Abstract
Diabetic nephropathy (DN) is a diabetic vascular complication, and abnormal protein kinase C (PKC) activation from increased diacylglycerol (DG) production in diabetic hyperglycemia is one of the causes of DN. Diacylglycerol kinase (DGK) converts DG into phosphatidic acid. In other words, DGK can attenuate PKC activity by reducing the amount of DG. Recently, we reported that intraperitoneally administered d-α-tocopherol (vitamin E, αToc) induces an amelioration of DN in vivo through the activation of DGKα and the prevention of podocyte loss. However, the effect of the oral administration of αToc on DN in mice remains unknown. Here, we evaluated the effect of oral administration of αToc on DN and its molecular mechanism using streptozocin-induced diabetic mice. Consequently, the oral administration of αToc significantly ameliorated the symptoms of DN by preventing the loss of podocytes, and it was revealed that the inhibition of PKCactivity was involved in this amelioration.
The assumed mechanisms of the protective effect of d-α-tocopherol on diabetic nephropathy.
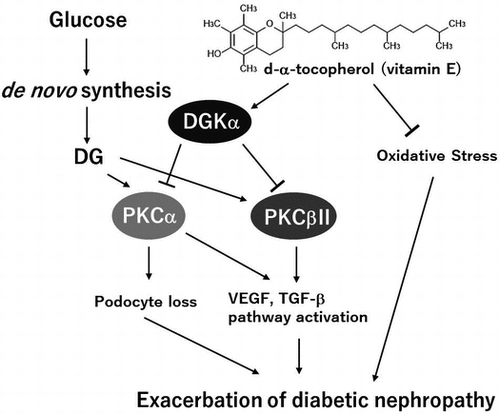
Diabetic nephropathy (DN) is one of the serious vascular complications of diabetes and is associated with increased cardiovascular mortality [Citation1]. Moreover, DN is the main reason for initiating renal replacement therapy. DN is a disorder of the microvasculature in the glomerulus caused by sustained hyperglycemia and causes the failure of glomerular filtration, which leads to albuminuria. It is well known that abnormal protein kinase C (PKC) activity is one of the causes of DN. Hyperglycemia results in the production of diacylglycerol (DG) from glucose by de novo synthesis [Citation2–5], and the produced DG abnormally activates PKC in diabetic patients [Citation6,7]. Indeed, it has been reported that PKC activity increases in the vascular tissues of diabetic rats because of the produced DG [Citation8,9]. These facts suggest that the normalization of the DG-PKC pathway in the glomeruli is important for ameliorating DN.
Diacylglycerol kinase (DGK) is a lipid kinase that phosphorylates DG, converting DG into phosphatidic acid (PA). It is known that PA activates phosphatidylinositol 4-phosphate 5-kinase [Citation10] and mammalian target of rapamycin (mTOR) [Citation11]. By contrast, the fact that DG activates PKC means that DGK can attenuate PKC activity by reducing the amount of DG, which suggests that DGK can improve DN by normalizing abnormal PKC activity during hyperglycemia [Citation12]. Indeed, it has been reported that d-α-tocopherol (vitamin E, αToc) normalizes the DG-PKC pathway through DGK activation, which contributes to the amelioration of DN in diabetic rats [Citation13]. So far, 10 subtypes of mammalian DGK have been reported [Citation14–16]. Of these, DGKα, γ, δ, ε and ζ are expressed in the glomeruli of rats. We previously demonstrated that αToc specifically activates DGK alpha (DGKα) among the DGK subtypes expressed in rat glomeruli in an in vitro study [Citation17]. Importantly, we proved that DGKα is involved in αToc-induced amelioration of DN in vivo by using DGKα-deficient mice [Citation18]. Moreover, we revealed that the activation of DGKαby αToc prevents the loss of glomerular epithelial cells (podocytes) that are known to be a cause of filtration failure in DN [Citation18]. However, the effect of the oral administration of αToc on DN is still unknown because the previous experiments were performed via intraperitoneal administration. Furthermore, the mechanism underlying the prevention of podocyte loss by DGKα remains unclear. Therefore, we evaluated the effect of the oral administration of αToc on DN in streptozocin-induced diabetic mice and investigated the mechanism underlying the prevention of podocyte loss by αToc.
Materials and methods
Materials
Streptozocin (STZ) was purchased from Sigma-Aldrich (St. Louis, MO, USA). D-α-tocopherol powder 50 (the purity is 50%) was provided by Eisai Food and Chemical Co., Ltd. (Tokyo, Japan). The powder diet (DC-8) was purchased from CLEA Japan, Inc. (Tokyo, Japan). The general component composition of DC-8 is shown in Supplemental Table 1. Vitamin E is contained in DC-8 but the detailed composition of DC-8 is not to be opened. According to CLEA Japan Inc., CE-2 have similar composition to DC-8 and CE-2 contains 6.4 mg/100 g of vitamin E. Therefore we estimated that amount of vitamin E in DC-8 was similar to that of CE-2. The general component composition of CE-2 is also shown in Supplemental Table 1. The Glu-testSensor and Glu-testEvery were purchased from Sanwa Kagaku Kenkyuusho (Nagoya, Japan). Wild-type male C57BL/6 mice were purchased from Japan SLC, Inc. (Shizuoka, Japan). Anti-HO-1 antibody was purchased from Enzo Life Science, Inc. (NY, USA). Anti-PKC (phospho S660) antibody and anti-PKCα (phospho S657) antibody were purchased from Abcam (Cambridge, UK).
