Abstract
Recent studies have revealed that various food components affect the immune response. These components act on various immune cells, and their effects are mediated through the intestinal immune system and, in some cases, the intestinal microbiota. In this review, we describe the immunomodulating effects of various food components, including probiotics, prebiotics, polysaccharides, vitamins, minerals, fatty acids, peptides, amino acids and polyphenols. Some of these components enhance immune responses, leading to host defense against infection, whereas others inhibit immune responses, thus suppressing allergy and inflammation.
Various food components act on various immune cells, and their effects are mediated through the intestinal immune system and, in some cases, the intestinal microbiota.
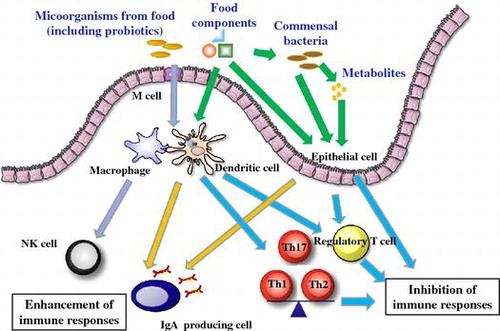
Recently it has been shown that various food components can affect the immune response. In this review, we provide an overview of the immune system, with a focus on the types of cells, the intestinal immune system, and the microbiota. Food can have beneficial effects on the immune system by both enhancing and inhibiting immune responses. Enhancement of the immune response provides host defenses against infection, and inhibition of immune responses suppresses allergies and inflammation.
Overview of the immune system
Immune cells
The immune system consists of the adaptive immune system and the innate immune system. The adaptive immune system recognizes specific, individual foreign antigens and remembers them for a long time through immunological memory. B cells and T cells, both of which are classified as lymphocytes, play central roles in the adaptive immune system by using specific receptors to recognize particular foreign antigens.
B cells produce antibodies, also known as immunoglobulins, against foreign antigens, such as those from pathogens and allergens causing hypersensitivity reactions. During primary activation after encountering its specific antigen, a B cell undergoes differentiation into a cell that produces different classes of immunoglobulin, including immunoglobulin A (IgA), IgG, and IgE, through the mechanism of isotype switching. IgA plays a critical role in protection from infection and control commensal bacteria at mucosal sites and is secreted in dimeric form in mucosal secretions, as well as in tears, saliva, sweat, and breast milk. IgG is the most abundant class of immunoglobulin in serum and reaches the fetus via the placenta, thus protecting the fetus from infection. IgE is responsible for the onset of type I allergy.
The majority of T cells express T-cell receptors consisting of α- and β-chains (although other, minor, T cells express γδ-type T-cell receptors) and can be categorized into two subpopulations depending on their surface expression of CD4 or CD8 molecules. CD4+ T cells are mainly helper T cells helping and controlling the activity of other immune cells. CD8+ T cells are cytotoxic; they kill cells infected by viruses or mutated cells with the potential to cause cancer.
Naïve CD4+ T cells, which are mature CD4+ T cells that have not yet been activated by encountering their specific antigen, differentiates into type 1, type 2, and type 17 helper T cells (abbreviated as Th1, Th2, and Th17), depending on the environment during its activation. These different types of helper T cells play specific roles by secreting different sets of cytokines. The cytokines characterizing each type of helper T cell are interferon γ (IFN-γ) for Th1, interleukin 4 (IL-4) for Th2, and IL-17 for Th17. Regulatory T cells (Tregs) are immunosuppressive T cells that downregulate the activation of effector T cells; some Treg cells are also differentiated from naïve CD4+ T cells in the periphery. Follicular helper T cells (Tfh), another subset of T cells differentiated from naïve CD4+ T cells, reside in the follicular (B-cell-rich) region of secondary lymphoid organs, such as the spleen and lymph nodes. They help B cells to undergo isotype switching, to make high affinity antibodies, and to differentiate into memory B cells [Citation1,2].
Although the cells of the innate immune system do not use specific receptors to recognize individual foreign antigens, they respond quickly to pathogen invasion by recognizing the molecular patterns characteristic of microbes via pattern recognition receptors, including Toll-like receptors (TLRs). Macrophages are phagocytic cells that ingest pathogens and dead or dying cells. Monocytes—progenitors of the macrophages circulating in the blood—differentiate into macrophages after migrating into the tissues via the blood vessel walls. Macrophages and dendritic cells function as antigen-presenting cells that activate naïve CD4+ T cells to become effector T cells. These cells are activated upon recognition of pathogens and process antigenic proteins into peptides, which are then presented to CD4+ T cells. Dendritic cells have the most potent ability to activate naïve CD4+ T cells and largely affect the direction of their functional differentiation.
Natural killer (NK) cells, another subpopulation of lymphocytes, are innate immune cells that eliminate virus-infected cells and mutated cells. The group of innate immune cells called innate lymphoid cells (ILCs), although they are of lymphoid lineage, have no antigen-specific receptors [Citation3,4]. ILCs play important roles in the inflammatory response and in maintaining immune homeostasis. ILCs are classified into three subsets, ILC1, ILC2, and ILC3, which have different functions and cytokine-expression patterns.
Neutrophils—the most abundant leukocytes in the blood, constituting 50% to 70% of the total—are phagocytic cells that are the fastest responders to pathogen invasion. Neutrophils, together with eosinophils and basophils, are called granulocytes, because they contain large numbers of granules. Eosinophils and basophils are involved in the inflammatory response and in allergic reactions. Mast cells, which also have granules, reside in the tissues but are barely found in the blood. They play a central role in type I allergy.
The intestinal immune system
The intestine is a large immune organ. The intestinal immune system consists of gut-associated lymphoid tissue (GALT) and other cells (Figure ) [Citation5]. In the small intestine are lymphoid structures called Peyer’s patches. The small intestinal villi are covered with a single layer of epithelial cells. Below the basement membrane are the diffuse structures of the lamina propria. These drain into the mesenteric lymph nodes. There are also lymphocytes in the epithelium. The large intestine contains organized lymphoid structures called colonic patches.
In the intestine there are various antigens, not only from pathogenic bacteria but also from food and commensal bacteria. The intestinal immune system has a number of characteristic functions that differ from those of the systemic immune system.
The first characteristic function is the induction of oral tolerance. Oral tolerance is immune tolerance induced by the ingestion of protein antigen [Citation6,7]. It is considered to be a mechanism that inhibits food allergy. Induction of anergy, clonal deletion of antigen-specific T cells, and induction of Tregs are the main mechanisms involved in oral tolerance. Recent advances point to the important role of Tregs. Dendritic cells in the mesenteric lymph nodes are efficient inducers of Foxp3+ Tregs because of their high levels of expression and activity of RALDH2—a key enzyme in the production of retinoic acid—and their capacity to activate TGF-β [Citation8,9].
Another important characteristic of the intestinal immune response is the secretion of IgA. IgA is the most abundant isotype produced in the intestine. It helps prevent invasion by pathogenic microorganisms and toxins and controls the intestinal microbiota. Various cells of the intestinal immune system contribute to the induction of IgA by producing factors that favor the IgA isotype. Importantly, intestinal dendritic cells produce IL-6, RALDH, and nitric oxide (NO) [Citation10–12], whereas intestinal epithelial cells produce IL-6 and TGF-β [Citation13].
At the T-cell level, induction of Tregs and Th17 is characteristic of the intestine. As previously mentioned, Tregs inhibit the inflammatory response. Th17 cells strengthen the mucosal barrier; they act on intestinal epithelial cells to stimulate their production of antimicrobial peptides [Citation14].
Intestinal microbiota
From among the enormous numbers and kinds of bacteria existing in nature, the intestinal symbiotic bacteria adapt to the environment of the host’s intestine and thus constitute the intestinal flora without being excluded. Because these bacteria are essentially substances foreign to the living body, they are at risk of being recognized by the intestinal immune system and excluded as foreign bodies. However, they are not actively excluded and enter the intestine with symbiotic characteristics. As a result, about 1000 species and more than several hundred trillions of bacteria exist in the human intestine, and their presence is thought to have a large influence on the intestinal environment. More than 100 trillion bacteria coexist in the human host, and more than 90% of these bacteria live in the intestinal tract. So whereas a human individual consists of about 60 trillion somatic cells, that individual contains many more coexisting ‘non-self’ bacteria. Mammals such as humans are inhabited by intestinal symbiotic bacteria shortly after birth in a sterile state, and these bacteria constitute the intestinal flora. The composition of the bacterial flora, and the numbers of bacteria, are strongly influenced by factors such as diet—especially if breast milk is given or not—and by the child’s growing and their nutritional conditions, such as meal conditions. Advances have been made in genetic analysis methods that are unique to microorganisms, such as 16S rRNA sequencing, and these analyses have revealed that the human intestinal microbiota is dominant in the phyla Firmicutes, Bacteroidetes, Actinobacteria and Proteobacteria [Citation15].
