Abstract
Administration of alpha-glycerophosphocholine (GPC), a choline compound in food, is expected to contribute to human health. In this study, we evaluated its effect on aging in senescence-accelerated mouse prone 8 (SAMP8) mice. Male SAMP8 mice had free access to a commercial stock diet and drinking water with or without GPC (0.07 mg/ml). Mice in the GPC group had significantly lower total senescence grading score than that of the control group at 36 weeks of age. Administration of GPC decreased the deposition of transthyretin (TTR), an amyloidogenic protein, in the brain. Aggregated TTR activated microglia and led to neuroinflammation. Thus, GPC would protect the brain by reducing TTR deposition and preventing neuroinflammation. In a histological study of knee joints, it was found that SAMP8 mice administered GPC showed decreased joint degeneration. These results suggest that GPC delays the aging process and may be a useful compound in anti-aging functional food development.
Graphical abstract
Transthyretin (TTR) deposition was reduced by administration of alpha-glycerophosphocholine in senescence-accelerated mouse prone 8 mice.
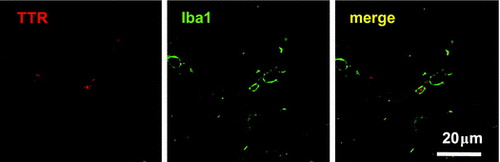
Globally, the average age of populations is increasing, and the occurrence of age-related diseases is growing as a result. Consequently, the social security benefits for the elder population place burdens in certain developed countries, like Japan. To reduce the cost, promoting a healthy lifestyle and reducing the duration of morbidity to death are necessary.
Dietary habit affects human health; therefore, maintaining a healthy diet has attracted attention. Numerous recent studies have shown that biological aging is modifiable and that certain food-derived bioactive compounds affect the life span of animal models, and the molecular mechanisms have been revealed as follows. Resveratrol, a bioactive compound found in red wine, activates sirtuin 1 (SIRT1), which is a deacetylase and promotes cell survival [Citation1], and consumption of resveratrol has been demonstrated to improve the physiological conditions and extended the life span of mice [Citation2]. Similar effects of epigallocatechin gallate, which is a major bioactive compound found in green tea that also activates SIRT1 [Citation3], were also confirmed in rats [Citation4]. These data suggest that some food-derived bioactive compounds modify the aging process and would be effective for healthy aging because delaying biological aging would simultaneously delay the onset and progression of age-related diseases [Citation5]. Therefore, extensive research has been conducted on how functional foods that contain bioactive compounds may affect and delay aging.
In this study, we examined the effect of alpha-glycerophosphocholine (GPC) on aging in the senescence-accelerated mouse prone 8 (SAMP8) mice. As SAMP8 mice are characterized by rapid aging and declining cognitive function, they have been used in aging research [Citation6]. GPC exists in various foods, including milk; liver; and sake cake, a byproduct of Japanese sake fermentation. GPC is thus recognized as a useful choline compound food ingredient. Orally administrated GPC is effectively absorbed in the intestines and is distributed throughout the body, including the brain [Citation7]. GPC is used in medicines and supplements for choline supplementation because choline deficiency affects brain function [Citation8] and increases the risk of neural tube defects [Citation9] and cancer [Citation10]. In addition, although it has been reported that GPC supplementation prevents the decline of cognitive function in patients with mild Alzheimer’s disease (AD) [Citation11], its effect on other aging process and its mechanism have not been fully understood yet. We evaluated its effects on senescence, the amyloidogenic proteins amyloid beta (Aβ), transthyretin (TTR), and osteoarthritis (OA) in SAMP8 mice. Protein aggregation diseases include age-related diseases such as AD. In AD, Aβ aggregates cause neuroinflammation and progressive neurodegeneration [Citation12]. As TTR interacts with Aβ and inhibits Aβ aggregation and cytotoxicity [Citation13], it may prevent AD. However, TTR also aggregates and causes senile systemic amyloidosis. Therefore, prevention of Aβ and TTR aggregation would reduce the risk for age-related diseases. Interestingly, TTR aggregation has been found in cartilages from patients with OA [Citation14,15], which is the most prevalent joint disease and lowers the quality of life of elderly, suggesting that prevention of TTR aggregation may also delay the progression of OA.