Mice
All animal studies were approved by the Institutional Animal Care and Use Committee (permission number: 25-07-03) and performed according to the Kobe University Animal Experimentation Regulations. All mice had free access to water and were bred under a 14-h light, 10-h dark cycle, and the temperature was maintained at 23 ± 2 °C.
Experimental design
To induce diabetes, six-week-old male C57BL/6 mice were intraperitoneally (i.p.) administered STZ (50 mg/kg) in 20 mM citrate buffer once per day for 5 consecutive days. For the control group, the same volume of vehicle was administered (i.p.). Both of STZ treated group and control group were divided into two groups that were fed with normal diet and 1% αToc containing diet after the final STZ administration (the mice number of each group was n = 5). To evaluate symptoms of DN, we collected urine samples from each group with metabolic cages under fasting conditions for 8 h (9:30 am ~ 5:30 pm) every week after final STZ administration until week 6, and we measured urine volume and amount of albumin. The fasting blood glucose levels and body weight were measured before/after STZ administration and every time after the urine collection. Final week of the experiment, all mice were perfused with 0.9% NaCl in the anesthetized condition with Ravonal (Mitsubishi Tanabe Pharma Corporation) before removal of kidney. We measured kidney weight and the kidneys were submitted to immunofluorescent staining and western blotting. In case of evaluation of the effect of low concentration αToc, we produced diabetic mice as described above and divided the mice into normal diet or 0.01, 0.1 and 0.5% αToc containing diet groups (the mice number of each group was n = 5). To evaluate symptoms of DN, we measured urine volume and amount of albumin every week until week 3 after final STZ administration.
Urine analysis
The collected urine was centrifuged at 1500 × g for 10 min, and the supernatant was used as the sample. The urine sample was subjected to SDS-PAGE and CBB staining to evaluate amount of urine albumin. The amount of urine albumin was normalized to the urine volume and body weight.
Immunofluorescent staining of nephrin in the kidney glomeruli
The removed kidneys were embedded in O.C.T. compound. After freezing at –30 °C, the kidney samples were sliced into 20 μm sections using a cryostat (Leica CM1850). The sections were fixed in acetone, and immunofluorescence staining was performed using a guinea pig anti-nephrin antibody (PROGEN Biotechnik, Heidelberg, Germany) as the primary antibody and an Alexa Fluor-conjugated secondary antibody. Finally, the fluorescence signal was analyzed using confocal microscopy.
Western blotting of the kidney lysate
The removed kidneys were lysed in homogenate buffer(20 mM Tris-HCl, 1 mM EDTA, 1 mM EGTA, 1 mM MgCl2, 1% Triton-X, 20 mg/ml leupeptin and 1 μM phenylmethylsulfonyl fluoride, pH 7.4). The protein concentration in cell lysate was measured with a BCA assay. Equal amounts of proteins were subjected to SDS-PAGE followed by immunoblotting using appropriate primary and secondary antibodies.
Statistical analysis
The data are presented as mean ± SE. Two way analysis of variance (ANOVA) was used to determine significant difference in the experiments of Figures , and then Scheffe’s test was used as a post hoc test when significant interaction between STZ treatment and αToc administration was obtained by two way ANOVA. The results of two way ANOVA were shown as a table in all graphs. In the experiment of Figure , the value of control group was considered as a reference data and the other data were analyzed Dunnett’s multiple comparison test comparing with STZ group. P value of less than 0.05 was considered to be significant.
Figure 1. Effects of the oral administration of αToc on blood glucose levels and body weight. The blood glucose levels (A) and body weight (B) of the mice in each group were measured before and after STZ administration (day 0 and day 5) and every week thereafter for 6 weeks. Number of mice in every group was n = 5. The values are means ± SE. The results of two way ANOVA were shown as a table in graphs. Different superscript letters indicate significant difference analyzed with two way ANOVA and Scheffe’s test among the groups.
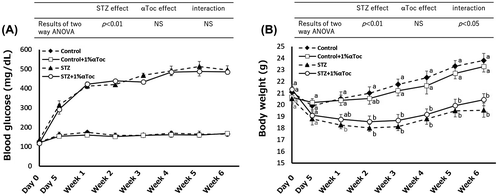
Figure 2. Changes in the symptoms of DN due to the oral administration of αToc. (A) Urine albumin levels were measured in each group from weeks 1 to 6. (B) The mean amounts of urine albumin from weeks 1 to 6 are shown. Urine albumin levels were normalized to body weights of the mice. (C) Urine volume was measured for each group using metabolic cages. (D) The mean volumes of urine from weeks 1 to 6 are shown. Urine volumes were normalized to the body weights of the mice. (E) Kidney weights were measured at end of the experiment (week 6) and normalized to the body weights. The error bars show ± SE. The results of two way ANOVA were shown as a table in graphs. Different superscript letters indicate significant difference analyzed with two way ANOVA and Scheffe’s test among the groups.
