Abstract
Inhibition of excessive fructose intake in the small intestine could alleviate fructose-induced diseases such as hypertension and non-alcoholic fatty liver disease. We examined the effect of phytochemicals on fructose uptake using human intestinal epithelial-like Caco-2 cells which express the fructose transporter, GLUT5. Among 35 phytochemicals tested, five, including nobiletin and epicatechin gallate (ECg), markedly inhibited fructose uptake. Nobiletin and ECg also inhibited the uptake of glucose but not of L-leucine or Gly-Sar, suggesting an inhibitory effect specific to monosaccharide transporters. Kinetic analysis further suggested that this reduction in fructose uptake was associated with a decrease in the apparent number of cell-surface GLUT5 molecules, and not with a change in the affinity of GLUT5 for fructose. Lastly, nobiletin and ECg suppressed the permeation of fructose across Caco-2 cell monolayers. These findings suggest that nobiletin and ECg are good candidates for preventing diseases caused by excessive fructose intake.
Graphical abstract
Nobiletin and ECg inhibited fructose uptake in human intestinal epithelial-like Caco-2 cells presumably by affecting GLUT5.
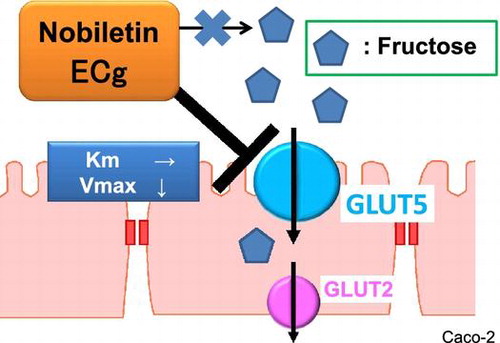
Abbreviations:
- ECg, epicatechin gallate
- EDTA, ethylenediaminetetraacetic acid
- HFCS, high-fructose corn syrup
- NAFLD, non-alcoholic fatty liver disease
- GLUT5, glucose transporter 5
- C, catechin
- EC, epicatechin
- Cg, catechin gallate
- GC, gallocatechin
- EGC, epigallocatechin
- GCg, gallocatechin gallate
- EGCg, epigallocatechin gallate
- PBS, phosphate-buffered saline
- MeCN, acetonitrile
- Gly-Sar, glycylsarcosine
- PepT1, peptide transporter 1
- TMF, trimethoxyflavone
- ELE, eucalyptus leaf extract
The incidence of metabolic syndrome has been increasing in recent decades, and the disorder is now recognized as a global and social problem. In particular, the prevalence of obesity has markedly increased globally, which is presumably due to excessive nutrient and energy intake, and its prevention or treatment has become a major public health concern. In terms of the consumption of nutrients, an increase in fructose intake is considered to be one of the major causes of obesity [Citation1,2]. Fructose is contained in sweet natural food substances such as fruits and honey, and because fructose exhibits the strongest sweetness property among dietary monosaccharides, it is commonly used as a sweetener in processed sweet foods and beverages. For example, high-fructose corn syrup (HFCS), which is generated through the isomerization of glucose, has been frequently used for preparing sweet foods and beverages. HFCS has been widely used in the food industry in the USA since the 1970s because it provides a strong sweet flavor and is relatively inexpensive. In 1977, the fructose intake per person in the USA was reported to be 37.0 g/day, accounting for 8.0% of the daily calorie consumption [Citation3]. However, in 2006, the fructose intake increased to 54.7 g/day, accounting for 10.2% of the total daily calorie intake [Citation3]. In Japan, HFCS use in sweet foods or soft drinks has been increasing, and the current demand for HFCS is approximately one-fourth that for sugar in soft drinks.
Fructose is more lipogenic than glucose, and the concentration of triacylglycerols in serum and hepatocytes is markedly elevated after consumption of diets containing 40–60% fructose [Citation4]. Increased fructose consumption is regarded as one of the risk factors contributing to the development of obesity and metabolic syndrome, which precedes the symptoms associated with insulin resistance, impaired glucose homeostasis, hypertension, and non-alcoholic fatty liver disease (NAFLD) [Citation2,5,6].
Intestinal absorption of fructose is widely recognized to be mediated by glucose transporter 5 (GLUT5; SLC2A5) expressed at the apical surface of intestinal epithelial cells [Citation6,7]. Although GLUT5 is one of the glucose transporters expressed by cells, it transports only fructose and not glucose or galactose. GLUT5 is highly expressed in the intestines, particularly in the jejunum, as well as in other organs such as the testis, kidney, muscle, adipose tissue, and brain [Citation7–9]. Phenotypic analysis of Glut5 knockout mice revealed that fructose absorption in the intestines was decreased by 75% relative to that in wild-type mice, and the plasma fructose concentration was also decreased by 90% [Citation10]. This strongly suggests that GLUT5 is mainly responsible for fructose absorption in the intestines. Therefore, inhibition of GLUT5 activity using dietary substances could offer an effective method for preventing fructose-induced diseases such as metabolic syndrome, hypertension, obesity, and NAFLD. However, little information is available regarding the food ingredients that could suppress intestinal fructose absorption, except for recent reports on a Eucalyptus globulus leaf extract and certain culinary plant extracts [Citation11,12].