Numerous studies have found that intestinal symbiotic bacteria (e.g., segmented filamentous bacteria, Bacteroides) have an important role in the formation of intestinal tissue, including GALT. For example, in germ-free mice lacking intestinal symbiotic bacteria, the numbers and size of Peyer’s patches and cecal patches, which are thought to be sites of induction of the intestinal tract immune system, are smaller than in conventional mice. Intestinal IgA production, which is important for the prevention of host infection, is usually hyporesponsive in germ-free mice compared with conventional mice [Citation16,17]. Furthermore, oral tolerance is less likely to be induced in germ-free mice than in conventional mice [Citation18].
Allergy
Allergy is hypersensitivity to harmless environmental antigens such as pollen and food [Citation19]. Protein allergens cause allergy. A typical allergic response will be when an allergen induces Th2 cells secreting IL-4, IL-5 and IL-13, and these cells then induce IgE production, enhancing the eosinophilic response and eliciting harmful reactions. IgE will bind to Fcε receptors of mast cells and when they are cross-linked with allergen, mast cells will release chemical mediators (Figure ).
Enhancement of immune responses by food
Probiotics and other microorganisms
Probiotics are defined as living microorganisms with beneficial effects when administered orally. Immune modulation by probiotics—especially lactic acid bacteria (LAB) and Bifidobacterium—has been well documented. Notably, at least some of these immunomodulatory effects are produced by killed bacteria, implying that at least part of the probiotic effect does not necessarily require live bacteria. One target for probiotics is enhancement of the immune response, which may lead to host defense.
Firstly, the enhancement of the antibody response may be useful in preventing infection. Many reports have shown that IgG and IgA responses are enhanced by LAB and Bifidobacterium [Citation20–24]. Enhancement of IgA secretion may be important in preventing pathogens from invading mucosal barriers. The mechanisms underlying IgA enhancement by LAB have been explored; and it has been reported that LAB can act on intestinal dendritic cells and intestinal epithelial cells. Certain strains induce the secretion of IL-6 or TGF-β from dendritic cells, and such responses are shown to be TLR2-dependent [Citation22–24]. Another mechanism may be enhancement of the expression of polymeric immunoglobulin receptor (pIgR) [Citation25].
Another target of probiotics is the NK response. Many LAB enhance NK activity, which in turn augments host defense. LAB enhance IL-12 production, and IL-12 then augments NK activity [Citation26,27]. Polysaccharides produced by LAB may mediate these effects. LAB have also been shown to enhance the phagocytic activity of cells, such as macrophages. Yet another target is enhancement of the host defense response of epithelial cells [Citation28].
The above mechanisms reduce the risk of infection. Indeed, many studies have shown that oral administration of LAB can inhibit infections such as influenza in humans and in animal models [Citation21,22,29].
Prebiotics and bacterial metabolites
Prebiotics are food ingredients that induce the growth or activity of beneficial microorganisms. Dietary prebiotics are typically non-digestible fiber compounds that pass undigested through the upper part of the gastrointestinal tract and stimulate the growth or activity of specific bacteria that colonize the gut by acting as substrates for them. For example, fructooligosaccharides (FOS) are non-digestible saccharides composed mainly of 1-kestose (GF2) and nystose (GF3). Ingested FOS are used by specific bacteria (e.g., Bifidobacterium, Bacteroides) as energy sources for proliferation in the gut. The resulting changes in the intestinal microbiota and its metabolites alter the intestinal environment. This alteration induces the production of IgA, which is an important infection-preventing component of the intestinal immune system [Citation30,31]. Furthermore, GF3, which is a larger molecule than GF2, is effective in increasing the numbers of intestinal Bacteroides [Citation31] and induces more IgA production in the gut than does GF2 (Hosono et al. unpublished observation). Oral administration of prebiotic formula milk containing galactooligosaccharides and FOS to infants results in more secretory IgA in the feces than when formula without prebiotics is given [Citation32].
Short-chain fatty acids (SCFAs) produced by intestinal bacteria also play an important role in defense against infection by acting on the immune system. Acetic acid produced by the metabolism of bifidobacteria on intestinal epithelial cells can help prevent infection with intestinal enterohemorrhagic Escherichia coli O157 [Citation33]. SCFA metabolites of intestinal bacteria are strongly associated with G-protein-coupled receptors such as GPR41 and GPR43 in the intestinal tract. SCFA activates GPR41 and GPR43 on intestinal epithelial cells and leads to immune responses through the rapid production of chemokines and cytokines. These reactions mediate protective immunity and tissue inflammation in mice [Citation34].
Polysaccharides
Most polysaccharides with immunomodulatory functions are non-digestible. Their immunomodulatory functions are mostly explained by the fact that these polysaccharides serve as an energy source for gut microbes, as described in the previous section, but some non-digestible polysaccharides also have direct effects on immune cells.
β-glucan is a typical, non-digestible polysaccharide. β-glucans derived from mushrooms to yeast, including lentinan, which is an anti-cancer medicine derived from shiitake mushrooms, have a β-1,3-glucan backbone with β-1,6-glucan side chains [Citation35]. These β-glucans have been reported to enhance protection against infection by pathogenic bacteria and viruses [Citation36–38]. β-glucan, derived from oat, is linked via β-1,3- and β-1,4-glycosidic bonds, and oral administration of this β-glucan has been reported to protect mice from herpes virus infection [Citation39] and bacterial infections [Citation40]. β-glucans with the β-1,3-linkage are recognized by dectin-1, a C-type lectin, expressed on macrophages, monocytes, dendritic cells, and B cells to function as a pattern-recognition receptor [Citation41]. β-glucans activate these cell types to produce pro-inflammatory cytokines, including IL-6, TNF-α, and the Th1-inducing cytokine IL-12. IL-12 promotes activity of NK cells and phagocytes by up-regulating IFN-γ production from Th1 cells, and this process is similar to the process induced by the components derived from probiotic bacteria, as described above.
Glycogen, also called animal starch, is a digestible polysaccharide that consists of glucose molecules joined via α-glycosidic bonds; the primary function of glycogen is to serve as an energy and carbon reserve in animals and microbes. Enzymatically synthesized glycogen (ESG) has been reported to possess immunomodulatory effects [Citation42–44]. In an in vitro study, ESG stimulation of a mouse macrophage cell line RAW264.7 resulted in the production of NO and pro-inflammatory cytokines, such as IL-6 and TNF-α [Citation42]. A TLR2-mediated effect caused by ESG was suggested from the observation that ESG stimulated peritoneal macrophages derived from wild-type mice, but not those derived from TLR2-deficient mice, and that direct binding was observed between ESG and TLR2 [Citation43]. Oral administration of ESG led to anti-tumor activity and increased NK cell activity in mice [Citation44].
Fucoidans are a group of sulfated polysaccharides found in edible brown algae, such as Saccharina japonica (kombu), Saccharina sculpera (gagome), Undaria pinnatifida (wakame), Undaria pinnatifida (mekabu), and Cladosiphon okamuranus (mozuku). Fucoidans contain sulfated L-fucose and several other types of saccharides, including mannose, galactose, and xylose. Immunomodulatory functions have been reported for fucoidans, including anti-cancer [Citation45] and antiviral activities [Citation46,47]. Oral administration of fucoidan, derived from Undaria pinnatifida (mekabu), reportedly protected mice from infection by the herpes simplex virus type 1 (HSV-1) or influenza virus, by up-regulating the activity of NK cells and cytotoxic T lymphocytes and the production of neutralizing antibodies [Citation46,47].
Chitin is a polymer of N-acetyl-D-glucosamine with β1–4-linkages that is present in the exoskeletons of crustaceans, such as crabs and shrimp, and the cell wall of fungi; the deacetylated derivative of chitin is chitosan. Immunomodulatory effects have been reported for chitosan, including anti-cancer effects [Citation48] and activation of macrophages [Citation49]. Although a cellular receptor(s) for chitosan has not yet been identified, it has been suggested that chitosan with different molecular sizes are recognized by different receptors, as the immunomodulatory functions tend to depend on the molecular size of chitosan [Citation48,49]. In a clinical study, the administration of a water-soluble, low molecular weight chitosan to radiotherapy patients, suffering from lung cancer, led to an increase in the number of NK cells and the level of IL-6 and TNF-α, compared with those in the control group [Citation50].
Vitamins and other micronutrients
Vitamin A is essential for the maintenance of gut immunity, and vitamin A deficiency causes chronic diarrhea and night blindness. Vitamin A is metabolized into retinoic acid by dendritic cells in GALT, which induces lymphocytes to express gut-homing receptors, integrin α4β7, and the chemokine receptor CCR9, and stimulates B cells to differentiate into IgA-producing cells [Citation11,51]. Retinoic acid has also been shown to be critical for gut migration of ILCs [Citation52].