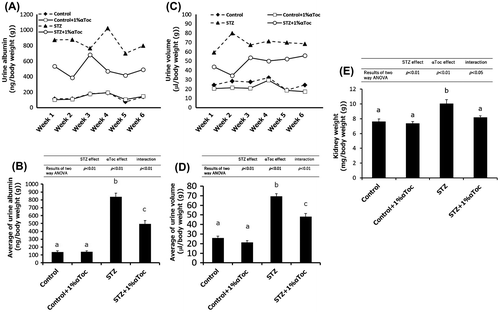
Figure 3. Effects of the oral administration of αToc on podocyte loss and the phosphorylation of PKCα in DN. The kidneys of the mice were removed at the end of the experiment (week 6) and were sectioned. We performed immunofluorescent staining of these sections using an anti-nephrin antibody for the primary antibody. The nephrin staining (red) was observed using confocal laser microscopy (A). The kidneys removed at the end of experiment (week 6) were lysed and subjected to SDS-PAGE followed by Western blotting using anti-nephrin antibody (B) and anti-PKCα (phospho S657) antibody (C). The intensities of nephrin and phospho-PKCα were analyzed with ImageJ and normalized to that of GAPDH. The values are means ± SE. The results of two way ANOVA were shown as a table in graphs. Different superscript letters indicate significant difference analyzed with two way ANOVA and Scheffe’s test among the groups.
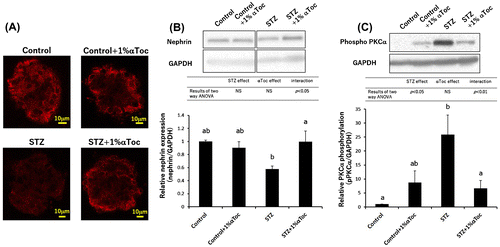
Figure 4. Changes in the level of phospho-PKCβII and HO-1 expression in the kidneys. The kidneys were removed at the end of the experiment (week 6) and were lysed and subjected to SDS-PAGE followed by Western blotting using anti-PKC (phospho S660) antibody (A) and anti-HO-1 antibody (B). The intensities were analyzed with ImageJ and normalized to that of GAPDH. The values are means ± SE. The results of two way ANOVA were shown as a table in graphs. Different superscript letters indicate significant difference analyzed with two way ANOVA and Scheffe’s test among the groups.
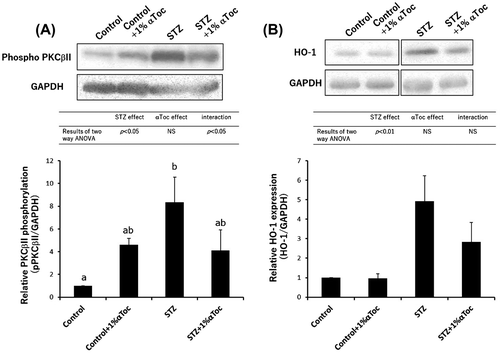
Figure 5. Effects of the oral administration of low-concentration αToc on DN. The blood glucose levels (A) and body weights (B) of the mice in each group were measured before and after STZ administration (day 0 and day 5) and at every week thereafter until 3 weeks. The mean urine albumin amount (C) and urine volume (D) from weeks 1 to 3 are shown. The values are means ± SE. Asterisks show significance compared with STZ group (p < 0.05; Dunnett’s multiple comparison test).
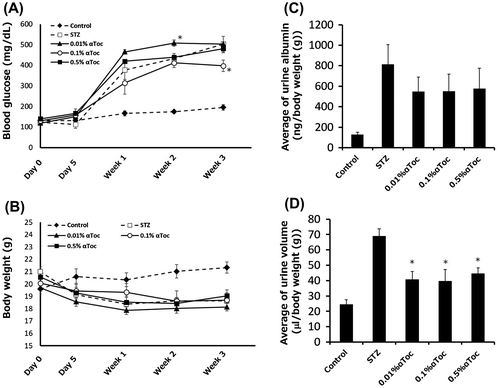
Results
αToc did not affect blood glucose levels or body weight
We first compared the food consumed by the mice on the normal diet and the mice on the 1% αToc-containing diet before the experiments. There was no significant difference in food consumption between the normal diet mice and the 1% αToc-containing diet mice (consumption of normal diet: 2.7 ± 0.3 g/day, 1% αToc-contained diet: 3.0 ± 0.8 g/day). Even after STZ treatment, the food consumption showed no significant difference (consumption of normal diet: 3.0 ± 0.6 g/day, 1% αToc-contained diet: 3.3 ± 0.5 g/day at 1 week after STZ administration).
The blood glucose levels of the STZ-treated mice increased at the final administration of STZ (Day 5), and the mice exhibited sustained high blood glucose levels until week 6 (Figure (A)). The mean of blood glucose level during weeks 1–6 for the STZ-treated mice was 466.5 ± 54.3 mg/dL and that for the STZ and αToc-treated mice was 458.6 ± 40.7 mg/dL. Two way ANOVA in the blood glucose level between week 1 and 6 showed a statistic difference for the effect of STZ treatment but not for the effect of αToc administration. Moreover, there was no interaction between STZ treatment and αToc administration (Table in Figure (A)).