Materials and methods
Animals
Twenty 3-week-old male SAMP8 mice were purchased from Japan SLC (Shizuoka, Japan) and maintained under controlled conditions (ambient temperature, 22 ± 2 °C, 12-h light/dark cycle, lights on from 12:00 am to12:00 pm, lights off from 12:00 pm to 12:00 am). The animals were housed (n = 5 per cage) in plastic cages (225 × 338 × 140 mm) with free access to food (MF, Oriental Yeast, Tokyo, Japan) and water. This study was approved by the Animal Care Committee of the National Research Institute of Brewing, Japan (ethical approval No. 26–1). After a 1-week acclimation period, the mice received deionized drinking water with or without 0.07 mg/ml GPC (NOF corporation; n = 10 mice per group) by 45 weeks.
Grading of senescence
The degree of senescence was evaluated using a scoring system that comprises the following: 11 items in four categories—behavior (reactivity and passivity), skin and hair (glossiness, coarseness, and hair loss), eyes (ulcer, periophthalmic lesions, cataracts, corneal ulcer, and corneal opacity), and skeleton (lordokyphosis) [Citation16]. In this system, the scores are 0 to 4, and the grading score is sum of the scores of all 11 items. Each mouse was examined at 36 and 44 weeks of age.
Elevated-plus-maze test
The plus maze comprised two pairs of opposing open and closed arms (6 cm × 30 cm) that extended from a central area (9 cm × 9 cm). The maze floor was 40 cm above the room floor. The open arms included a slightly raised edge that was 2 cm high to reduce the likelihood of animals falling over. The arms were enclosed within three walls, with open roofs that were 10 cm high. The test was performed from 1:00 pm to 3:00 pm. At the start of the test, each mouse was placed at the center of the maze facing the open arm. Its behavior in the plus maze was observed for 10 min. Arm entry was defined as all the four paws inside the arm. The time spent in the arms was recorded. The time spent in the open arms was defined as the measure of anxiety.
Immunohistochemistry
The SAMP8 mice were killed at 45 weeks of age. Their brains were removed, coronally sliced, fixed in 10% formalin at 4 °C for 1 day, embedded in paraffin, and cut into 8-μm-thick sections. The sections were immunostained using antibodies specific to Aβ1–42 (Immuno-Biological Laboratories Co., Ltd., Gunma, Japan; No. 18582), TTR (Abcam, Cambridge, MA, USA; ab9015), Iba1 (Wako Pure Chemical Industries, Ltd., Osaka, Japan; No. 019–19741). We performed antigen retrieval by formic acid treatment (90% formic acid for 5 min at room temperature) for Aβ1–42 or by autoclaving (121 °C for 5 min) for Iba1. To detect the antibodies, we used commercial kits: a DAKO EnVision + kit for Aβ1–42 and Iba1 and a VECTASTAIN Elite ABC kit for TTR. The experiments were performed following the manufacturers’ protocols. Briefly, after blocking treatment using blocking solutions of the kits, the slides were incubated with specific antibodies (Aβ1–42, 1 μg/ml; TTR, 1:1,000; Iba1, 2 μg/ml) overnight at 4 °C. Reactivity was visualized using the kits. For microscopic analysis, Aβ1–42 and TTR depositions were counted in visual fields (0.75 mm × 1 mm), and the maximum number was used for comparison. The left and right sides of the cortex and the hippocampus were analyzed in each mouse.
Confocal microscopy analysis
For brain tissue imaging by fluorescence analysis, the brain tissue slides were immunostained using antibodies specific to TTR and Iba1 overnight at 4 °C, followed by incubation with secondary antibodies for 2 h at room temperature: goat anti-rabbit IgG conjugated with Alexa Fluor 488 (Cell Signaling Technology, Inc., Danvers, MA, USA) for Iba1 and anti-sheep IgG conjugated with Alexa Fluor 647 (Abcam) for TTR. The tissues were imaged with an LSM 700 confocal microscope (Zeiss, GmbH, Jena, Germany).
Histological analysis of knee joints
Knee joints from 45-week-old SAMP8 mice were harvested. The joints were fixed in 4% paraformaldehyde for 24 h, decalcified, and embedded in paraffin. Serial sections (4 μm) were cut, stained with Safranin O-fast green, and examined for histopathological changes using a semiquantitative scoring system from the OA Research Society International [Citation17]. In this system, the scores were defined as follows: 0, normal cartilage; 0.5, loss of proteoglycan with an intact surface; 1, superficial fibrillation without the loss of cartilage; 2, vertical clefts and loss of surface lamina (any percentage of joint surface area); 3, vertical clefts/erosion to the calcified layer for 1–25% of the quadrant width; 4, lesion reached the calcified cartilage for 25–50% of the quadrant width; 5, lesion reached the calcified cartilage for 50–75% of the quadrant width; and 6, lesion reached the calcified cartilage for over 75% of the quadrant width.