Vitamin E works principally as an antioxidant that stabilizes the cellular membrane. Vitamin E deficiency is reported to cause a decrease in antibody response, T cell proliferation against mitogenic stimulation, and phagocytic function [Citation53,54]. Supplementation of vitamin E at an amount above the recommended daily intake (6–15 mg/day, depending on the responsible organization) is known to up-regulate immune function [Citation55]. A daily intake of 200 mg of vitamin E increased lymphocyte proliferation and the number of B cells, compared to those in the placebo group [Citation56].
Vitamins involved in energy metabolism also have an impact on immune responses [Citation57]. Vitamin B1 is essential for the TCA cycle in energy metabolism and plays an important role in maintaining immune homeostasis. A vitamin B1-deficient diet caused impaired antigen-specific immune responses, such as decreased numbers of naïve B cells, which predominantly utilize the TCA cycle for energy generation, in the Peyer’s patch of mice administered with an oral vaccine [Citation58].
Vitamin B2 is also an important co-factor of various enzymes that function in energy metabolism [Citation59]. It has been reported that bacterial metabolites of vitamin B2, in the form of a complex with a non-classical MHC class I molecule MR1, stimulate innate mucosal-associated invariant T (MAIT) cells [Citation60], which play an important role in mucosal defense and inflammation in the gut by producing IFN-γ and IL-17 [Citation61].
Zinc, a constituent of various kinds of enzymes and transcription factors, is one of the most important micronutrients for the maintenance of immune homeostasis. Zinc deficiency has demonstrated a profound impairment of immune system development and innate and adaptive immune system functions, including thymus atrophy, decreased NK-cell and phagocytic-cell function, and decreased lymphocyte proliferation [Citation62]. As these features are similar to those seen in immune system of elderly people, appropriate zinc supplementation has been suggested to serve as an effective strategy to potentiate immune responses in elderly people [Citation55]. In people in developing countries suffering from a zinc deficiency, zinc supplementation has reduced the occurrence of diarrhea and respiratory infection and the severity of malaria symptoms [Citation63]. The zinc ion has been revealed to function as a second messenger in cellular signaling pathways, like a calcium ion [Citation64].
Selenium is also essential for the maintenance of immune homeostasis [Citation65]. Keshan disease, an endemic disease caused by coxsackievirus infection, is predominant in a local area in China, where the soil content of selenium is very low. Selenium deficiency impairs activation of lymphocytes, leading to decreased cellular immune responses and B cell antibody production. As selenium is a component of antioxidant enzymes, such as glutathione peroxidase and thioredoxin reductase, selenium deficiency causes increased production of reactive oxygen species (ROS) in neutrophils and macrophages. Epidemiological studies have suggested an inverse association between selenium levels and colon cancer risk [Citation66].
Fatty acids
Fatty acids are known to influence immune function and inflammatory responses [Citation67,68]. SCFAs are generated by gut microbiota that metabolize dietary fibers and affect various aspects of immune responses in the gut, as described in the section ‘Prebiotics and bacterial metabolites.’ It has been reported that mice fed a diet enriched with palmitic acid, a saturated long chain fatty acid, demonstrated increased intestinal IgA production without affecting the serum antibody responses [Citation69]. It was also found that palmitic acid increases antibody production by stimulating antibody-producing B cells (plasma cells).
Amino acids
Among the amino acids, arginine (Arg), glutamine (Gln), and tryptophan (Trp) have a large impact on the immune system. Arg is known to have strong immunopotentiating effects [Citation70,71]. Arg metabolism is mainly regulated by three enzymes, namely, inducible nitric oxide synthase (iNOS), arginase-1 (ARG1), and arginase-2 (ARG2). Micro-environmental Arg starvation, caused by ARG1 release from a myeloid-derived suppressor cell (MDSC) [Citation72], leads to the inhibition of T cell proliferation by decreasing cell surface expression of the TCR complex [Citation71,73], and to the decrease of NK-cell function [Citation70,74]. Catabolism of Arg results in the formation of ornithine, which in turn forms polyamines, such as spermine and spermidine. Polyamines play an important role in enhancing intestinal barrier function [Citation75,76]. Gut microbiota also generate polyamines.
Gln is important as a precursor of nucleic acids and as an energy source for fast dividing cells, such as lymphocytes and intestinal epithelial cells [Citation77,78]. When macrophages perform phagocytosis and pinocytosis, glucose is mainly used for lipid synthesis, but not for NADPH synthesis via the pentose phosphate pathway. In this situation, Gln plays an important role as a supplier of NADPH, an essential coenzyme for lipid synthesis. To compensate for the decreased serum level of Gln, caused by its increased demand during infection, wound, or surgery, the Gln supply from skeletal muscle is increased. If the serum Gln level does not reach the required level, it will cause a decrease in proliferation and differentiation of B and T lymphocytes, cytokine production, antigen presentation, and phagocytic activity [Citation78]. It was found that oral supplementation of Gln improved intestinal immune function and suppressed bacterial translocation [Citation79]. A recent study demonstrated that Gln deprivation promoted differentiation of CD4+ T cells to regulatory T cells [Citation80].
Trp is one of the essential amino acids and plays an important role in the immune response and neurotransmission through kynurenine synthesis and serotonin synthesis [Citation81]. Trp metabolism, as well as that of Arg, is critical in controlling immunity. Three enzymes, indoleamine 2,3-dioxygenase 1 (IDO1), IDO2, and tryptophan 2,3-dioxygenase (TDO), are involved in degrading Trp and synthesizing kynurenines [Citation82]. The IDO1 pathway for Trp degradation has been suggested to be immunosuppressive, as myeloid cells expressing IDO1 incorporate and degrade Trp, leading to a local deprivation of the amino acid and in turn causing cell cycle arrest and apoptosis of T cells [Citation83]. Kynurenines have various effects on the innate and adaptive immune responses, including the induction of regulatory T cells [Citation81,84].
Other compounds
Generally, diets rich in vegetables have been epidemiologically demonstrated to have health benefits. Although most research concerning the immunomodulatory effects of phytochemicals have focused on anti-inflammatory or anti-allergic effects [Citation85], some phytochemicals, including quercetin, naringenin, and genistein, have been shown to have immune potentiating effects, such as up-regulation of NK-cell activity [Citation86]. Extracts from stems and roots of Salacia reticulata have been used as a traditional medicine in Asia, like the ayurvedic medicine, for treatment of diabetes and other health problems. When administered orally, these extracts, which contain various phytochemicals, such as salacinol, kotalanol, and catechins, have been reported to show antiviral effects in influenza virus-infected mice, through up-regulation of NK-cell activity [Citation87].
High-polymeric inorganic polyphosphates are known to occur in all living organisms and possess regulatory roles. In a study on the molecules responsible for the protective effect of probiotic lactic acid bacteria on the intestinal epithelium against injurious stimuli, polyphosphate was found as the cytoprotective compound derived from the culture of a Lactobacillus strain [Citation88]. Polyphosphate was found to enhance the epithelial barrier function by inducing expression of a heat shock protein, HSP27, in the human intestinal epithelial cell line Caco2, and to suppress the oxidant-induced intestinal permeability in mouse small intestines by activating the integrin β1-p38 MAPK pathway.
Nucleotides have been considered as conditionally-essential nutrients for neonates and stressed patients, as they provide purines and pyrimidines for nucleic acid synthesis [Citation89,90]. Dietary nucleotides have been reported to have various immunomodulatory effects, including skewed induction of Th1-type responses [Citation91], increase of TCRγδ-bearing IEL populations [Citation92], and enhancement of mucosal IgA responses [Citation93] in mice.
Inhibition of immune responses by food
Hypoallergic food and use of oral tolerance
Hypoallergenic food, which is prepared by enzymatic degradation of allergens, carries a reduced risk of allergic symptoms developing after its ingestion. This strategy has been widely used as infant formula. Hypoallergenic wheat flour has also been developed [Citation94].
Oral tolerance has been used as a way of inhibiting allergy. Recently, it has been shown that allergies are more persistent in children who have avoided allergenic foods [Citation95]. This recent finding strongly suggests that oral tolerance is induced in humans by food antigens. The use of oral tolerance as a means of inhibiting allergy and autoimmune diseases has been attempted for many years [Citation6]. Previous studies have focused on multiple sclerosis and rheumatoid arthritis. Trials in transgenic rice expressing epitope peptides for the treatment of pollen allergy are ongoing [Citation96].
Probiotics and other microorganisms
At the beginning of this century it was reported that the intestinal microbiota differs between allergic and non-allergic individuals [Citation97,98]. Trials to inhibit allergy by administration of LAB were then performed. Kalliomaki et al. [Citation99] reported that administration of a Lactobacillus strain to pregnant mothers and their newborn babies could prevent atopic skin disease in the newborns. Since then, many trials to prevent or inhibit allergy by oral administration of LAB or other probiotic bacteria have been performed. Japanese studies have shown that oral administration of Lactobacillus or Bifidobacterium can alleviate pollen allergy [Citation100,101]. There are also reports on the effectiveness of LAB in atopic dermatitis [Citation102] and rhinitis [Citation103]. Meta-analyses have examined the effects of LAB on allergy [Citation104,105]. One such analysis has shown that probiotics are more efficient at preventing pediatric atopic dermatitis than at treating it [Citation104]. Another study has found that prenatal or early-life probiotic administration reduces the risk of atopic sensitization and decreases IgE levels in children [Citation105].