In this study, we examined how phytochemicals, which represent one family of food substances, affect fructose uptake in human intestinal epithelial-like Caco-2 cells. The Caco-2 cell line spontaneously differentiates and exhibits various enterocytic characteristics, expressing functional molecules such as brush-border membrane enzymes and nutrient transporters, including GLUT5 [Citation13]. Furthermore, we examined the effect of phytochemicals on the transepithelial transport of fructose using Caco-2 cell monolayers cultured on semi-permeable membranes.
Materials and methods
Phytochemicals
The following phytochemicals were tested: myricetin, morin, puerarin, diosmin, apigenin, flavonol, flavone, hesperetin, genistein, daidzein, naringin, naringenin, caffeine, catechin (C), epicatechin (EC), catechin gallate (Cg), epicatechin gallate (ECg), gallocatechin (GC), epigallocatechin (EGC), gallocatechin gallate (GCg), and epigallocatechin gallate (EGCg) (Sigma, St. Louis, MO, USA); rutin, kaempferol, baicalein, flavanone, tangeretin, daidzin, nobiletin, glycitin, and glycitein (Wako pure Chemicals, Ind. Ltd., Osaka, Japan); quercitrin and quercetin (Tokyo Kasei Kogyo, Tokyo, Japan); genistin (Fujicco, Kobe, Japan); ginkgolides B and J (Tama Biochemical, Tokyo, Japan); and equol (LC laboratories, Woburn, MA, USA). All phytochemicals were >95% pure. Each phytochemical was diluted in dimethyl sulfoxide (DMSO) before use.
Cell culture
Caco-2 cells (American Type Culture Collection, Rockville, MD, USA) were cultured in 78.5-cm2 plastic dishes with a culture medium consisting of Dulbecco’s modified Eagle medium (Wako pure Chemicals, Ind. Ltd.), 10% fetal calf serum (Life Technologies, Grand Island, NY, USA), 1% non-essential amino acids (Cosmobio, Tokyo, Japan), 2% glutamine (Wako pure Chemicals, Ind. Ltd.), 100 U/mL penicillin, and 100 μg/mL streptomycin (10,000 U/mL and 10 mg/mL in 0.9% sodium chloride, respectively; Gibco, Gaithersburg, MD, USA). The cells were incubated at 37 °C in a humidified atmosphere of 5% CO2 in air and the culture medium was renewed on alternate days. When the cells reached confluence, they were passaged at a split ratio of 4 to 8 by trypsinizing with a solution containing 0.1% trypsin and 0.02% ethylenediaminetetraacetic acid (EDTA) in phosphate-buffered saline (PBS). For all experiments, the cells were used between passages 40 and 66. An accumulation experiment was performed using Caco-2 cells that had been cultured at a density of 1.0 × 105 cells/well in 24-well plates pre-coated with a type-I collagen solution (Nitta Gelatin, Osaka, Japan). The cells for the uptake experiments were used after 14 days of culture.
Fructose uptake analysis
The uptake of [3H]-fructose (specific radioactivity: 5.0 Ci/mmol; GE Healthcare, Fairfield, CT, USA) by Caco-2 cells was measured. Caco-2 cells were washed twice with 700 μL of PBS and then once with 300 μL of uptake buffer (140 mM NaCl, 0.34 mM Na2HPO4, 0.44 mM KH2PO4, 5.33 mM KCl, 1.26 mM CaCl2, 0.49 mM MgCl2, 0.41 mM MgSO4, and 4.16 mM NaHCO3; pH adjusted to 7.4 with KOH). The cells were next incubated at 37 °C for 10 min with 200 nM [3H]-fructose (1 mCi/L) in 300 μL of the uptake buffer, with or without excess (500 mM) fructose. After incubation, the buffer was removed and the cells in each well were carefully washed thrice with 700 μL of ice-cold PBS containing 0.05% sodium azide. Subsequently, 250 μL 0.1% Triton X-100 was added to each well and the dissolved cells were collected into 3 mL of a scintillation cocktail, and then the tritium content of the cells from each well was measured using an LSC 5100 liquid scintillation analyzer (Aloka, Tokyo, Japan). We also investigated the uptake of glucose, L-leucine (L-Leu), or glycylsarcosine (Gly-Sar), using [3H]-glucose (21.2 Ci/mmol; GE Healthcare, Fairfield, CT, USA), [3H]-L-leucine (142 Ci/mmol; GE Healthcare), and [3H]-glycylsarcosine (0.2 Ci/mmol; Moravek, Brea, CA, USA), respectively.