Statistical analysis
Data are presented as mean ± SD or SEM. Data were analyzed by Student’s t test, along with a Mann–Whitney test. The frequencies of protein deposition were analyzed by Fisher’s exact test. p < 0.05 was considered significant.
Results
Senescence grading score
To assess the effect of GPC on the progression of senescence in SAMP8 mice, grading of four categories was performed in 36- and 44-week-old mice. The total score and each grading score of the control and the GPC groups are shown in Table . At 36 weeks of age, the total score of the GPC group was significantly lower than that of the control group. However, at 44 weeks of age, there was no significant difference between the groups. Among the four categories, a significant difference only in behavior was observed at 36 weeks of age (p < 0.01). The grading scores for behavior at 44 weeks of age and skeleton at 36 and 44 weeks of age were lower in the GPC group than in the control group, although the differences were not significant. This suggests that GPC may delay aging of the brain and movement decline in SAMP8 mice.
Table 1. Senescence grading score in SAMP8 mice.
Elevated-plus-maze test
The time spent in the open arms in the GPC group was decreased modestly, but not significantly, compared to that in the control group (p = 0.055) (Figure ). It has been shown that SAMP8 mice exhibit age-related changes in emotional behavior, and in the elevated-plus-maze test, they remained in the open arms longer than senescence-accelerated mice resistant 1 mice [Citation18], which age normally and are used as control mice for the SAMP8 model. Our findings thus suggest that administration of GPC may affect age-related changes in emotional behavior.
Aβ and TTR deposition
Examination of the cortex and hippocampus of both groups with antibodies against Aβ1–42 and TTR was performed. Although Aβ1–42 staining was observed in both groups, there was no significant difference in Aβ1–42 plaques (supplementary data, Figure S1). We performed TTR staining in 16 right and left cortical sections from each group. All cortical sections from mice in the control group were stained with the TTR antibody, but five cortical sections from mice in the GPC group were not stained (Figure (A)–(D) and Table ). The TTR staining of the cortex of the GPC group was significantly decreased compared with the control group (Table ), suggesting that consumption of GPC prevented TTR aggregation. However, plaques of TTR in the cortex and hippocampus of the groups were not significantly different. Diffuse TTR staining was observed around blood vessels (Figure (A), (C), and (D)).
Figure 2. TTR deposition in SAMP8 mice. Representative images are presented in Figure 2(A)–(D). (A—D) Images of the cortex (A: control; B: GPC) and hippocampus (C: control; D: GPC) are shown. (E, F) TTR deposition data (average number ± S.D.) are shown for the (E) control (□) and (F) GPC (■) groups, respectively. There was no significant difference between the control and GPC groups. (G) Activated microglia (green) internalized TTR deposition (red).
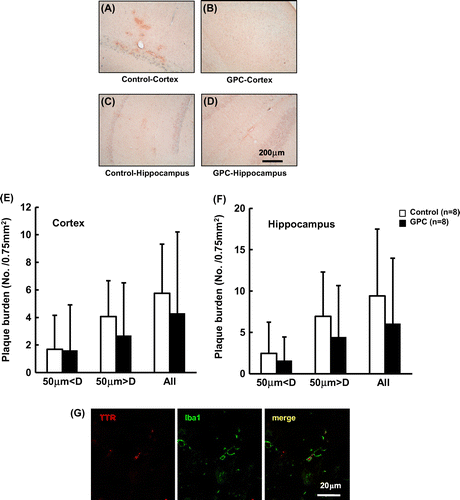
Table 2. TTR deposition in SAMP8.
To investigate whether TTR aggregates activated microglia in SAMP8 mice, double staining with antibodies against TTR and Iba1 was performed. Iba1 is a marker of microglia in the brain. We observed that activated microglia existed around TTR aggregates (Figure (E) and (F)), suggesting that TTR aggregates induced neuroinflammation in SAMP8 mice.