Direct effects through bacterial components and indirect effects through intestinal microbiota may both play a role in alleviating allergy. Inhibition of excessive Th2 response by LAB is one mechanism that has been shown in cellular and animal models. One well-defined mechanism is the induction of Th1 and inhibition of the Th2 response through enhancement of the IL-12 response [Citation106–108]. However, not all LAB function in this way. Certain LAB can inhibit Th2 responses without enhancing the Th1 response. Inhibition of Th2 in this case may be due to apoptosis induction [Citation109]. A further mechanism by which Th2 responses are inhibited is the induction of Tregs [Citation110]. In these cases, IL-10 may be produced [Citation111]. The induction of RALDH2 activity is an interesting mechanism [Citation112]. Another mechanism by which Bifidobacterium inhibits the Th2 response is by inhibiting the production of Th2 chemokines [Citation113]. Lactobacillus strains can enhance oral tolerance induction via an increase in Tregs [Citation114]. There also may be indirect effects of LAB through the intestinal microbiota discussed below.
LAB, Bifidobacterium, and other microorganisms have anti-inflammatory effects, potentially suppressing not only allergy but also other diseases such as inflammatory bowel diseases (IBD)—especially ulcerative colitis. Such effects have been studied in animal models such as dextran sodium sulfate (DSS)-induced colitis [Citation115]. There are also reports of bacteria or its components inhibiting production of inflammatory cytokines from intestinal epithelial cells [Citation116].
As previously mentioned, these direct effects of bacterial components are observable in the case of killed bacteria, showing that at least some of the probiotic effects do not necessarily require live bacteria. These bacterial components are recognized by immune competent cells via pattern recognition receptors such as TLRs. Some studies have demonstrated the involvement of TLR2, which may preferentially induce IL-10 [Citation117]. In contrast, IL-12 induction may not be mediated by TLRs. Lactobacillus strains with rigid cell wall induces large quantities of IL-Citation12,118) nevertheless an IL-12-enhancing effect is still observable in TLR2-/-TLR4-/-TLR9-/- mice [Citation119]. Interestingly, a recent report has demonstrated that RNA derived from LAB is recognized via TLR3 and induces type I interferon production [Citation120]. The mechanism of LAB uptake into the intestinal immune system has attracted attention. It has been previously shown that LAB can be incorporated into the Peyer’s patches, and recently there was an interesting report that a Lactobacillus acidophilus strain was internalized by mouse M cells via uromodulin-SlpA interaction [Citation121].
Prebiotics and bacterial metabolites
Prebiotics can also regulate host immune responses by altering intestinal microbiota. To determine the effects of oral administration of the non-digestible oligosaccharide raffinose in a food allergy model (using ovalbumin-specific T-cell receptor transgenic mice), IL-4 production by intestinal lymph node cells and serum antibody (IgE) titers were measured. Raffinose significantly suppressed IgE elevation and inhibited the Th2 response characteristic of the onset of food allergy [Citation122]. Furthermore, it has been shown that oral administration of FOS in this food allergy model attenuates the induction of intestinal Th2 cytokine (IL-4, IL-10, and IL-13) responses by regulating early activation of naïve CD4+ T cells, which produce both Th1 and Th2 cytokines [Citation123]. The inflammation-regulation effect of FOS ingestion has also been examined in a mouse model of airway inflammation induced by the house dust mite allergen Dermatophagoides farinae (Der f). FOS lowered serum allergen-specific IgG1 levels. In addition, FOS tends to suppress pulmonary production of IL-5 and eotaxin, which is enhanced by mite allergens, suggesting that FOS can prevent or improve the airway inflammation induced by mite allergens [Citation124].
Prebiotics alter the composition of the intestinal microbiota and at the same time change the intestinal environment via metabolites such as SCFAs produced by intestinal commensal bacteria. It has recently been reported that intestinal soluble factors such as SCFAs can induce immunoregulation in the intestinal immune system. Because the intestinal tract contains foreign substances such as food components and enormous numbers of bacteria, it must maintain homeostasis while minimizing inflammatory reactions. It is thus important that hypersensitive immune responses be controlled. Certain bacteria in the intestinal microbiota induce the differentiation of particular T-cell subsets. For example, Clostridium induces the differentiation of peripheral-induced Tregs in the mouse large intestine. Among the SCFAs produced by intestinal bacteria, butyric acid is a strong inducer of Tregs [Citation125].
Polysaccharides
Primarily, the suppressive effects of polysaccharides on allergic responses are closely related to their immune-potentiating activity, which enhances innate immune responses to increase IL-12 production, leading to the upregulation of Th1 and NK cell activity. From the view point of allergy suppression, the enhancement of Th1 responses results in correction of the Th1/Th2 balance; other mechanisms include the enhancement of immune-suppressive functions, especially the induction of Tregs or increase in the production of immune-suppressive cytokines such as IL-10 and TGF-β.
Anti-allergic effects of β-glucan were reported in a mouse model [Citation126] and a clinical trial for Japanese cedar polinosis patients [Citation127]. Suppression of Th2 responses [Citation128] and inhibition of IgE production [Citation129] by fucoidan were also reported in mouse models. Additionally, dietary oat β-glucan [Citation130] and fucoidans [Citation131] have been effective in ameliorating colitis-associated symptoms and decreasing the expression of pro-inflammatory cytokines in colonic tissues in DSS-induced colitis mice. Oral administration of oat β-glucan has also shown protective effects in LPS-induced enteritis [Citation132].
Fatty acids
Polyunsaturated fatty acids (PUFAs) are classified as ω6 fatty acids such as arachidonic acid and linoleic acid, and ω3 fatty acids such as eicosapentaenoic acid (EPA) and docosahexaenoic acid (DHA). ω6 PUFAs give rise to pro-inflammatory eicosanoids, whereas ω3 PUFAs are metabolized into less inflammatory or anti-inflammatory eicosanoids [Citation133]. Therefore, the intake of oils rich in ω3 PUFAs is expected to reduce the risk of diseases caused by inflammatory responses, and the beneficial effects of ω3 PUFAs have been demonstrated in a range of animal models for inflammatory conditions and in human trials for rheumatoid arthritis [Citation134]. However, in regards to the effect on the development of allergies, such as asthma, a recent meta-analysis of randomized clinical trials for ω3 PUFA supplementation did not show improvement in clinical outcomes [Citation135]. In line with this observation, it was recently found that ω3 PUFA epoxides are produced in mast cells, and these compounds promote IgE-mediated activation of mast cells, suggesting the enhancing effect of ω3 PUFAs on allergic reactions [Citation136].
Vitamins and other micronutrients
As already described, retinoic acid has been shown to be critical for the differentiation of Tregs [Citation8]. Besides maintaining calcium homeostasis and bone health, vitamin D affects the innate and adaptive immune responses [Citation137,138]. The active forms of vitamin D have been shown to promote the induction of Tregs and tolerogenic dendritic cells, and therefore, a beneficial effect has been suggested for the prevention and treatment of IBD and autoimmune diseases such as type 1 diabetes mellitus and multiple sclerosis [Citation137,138]. A recent systematic review of clinical trials for vitamin D supplementation for the prevention of allergy development found no significant evidence, because of the limited information available, and concluded that its potential impact remains uncertain [Citation139].
Vitamin B9 (folate) is also known to be essential for the maintenance of Tregs [Citation140], which express high levels of the folate receptor (FR4) [Citation141]. It has been reported that vitamin B9 deficiency causes intestinal inflammation [Citation142].
Concerning minerals, an inverse association between selenium levels and IBD severity have been suggested in epidemiological studies [Citation143], and the protective effect of selenium supplementation has been reported in DSS-induced colitis mice [Citation144].
Peptides, proteins and related substances
Some kinds of peptides are known to have anti-inflammatory functions. Carnosine (β-alanyl-l-histidine), which is an imidazole dipeptide found in various kinds of meat and fish, was shown to have an anti-inflammatory effect in an intestinal epithelial cell line [Citation145]. When a human intestinal cell line, Caco2, was stimulated by either hydrogen peroxide or TNF-α, the secretion of IL-8, an inflammatory cytokine, was suppressed in the presence of carnosine. In a double-blind, randomized, placebo-controlled clinical trial of healthy volunteers (60 years of age or older), it was demonstrated that the intake of an imidazole dipeptide-enriched extract from chicken meat for three months reduced the serum levels of IL-8 and improved cognitive function [Citation146]. Milk basic protein show an anti-inflammatory effect in a food–allergic enteropathy model mouse [Citation147]. Polyamines which are formed in Arg metabolism, are known to inhibit production of inflammatory cytokines by suppressing expression of LFA-1 on immune cells [Citation148,149].