It is well known that diabetic patients cause body weight loss because of they unable to utilize glucose as energy as a result of insulin tolerance or decrease of insulin secretion. Indeed, STZ treatment decreased the body weights of the mice in this experiment (Figure (B)). Two way ANOVA in the body weight between week 1 and 6 showed a statistic difference for the effect of STZ treatment but not for the effect of αToc administration. Moreover, interaction between STZ treatment and αToc administration was detected. Therefore, we performed Scheffe’s test as a post hoc test but significant differences were not detected between normal diet groups and 1% αToc containing diet groups not only in the control mice but also in the STZ-treated diabetic mice (Figure (B)).
These results indicate that the oral administration of αToc did not improve diabetes itself, which is similar to the results of previous research performed with intraperitoneal administration of αToc [Citation18].
Oral administration of αToc ameliorated DN
To evaluate the symptoms of DN, we measured the urine albumin amount, the urine volume and the kidney weight because it is well known that these markers increase in patients with DN. As illustrated in Figure (A), the urine albumin levels in the STZ-treated group increased at week 1 and exhibited sustained high levels until week 6. However, a reduction of urine albumin was detected following the oral administration of αToc at week 1, and this reduction was maintained (Figure (A)). Therefore, we analyzed the average of urine albumin from week 1 to week 6 by two way ANOVA. The two way ANOVA showed a statistic difference in all parameters including interaction. Therefore, the data were analyzed Scheffe’s test as a post hoc test. The average of urine albumin in the STZ-treated mice was significantly high compared with non-diabetic mice (control and control + 1% αToc) but that in the STZ and 1% αToc-treated mice was significantly lower than that in STZ-treated mice, indicating that 1% αToc containing diet significantly suppressed increase of urine albumin by diabetes (Figure (B)).
Similarly, the urine volume in the STZ-treated mice remained at a sustained high level during weeks 1 to 6 (Figure (C)), and the statistical analysis indicated that oral administration of αToc reduced urine volume compared that of the STZ-treated mice (Figure (D)). Additionally, kidney weight at week 6 also showed a statistic difference in all parameters including interaction by two way ANOVA, and Scheffe’s test showed that αToc administration normalized the STZ-induced increase of kidney weight (Figure (E)). These results clearly indicated that the oral administration of 1% αToc could ameliorate conditions of DN in mice.
Oral administration of αToc prevented podocyte loss
Glomerular epithelial cells, known as podocytes, form a slit membrane structure that functions as a filtration barrier in the glomeruli [Citation19]. It is well known that DN induces podocyte loss, which leads to filtration failure [Citation20]. Our previous research demonstrated that the intraperitoneal administration of αToc prevented podocyte loss in DN [Citation18]. Therefore, to confirm whether the oral administration of αToc also prevented podocyte loss, we performed immunofluorescent staining and western blotting of nephrin, which is a marker of podocytes. The nephrin staining became weak, and the stained area was decreased in the kidneys of the STZ-treated mice (Figure (A)). The oral administration of αToc rescued the nephrin staining pattern, which indicated that podocyte loss was prevented by the oral administration of αToc (Figure (A)). To confirm the prevention of podocyte loss by αToc, we checked amount of nephrin in the kidney by western blotting. Two way ANOVA in the western blotting of nephrin showed interaction between STZ treatment and αToc administration. The nephrin expression in the STZ-treated mice showed tendency to decrease compared to control groups (p < 0.1; Scheffe’s test) and the decrease in nephrin expression was significantly prevented by oral administration of αToc (Figure (B)).
It has been reported that PKCα is implicated in the exacerbation of DN and the abnormal activation of PKCα in DN mediates nephrin endocytosis in podocytes [Citation21–23]. Therefore, we next checked the phosphorylation level of PKCα which shows amount of activated PKCα in the kidney.Two way ANOVA in the western blotting of phosphorylated PKCα showed statistic difference for the effect of STZ treatment and interaction. The result of Scheffe’s test showed that phosphorylation of PKCα in the STZ-treated mice significantly increased compared with control, and the increase in phosphorylated PKCα was significantly normalized by the oral administration of αToc (Figure (C)). These results indicated that the oral administration of 1% αToc prevented the loss of podocytes in DN and suggested that the suppression of phosphorylated PKCα by αToc was one of causes of the prevention of podocyte loss.
Oral administration of αToc suppressed the phosphorylation of PKCβII and the expression of HO-1
As described above, it is known that abnormal PKC activation, especially PKCβ [Citation24], is one of the causes of DN. Our previous study revealed that the intraperitoneal administration of αToc suppressed PKCβII phosphorylation [Citation18]. Therefore, we evaluated whether the oral administration of αToc suppressed the phosphorylation of PKCβII. The result of two way ANOVA in the western blotting of phosphorylated PKCβII showed interaction between STZ treatment and αToc administration. Scheffe’s test showed that STZ treatment significantly induced an increase in PKCβII phosphorylation in the kidney but not in STZ and αToc-administrated group (Figure (A)), which indicated that the oral administration of αToc ameliorated DN by suppressing both abnormal PKCα and PKCβII activation.