Nobiletin and ECg uptake analysis
Caco-2 cells cultured in 24-well plastic plates for 14 days were washed with uptake buffer twice and then incubated with uptake buffer at 37 °C for 10 min. Next, the uptake buffer was removed, and 300 μL of 100 μM nobiletin or 1 mM ECg in uptake buffer was added to each well and incubated at 37 °C for 10 min or 6 h, respectively. After the incubation, the buffer was removed and the cells in each well were carefully washed thrice with 700 μL of ice-cold PBS containing 0.05% sodium azide, and then the cells were scraped with a cell scraper and cell lysates were extracted using methanol. After vigorous mixing, the lysates were centrifuged at 14,000 × g for 30 min, the supernatant was recovered and evaporated (dried), and then dissolved in the 50% acetonitrile (MeCN) solution that was used for measuring nobiletin and ECg concentrations in the cell extracts using high-performance liquid chromatography (HPLC).
HPLC analysis of nobiletin
A Gulliver HPLC system (Jasco International Co., Tokyo, Japan) was used consisting of a PU-2080 Plus intelligent HPLC pump, a UV-1575 intelligent UV–vis detector, and an 807-IT integrator. Chromatographic separation was performed in a C18 column (Cosmosil 5C18-MS-II, 4.6 × 150 mm, Nacalai Tesque, Kyoto, Japan). The mobile phase was a 50% MeCN solution containing 0.1% acetic acid, applied at a flow rate of 1.0 mL/min at 35 °C. Nobiletin was monitored based on its absorbance at 280 nm, and its concentration was determined from the peak area.
HPLC analysis of ECg
For ECg analysis, we used an HPLC system consisting of an LC-20AD pump, an SIL-20A autosampler, and a CTO-10AS column oven (all three from Shimadzu Co., Kyoto, Japan), along with a Coulochem III electrochemical detector featuring a model 5020 guard cell and a model 5011 analytical cell (ESA Inc., Bedford, MA, USA) and a computer equipped with Chromato-Pro version 4 chromatography data integrator (Runtime Instruments, Ltd., Tokyo, Japan). Chromatographic separation was performed in a C18 column (Capcellpak AQ, 4.6 × 150 mm, Shiseido Co. Ltd, Tokyo, Japan). The mobile phase was a 0.1 M sodium phosphate buffer containing 0.5 mM Na2EDTA (pH 3.5)/MeCN (85/15, v/v), used at a flow rate of 0.8 mL/min at 40 °C. The applied voltage of the analytical cell was set at -200 mV for electrode 1,+200 mV for electrode 2, and +250 mV for the guard cell. Ethyl gallate was used as an internal standard for HPLC analysis of ECg.
Fructose permeability analysis
Caco-2 cells were cultured on membrane cell inserts for 14 days to evaluate permeation. 3H-fructose was added to the apical side, and the membrane was incubated at 37 °C for 10 min. Then, the basal solution was recovered from each well and the tritium content in the basal solution was measured using an LSC 5100 liquid scintillation analyzer.
Statistical analyses
All data presented are the means ± standard error of the mean values of ≥3 independent experiments performed in triplicate. Statistical comparisons were performed using Student’s t-test or Tukey’s test; differences were considered significant at p < 0.01.
Results
Effect of phytochemicals on fructose uptake in human intestinal epithelial Caco-2 cells
We examined the effects of 35 distinct phytochemicals on fructose uptake in human intestinal epithelial-like Caco-2 cells (Figure ). Five phytochemicals, kaempferol, nobiletin, apigenin, tangeretin, and ECg, significantly inhibited fructose uptake in these cells. Especially, nobiletin, tangeretin, and ECg reduced the uptake to 60% of the control level. Because nobiletin and tangeretin are structurally similar and because nobiletin inhibited fructose uptake slightly more potently than tangeretin did, we focused on nobiletin, together with ECg, in further analyses. The results of dose-response assays showed that nobiletin (Figure (A)) and ECg (Figure (B)) suppressed fructose uptake by Caco-2 cells in a concentration-dependent manner (0–150 μM).
Figure 1. Effect of phytochemicals on fructose uptake by Caco-2 cells. Fructose uptake was measured in the absence or presence of 10 μM each phytochemical at 37 °C for 10 min as described in the Materials and Methods. The relative fructose uptake activity measured for Caco-2 cells treated with the vehicle (dimethyl sulfoxide) was taken as 100%. The values shown are means ± SEM (n = 3); * p < 0.01 vs. the control value (Student’s t-test).
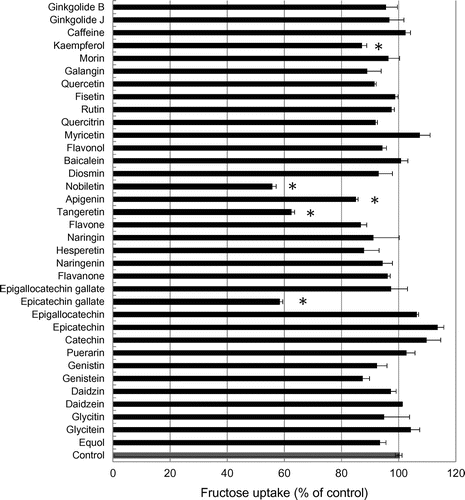
Figure 2. Dose-dependent inhibition of fructose uptake by nobiletin and ECg in Caco-2 cells. Fructose uptake was measured in the absence or presence of 0–150 μM nobiletin (A) or ECg (B) at 37 °C for 10 min as described in the Materials and Methods. The values shown are means ± SEM (n = 3), and the values indicated by different letters are significantly different from each other (Tukey’s test; p < 0.01).