Polyphenols
The immune-suppressive effects of polyphenols include modulation of T-cell functions, inhibition of mast cell degranulation, and downregulation of inflammatory cytokine responses. In a mouse model, dietary supplementation of a green-tea polyphenol, epigallocatechin-3-gallate (EGCG), demonstrated a decrease in the Th1 and Th17 populations [Citation150]. Baicalin, a flavone glycoside found in the Chinese medical herb Huangqin (Scutellaria baicalensis; Koganebana in Japanese), was demonstrated to have an inhibitory effect on Th17 cell differentiation [Citation151]. Some polyphenols, including EGCG [Citation152] and baicalin [Citation153], have been demonstrated to upregulate Treg induction in mice. Naringenin, which can activate aryl hydrocarbon receptor (AhR)-mediated gene transcription, promoted Treg-inducing activity through AhR-mediated responses in an in vitro assay, using mouse naïve CD4+ T cells [Citation154].
As for the inhibition of an allergic reaction, epigallocatechin-3-O-(3-O-methyl) gallate (EGCG3″Me) isolated from Cv. ‘Benifuuki’ green tea has been demonstrated to inhibit the degranulation of human mast cells [Citation155] and thus, to alleviate allergy symptoms in human clinical trials [Citation156]. Naringenin chalcone, isolated from tomato skin extract, has also been revealed to have an anti-allergic effect, mediated by inhibiting degranulation of mast cells [Citation157].
Anti-inflammatory effects of polyphenols have been reported for numerous kinds of polyphenolic compounds [Citation85]. For instance, quercetin is a flavonol that is found in a wide range of fruits and vegetables and has multiple biological effects. In addition to chatechins, quercetin is one of the most extensively studied polyphenols, and its anti-inflammatory activity has been reported, based on animal models and also human clinical trials [Citation158]. When administered orally, the biological activity of quercetin glycoside in vivo was much higher than the activity of the other forms of quercetin [Citation159]. Chlorogenic acid has been reported to inhibit the secretion of the chemokine IL-8 from human intestinal epithelium-derived Caco-2 cells and to decrease the amount of the chemokine MIP-2 in the intestine of DSS-induced colitis mice [Citation159]. Although the immune-modulatory effect of polyphenols in humans has not yet been fully substantiated by the published clinical studies, a systematic review reported that the effect of flavonoid-rich foods on reducing serum TNF-α levels seems valid in inflammatory situations, but not in healthy people [Citation160].
Conclusions
Both enhancement and inhibition of immune responses by food components described in this review are summarized in Table . Studies using animal and cellular models are revealing the cellular and molecular mechanisms of immunomodulation. In addition, the receptors recognizing food components, as well as the intracellular pathways involved, are being defined for individual cases. Such scientific evidence will be important in the development of functional foods. Another important issue is the examination of immune responses in individuals not in the diseased state to ascertain the distribution of individual parameters. We are convinced that the elucidation of immunomodulation by food will help promote good health.
Table 1. Immunomodulation by food components.
Disclosure statement
No potential conflict of interest was reported by the authors.
References
- Tangye SG, Ma CS, Brink R, et al. The good, the bad and the ugly - TFH cells in human health and disease. Nat Rev Immunol. 2013;13:412–426.
- Qi H. T follicular helper cells in space-time. Nat Rev Immunol. 2016;16:612–625.
- Walker JA, Barlow JL, McKenzie AN. Innate lymphoid cells–how did we miss them? Nat Rev Immunol. 2013;13:75–87.
- Eberl G, Colonna M, Di Santo JP, et al. Innate lymphoid cells: a new paradigm in immunology. Science. 2015;348:aaa6566.
- Suzuki K, Kawamoto S, Maruya M, et al. GALT: organization and dynamics leading to IgA synthesis. Adv Immunol. 2010;107:153–185.
- Rezende RM, Weiner HL. History and mechanisms of oral tolerance. Semin Immunol. 2017;30:3–11.
- Ise W, Nakamura K, Shimizu N, et al. Orally tolerized T cells can form conjugates with APCs but are defective in immunological synapse formation. J Immunol. 2005;175:829–838.
- Mucida D, Park Y, Kim G, et al. Reciprocal TH17 and regulatory T cell differentiation mediated by retinoic acid. Science. 2007;317:256–260.
- Shiokawa A, Kotaki R, Takano T, et al. Mesenteric lymph node CD11b- CD103+ PD-L1High dendritic cells highly induce regulatory T cells. Immunology. 2017;152:52–64.
- Sato A, Hashiguchi M, Toda E, et al. CD11b+ Generation of gut-homing IgA-secreting B cells by intestinal dendritic cells. Peyer’s patch dendritic cells secrete IL-6 and induce IgA secretion from naive B cells. J Immunol. 2003;171:3684–3690.
- Mora JR, Iwata M, Eksteen B, et al. Generation of gut-homing IgA-secreting B cells by intestinal dendritic cells. Science. 2006;314:1157–1160.
- Tezuka H, Abe Y, Iwata M, et al. Regulation of IgA production by naturally occurring TNF/iNOS-producing dendritic cells. Nature. 2007;448:929–933.
- Goodrich ME, McGee DW. Preferential enhancement of B cell IgA secretion by intestinal epithelial cell-derived cytokines and interleukin-2. Immunol Invest. 1999;28:67–75.
- Atarashi K, Umesaki Y, Honda K. Microbiotal influence on T cell subset development. Semin Immunol. 2011;23:146–153.
- Hattori M, Taylor TD. The human intestinal microbiome: a new frontier of human biology. DNA Res. 2009;16:1–12.
- Umesaki Y, Okada Y, Matsumoto S, et al. Segmented filamentous bacteria are indigenous intestinal bacteria that activate intraepithelial lymphocytes and induce MHC class II molecules and fucosyl asialo GM1 glycolipids on the small intestinal epithelial cells in the ex-germ-free mouse. Microbiol Immunol. 1995;39:555–562.
- Yanagibashi T, Hosono A, Oyama A, et al. IgA production in the large intestine is modulated by a different mechanism than in the small intestine: Bacteroides acidifaciens promotes IgA production in the large intestine by inducing germinal center formation and increasing the number of IgA+ B cells. Immunobiology. 2013;218:645–651.
- Sudo N, Sawamura S, Tanaka K, et al. The requirement of intestinal bacterial flora for the development of an IgE production system fully susceptible to oral tolerance induction. J Immunol. 1997;159:1739–1745.
- Agache I, Sugita K, Morita H, et al. The complex type 2 endotype in allergy and asthma: from laboratory to bedside. Curr Allergy Asthma Rep. 2015;15:29.
- Takahashi T, Nakagawa E, Nara T, et al. Effects of orally ingested Bifidobacterium longum on the mucosal IgA response of mice to dietary antigens. Biosci Biotechnol Biochem. 1998;62:10–15.
- Tominari K, Sakai F, Yasui H. Prevention of rotavirus-induced diarrhea by preferential secretion of IgA in breast milk via maternal administration of Lactobacillus gasseri SBT2055. J Pediatr Gastroenterol Nutr. 2012;55:66–71.
- Kikuchi Y, Kunitoh-Asari A, Hayakawa K, et al. Oral administration of Lactobacillus plantarum strain AYA enhances IgA secretion and provides survival protection against influenza virus infection in mice. PLoS One. 2014;9:e86416.
- Sakai F, Hosoya T, Ono-Ohmachi A, et al. Lactobacillus gasseri SBT2055 induces TGF-β expression in dendritic cells and activates TLR2 signal to produce IgA in the small intestine. PLoS One. 2014;9:e105370.
- Kotani Y, Kunisawa J, Suzuki Y, et al. Role of Lactobacillus pentosus Strain b240 and the Toll-like receptor 2 axis in Peyer’s patch dendritic cell-mediated immunoglobulin A enhancement. PLoS One. 2014;9:e91857.
- Nakamura Y, Terahara M, Iwamoto T, et al. Upregulation of Polymeric Immunoglobulin Receptor Expression by the Heat-Inactivated Potential Probiotic Bifidobacterium bifidum OLB6378 in a Mouse Intestinal Explant Model. Scand J Immunol. 2012;75:176–183.
- Takeda K, Suzuki T, Shimada SI, et al. Interleukin-12 is involved in the enhancement of human natural killer cell activity by Lactobacillus casei Shirota. Clin Exp Immunol. 2006;146:109–115.
- Makino S, Sato A, Goto A, et al. Enhanced natural killer cell activation by exopolysaccharides derived from yogurt fermented with Lactobacillus delbrueckii ssp. bulgaricus OLL1073R-1. J Dairy Sci. 2016;99:915–923.
- Ishizuka T, Kanmani P, Kobayashi H, et al. Immunobiotic Bifidobacteria Strains Modulate Rotavirus Immune Response in Porcine Intestinal Epitheliocytes via Pattern Recognition Receptor Signaling. PLoS One. 2016;11:e0152416.
