Abstract
Rice powder extract (RPE) from black and brown rice (Oryza sativa L. indica) improves hepatic lipid accumulation in obese and diabetic model mice via peroxisomal fatty acid oxidation. RPE showed PPARα agonistic activity which did not differ between black and brown RPE despite a higher anthocyanin content in black RPE.
Brown rice (BR) is a functional food against type 2 diabetes mellitus (T2DM) in rice-consuming populations with various mechanisms.Citation1, 2) In recent years, the treatment of non-alcoholic fatty liver disease is considered indispensable for the prevention and management of T2DM.Citation3) BR and germinated BR extracts improve abnormalities in hepatic lipid metabolism.Citation4–6) Hepatic lipid metabolism is regulated by various factors. One of the master regulators is peroxisome proliferator-activated receptor-α (PPARα), which is activated by various food-derived compounds.7) Rice powder extract (RPE) from a black japonica rice (BL) increases the hepatic expression of PPARα.Citation4) In this study, the effects of extracts from BR and BL on hepatic lipid accumulation were examined.
The therapeutic effects of RPE against T2DM in the presence of obesity, its most common co-morbidity, were examined (how to prepare RPE is described in the Supplemental materials). For this purpose, five-week-old male KK-Ay mice (CLEA Japan, Tokyo, Japan) were used as a severely obese and diabetic model. Mice were divided into four groups (n = 8/group), after receiving a normal diet for 1 week. The groups were as follows: (1) the C group, C57BL/6 J mice (CLEA Japan, Tokyo, Japan) under adlib feeding conditions with 1% DMSO in 20 cal% fat AIN-93G (normal diet); (2) the KP group, KK-Ay mice pair-fed with 1% DMSO in the normal diet; (3) K group, KK-Ay mice under adlib feeding conditions with 1% DMSO in the normal diet; (4) BL group, KK-Ay mice fed 1% black RPE in the normal diet; and (5) BR group, KK-Ay mice fed 1% brown RPE in the normal diet. The K, BL, and BR groups, were pair-fed to adjust the energy intake of all mice, although each RPE-feeding did not change food intake. The RPE-supplemented diets were provided for 4 weeks. All mice were fasted for 5 h before organ resection. This animal experiment was approved by Animal Care Committee in Tokyo University of Agriculture (approval ID: No.280084). Materials and methods of histochemical analysis and gene expression analysis are described in Supplemental materials. Multiple comparisons were performed with Tukey’s test after one-way ANOVA. p < 0.05 was considered to be statistically significant.
Organ weights did not differ between the K, BL, and BR groups, except for liver weights (Supplemental Table 1). The liver weights were significantly higher in the BL and BR groups than in the K group. A histochemical analysis and hepatic TG measurements revealed that BL and BR RPE feeding ameliorated TG accumulation in the liver (Fig. (A) and (B)). In contrast, serum TG levels were higher in the BL and BR groups than in the K group (Fig. (C)). This might be because each RPE contained 3–4% TG (each RPE contained 1–2% TG; Felix et al. unpublished data). However, further investigation is necessary for elucidating the conflict, because the reason for the conflict is unclear. In addition, serum glucose levels did not differ among the three groups (K, BL, and BR) (Supplemental Fig. 1). To elucidate the mechanism underlying the improvement in hepatic TG accumulation, gene expression was evaluated using real-time PCR. The mRNA expression levels of acyl-CoA oxidase (AOX), a rate-limiting enzyme of peroxisomal fatty acid oxidation, were significantly higher in the BL and BR groups than in the K group. The documented increase in the mRNA expression of this fatty acid oxidation-related gene is consistent with the results of a previous report.Citation4) However, we did not observe increases in the expression levels of other fatty acid oxidation-related genes (Fig. (A)). Moreover, inhibitory effects of RPEs were not observed in the mRNA expression levels of fatty acid synthesis-related genes, such as sterol-response element-binding protein-1c (SREBP1c) and fatty acid synthase, (FAS), and pro-inflammatory genes, such as tumor necrosis factor-α (TNFα) and monocyte chemoattractant protein-1 (MCP-1). The reason why such changes in gene expression were observed is unknown. However, these results suggest, at least in part, that the RPE increased peroxisomal fatty acid oxidation and that anti-inflammatory effects of RPE demonstrated by previous reports were not involved in the decreases in hepatic TG levels under our experimental conditions. However, it has been reported that fatty acid synthesis is inhibited by feeding with germinated BR RPE.Citation4) This difference may be explained by the difference in mouse models (KK-Ay vs. C57BL/6J, a moderate obese and diabetic model). RPE is believed to contain some PPAR-α-activating compounds, which reduce hepatic TG accumulation.Citation9) PPARα activation generally enhances peroxisomal fatty acid oxidation, accompanied by liver enlargement.Citation9) To evaluate the PPARα ligand activity of each RPE, a dual luciferase assay (Promega, WI, USA) was performed in comparison to bezafibrate (Beza), a potent commercial PPARα agonist. The assay revealed that both BL and BR RPEs activated PPARα (Fig. (B)).
Fig. 1. The effects of RPE on hepatic TG accumulation.
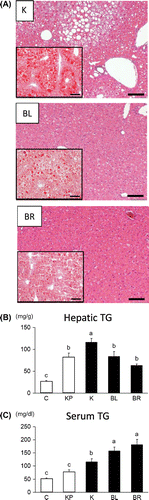
Fig. 2. Hepatic gene expression analysis and PPARα ligand assay.