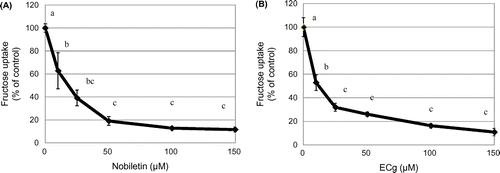
Effect of nobiletin and ECg on the uptake activity of nutrient transporters in Caco-2 cells
We investigated the effect of nobiletin and ECg on the uptake of [3H]-glucose (50 nM, 1.06 mCi/L), [3H]-L-leucine (L-Leu) (6.25 nM, 0.888 mCi/L), and [3H]-glycylsarcosine (Gly-Sar) (50 μM, 10 mCi/L), a typical substrate of peptide transporter 1 (PepT1) [Citation14,15]. Although nobiletin suppressed the uptake of both glucose and fructose, it did not markedly affect the uptake of either the amino acid (L-Leu) or peptide (Gly-Sar) (Figure (A)). ECg also produced similar inhibitory effects on the uptake activity of the cells (Figure (B)). These results suggest that nobiletin and ECg selectively inhibit the transporter activity of monosaccharide transporters.
Figure 3. Effect of nobiletin and ECg on the uptake activity of nutrient transporters in Caco-2 cells. The uptake of fructose, glucose, L-leucine, and Gly-Sar was measured in the absence or presence of 25 μM nobiletin (A) or ECg (B) at 37 °C for 10 min. The values shown are means ± SEM (n = 3); *p < 0.01 vs. the control value (Student’s t -test).
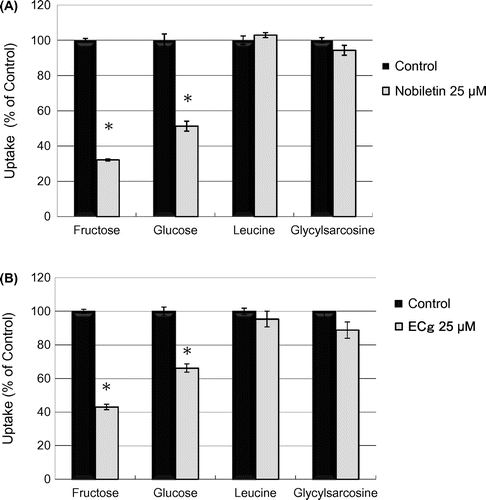
Effects of phytochemicals with a nobiletin-like structure on fructose uptake in Caco-2 cells
To examine the structural characteristics of nobiletin that affect its inhibition of fructose uptake by Caco-2 cells, we compared the cellular fructose uptake in the presence of phytochemicals with similar structures, including nobiletin, tangeretin, sinensetin, and hesperetin at 25 μM each (Figure (A)). As shown in Figure (B), nobiletin, tangeretin, and sinensetin all reduced fructose uptake to 40% of the control level.
Figure 4. Chemical structures of phytochemicals featuring a nobiletin-like structure (A) and the effect of these phytochemicals on fructose uptake by Caco-2 cells (B). Fructose uptake was measured in the absence or presence of 25 μM nobiletin, tangeretin, sinensetin, or hesperetin at 37 °C for 10 min as described in the Materials and Methods. The values shown are means ± SEM (n = 3), and the values indicated by different letters are significantly different from each other (Tukey’s test; p < 0.01).
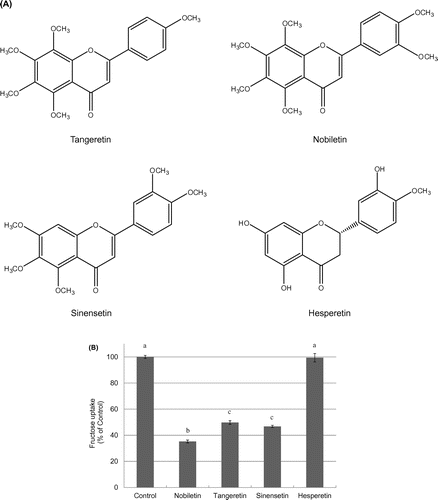
Effect of catechins on fructose uptake by Caco-2 cells
We also compared the effects of eight distinct catechins (C, EC, Cg, ECg, GC, EGC, GCg, and EGCg) on fructose uptake; their structures are shown in Figure (A). At a concentration of 25 μM, ECg, and Cg significantly and markedly suppressed fructose uptake, but C, EC, GC, EGC, GCg, and EGCg produced no statistically significant effect on the uptake (Figure (B)). These results indicate that the inhibitory effect of catechins on fructose uptake was dependent on the specific catechin structure.