- Hori T, Kiyoshima J, Shida K, et al. Augmentation of cellular immunity and reduction of influenza virus titer in aged mice fed Lactobacillus casei strain Shirota. Clin Diagn Lab Immunol. 2002;9:105–108.
- Hosono A, Ozawa A, Kato R, et al. Dietary fructooligosaccharides induce immunoregulation of intestinal IgA secretion by murine Peyer’s patch cells. Biosci Biotechnol Biochem. 2003;67:758–764.
- Nakanishi Y, Murashima K, Ohara H, et al. Increase in terminal restriction fragments of Bacteroidetes-derived 16S rRNA genes after administration of short-chain fructooligosaccharides. Appl Environ Microbiol. 2006;72:6271–6276.
- Bakker-Zierikzee AM, Tol EA, Kroes H, et al. Faecal SIgA secretion in infants fed on pre- or probiotic infant formula. Pediatr Allergy Immunol. 2006;17:134–140.
- Fukuda S, Toh H, Hase K, et al. Bifidobacteria can protect from enteropathogenic infection through production of acetate. Nature. 2011;469:543–547.
- Kim MH, Kang SG, Park JH, et al. Short-chain fatty acids activate GPR41 and GPR43 on intestinal epithelial cells to promote inflammatory responses in mice. Gastroenterology. 2013;145:396–406.e1-10.
- Dalonso N, Goldman GH, Gern RMM. β-(1→3), (1→6)-Glucans: medicinal activities, characterization, biosynthesis and new horizons. Appl Microbiol Biotechnol. 2015;99:7893–7906.
- Brown GD, Gordon S. Immune recognition of fungal β-glucan. Cell Microbiol. 2005;7:471–479.
- Zekovic DB, Kwiatkowski S, Vrvic MM, et al. Natural and modified (1→3)-β-D-glucan in health promotion and disease alleviation. Crit Rev Biotechnol. 2005;25:205–230.
- Shi SH, Yang WT, Huang KY, et al. β-glucan from Coriolus versicolor protect mice against S. typhimurium challenge by activation of macrophages. Int J Biol Macromol. 2016;86:352–361.
- Davis JM, Murphy EA, Brown AS, et al. Effects of moderate exercise and oat beta-glucan on innate immune function and susceptibility to respiratory infection. Am J Physiol Regul Integr Comp Physiol. 2004;286:R366–R372.
- Yun CH, Estrada A, Van Kessel A, et al. β-Glucan, extracted from oat, enhances disease resistance against bacterial and parasitic infections. FEMS Immunol Med Microbiol. 2003;35:67–75.
- Dambuza IM, Brown GD. C-type lectins in immunity: recent developments. Current Opin Immunol. 2015;32:21–27.
- Kakutani R, Adachi Y, Kajiura H, et al. Relationship between structure and immunostimulating activity of enzymatically synthesized glycogen. Carbohydr Res. 2007;342:2371–2379.
- Kakutani R, Adachi Y, Takata H, et al. Essential role of Toll-like receptor 2 in macrophage activation by glycogen. Glycobiology. 2012;22:146–159.
- Kakutani R, Adachi Y, Kajiura H, et al. The effect of orally administered glycogen on anti-tumor activity and natural killer cell activity in mice. Int Immunopharmacol. 2012;12:80–87.
- Maruyama H, Tamauchi H, Iizuka M, et al. The Role of NK cells in antitumor activity of dietary fucoidan from Undaria pinnatifida sporophylls (mekabu). Planta Med. 2006;72:1415–1417.
- Hayashi K, Nakano T, Hashimoto M, et al. Defensive effects of a fucoidan from brown alga Undaria pinnatifida against herpes simplex virus infection. Int Immunopharmacol. 2008;8:109–116.
- Hayashi K, Lee J-B, Nakano T, et al. Anti-influenza A virus characteristics of a fucoidan from sporophyll of Undaria pinnatifida in mice with normal and compromised immunity. Microb Infection. 2013;15:302–309.
- Zou P, Yang X, Wang J, et al. Advances in characterisation and biological activities of chitosan and chitosan oligosaccharides. Food Chem. 2016;190:1174–1181.
- Lundahl MLE, Scanlan EM, Lavelle EC. Therapeutic potential of carbohydrates as regulators of macrophage activation. Biochem Pharmacol. 2017;146:23–41.
- Ma J-X, Qian L, Zhou Y. Stimulation effect of chitosan on the immunity of radiotherapy patients suffered from lung cancer. Int J Biol Macromol. 2015;72:195–198.
- Iwata M, Hirakiyama A, Eshima Y, et al. Retinoic acid imprints gut-homing specificity on T cells. Immunity. 2004;21:527–538.
- Kim MH, Taparowsky EJ, Kim CH. Retinoic acid differentially regulates the migration of innate lymphoid cell subsets to the gut. Immunity. 2015;43:107–119.
- Gebremichael A, Levy EM, Corwin LM. Adherent cell requirement for the effect of vitamin E on in vitro antibody synthesis. J Nutr. 1984;114:1297–1305.
- Kowdley KV, Mason JB, Meydani SN, et al. Vitamin E deficiency and impaired cellular immunity related to intestinal fat malabsorption. Gastroenterology. 1992;102:2139–2142.
- Pae M, Wu D. Nutritional modulation of age-related changes in the immune system and risk of infection. Nutr Res. 2017;41:14–35.
- Jubri Z, Latif AA, Top AG, et al. Perturbation of cellular immune functions in cigarette smokers and protection by palm oil vitamin E supplementation. Nutr J. 2013;12:2–13.
- Pearce EL, Pearce EJ. Metabolic pathways in immune cell activation and quiescence. Immunity. 2013;38:633–643.
- Kunisawa J, Sugiura Y, Wake T, et al. Mode of bioenergetic metabolism during B cell differentiation in the intestine determines the distinct requirement for vitamin B1. Cell Rep. 2015;13:122–131.
- Huskisson E, Maggini S, Ruf M. The role of vitamins and minerals in energy metabolism and well-being. J Int Med Res. 2007;35:277–289.
- Corbett AJ, Eckle SB, Birkinshaw RW, et al. T-cell activation by transitory neo-antigens derived from distinct microbial pathways. Nature. 2014;509:361–365.
- Cowley SC. MAIT cells and pathogen defense. Cell Mol Life Sci. 2014;71:4831–4840.
- Gammoh NZ, Rink L. Zinc in infection and inflammation. Nutrients. 2017;9:624.
- Zinc Investigators’ Collaborative Group. Prevention of diarrhea and pneumonia by zinc supplementation in children in developing countries: pooled analysis of randomized controlled trials. J Pediatr. 1999;135:689–697.
- Haase H, Rink L. Functional significance of zinc-related signaling pathways in immune cells. Annu Rev Nutr. 2009;29:133–152.
- McKenzie RC, Rafferty TS, Beckett GJ. Selenium: an essential element for immune function. Immunol Today. 1998;19:342–345.
- Connelly-Frost A, Poole C, Satia JA, et al. Selenium, folate, and colon cancer. Nutr Cancer. 2009;61:165–178.
- Galli C, Calder PC. Effects of fat and fatty acid intake on inflammatory and immune responses: a critical review. Ann Nutr Metab. 2009;55:123–139.
- de Jong AJ, Kloppenburg M, Toes REM, et al. Fatty acids, lipid mediators, and T-cell function. Front Immunol. 2014;5:483.
- Kunisawa J, Hashimoto E, Inoue A, et al. Regulation of intestinal IgA responses by dietary palmitic acid and its metabolism. J Immunol. 2014;193:1666–1671.
- Lamas B, Vergnaud-Gauduchon J, Goncalves-Mendes N, et al. Altered functions of natural killer cells in response to L-arginine availability. Cell Immunol. 2012;280:182–190.
- Rodriguez PC, Quiceno DG, Ochoa AC. L-arginine availability regulates T-lymphocyte cell-cycle progression. Blood. 2007;109:1568–1573.
- Rodríguez PC, Ochoa AC. Arginine regulation by myeloid derived suppressor cells and tolerance in cancer: mechanisms and therapeutic perspectives. Immunol Rev. 2008;222:180–191.
- Norian LA, Rodriguez PC, O’Mara LA, et al. Tumor-infiltrating regulatory dendritic cells inhibit CD8+ T cell function via L-arginine metabolism. Cancer Res. 2009;69:3086–3094.
- Oberlies J, Watzl C, Giese T, et al. Regulation of NK cell function by human granulocyte arginase. J Immunol. 2009;182:5259–5267.
- Dufour C, Dandrifosse G, Forget P, et al. Spermine and spermidine induce intestinal maturation in the rat. Gastroenterology. 1988;95:112–116.
- Deloyer P, Peulen O, Dandrifosse G. Dietary polyamines and non-neoplastic growth and disease. Eur J Gastroenterol Hepatol. 2001;13:1027–1032.