Effect of GPC on OA
As shown in Figure , all knee joints of the control group showed severe cartridge degradation, but some of the knee joints of the GPC group showed moderate cartilage degradation. Comparison of the scores of both groups showed a significant decrease in cartilage pathology in the GPC group compared with the control group.
Discussion
In this study, we evaluated the effect of GPC on aging. The laboratory diet of mice contained GPC (33.2 mg/100 g). The estimated GPC intakes of mice in the different groups are shown in Table . The total GPC intake of the GPC group (50.4 mg/kg body weight/day) was 17% higher than that of the control group (43.2 mg/kg body weight/day). Thus, the observed effects are attributed to additional GPC. However, there is a possibility that the observed effects were due to additional choline intake. To clarify this issue, other experiments using other choline molecules are necessary.
Table 3. Estimated dose of GPC intake in this study.
As the administration of GPC has been reported to GPC prevent a decline in cognitive function in AD patients [Citation11], it may delay brain aging. However, its effect on the aging of other organs remains unknown. In this study, we found that administration of GPC resulted in an improved senescence grading score in the SAMP8 mice compared to that in the control group (Table ), although its effect disappeared at 44 weeks. Thus, GPC may at least partially delay aging. As the grading scores for behavior and skeleton at 44 weeks of age were lower in the GPC group than in the control group, administration of GPC may delay aging of the brain and cause a motor decline in SAMP8 mice.
The results of the elevated-plus-maze test suggested that GPC may slightly improve age-related low anxiety-like behavior in SAMP8 mice, which is consistent with the senescence grading score, showing that GPC may delay aging of the brain, as discussed above.
We evaluated its effect on Aβ1–42 deposition in the brains of SAMP8 mice because Aβ1–42 is a major form of Aβ plaques in AD patients [Citation19]. There was no significant difference in Aβ1–42 deposition in the cortex and hippocampus between the control and GPC groups (supplementary data, Figure S). Interestingly, TTR deposition was not observed in 5 of 16 cortical sections from mice in the GPC group (Figure (B) and Table ), suggesting that GPC may delay TTR deposition. TTR, mainly produced in hepatocytes, is a homotetrameric protein and transports thyroxin and retinol-binding protein. It has been shown that TTR inhibits Aβ aggregation and cytotoxicity [Citation13]. Furthermore, TTR is also synthesized in cortical and hippocampal neurons [Citation20–22] and protects AD model mice from Aβ toxicity [Citation23]. Thus, TTR is hypothesized to prevent AD. On the other hand, TTR is also an amyloidogenic protein and causes senile systemic amyloidosis. TTR amyloid activates microglia resulting in neuroinflammation and synapse loss [Citation24]. It has not been reported whether TTR deposition occurs in the brain of SAMP8 mice, but in this study, we found that TTR aggregated in the brain and activated microglia (Figure ). Therefore, TTR deposition in the brain of SAMP8 mice may cause neuroinflammation and result in a decline in their cognitive function. As TTR deposition was reduced in the brain by administration of GPC, it would prevent neuroinflammation and protect neural cells. However, Aβ accumulation was unchanged between the two groups, suggesting that GPC did not directly stabilize TTR or stimulate Aβ elimination. In fact, we previously confirmed that GPC did not stabilize TTR in a thermal shift assay (unpublished data). Thus, the preventive effect of GPC on TTR deposition may be attributed to other mechanisms. In aged SAMP8 mice, as the blood-brain barrier (BBB) increases in permeability, macromolecule extravasation occurs and induces inflammation in the brain [Citation25,26]. The concentration of TTR in the plasma is higher than that of cerebrospinal fluid (CSF), and dysfunction of the BBB disturbs Aβ elimination by TTR from CSF into serum [Citation27] and increases the risk of TTR deposition. This hypothesis is consistent with the result that diffuse TTR staining is also observed around blood vessels. Thus, GPC may sustain BBB function, prevent inflammation, and delay TTR deposition. To clarify the mechanism of the preventive effect of GPC on TTR deposition, further research is necessary. There is another mechanism by which GPC may exert its protective effects in the brain. It has been shown that administration of GPC increases growth hormone production and enhances fat oxidation, resulting in increasing 3-hydroxybutyrate levels in serum [Citation28]. 3-Hydroxybutyrate inhibits histone deacetylases and increases the levels of oxidative stress resistance factors [Citation29]. As oxidative stress accelerates aging, this effect of GPC may protect brain function in SAMP8 mice.