In addition to the abnormal activation of PKC, oxidative stress is known to be one of the causes of DN [Citation25], and αToc is well-known as an antioxidant. Therefore, the effects of the oral administration of αToc on the expression of heme oxygenase-1 (HO-1), which is an antioxidant enzyme, were investigated based on the report that the expression of HO-1 increases in the glomeruli of diabetic rats, and the expression of HO-1 was normalized by αToc [Citation26]. Two way ANOVA of HO-1 expression showed significance for effect of STZ treatment, but significance for effect of αToc and interaction between STZ treatment and αToc administration were not obtained. However, αToc administration to STZ-induced diabetic mice showed tendency to reduce HO-1 expression compared with mice with STZ treatment (the HO-1 expression in STZ + 1% αToc group was approximately 58% of that in STZ group) (Figure (B)). These results suggest that αToc acted not only as a DGKα activator but also as an antioxidant that ameliorated DN.
0.01% αToc significantly improved DN
Finally, we checked whether a lower concentration of αToc could ameliorate DN. STZ-treated mice were fed 0.5, 0.1 and 0.01% αToc containing diets, and urine albumin amounts and urine volumes were measured. This experiment was performed until week 3 after STZ administration. We confirmed elevation of blood glucose level in all STZ-treated groups compared to control, and then blood glucose level of STZ and αToc-treated groups at every week were analyzed with Dunnett’s multiple comparison test comparing with STZ group. As a result, although blood glucose level of 0.01% αToc group at week 2 was significantly higher than STZ group and 0.1% αToc group at week 3 was significantly lower than STZ group (Figure (A)), there were no significant differences in other points compared to STZ group. We also analyzed body weight with the same way in the case of blood glucose level, but there were no significant differences (Figure (B)). It was noteworthy that 0.5% αToc group did not show significant differences in blood glucose level and body weight. These results suggested that these concentration of αToc administration did not ameliorate diabetes itself similarly to 1% αToc as shown Figure .
Next, we investigated effect of lower dose of αToc on urine albumin and urine volume. Although there were no significance detected between STZ group and all αToc-treated groups by Dunnett’s test comparing with STZ group, the STZ-induced increase in the amount of urine albumin tended to be suppressed in all concentrations of αToc-treated groups (Figure (C)). On the other hand, the STZ-induced increase in urine volumes were significantly suppressed in all αToc-treated groups compared with STZ-treated group (Figure (D)). These results indicated that even 0.01% αToc ameliorated some conditions of DN.
Discussion
Our previous study revealed that intraperitoneal administration of αToc ameliorated DN by preventing the loss of podocytes through DGKα activation [Citation18]. In the present study, we demonstrated for the first time that oral administration of 1% αToc can also ameliorate DN by preventing the loss of podocytes. Moreover, αToc administration significantly suppressed not only PKCβII phosphorylation but also the PKCα phosphorylation increase in STZ-induced diabetic mouse kidneys.
The slit membrane structure that functions as a filtration barrier in the glomeruli is formed by interdigitating foot processes (FP) that are membrane swellings of the podocytes [Citation19]. In short, the morphology of the podocytes and the FP formations are essential for the filtration ability of the glomeruli. It is well known that nephrin plays a pivotal role in the adhesion between the FPs and the neighboring FPs to maintain the slit membrane structure [Citation27–29]. Interestingly, the abnormal activation of PKCαin DN mediates nephrin endocytosis in podocytes, and the inhibition of abnormal PKCα activation reduces albuminuria in diabetic mice [Citation23,30]. Additionally, we previously demonstrated that DGKα is expressed in podocytes [Citation31]. Therefore, we measured PKCα phosphorylation to investigate the mechanism by which αToc prevented podocyte nephrin loss. Oral administration of 1% αToc significantly suppressed the abnormal increase in PKCα phosphorylation in the STZ-induced diabetic mice (Figure (C)), which indicated that αToc administration ameliorated DN by regulating nephrin endocytosis through PKCα inhibition by activating DGKα in the podocytes.
In addition to nephrin, it is also known that α3β1 integrin maintains podocyte morphology [Citation32,33]. Podocytes are attached to the glomerular basement membrane (GBM), and α3β1 integrin is involved in the adhesion between podocytes and the GBM [Citation34]. It has been reported that DGKα interacts with β1 integrin [Citation35,36]. Therefore, there is the possibility that αToc enhances the attachment of podocytes to the GBM by regulating β1 integrin via DGKα activation, which results in the prevention of the loss of podocytes. However, the effect of αToc on the interaction between DGKα and β1 integrin remains unknown. Thus, additional investigations will be needed.
There is convincing evidence that cytokines, such as transforming growth factor-β (TGF-β) and vascular endothelial growth factor (VEGF), are involved in the aggravation of kidney disease [Citation37,38]. Indeed, it has been demonstrated that treatment with monoclonal antibodies against TGF-β or VEGF ameliorates DN in animal models [Citation39,40]. Moreover, it has been reported that not only PKCα but also PKCβII regulates the signaling of TGF-β and the expression of VEGF in podocytes [Citation41,42]. Therefore, we hypothesized that, in addition to the prevention of the podocyte loss, αToc administration might regulate TGF-β and VEGF signaling via the attenuation of both PKCα and PKCβII activities by DGKα activation to contribute to the amelioration of DN.