- van der Hulst RR, von Meyenfeldt MF, Soeters PB. Glutamine: an essential amino acid for the gut. Nutrition. 1996;12:S78–S81.
- Calder PC, Yaqoob P. Glutamine and the immune system. Amino Acids. 1999;17:227–241.
- Santos RG, Quirino IE, Viana ML, et al. Effects of nitric oxide synthase inhibition on glutamine action in a bacterial translocation model. Br J Nutr. 2014;111:93–100.
- Klysz D, Tai X, Robert PA, et al. Glutamine- dependent -ketoglutarate production regulates the balance between T helper 1 cell and regulatory T cell generation. Sci Signal. 2015;8:ra97–ra97.
- Fallarino F, Grohmann U, You S, et al. Tryptophan catabolism generates autoimmune-preventive regulatory T cells. Transpl Immunol. 2006;17:58–60.
- Yamamoto S, Hayaishi O. Tryptophan pyrrolase of rabbit intestine d-and l-tryptophan-cleaving enzyme or enzymes. J Biol Chem. 1967;242:5260–5266.
- Munn DH, Sharma MD, Baban B, et al. GCN2 kinase in T cells mediates proliferative ar- rest and anergy induction in response to indoleamine 2, 3-dioxygenase. Immunity. 2005;22:633–642.
- Manlapat AK, Kahler DJ, Chandler PR, et al. Cell-autonomous control of interferon type I expression by indoleamine 2, 3-dioxygenase in regulatory CD19+ dendritic cells. Eur J Immunol. 2007;37:1064–1071.
- Cuevas A, Saavedra N, Salazar LA, et al. Modulation of immune function by polyphenols: possible contribution of epigenetic factors. Nutrients. 2013;5:2314–2332.
- Burkard M, Leischner C, Lauer UM, et al. Dietary flavonoids and modulation of natural killer cells: implications in malignant and viral diseases. J Nutr Biochem. 2017;46:1–12.
- Romero-Pérez GA, Egashira M, Harada Y, et al. Orally administered Salacia reticulata extract reduces h1n1 in uenza clinical symptoms in Murine lung Tissues Putatively Due to enhanced natural Killer cell activity. Front Immunol. 2016;7:115.
- Segawa S, Fujiya M, Konishi H, et al. Probiotic-derived polyphosphate enhances the epithelial barrier function and maintains intestinal homeostasis through integrin-p38 MAPK pathway. PLoS One. 2011;6:e23278.
- Carver JD. Dietary nucleotides: effects on the immune and gastrointestinal systems. Acta Pediatr Suppl. 1999;430:83–88.
- Grimble GK, Westwood OM. Nucleotides as immunomodulators in clinical nutrition. Curr Opin Clin Nutr Metab Care. 2001;4:57–64.
- Nagafuchi S, Hachimura S, Totsuka M, et al. Dietary nucleotides can up-regulate antigen-specific Th1 immune responses and suppress antigen-specific IgE responses in mice. Int Arch Allergy Immunol. 2000;122:33–41.
- Nagafuchi S, Totsuka M, Hachimura S, et al. Dietary nucleotides increase the proportion of a TCRγδ+ subset of intraepithelial lymphocytes (IEL) and IL-7 production by intestinal epithelial cells (IEC); Implications for modification of cellular and molecular cross-talk between IEL and IEC by dietary nucleotides. Biosci Biotechnol Biochem. 2000;64:1459–1465.
- Nagafuchi S, Totsuka M, Hachimura S, et al. Dietary nucleotides increase the mucosal IgA response and the secretion of transforming growth factor β from intestinal epithelial cells in mice. Cytotechnology. 2002;40:49–58.
- Watanabe M, Watanabe J, Sonoyama K, et al. Novel method for producing hypoallergenic wheat flour by enzymatic fragmentation of the constituent allergens and its application to food processing. Biosci Biotechnol Biochem. 2000;64:2663–2667.
- Du Toit G, Roberts G, Sayre PH, et al. Randomized trial of peanut consumption in infants at risk for peanut allergy. N Engl J Med. 2015;372:803–813.
- Fukuda K, Ishida W, Harada Y, et al. Efficacy of oral immunotherapy with a rice-based edible vaccine containing hypoallergenic Japanese cedar pollen allergens for treatment of established allergic conjunctivitis in mice. Allergol Int. 2017;S1323-8930(17):30070-9.
- Björkstén B, Sepp E, Julge K, et al. Allergy development and the intestinal microflora during the first year of life. J Allergy Clin Immunol. 2001;108:516–520.
- Kalliomäki M, Kirjavainen P, Eerola E, et al. Distinct patterns of neonatal gut microflora in infants in whom atopy was and was not developing. J Allergy Clin Immunol. 2001;107:129–134.
- Kalliomäki M, Salminen S, Arvilommi H, et al. Probiotics in primary prevention of atopic disease: a randomised placebo-controlled trial. Lancet. 2001;357:1076–1079.
- Ishida Y, Nakamura F, Kanzato H, et al. Effect of milk fermented with Lactobacillus acidophilus strain L-92 on symptoms of Japanese cedar pollen allergy: a randomized placebo-controlled trial. Biosci Biotechnol Biochem. 2005;69:1652–1660.
- Xiao JZ, Kondo S, Yanagisawa N, et al. Probiotics in the treatment of Japanese cedar pollinosis: a double-blind placebo-controlled trial. Clin Exp Allergy. 2006;36:1425–1435.
- Torii S, Torii A, Itoh K, et al. Effects of oral administration of Lactobacillus acidophilus L-92 on the symptoms and serum markers of atopic dermatitis in children. Int Arch Allergy Immunol. 2011;154:236–245.
- Ishida Y, Nakamura F, Kanzato H, et al. Clinical effects of Lactobacillus acidophilus strain L-92 on perennial allergic rhinitis: a double-blind, placebo-controlled study. J Dairy Sci. 2005;88:527–533.
- Lee J, Seto D, Bielory L. Meta-analysis of clinical trials of probiotics for prevention and treatment of pediatric atopic dermatitis. J Allergy Clin Immunol. 2008;121:116–121.
- Elazab N, Mendy A, Gasana J, et al. Probiotic administration in early life, atopy, and asthma: a meta-analysis of clinical trials. Pediatrics. 2013;132:e666–76.
- Shida K, Makino K, Morishita A, et al. Lactobacillus casei inhibits antigen-induced IgE secretion through regulation of cytokine production in murine splenocyte cultures. Int Arch Allergy Immunol. 1998;115:278–287.
- Murosaki S, Yamamoto Y, Ito K, et al. Heat-killed Lactobacillus plantarum L-137 suppresses naturally fed antigen-specific IgE production by stimulation of IL-12 production in mice. J Allergy Clin Immunol. 1998;102:57–64.
- Fujiwara D, Inoue S, Wakabayashi H, et al. The anti-allergic effects of lactic acid bacteria are strain dependent and mediated by effects on both Th1/Th2 cytokine expression and balance. Int Arch Allergy Immunol. 2004;135:205–215.
- Kanzato H, Fujiwara S, Ise W, et al. Lactobacillus acidophilus strain L-92 induces apoptosis of antigen-stimulated T cells by modulating dendritic cell function. Immunobiology. 2008;213:399–408.
- Yoshida T, Fujiwara W, Enomoto M, et al. An increased number of CD4+CD25+ cells induced by an oral administration of Lactobacillus plantarum NRIC0380 are involved in antiallergic activity. Int Arch Allergy Immunol. 2013;162:283–289.
- Noguchi S, Hattori M, Sugiyama H, et al. Lactobacillus plantarum NRIC1832 enhances IL-10 production from CD4⁺ T cells in vitro. Biosci Biotechnol Biochem. 2012;76:1925–1931.
- Yoshida T, Enomoto M, Nakayama S, et al. Induction of ALDH activity in intestinal dendritic cells by Lactobacillus plantarum NRIC0380. Biosci Biotechnol Biochem. 2013;77:1826–1831.
- Iwabuchi N, Takahashi N, Xiao JZ, et al. Suppressive effects of Bifidobacterium longum on the production of Th2-attracting chemokines induced with T cell-antigen-presenting cell interactions. FEMS Immunol Med Microbiol. 2009;55:324–334.
- Aoki-Yoshida A, Yamada K, Hachimura S, et al. Enhancement of oral tolerance induction in DO11.10 mice by Lactobacillus gasseri OLL2809 via Increase of Effector Regulatory T Cells. PLoS One. 2016;11:e0158643.
- Miyauchi E, Ogita T, Miyamoto J, et al. Bifidobacterium longum alleviates dextran sulfate sodium-induced colitis by suppressing IL-17A response: involvement of intestinal epithelial costimulatory molecules. PLoS One. 2013;8:e79735.
- Shimazu T, Villena J, Tohno M, et al. Immunobiotic Lactobacillus jensenii elicits anti-inflammatory activity in porcine intestinal epithelial cells by modulating negative regulators of the Toll-like receptor signaling pathway. Infect Immun. 2012;80:276–288.