Aging is also a risk factor for OA, and OA progression is associated with TTR deposition [Citation14,15]. TTR deposition was reduced in the brains of mice in the GPC group, and GPC was shown to potentially prevent a decline in motor function, as indicated by the senescence grading scores. GPC may thus delay OA progression by preventing TTR deposition in joints. Pathological analyses of knee joints of SAMP8 mice showed that administration of GPC delayed OA progression (Figure ). The SAMP8 mice developed knee joint degeneration at the age of several months [Citation30], but it remains unknown whether TTR deposition occurs in knee joints in SAMP8 mice [Citation31]. We are investigating changes in TTR deposition in joints from SAMP8 mice at various ages and the effects that GPC administration has on these changes. As previously reported, TTR deposition in human OA cartilage was observed and exerted cytotoxicity for human chondrocytes [Citation15]. Very recently, Matsuzaki et al. have reported an intriguing mechanism by which TTR deposition promotes OA in murine models [Citation32]. TTR deposition was found in the synovium of aged mice and enhanced inflammatory gene expression, resulting in OA progression. Thus, GPC may reduce TTR deposition in the synovium in SAMP8 mice and delay OA. As explained above, GPC does not stabilize TTR; therefore, the underlying mechanism of the preventive effect of GPC on OA remains to be elucidated. Investigations of its effect on chondrocytes, synoviocytes, and osteoclasts, which play important roles in OA, are also ongoing in our laboratories.
In conclusion, in this study, we show that administration of GPC delays aging and prevents OA progression and TTR deposition in the brains of SAMP8 mice. These results suggest that GPC would be a potential substance for preventing age-related diseases, and that GPC-containing foods may exert an anti-aging effect. However, the mechanism by which GPC exerts its anti-aging effects remains unknown. We have examined the effect of GPC using in vitro models using some cell lines but not found its clear effect yet. Thus, its metabolites may exert anti-aging effects. In fact, it has been reported that orally administrated GPC is metabolized to choline and some unidentified choline metabolites in 5 h [Citation7]. It is of interest to elucidate the exact mechanisms.
Author contributions
K.M., S.M., T.O., H.I., and T.F. conceived the experiments and wrote the manuscript. K.M., M.O., S.S., S.M., and H.I. performed the experiments and analyzed the data. All authors read and approved the manuscript.
Disclosure statement
K.M., S.M., H.I., and T.F. have filed a patent on the use of GPC for OA prevention (Japan patent application: P2016–117881).
Funding
This work was supported in part by the Council for Science, Technology, and Innovation (CSTI); the Cross-Ministerial Strategic Innovation Promotion Program (SIP); “Technologies for Creating Next-Generation Agriculture, Forestry, and Fisheries” (K.M., H.I., and T.F.) and JSPS KAKENHI [grant number JP17K07791] (K.M. and S.M.).
Supplemental data
Supplemental data for this article can be accessed https://doi.org/10.1080/09168451.2017.1403883
Fig._S1.pptx
Download MS Power Point (496 KB)BBB_Supplementary_data_by_Matsubara_et_al..docx
Download MS Word (94.4 KB)Acknowledgements
We thank Prof Lotz, M., the Scripps Research Institute, San Diego, CA, USA for his helpful comments on TTR and OA research.
References
- Howitz KT, Bitterman kJ, Cohen HY, et al. Small molecule activators of sirtuins extend Saccharomyces cerevisiae lifespan. Nature. 2003;425(6954):191–196. DOI:10.1038/nature01960. PubMed PMID: 12939617.
- Baur JA, Pearson kJ, Price NL, et al. Resveratrol improves health and survival of mice on a high-calorie diet. Nature. 2006;444(7117):337–342. DOI:10.1038/nature05354. PubMed PMID: 17086191; PubMed Central PMCID: PMCPMC4990206.
- de Boer VC, de Goffau MC, Arts IC, et al. SIRT1 stimulation by polyphenols is affected by their stability and metabolism. Mech Ageing Dev. 2006;127(7):618–627. DOI:10.1016/j.mad.2006.02.007 . PubMed PMID: 16603228.
- Niu Y, Na L, Feng R, et al. The phytochemical, EGCG, extends lifespan by reducing liver and kidney function damage and improving age-associated inflammation and oxidative stress in healthy rats. Aging Cell. 2013;12(6):1041–1049. DOI:10.1111/acel.12133 . PubMed PMID: 23834676.