Figure 5. Chemical structure of catechins (A) and effect of catechins on fructose uptake by Caco-2 cells (B). Fructose uptake was measured in the absence or presence of eight catechins (used at 25 μM) at 37 °C for 10 min as described in the Materials and Methods. The values shown are means ± SEM (n = 3), and the values indicated by different letters are significantly different from each other (Tukey’s test; p < 0.01).
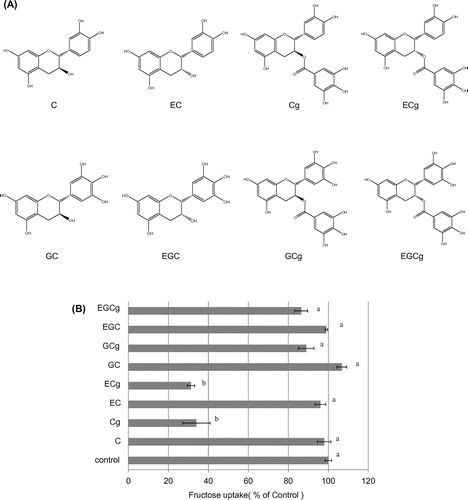
Effect of fructose on nobiletin and ECg uptake by Caco-2 cells
Next, we examined how fructose (0, 50, and 100 mM) affected the uptake of nobiletin or ECg by Caco-2 cells, as determined with HPLC. Fructose did not significantly inhibit the uptake of either nobiletin (Figure (A)) or ECg (Figure (B)) by Caco-2 cells, suggesting that nobiletin and ECg are not substrates of GLUT5. These results also indicate that direct binding between nobiletin/ECg and fructose does not account for their inhibitory effects on fructose uptake by Caco-2 cells.
Figure 6. Effect of fructose on nobiletin and ECg uptake by Caco-2 cells. Caco-2 cells were cultured in 24-well plates for 14 days, after which 100 μM nobiletin or 1 mM ECg was added to the cells and then nobiletin/ECg uptake was measured in the presence of 0, 50, and 100 mM fructose at 37 °C for 10 min or 6 h. The values shown are means ± SEM (n = 3).
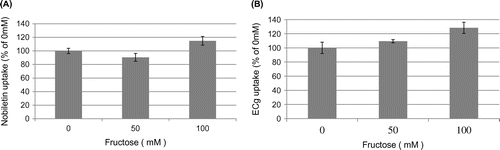
Kinetic analysis of fructose uptake by Caco-2 cells in the presence of nobiletin or ECg
To further understand how nobiletin and ECg exert their inhibitory effects, we performed kinetic analyses of fructose uptake in the absence or presence of 25 μM nobiletin (Figure (A)) or ECg (Figure (B)). Lineweaver-Burk plots were constructed to calculate the maximal velocity (Vmax) and Km values for fructose uptake in the absence or presence of 25 μM nobiletin (Figure (C)) or ECg (Figure (D)). The average Vmax in the presence of nobiletin or ECg was considerably lower than that measured for the control cells, whereas the Km value was not markedly altered by the inhibitors (Table ). Our results suggest that the inhibition of fructose uptake by nobiletin and ECg was mainly due to a decrease in the Vmax value.
Figure 7. Kinetic analysis of fructose uptake in the absence or presence of nobiletin (A) or ECg (B). Fructose uptake was measured over the concentration range 5–200 mM fructose in the absence or presence of 25 μM nobiletin (A) or ECg (B) at 37 °C for 10 min. The values shown are means ± SEM (n = 3). Lineweaver-Burk plots were constructed to calculate the Vmax and Km values for fructose uptake in the absence or presence of 25 μM nobiletin (C) or ECg (D).
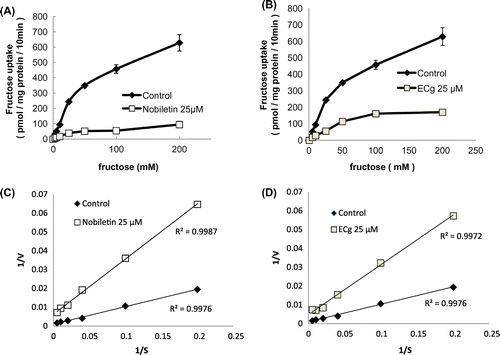
Table 1. Kinetic analysis of fructose uptake in the absence or presence of nobiletin or ECg.
Effect of nobiletin and ECg on fructose permeability through Caco-2 cell monolayers
Finally, we examined whether the phytochemicals that inhibit fructose uptake in human epithelial cells might also affect fructose permeation across cell monolayers. Both nobiletin (Figure (A)) and ECg (Figure (B)) significantly inhibited the fructose permeation in a dose-dependent manner. This result suggests that nobiletin and ECg suppressed not only the uptake of fructose into intestinal epithelial cells but also the transepithelial transport of fructose across intestinal epithelial cell monolayers.