- Kaji R, Kiyoshima-Shibata J, et al. Bacterial teichoic acids reverse predominant IL-12 production induced by certain Lactobacillus strains into predominant IL-10 production via TLR2-dependent ERK activation in macrophages. J Immunol. 2010;184:3505–3513.
- Shida K, Kiyoshima-Shibata J, et al. Induction of interleukin-12 by Lactobacillus strains having a rigid cell wall resistant to intracellular digestion. J Dairy Sci. 2006;89:3306–3317.
- Ichikawa S, Fujii R, Fujiwara D, et al. MyD88 but not TLR2, 4 or 9 is essential for IL-12 induction by lactic acid bacteria. Biosci Biotechnol Biochem. 2007;71:3026–3032.
- Kawashima T, Kosaka A, Yan H, et al. Double-stranded RNA of intestinal commensal but not pathogenic bacteria triggers production of protective interferon-β. Immunity. 2013;38:1187–1197.
- Yanagihara S, Kanaya T, Fukuda S, et al. Uromodulin-SlpA binding dictates Lactobacillus acidophilus uptake by intestinal epithelial M cells. Int. Immunol. 2017;29:357–363.
- Nagura T, Hachimura S, Hashiguchi M, Ueda Y, Kanno T, Kikuchi H, Sayama K, Kaminogawa S. Suppressive effect of dietary raffinose on T-helper 2 cell-mediated immunity. Br J Nutr. 2002;88:421–426.
- Tsuda M, Arakawa H, Ishii N, et al. Dietary fructooligosaccharides attenuate early activation of CD4+ T cells which produce both Th1 and Th2 cytokines in the intestinal lymphoid tissues of a murine food allergy model. Int Arch Allergy Immunol. 2017;174:121–132.
- Yasuda A, Inoue KI, Sanbongi C, et al. Dietary supplementation with fructooligosaccharides attenuates airway inflammation related to house dust mite allergen in mice. Int J Immunopathol Pharmacol. 2010;23:727–735.
- Furusawa Y, Obata Y, Fukuda S, et al. Commensal microbe-derived butyrate induces the differentiation of colonic regulatory T cells. Nature. 2013;504:446–450.
- Kimura Y, Sumiyoshi M, Suzuki T, et al. Inhibitory effects of water-soluble low-molecular-weight β-(1,3–1,6) d-glucan purified from Aureobasidium pullulans GM-NH-1A1 strain on food allergic reactions in mice. Int Immunopharmacol. 2007;7:963–972.
- Yamada J, Hamuro J, Hatanaka H, et al. Alleviation of seasonal allergic symptoms with superfine beta-1,3-glucan: a randomized study. J Allergy Clin Immunol. 2007;119:1119–1126.
- Maruyama H, Tamauchi H, Hashimoto M, et al. Suppression of Th2 immune responses by mekabu fucoidan from undaria pinnatifida sporophylls. Int Arch Allergy Immunol. 2005;137:289–294.
- Oomizu S, Yanase Y, Suzuki H, et al. Fucoidan prevents C epsilon germline transcription and NF-κB p52 translocation for IgE production in B cells. Biochem Biophys Res Commun. 2006;350:501–507.
- Liu B, Lin Q, Yang T, et al. Oat β-glucan ameliorates dextran sulfate sodium (DSS)-induced ulcerative colitis in mice. Food Funct. 2015;6:3454–3463.
- Lean QY, Eri RD, Fitton JH, et al. Fucoidan extracts ameliorate acute colitis. PLoS ONE. 2015;10:e0128453.
- Suchecka D, Harasym JP, Wilczak J, et al. Antioxidative and anti-inflammatory effects of high β-glucan concentration purified aqueous extract from oat in experimental model of LPS-induced chronic enteritis. J Func Foods. 2015;14:244–254.
- Miyata J, Arita M. Role of omega-3 fatty acids and their metabolites in asthma and allergic diseases. Allergol Intern. 2015;64:27–34.
- Calder PC. Omega-3 fatty acids and inflammatory processes: from molecules to man. Biochem Soc Trans. 2017;45:1105–1115.
- Wendell SG, Baffi C, Holguin F. Fatty acids, inflammation, and asthma. J Allergy Clin Immunol. 2014;133:1255–1264.
- Shimanaka Y, Kono N, Taketomi Y, et al. Omega-3 fatty acid epoxides are autocrine mediators that control the magnitude of IgE-mediated mast cell activation. Nat Med. 2017;23(11):nm.4417.
- Prietl B, Treiber G, Pieber TR, et al. Vitamin D and immune function. Nutrients. 2013;5:2502–2521.
- Wei R, Christakos S. Mechanisms underlying the regulation of innate and adaptive immunity by Vitamin D. Nutrients. 2015;7:8251–8260.
- Yepes-Nuñez JJ, Brozek JL, Fiocchi A, et al. Vitamin D supplementation in primary allergy prevention: systematic review of randomized and non-randomized studies. Allergy. 2018;73:37–39.
- Kunisawa J, Hashimoto E, Ishikawa I, et al. A pivotal role of vitamin B9 in the maintenance of regulatory T cells in vitro and in vivo. PLoS One. 2012;7:e32094.
- Yamaguchi T, Hirota K, Nagahama K, et al. Control of immune responses by antigen-specific regulatory T cells expressing the folate receptor. Immunity. 2007;27:145–159.
- Kinoshita M, Kayama H, Kusu T, et al. Dietary folic acid promotes survival of Foxp3+ regulatory T cells in the colon. J Immunol. 2012;189:2869–2878.
- Kudva AK, Shay AE, Prabhu KS. Selenium and inflammatory bowel disease. Am J Physiol Gastrointest Liver Physiol. 2015;309:G71–G77.
- Kaushal N, Kudva AK, Patterson AD, et al. Crucial role of macrophage selenoproteins in experimental colitis. J Immunol. 2014;193:3683–3692.
- Son DO, Satsu H, Kiso Y, et al. Inhibitory effect of carnosine on interleukin-8 production in intestinal epithelial cells through translational regulation. Cytokine. 2008;42:265–276.
- Hisatsune T, Kaneko J, Kurashige H, et al. Effect of anserine/carnosine supplementation on verbal episodic memory in elderly people. J Alzheimer’s Dis. 2016;50:149–159.
- Ono-Ohmachi A, Nakajima-Adachi H, Morita Y, Kato K, Hachimura S. Milk basic protein supplementation exerts an anti-inflammatory effect in a food-allergic enteropathy model mouse. J Dairy Sci. In press.
- Soda K, Kano Y, Nakamura T, et al. Spermine, a natural polyamine, suppresses LFA-1 expression on human lymphocyte. J Immunol. 2005;175:237–245.
- Kano Y, Soda K, Konishi F. Suppression of LFA-1 expression by spermine is associated with enhanced methylation of ITGAL, the LFA-1 promoter area. PLoS One. 2013;8:e56056.
- Wang J, Pae M, Meydani SN, et al. Green tea epigallocatechin-3-gallate modulates differentiation of naive CD4+ T cells into specific lineage effector cells. J Mol Med. 2013;91:485–495.
- Yang J, Yang X, Chu Y, et al. Identification of baicalin as an immunoregulatory compound by controlling t(h)17 cell differentiation. PLoS One. 2011;6:e17164.
- Wong CP, Nguyen LP, Noh SK, et al. Induction of regulatory T cells by green tea polyphenol EGCG. Immunol Lett. 2011;139:7–13.
- Yang J, Yang X, Li M. Baicalin, a natural compound, promotes regulatory T cell differentiation. BMC Complement Altern Med. 2012;12:64.
- Wang HK, Yeh CH, Iwamoto T, et al. Dietary flavonoid naringenin induces regulatory T cells via an aryl hydrocarbon receptor mediated pathway. J Agric Food Chem. 2012;60:2171–2178.
- Tachibana H, Sunada Y, Miyase T, et al. Identification of a methylated tea catechin as an inhibitor of degranulation in human basophilic KU812 cells. Biosci Biotechnol Biochem. 2000;64:452–454.
- Maeda-Yamamoto M. Human clinical studies of tea polyphenols in allergy or life style-related diseases. Curr Pharm Des. 2013;19:6148–6155.
- Yamamoto T, Yoshimura M, Yamaguchi F, et al. Anti-allergic activity of naringenin chalcone from a tomato skin extract. Biosci Biotechnol Biochem. 2004;68:1706–1711.
- Li Y, Yao J, Han C, et al. Quercetin, inflammation and immunity. Nutrients. 2016;8:167.
- Shin HS, Bae MJ, Zhao Z, et al. Anti-inflammatory effect of chlorogenic acid on the IL-8 production in Caco-2 cells and the dextran sulfate sodium-induced colitis symptoms in C57BL/6 mice. Food Chem. 2015;168:167–175.
- Peluso I, Miglio C, Morabito G, et al. Flavonoids and immune function in human: A systematic review. Crit Rev Food Sci Nutr. 2015;55:383–395.