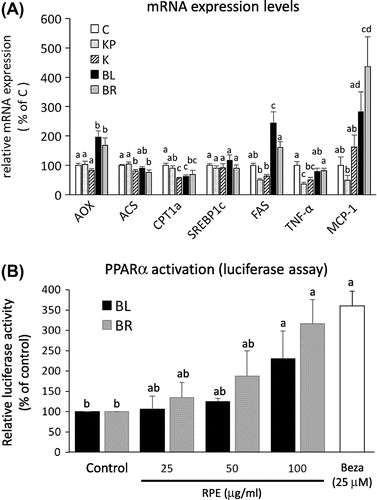
Previous studies have shown that RPE attenuates hepatic TG accumulation by various mechanisms, such as antioxidative stress and anti-inflammatory effects.Citation4–6) In the current study, we found that RPE improves hepatic lipid accumulation via the activation of PPARα. This proposed mechanism is based on the observed liver enlargement, the increase in AOX, and the results of the luciferase assay. Contrary to the results of a previous study demonstrating PPARα-agonistic activity of cyanidin,Citation4) we did not observe any difference in the PPARα agonist activities of BL and BR RPEs, despite the higher cyanidin content of BL RPE (147.6 vs. 2.98 mg in 100 g for BR RPE; A Felix, unpublished data). It is likely that another compound has PPARα agonistic activity in RPE or that RPE contains compounds that modify the activity. Oryzanol and ferulic acid are strong candidates, since the two compounds alleviate metabolic syndrome parameters in rats fed a high-fat and high-fructose diet.Citation9) However, the interaction of these two compounds and PPARα reminds unclear. To the best of our knowledge, this is the first study to report the PPARα agonistic activities in RPE, both in vivo and in vitro, although further studies are needed to test this hypothesis and to establish the underlying mechanism of action.
Overall, our study revealed that RPE can improve hepatic liver accumulation via the activation of PPARα in an obese and diabetic mouse model. This is particularly relevant because diets in rice-consuming, low-to-middle income societies have shifted toward higher fat contents, and the incidences of diseases related to lipid metabolism have increased dramatically, in parallel to the increases in T2DM and obesity.
Author contributions
A.F. and N.T. designed the experiments. A.F., M.T., R.K.T., R.S., and S.H.T. performed experiments. All authors discussed the data. A.F., N.T. and M.U. wrote the paper.
Disclosure statement
No potential conflict of interest was reported by the authors.
Funding
This work was supported by Grants-in-Aid for Scientific Research from the Ministry of Education, Culture, Sport, Science and Technology of Japan [grant number 15H01767], [grant number 15K07441].
Supplemental material
The supplementary material for this article is available online at https://doi.org/10.1080/09168451.2017.1372178.
Final_Manuscript__BBB-170245.R1__Supplements.doc
Download MS Word (99.5 KB)Acknowledgments
The authors thank Kaho Inagaki, Shiho Kanie, Yuto Yokota, Miwa Kokubo, and Mutsumi Sato for technical assistance.
Notes
Abbreviations: RPE, rice powder extract; TG, triacylglycerol; PPARα, peroxisome proliferator-activated receptor-α.
References
- Goufo P, Trindade H. Rice antioxidants: phenolic acids, flavonoids, anthocyanins, proanthocyanidins, tocopherols, tocotrienols, γ-oryzanol and phytic acid. Food Sci Nutr. 2014;2:75–104.10.1002/fsn3.86
- Imam M, Azmi N, Bhanger M, et al. Antidiabetic properties of germinated brown rice: a systematic review. Evid Based Complement Alternat Med. 2012. DOI:10.1155/2012:816501
- Lonardo A, Ballestri S, Marchesini G, et al. Nonalcoholic fatty liver disease: A precursor of the metabolic syndrome. Dig Liver Dis. 2015;47:181–190.10.1016/j.dld.2014.09.020
- Jang HH, Park MY, Kim HW, et al. Black rice extract attenuates hepatic steatosis in C57BL/6J mice fed a high-fat diet via fatty acid oxidation. Nutr Metab. 2012;9:27.10.1186/1743-7075-9-27
- Shen KP, Hao CL, Yen HW, et al. Pre-germinated brown rice prevented high fat diet induced hyperlipidemia through ameliorating lipid synthesis and metabolism in C57BL/6J mice. J Clin Biochem Nutr. 2016;59:39–44.10.3164/jcbn.15-117
- Ho JN, Son ME, Lim WC, et al. Anti-obesity effects of germinated brown rice extract through down-regulation of lipogenic genes in high fat diet-induced obese mice. Biosci Biotechnol Biochem. 2012;76:1068–1074.10.1271/bbb.110666
- Georgiadi A, Kersten S. Mechanisms of gene regulation by fatty acids. Adv Nutr. 2012;3:127–134.10.3945/an.111.001602
- Goto T, Kim YI, Takahashi N, et al. Natural compounds regulate energy metabolism by the modulating the activity of lipid-sensing nuclear receptors. Mol Nutr Food Res. 2013;57:20–33.10.1002/mnfr.v57.1
- Wang O, Liu J, Cheng Q, et al. Effects of ferulic acid and γ-oryzanol on high-fat and high fructose diet-induced metabolic syndrome in rats. PLoS ONE. 2015;10:e0118135.