Protein tyrosine nitration: biochemical mechanisms and structural basis of functional effects. Acc Chem Res. 2013;46:550–559.10.1021/ar300234c