Moreover, hyperglycemia produces reactive oxygen species, and oxidative stress is known as one of causes of DN [Citation25,43]. Thus far, many experiments seeking to ameliorate DN have been performed, focusing on oxidative stress and have demonstrated that reducing oxidative stress ameliorates DN [Citation44–46]. In our study, the expression of HO-1 tended to be suppressed by the αToc treatment compared with the STZ-induced diabetic mice [Citation18]. This fact suggests that the antioxidative effect as well as the DGKα activation effect of αToc contributed to the amelioration of DN.
We used DC-8 powder diet as a control diet in this study, but DC-8 containing some αToc. It is considered that αToc in control diet also contributed to amelioration of DN somehow. However, it is difficult to conclude it because we have not prepared the diet with no αToc. At least, we can consider that the concentration of αToc in DC-8 was insufficient to ameliorate DN because additional αToc induced amelioration of DN.
Finally, we showed that even 0.01% αToc significantly reduced STZ-induced increase of urine volume and tended to decrease urine albumin amount, suggesting the possibility of αToc as a functional food for DN. But a huge-scale human clinical trial demonstrated that vitamin E supplementation did not improve renal insufficiency in people with and without diabetes [Citation47]. However, approximately 70% of the participants in this trial were non-diabetic patients with renal insufficiency. Thus, the exact effect of αToc on DN remains unclear. Moreover, this trial was performed with 400 IU/day αToc (approximately 270 mg/day for a natural source). The dose of αToc might have been insufficient to ameliorate renal insufficiency. Therefore, higher dose αToc supplementation might ameliorate DN in human. In this study, we demonstrated that the oral administration of 0.01% (approximately 24 mg/kg/day) αToc could ameliorate some symptoms of DN in mice, suggesting that high-dose αToc supplementation might ameliorate DN in humans.
On the other hand, we should consider the dose of αToc, because the tolerable upper intake levels for vitamin E are up to 1000 mg/day (equal to approximately 16 mg/kg/day, 1500 IU/day) for males and females more than 19 years old according to the National Institutes of Health, and some long-term human trials indicate that intakes of greater than 400 IU/day αToc significantly increase mortality [Citation48]. Therefore, we should consider the total dose of αToc. Our data demonstrated that the efficiency of 0.01% αToc was almost equal to that of 0.5% αToc in terms of the symptoms of DN (Figure (C) and (D)), which suggests that the oral administration of αToc at doses lower than 0.01% may ameliorate some symptoms of DN. Therefore, it will be worthy to check the effect of αToc lower than 0.01% on DN in the near future.
In conclusion, the oral administration of 1% αToc ameliorated DN in mice by preventing the loss of podocytes. Moreover, the attenuation of PKCα activity is implicated as one of the mechanisms by which αToc prevented the loss of podocytes in DN. Additionally, αToc suppressed the abnormal phosphorylation of PKCα and PKCβII and the expression of HO-1, which suggests that αToc acts as an activator of DGKα and an antioxidant under diabetic conditions to ameliorate DN. In addition, these findings suggest that αToc can be functional food for DN, although we should check the effect of lower than 0.01% of αToc supplementation on DN.
Author contribution
D. H. performed the experiments and analysed the data. S. U., M. Y. and H. A. gave advice about the experiments. H. A. gave advice about statistical analysis. D. H. and Y. S. conceived the project and wrote the manuscript. Y. S. supervised the research.
Disclosure statement
No potential conflict of interest was reported by the authors.
Funding
This work was supported by Grant-in-Aid for Japan Society for the Promotion of Science (JSPS) Fellow [grant number JP16J02115].
Supplemental data
Supplemental data for this article can be accessed https://doi.org/10.1080/09168451.2017.1411184.
Supplemental.jpg
Download JPEG Image (39.2 KB)References
- Valmadrid CT, Klein R, Moss SE, et al. The risk of cardiovascular disease mortality associated with microalbuminuria and gross proteinuria in persons with older-onset diabetes mellitus. Arch Intern Med. 2000;160:1093–1100.10.1001/archinte.160.8.1093
- Williams B, Gallacher B, Patel H, et al. Glucose-induced protein kinase C activation regulates vascular permeability factor mRNA expression and peptide production by human vascular smooth muscle cells in vitro. Diabetes. 1997;46:1497–1503.10.2337/diab.46.9.1497
- Ruan X, Arendshorst WJ. Role of protein kinase C in angiotensin II-induced renal vasoconstriction in genetically hypertensive rats. Am J Physiol. 1996;270:945–952.