- Kaeberlein M. Longevity and aging. F1000Prime Rep. 2013;5:5. DOI:10.12703/P5-5. PubMed PMID: 23513177; PubMed Central PMCID: PMCPMC3590784.
- Akiguchi I, Pallàs M, Budka H, et al. SAMP8 mice as a neuropathological model of accelerated brain aging and dementia: Toshio Takeda’s legacy and future directions. Neuropathology. 2017;37(4):293–305. DOI:10.1111/neup.12373 . PubMed PMID: 28261874.
- Abbiati G, Fossati T, Lachmann G, et al. Absorption, tissue distribution and excretion of radiolabelled compounds in rats after administration of [14C]-L-alpha-glycerylphosphorylcholine. Eur J Drug Metab Pharmacokinet. 1993;18(2):173–180 . PubMed PMID: 8243501.10.1007/BF03188793
- Zeisel SH, Niculescu MD. Perinatal choline influences brain structure and function. Nutr Rev. 2006;64(4):197–203. PubMed PMID: 16673755; PubMed Central PMCID: PMCPMC2438605.
- Fisher MC, Zeisel SH, Mar MH, et al. Inhibitors of choline uptake and metabolism cause developmental abnormalities in neurulating mouse embryos. Teratology. 2001;64(2):114–122. doi:10.1002/tera.1053 . PubMed PMID: 11460263.
- da Costa KA, Niculescu MD, Craciunescu CN, et al. Choline deficiency increases lymphocyte apoptosis and DNA damage in humans. Am J Clin Nutr. 2006;84(1):88–94. PubMed PMID: 16825685; PubMed Central PMCID: PMCPMC2430662.
- Moreno MDJM. Cognitive improvement in mild to moderate Alzheimer’s dementia after treatment with the acetylcholine precursor choline alfoscerate: a multicenter, double-blind, randomized, placebo-controlled trial. Clin Ther. 2003;25(1):178–193 . PubMed PMID: 12637119.10.1016/S0149-2918(03)90023-3
- Querfurth HW, LaFerla FM. Alzheimer’s disease. N Engl J Med. 2010;362(4):329–344. DOI:10.1056/NEJMra0909142 . PubMed PMID: 20107219.
- Giunta S, Valli MB, Galeazzi R, et al. Transthyretin inhibition of amyloid beta aggregation and toxicity. Clin Biochem. 2005;38(12):1112–1119. doi:10.1016/j.clinbiochem.2005.08.007 . PubMed PMID: 16183049.
- Niggemeyer O, Steinhagen J, Fuerst M, et al. Amyloid deposition in rheumatoid arthritis of the hip. Rheumatol Int. 2012;32(9):2645–2651. DOI:10.1007/s00296-011-2005-2009 . PubMed PMID: 21786121.
- Akasaki Y, Reixach N, Matsuzaki T, et al. Transthyretin deposition in articular cartilage: a novel mechanism in the pathogenesis of osteoarthritis. Arthritis Rheumatol. 2015;67(8):2097–2107. DOI:10.1002/art.39178. PubMed PMID: 25940564; PubMed Central PMCID: PMCPMC4519374.
- Hosokawa M, Kasai R, Higuchi K, et al. Grading score system: a method for evaluation of the degree of senescence in Senescence Accelerated Mouse (SAM). Mech Ageing Dev. 1984;26(1):91–102 . PubMed PMID: 6748759.10.1016/0047-6374(84)90168-4
- Glasson SS, Chambers MG, Van Den Berg WB, et al. The OARSI histopathology initiative – recommendations for histological assessments of osteoarthritis in the mouse. Osteoarthritis Cartilage. 2010;18(Suppl 3):S17–S23. doi:10.1016/j.joca.2010.05.025 . PubMed PMID: 20864019.
- Miyamoto M, Kiyota Y, Nishiyama M, et al. Senescence-accelerated mouse (SAM): age-related reduced anxiety-like behavior in the SAM-P/8 strain. Physiol Behav. 1992;51(5):979–985 . PubMed PMID: 1615059.10.1016/0031-9384(92)90081-C
- Saito T, Matsuba Y, Mihira N, et al. Single App knock-in mouse models of Alzheimer’s disease. Nat Neurosci. 2014;17(5):661–663. DOI:10.1038/nn.3697 . PubMed PMID: 24728269.