Figure 8. Effect of nobiletin and ECg on the transepithelial transport of fructose across Caco-2 cell monolayers. Caco-2 cells were cultured on membrane cell inserts for 14 days and used for transport experiments, in which 3H-fructose was added to the apical side together without or with nobiletin or ECg at the indicated concentrations and incubated at 37 °C. After 10-min incubation, the basal solution was recovered and the fructose content was measured. The values shown are means ± SEM (n = 3), and the values indicated by different letters are significantly different from each other (Tukey’s test; p < 0.01).
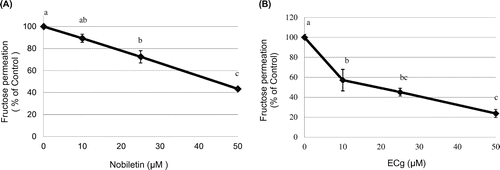
Discussion
In this study, we examined the effects of phytochemicals on fructose uptake in human intestinal epithelial-like Caco-2 cells. Five of the 35 phytochemicals screened strongly inhibited fructose uptake; among these, nobiletin and tangeretin, which are typical methoxyflavonoids, and ECg inhibited the uptake substantially and in a dose-dependent manner. Kinetic analysis revealed that the inhibition of fructose uptake by nobiletin and ECg was mainly due to a decrease in the apparent number of transporters and not due to a change in the transporter’s affinity for fructose. We further showed that fructose permeation across Caco-2 cell monolayers was potently suppressed by nobiletin and ECg. Because intestinal epithelial cells are continuously exposed to high concentrations of dietary substances, dietary phytochemicals such as nobiletin and ECg could potentially modulate the intestinal absorption of fructose by inhibiting GLUT5 activity.
Phytochemicals are a complex group of low-molecular-weight chemical compounds that are widely distributed throughout the plant kingdom and thus form an integral part of the human diet [Citation16,17]. Polyphenols are major members of the phytochemical family, and the functions of polyphenols have attracted considerable research interest in recent decades [Citation17]. Nobiletin (3′,4′,5,6,7,8-hexamethoxyflavone) and tangeretin (4′,5,6,7,8-pentamethoxyflavone), which are natural polyphenolic compounds that are categorized as flavonoids, are primarily found in citrus fruits such as those of Citrus unshiu, C. depressa, C. tangerina, and C. hassaku. Because these compounds contain methoxy residues in a flavone frame in the place of hydroxyl residues, these flavonoids are also called methoxyflavonoids [Citation18,19].
In recent years, diverse physiological functions of methoxyflavonoids have been reported [Citation18,19]. The compounds produced by methoxyflavonoids are widely recognized to show anti-inflammatory and anti-tumor effects [Citation20–23]. Although C. unshiu contains several bioactive components, including auraptene, β-cryptoxanthin, and limonine, methoxyflavonoids such as nobiletin, tangeretin, and sinensetin are considered to contribute substantially to some of its well-known biological functions [Citation24]. The present finding that nobiletin and tangeretin suppress intestinal fructose uptake provides new insight into the physiological functions of methoxyflavonoids.
We further found that trimethoxyflavone (TMF) [Citation25], a synthetic compound containing three methyl groups, also significantly inhibited fructose uptake, but to a considerably lesser extent than observed with nobiletin and tangeretin (data not shown). Together, these results suggest that the presence of several methoxy residues is critical for the ability of a compound to inhibit fructose uptake, although the relationship between the inhibitory activity and the position of methylation remains to be elucidated. Currently, there are no commercially available compounds that are structurally related to nobiletin and tangeretin; thus, detailed analysis of the structure-activity relationship requires preparation of a chemical compound library of methoxyflavonoids.
Catechins, one of the most widely recognized groups of functional phytochemicals, are categorized as flavonoids. Our results showed that galloyl catechins such as ECg and Cg markedly inhibited fructose uptake by Caco-2 cells. Therefore, we also examined the effect of gallic acid on fructose uptake, which did not itself inhibit the uptake (data not shown). This suggests that the basic catechin structure, together with the gallate residue, is essential for the inhibitory activity. Our results also suggest that the hydroxyl group at the 5′-B-ring of ECg and Cg interferes with the inhibition of fructose uptake. By contrast, the difference between the epi-form and non-epi-form did not influence the inhibitory activity of the catechins.
The physiological functions of catechins, such as their inhibition of carcinogenesis and microbial infection, have been extensively investigated [Citation26,27], and EGCg, the most abundant catechin in green tea, is typically regarded as the most biologically active catechin [Citation28,29]. Intriguingly, in the present study, the fructose uptake activity was not suppressed upon treatment with 25 μM EGCg, whereas ECg at 25 μM potently inhibited the activity. However, EGCg inhibited uptake activity when applied at 50 μM (data not shown), and thus it also shows potential to inhibit fructose uptake, although this inhibitory activity is considerably weaker than that of ECg. This difference between result showing ECg and EGCg might represent a rare case. The higher sensitivity of GLUT5 to ECg than EGCg is unexpected and previously unrecognized, particularly from the viewpoint of catechin research.