- Berne C. The metabolism of lipids in mouse pancreatic islets, the biosynthesis of triacylglycerols and phospholipids. Biochem J. 1975;152:667–673.10.1042/bj1520667
- Dunlop ME, Larkins RG. Pancreatic islets synthesize phospholipids de novo from glucose via acyl-dihydroxyacetone phosphate. Biochem Biophys Res Commun. 1985;132:467–473.10.1016/0006-291X(85)91157-X
- Craven AP, Davidson CM, DeRubertis FR. Increase in diacylglycerol mass in isolated glomeruli by glucose from de novo synthesis of glycerolipids. Diabetes. 1990;47:667–674.10.2337/diab.39.6.667
- Koya D, King GL. Protein kinase C activation and the development of diabetic complications. Diabetes. 1998;47:859–866.10.2337/diabetes.47.6.859
- Shiba T, Inoguchi T, Sportsman JR, et al. Correlation of diacylglycerol and protein kinase C activity in rat retina to retinal circulation. Am J Physiol. 1993;136:1339–1348.
- Inoguchi T, Battan R, Handler E, et al. Preferential elevation of protein kinase C isoform beta II and diacylglycerol levels in the aorta and heart of diabetic rats: differential reversibility to glycemic control by islet cell transplantation. Proc Natl Acad Sci USA. 1992;89:11059–11063.10.1073/pnas.89.22.11059
- Jones David R, Sanjuan Miguel A, Mérida Isabel. Type Iα phosphatidylinositol 4-phosphate 5-kinase is a putative target for increased intracellular phosphatidic acid. FEBS Lett. 2000;476:160–165.10.1016/S0014-5793(00)01702-6
- Fang Y, Vilella-Bach M, Bachmann R, et al. Phosphatidic acid-mediated mitogenic activation of mTOR signaling. Science. 2001;30:1942–1945.10.1126/science.1066015
- Takai Y, Kishimoto A, Kikkawa U, et al. Unsaturated diacylglycerol as a possible messenger for the activation of calcium-activated, phospholipid dependent protein kinase system. Biochem Biophys Res Commun. 1979;91:1218–1224.10.1016/0006-291X(79)91197-5
- Koya D, Lee IK, Ishii H, et al. Prevention of glomerular dysfunction in diabetic rats by treatment with d-alphaTocopherol. J Am Soc Nephrol. 1997;8:426–435.
- Topham MK, Prescott SM. Mammalian diacylglycerol kinases, a family of lipid kinases with signaling functions. J Biol Chem. 1999;274:11447–11450.10.1074/jbc.274.17.11447
- van Blitterswijk WJ, Brahim H. Properties and functions of diacylglycerol kinases. Cell Signal. 2000;12:595–605.10.1016/S0898-6568(00)00113-3
- Kanoh H, Yamada K, Sakane F. Diacylglycerol kinases: emerging downstream regulators in cell signaling systems. J Biochem. 2002;131:629–633.10.1093/oxfordjournals.jbchem.a003144
- Fukunaga-Takenaka R, Shirai Y, Yagi K, et al. Importance of chroman ring and tyrosine phosphorylation in the subtype-specific translocation and activation of diacylglycerol kinase α by d-α-tocopherol. Genes Cell. 2005;10:311–319.10.1111/gtc.2005.10.issue-4
- Hayashi D, Yagi K, Song, C, et al. Diacylglycerol kinase α is involved in the vitamin E-induced amelioration of diabetic nephropathy in mice. Sci Rep. 2017;7:2597.10.1038/s41598-017-02354-3
- Mundel P, Kriz W. Structure and function of podocytes: an update. Anat Embryol. 1995;192:385–397.
- Pagtalunan ME, Miller PL, Jumping-Eagle S, et al. Podocyte loss and progressive glomerular injury in type II diabetes. J Clin Invest. 1997;99:342–348.10.1172/JCI119163
- Kang N, Alexander G, Park JK, et al. Differential expression of protein kinase C isoforms in streptozotocin induced diabetic rats. Kidney Int. 1999;56(5):1737–1750.10.1046/j.1523-1755.1999.00725.x
- Menne J, Park JK, Boehne M, et al. Diminished loss of proteoglycans and lack of albuminuria in protein kinase Calpha-deficient diabetic mice. Diabetes. 2004;53(8):2101–2109.10.2337/diabetes.53.8.2101
- Quack I, Woznowski M, Potthoff SA, et al. PKCα mediates β-arrestin2-dependent nephrin endocytosis in hyperglycemia. J Cell Biol. 2011;286:12959–12970.
- Ishii H, Jirousek MR, Koya D, et al. Amelioration of vascular dysfunctions in diabetic rats by an oral PKC β inhibitor. Science. 1996;272:728–731.10.1126/science.272.5262.728
- Suzuki D, Miyata T, Saotome N, et al. Immunohistochemical evidence for an increased oxidative stress and carbonyl modification of proteins in diabetic glomerular lesions. J Am Soc Nephrol. 1999;10:822–832.
- Koya D, Hayashi K, Kitada M, et al. Effects of antioxidants in diabetes-induced oxidative stress in the glomeruli of diabetic rats. J Am Soc Nephrol. 2003;14:S250–S253.10.1097/01.ASN.0000077412.07578.44
- Ruotsalainen V, Ljungberg P, Wartiovaara J, et al. Nephrin is specifically located at the slit diaphragm of glomerular podocytes. Proc Natl Acad Sci USA. 1999;96:7962–7967.10.1073/pnas.96.14.7962
- Holthofer H, Ahola H, Solin Marja-Liisa, et al. Nephrin localizes at the podocyte filtration slit area and is characteristically spliced in the human kidney. Am J Pathol. 1999;155:1681–1687.10.1016/S0002-9440(10)65483-1
- Holzman LB, St John PL, Kovari IA, et al. Nephrin localizes to the slit pore of the glomerular epithelial cell. Kidney Int. 1999;56:1481–1491.