- Li X, Masliah E, Reixach N, et al. Neuronal production of transthyretin in human and murine Alzheimer's disease: is it protective? J Neurosci. 2011;31(35):12483–12490. doi:10.1523/JNEUROSCI.2417-11.2011. PubMed PMID: 21880910; PubMed Central PMCID: PMCPMC3172869.
- Kerridge C, Belyaev ND, Nalivaeva NN, et al. The Abeta-clearance protein transthyretin, like neprilysin, is epigenetically regulated by the amyloid precursor protein intracellular domain. J Neurochem. 2014;130(3):419–431. doi:10.1111/jnc.12680 . PubMed PMID: 24528201.
- Wang X, Cattaneo F, Ryno L, et al. The systemic amyloid precursor transthyretin (TTR) behaves as a neuronal stress protein regulated by HSF1 in SH-SY5Y human neuroblastoma cells and APP23 Alzheimer’s disease model mice. J Neurosci. 2014;34(21):7253–7265. DOI:10.1523/JNEUROSCI.4936-4913.2014. PubMed PMID: 24849358; PubMed Central PMCID: PMCPMC4028500.
- Buxbaum JN, Ye Z, Reixach N, et al. Transthyretin protects Alzheimer’s mice from the behavioral and biochemical effects of Abeta toxicity. Proc Natl Acad Sci USA. 2008;105(7):2681–2686. DOI:10.1073/pnas.0712197105. PubMed PMID: 18272491; PubMed Central PMCID: PMCPMC2268196.
- Azevedo EP, Ledo JH, Barbosa G, et al. Activated microglia mediate synapse loss and short-term memory deficits in a mouse model of transthyretin-related oculoleptomeningeal amyloidosis. Cell Death Dis. 2013;4:e789. DOI:10.1038/cddis.2013.325. PubMed PMID: 24008733; PubMed Central PMCID: PMCPMC3789183.
- Pelegrí C, Canudas AM, del Valle J, et al. Increased permeability of blood-brain barrier on the hippocampus of a murine model of senescence. Mech Ageing Dev. 2007;128(9):522–528. doi:10.1016/j.mad.2007.07.002 . PubMed PMID: 17697702.
- del Valle J, Duran-Vilaregut J, Manich G, et al. Time-course of blood-brain barrier disruption in senescence-accelerated mouse prone 8 (SAMP8) mice. Int J Dev Neurosci. 2009;27(1):47–52. doi:10.1016/j.ijdevneu.2008.10.002 . PubMed PMID: 18992318.
- Alemi M, Gaiteiro C, Ribeiro CA, et al. Transthyretin participates in beta-amyloid transport from the brain to the liver–involvement of the low-density lipoprotein receptor-related protein 1? Sci Rep. 2016;6:429. doi:10.1038/srep20164. PubMed PMID: 26837706; PubMed Central PMCID: PMCPMC4738280.
- Kawamura T, Okubo T, Sato K, et al. Glycerophosphocholine enhances growth hormone secretion and fat oxidation in young adults. Nutrition. 2012;28(11–12):1122–1126. doi:10.1016/j.nut.2012.02.011 . PubMed PMID: 22673596.
- Shimazu T, Hirschey MD, Newman J, et al. Suppression of oxidative stress by beta-hydroxybutyrate, an endogenous histone deacetylase inhibitor. Science. 2013;339(6116):211–214. doi:10.1126/science.1227166. PubMed PMID: 23223453; PubMed Central PMCID: PMCPMC3735349.
- Chen WH, Hosokawa M, Tsuboyama T, et al. Age-related changes in the temporomandibular joint of the senescence accelerated mouse. SAM-P/3 as a new murine model of degenerative joint disease. Am J Pathol. 1989;135(2):379–385. PubMed PMID: 2782378; PubMed Central PMCID: PMCPMC1879928.
- Shimizu K, Higuchi K, Matsushita M, et al. Immunohistochemical studies of age-associated amyloid deposition in the joint of senescence-accelerated mouse (SAM). Z Rheumatol. 1992;51(5):243–248 . PubMed PMID: 1476009.
- Matsuzaki T, Akasaki Y, Olmer M, et al. Transthyretin deposition promotes progression of osteoarthritis. Aging Cell. 2017;16(6):1313–1322. DOI:10.1111/acel.12665 . PubMed PMID: 28941045.