We found that nobiletin and ECg inhibited fructose uptake in a dose-dependent manner, which suggested that these flavonoids might also act as substrates of GLUT5 and thus competitively inhibit fructose uptake by GLUT5. However, HPLC analysis indicated no difference in the intracellular uptake of nobiletin and ECg in the absence or presence of fructose, indicating that neither flavonoid is a GLUT5 substrate.
The results of kinetic analyses showed that the Vmax of fructose uptake was substantially decreased following nobiletin or ECg treatment, but the Km was not altered markedly. This suggests that nobiletin and ECg cause a reduction in fructose uptake by decreasing the number of cell-surface GLUT5 molecules, but not by changing the affinity of the transporter for fructose. In these experiments, Caco-2 cells were incubated with nobiletin for only 10 min, and thus nobiletin treatment is unlikely to have changed the expression level of GLUT5; we suggest instead that the number of active (functional) GLUT5 molecules was decreased through the interaction with nobiletin. Furthermore, we cannot rule out the possibility that nobiletin and ECg suppress GLUT5 activity by altering cell-membrane characteristics. To reveal the molecular underpinnings of the mechanism by which nobiletin and ECg inhibit fructose uptake, the behaviors of these compounds, including their direct interaction with the fructose-binding domain of GLUT5, must be comprehensively analyzed in future studies.
With regard to the food substances that inhibit fructose uptake, Sugimoto et al. [Citation11] reported that a eucalyptus leaf extract (ELE) inhibited intestinal fructose absorption and suppressed dietary sucrose-induced adiposity in rats. The same group also reported that ELE suppressed the postprandial elevation of portal, cardiac, and peripheral fructose concentrations after sucrose ingestion in rats [Citation30]. Although the active compounds of ELE remain to be identified, the approach of analyzing food ingredients to determine which of these compounds inhibit intestinal fructose absorption is a promising method for finding new targets for preventing or treating disorders induced by excessive fructose intake. We are also currently preparing in vivo studies to examine whether nobiletin and ECg inhibit fructose absorption in the small intestine and determine the therapeutic potential of these phytochemicals.
In conclusion, we have provided the first demonstration that nobiletin and ECg inhibit fructose uptake by human intestinal epithelial Caco-2 cells as well as the fructose permeability across Caco-2 cell monolayers. These new findings should facilitate the development of methods to prevent or treat diseases caused by excessive fructose intake, such as metabolic syndrome, obesity, hypertension, and NAFLD.
Author contribution
H.S. designed the study, performed the experiment and wrote the manuscript. S.A. performed the experiment and analyzed data. T.U. performed the experiment and wrote the manuscript. M.S. contributed to the development of the manuscript.
Funding
This work was supported by a Grant-in-Aid for Scientific Research (C) from the Ministry of Education, Culture, Sports Science and Technology (MEXT) [grant number 24580176]; The Foundation for Dietary Scientific Research.
Disclosure statement
No potential conflict of interest was reported by the authors.
References
- Bray GA. How bad is fructose? Am J Clin Nutr. 2007;86:895–896.
- Tappy L, Le KA. Metabolic effects of fructose and the worldwide increase in obesity. Physiol Rev. 2010;90:23–46.10.1152/physrev.00019.2009
- Vos MB, Kimmons JE, Gillespie C, et al. Dietary fructose consumption among US children and adults: the Third National Health and Nutrition Examination Survey. Medscape J Med. 2008;10(7):160.
- Marriott BP, Cole N, Lee E. National estimates of dietary fructose intake increased from 1977 to 2004 in the United States. J Nutr. 2009;139(6):1228S–1235S.10.3945/jn.108.098277
- Dekker MJ, Su Q, Baker C, et al. Fructose: a highly lipogenic nutrient implicated in insulin resistance, hepatic steatosis, and the metabolic syndrome. Am J Physiol Endocrinol Metab. 2010;299:E685–E694.10.1152/ajpendo.00283.2010
- Douard V, Ferraris RP. Regulation of the fructose transporter GLUT5 in health and disease. Am J Physiol Endocrinol Metab. 2008;295:E227–E237.10.1152/ajpendo.90245.2008
- Douard V, Ferraris RP. The role of fructose transporters in diseases linked to excessive fructose intake. J Physiol. 2013;591(2):401–414.10.1113/jphysiol.2011.215731
- Corpe CP, Bovelander FJ, Munoz CM, et al. Cloning and functional characterization of the mouse fructose transporter, GLUT5. Biochim Biophys Acta. 2002;1576(1–2):191–197.10.1016/S0167-4781(02)00284-1