- Tossidou I, Teng B, Menne J, et al. Podocytic PKC-α is regulated in murine and human diabetes and mediates nephrin endocytosis. PLoS ONE. 2010;5:e10185.10.1371/journal.pone.0010185
- Hayashi D, Ueda S, yamanoue, M, et al. Epigallocatechin-3-gallate activates diacylglycerol kinase alpha via a 67 kDa laminin receptor: a possibility of galloylated catechins as functional food to prevent and/or improve diabetic renal dysfunctions. J Funct Foods. 2015;15:561–569.10.1016/j.jff.2015.04.005
- Kerjaschki D, Ojha PP, Susani M, et al. A beta 1-integrin receptor for fibronectin in human kidney glomeruli. Am J Pathol. 1989;134:481–489.
- Adler S. Characterization of glomerular epithelial cell matrix receptors. Am J Pathol. 1992;141:571–578.
- Faul C, Asanuma K, Yanagida-Asanuma E, et al. Actin up: regulation of podocyte structure and function by components of the actin cytoskeleton. Trends Cell Biol. 2007;17:428–437.
- Rainero E, Caswell PT, Muller PA, et al. Diacylglycerol kinase α controls RCP-dependent integrin trafficking to promote invasive migration. J Cell Biol. 2012;196:277–295.10.1083/jcb.201109112
- Rainero E, Cianflone C, Porporato PE, et al. The diacylglycerol kinase α/Atypical PKC/β1 integrin pathway in SDF-1a mammary carcinoma invasiveness. PLoS ONE. 2014;9:e97144.10.1371/journal.pone.0097144
- Reeves MB, Andreoli TE. Transforming growth factor b contributes to progressive diabetic nephropathy. Proc Natl Acad Sci USA. 2000;97:7667–7669.10.1073/pnas.97.14.7667
- Cooper ME, Vranes D, Youssef S, et al. Increased renal expression of vascular endothelial growth factor (VEGF) and its receptor VEGFR-2 in experimental diabetes. Diabetes. 1999;48:2229–2239.10.2337/diabetes.48.11.2229
- Ziyadeh F, Hoffman BB, Han DC, et al. Long-term prevention of renal insufficiency, excess matrix gene expression, and glomerular mesangial matrix expansion by treatment with monoclonal anti transforming growth factor-β antibody in db/db diabetic mice. Proc Natl Acad Sci USA. 2000;97:8015–8020.10.1073/pnas.120055097
- de Vriese AS, Tilton RG, Elger M, et al. Antibodies against vascular endothelial growth factor improve early renal dysfunction in experimental diabetes. J Am Soc Nephrol. 2001;12:993–1000.
- Tossidou I, Starker G, Kruger J, et al. PKC-alpha modulates TGF-beta signaling and impairs podocyte survival. Cell Physiol Biochem. 2009;24:627–634.10.1159/000257518
- Hoshi S, Nomoto K, Kuromitsu J, et al. High glucose induced VEGF expression via PKC and ERK in glomerular podocytes. Biochem Biophys Res Commun. 2002;290:177–184.10.1006/bbrc.2001.6138
- Giardino I, Edelstein D, Brownlee M. BCL-2 expression or antioxidants prevent hyperglycemia-induced formation of intracellular advanced glycation endproducts in bovine endothelial cells. J Clin Invest. 1996;97:1422–1428.10.1172/JCI118563
- Lee EA, Seo JY, Jiang Z, et al. Reactive oxygen species mediate high glucose-induced plasminogen activator inhibitor-1 upregulation in mesangial cells and in diabetic kidney. Kidney Int. 2005;67:1762–1771.10.1111/j.1523-1755.2005.00274.x
- Elbe H, Vardi N, Esrefoglu M, et al. Amelioration of streptozotocin-induced diabetic nephropathy by melatonin, quercetin, and resveratrol in rats. Hum Exp Toxico. 2015;34(1):100–113.10.1177/0960327114531995
- Du X, Matsumura T, Edelstein D, et al. Inhibition of GAPDH activity by poly(ADP-ribose) polymerase activates three major pathways of hyperglycemic damage in endothelial cells. J Clin Invest. 2003;112:1049–1057.10.1172/JCI18127
- Mann JFE, Lonn EM, Yi Q, et al. Effects of vitamin E on cardiovascular outcomes in people with mild-to-moderate renal insufficiency: results of the HOPE Study. Kidney Int. 2004;65:1375–1380.10.1111/j.1523-1755.2004.00513.x
- Edgar RM 3rd, Pastor-Barriuso R, Dalal D, et al. Meta-analysis: high-dosage vitamin E supplementation may increase all-cause mortality. Ann Intern Med. 2005;142(1):37–46.