- Crouzoulon G, Korieh A. Fructose transport by rat intestinal brush border membrane vesicles. Effect of high fructose diet followed by return to standard diet. Comp Biochem Physiol A Comp Physiol. 1991;100(1):175–182.10.1016/0300-9629(91)90203-O
- Barone S, Fussell SL, Singh AK, et al. Slc2a5 (Glut5) is essential for the absorption of fructose in the intestine and generation of fructose-induced hypertension. J Biol Chem. 2009;284(8):5056–5066.10.1074/jbc.M808128200
- Sugimoto K, Suzuki J, Nakagawa K, et al. Eucalyptus leaf extract inhibits intestinal fructose absorption, and suppresses adiposity due to dietary sucrose in rats. Brit J Nutr. 2005;93:957–963.10.1079/BJN20051436
- Lee Y, Lim Y, Kwon O. Selected phytochemicals and culinary plant extracts inhibit fructose uptake in Caco-2 cells. Molecules. 2015;20:17393–17404.10.3390/molecules200917393
- Hidalgo IJ, Raub TJ, Borchardt RT. Characterization of the human colon carcinoma cell line (Caco-2) as a model system for intestinal epithelial permeability. Gastroenterology. 1989;96(3):736–749.10.1016/S0016-5085(89)80072-1
- Miguel M, Dávalos A, Manso MA, et al. Transepithelial transport across Caco-2 cell monolayers of antihypertensive egg-derived peptides. PepT1-mediated flux of Tyr-Pro-Ile. Mol Nutr Food Res. 2008;52(12):1507–1513.10.1002/mnfr.v52:12
- Fraga S, Pinho MJ, Soares-da-Silva P. Expression of LAT1 and LAT2 amino acid transporters in human and rat intestinal epithelial cells. Amino Acids. 2005;29(3):229–233.10.1007/s00726-005-0221-x
- Lindsay DG. The nutritional enhancement of plant foods in Europe “NEODIET”. Trends Food Sci Technol. 2000;11:145–151.10.1016/S0924-2244(00)00048-0
- Harborne JB, Williams CA. Advances in flavonoid research since 1992. Phytochemistry. 2000;55:481–504.10.1016/S0031-9422(00)00235-1
- Meiyanto E, Hermawan A. Natural products for cancer-targeted therapy: citrus flavonoids as potent chemopreventive agents. Asian Pac J Cancer Prev. 2012;13(2):427–436.10.7314/APJCP.2012.13.2.427
- Assini JM, Mulvihill EE, Huff MW. Citrus flavonoids and lipid metabolism. Curr Opin Lipidol. 2013;24(1):34–40.10.1097/MOL.0b013e32835c07fd
- Miyata Y, Sato T, Yano M, et al. Activation of protein kinase C II/-c-Jun NH2-terminal kinase pathway and inhibition of mitogen-activated protein/extracellular signal-regulated kinase 1/2 phosphorylation in antitumor invasive activity induced by the polymethoxy flavonoid, nobiletin. Mol Cancer Ther. 2004;3:839–847.
- Sato T, Koike L, Miyata Y, et al. Inhibition of activator protein-1 binding activity and phosphatidylinositol 3-kinase pathway by nobiletin, a polymethoxy flavonoid, results in augmentation of tissue inhibitor of metalloproteinases-1 production and suppression of production of matrix metalloproteinases-1 and -9 in human fibrosarcoma HT-1080 cells. Cancer Res. 2002;62:1025–1029.
- Wu YQ, Zhou CH, Tao J, et al. Antagonistic effects of nobiletin, a polymethoxyflavonoid, on eosinophilic airway inflammation of asthmatic rats and relevant mechanisms. Life Sci. 2006;78:2689–2696.10.1016/j.lfs.2005.10.029
- Satsu H, Hiura Y, Mochizuki K, et al. Activation of pregnane X receptor and induction of MDR1 by dietary phytochemicals. J Agric Food Chem. 2008;56(13):5366–5373.10.1021/jf073350e
- Tanabe S, Kinuta Y, Yasumatsu H, et al. Effects of citrus unshiu powder on the cytokine balance in peripheral blood mononuclear cells of patients with seasonal allergic rhinitis to pollen. Biosci Biotechnol Biochem. 2007;71:2852–2855.10.1271/bbb.70397
- Murray IA, Flaveny CA, DiNatale BC, et al. Antagonism of aryl hydrocarbon receptor signaling by 6,2′,4′-trimethoxyflavone. J Pharmacol Exp Ther. 2010;332(1):135–144.10.1124/jpet.109.158261
- Yang CS, Wang H. Cancer preventive activities of tea catechins. Molecules. 2016;21(12):pii:E1679.
- Ide K, Kawasaki Y, Kawakami K, et al. Anti-influenza virus effects of catechins: a molecular and clinical review. Curr Med Chem. 2016;23(42):4773–4783.10.2174/0929867324666161123091010
- Chakrawarti L, Agrawal R, Dang S, et al. Therapeutic effects of EGCG: a patent review. Expert Opin Ther Pat. 2016;26(8):907–916.10.1080/13543776.2016.1203419
- Chowdhury A, Sarkar J, Chakraborti T, et al. Protective role of epigallocatechin-3-gallate in health and disease: a perspective. Biomed Pharmacother. 2016;78:50–59.10.1016/j.biopha.2015.12.013
- Sugimoto K, Hosotani T, Kawasaki T, et al. Eucalyptus leaf extract suppresses the postprandial elevation of portal, cardiac and peripheral fructose concentrations after sucrose ingestion in rats. J Clin Biochem Nutr. 2010;46(3):205–211.10.3164/jcbn.09-93