ABSTRACT
The epithelial sodium channel (ENaC) plays a pivotal role in sodium homeostasis, and the development of drugs that modulate ENaC activity is of great potential therapeutic relevance. We screened 6100 chemicals for their ability to activate sodium permeability of ENaC. We used a two-step strategy: a high throughput cell-based assay and an electrophysiological assay. Five compounds were identified showing common structural features including an indole or benzothiophene ring. ENaC consists of three subunits: α, β, and γ. Changing the heteromeric combination of human and mouse ENaC αβγ subunits, we found that all five compounds activated the human β subunit but not the mouse subunit. However, four of them exhibited lower activity when the human γ subunit was substituted by the mouse γ subunit. Our findings provide a structural basis for designing human ENaC activity modulators.
Abbreviations: ENaC: Epithelial sodium channel; ΔRFU: delta relative fluorescence units; EC50: Half-maximal effective concentration; Emax: maximum effect value.
Graphical Abstract
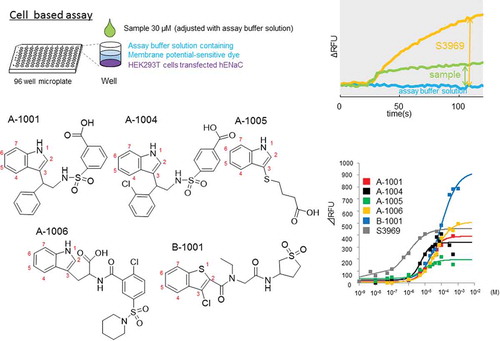
We screened 6100 chemicals for their ability to activate hENaC. Five compounds were identified showing common structural features including an indole or benzothiophene ring. All five compounds activated the human β subunit and four of them exhibited lower activity for human γ.
The epithelial sodium channel (ENaC) is a non-voltage-dependent Na+ channel belonging to the ENaC/degenerin family [Citation1–Citation3]. One ENaC subunit has two transmembrane regions and functions in a heterotrimeric configuration with two known variations: αβγ trimer, which is mainly expressed in the stomach, kidney, colon, and tongue, and δβγ trimer, which is mainly expressed in the cerebral cortex, cerebellum, hippocampus, nerve tissue, and testis [Citation4–Citation6]. With reference to the taste system, expression of ENaC αβγ subunits in human and mouse taste bud cells has been reported [Citation7,Citation8]. It is known that amiloride inhibits the Na+ taste response of the mouse chorda tympani nerve, which projects to the anterior half of the tongue [Citation9,Citation10]. Using ENaC α_KO mice, Chandrashaker et al. showed that ENaC is involved in perception of low-concentration (less than 150 mM) NaCl taste [Citation11]. In epithelial tissues other than the taste buds, ENaCs have other functions related to Na+ homeostasis. Several spontaneous mutations in human ENaC αβγ (hENaCαβγ) genes are linked to hereditary diseases including Pseudohypoaldosteronism type 1 (PHA1), Liddle’s syndrome, and Cystic fibrosis-like diseases [Citation12–Citation14]. Control of hENaCαβγ activity in vivo is therefore important in the treatment of such diseases. However, while amiloride, a strong inhibitor of hENaCαβγ, is used as a therapeutic agent in Liddle’s syndrome (the mutations in hENaC β or γ gene upregulate the activity of hENaC resulting in excessive sodium reabsorption) [Citation1,Citation15], less is known about therapeutic potential of hENaCαβγ activators. S3969, a known small molecule activator, may be useful for the treatment of sodium wasting diseases (loss of sodium reabsorption), such as PHA1 (the mutations in hENaC α or β gene cause to suppress the function of hENaC) and neonatal respiratory distress syndrome [Citation16,Citation17]. In this study, we screened a large-scale chemical library, aiming to identify other small molecule activators of hENaCαβγ, and to elucidate their mechanisms of activity.
Materials and methods
Chemicals
We screened 6100 samples from the chemical libraries of the Drug Discovery Initiative at The University of Tokyo (DDI). These samples consisted of the 4800 compounds in DDI’s core library, a natural product library of 634 compounds, and 666 additional compounds, 347 of which were selected for similarity to A-1001, and 319 of which were similar to B-1001. Compounds having 60% or more homology to A-1001 or B-1001 using software (ChemBioFinder Ultra 12.0 PerkinElmer, MA, USA) were selected as similar structural compounds. We synthesized S3969 as described previously [Citation16]. Amiloride was purchased from Sigma-Aldrich Japan (Tokyo, Japan). After screening, five selected compounds were purchased from Namiki Shoji (Tokyo, Japan).
cDNA clones
Human ENaC α, β, and γ cDNA (accession no. BC006526, BC036352, and BC059391) and mouse ENaC α, β, and γ cDNA (accession no. BC133688, BC131969, and BC021338) were purchased from Darmacon (CO, USA). Each cDNA fragment was introduced into the pEAK10 expression vector (Edge Biosystems, MD, USA) for cell-based assay, or into the house vector with T7 promoter for complementary RNA (cRNA) synthesis. Sequences of all cDNA fragments of human and mouse ENaCαβγ in both vectors were confirmed using a genetic analyzer (PRISM 3100, Applied Biosystems, CA, USA).
Cell based assay
Human embryonic kidney (HEK)293T cells were maintained in Dulbecco’s modified Eagle’s medium (DMEM 5796, Sigma-Aldrich Japan) supplemented with 10% fetal bovine serum (FBS, Invitrogen, CA, USA) at 37 °C under 5% CO2. To express ENaCαβγ proteins, ENaCαβγ cDNA (0.33 μg each, for a total of 0.99 μg) was transfected into HEK293T cells (approximately 1.2 × 106 cells per 35 mm plate) with Lipofectamine LTX (Invitrogen). Transfected cells were seeded at a density of approximately 90,000 cells/well into 96-well black-walled CellBIND surface plates (Corning, NY, USA) and cultured for 24 h at 37 °C under 5% CO2 in DMEM containing 10% FBS and 10 μM amiloride.
The human-mouse chimeric ENaCs were transfected to HEK293T cells described above. In order to confirm whether each chimeric ENaCs functions as amiloride sensitive sodium channel, whole cell patch clamp was performed. All chimeric human and mouse ENaCs with various subunit combinations responded to amiloride showing that they function as a sodium channel (data not shown).
After a 24 h of incubation, transfected cells were washed with assay buffer solution (10 mM 4-(2-hydroxyethyl)-1-piperazineethanesulfonic acid (HEPES), 130 mM NaCl, 10 mM glucose, 5 mM KCl, 2 mM CaCl2, and 1.2 mM MgCl2, pH adjusted to 7.4 with NaOH). They were then loaded with a membrane potential assay kit blue (R8042 Molecular Devices, CA, USA) and 2.5 mM probenecid (Wako, Osaka, Japan) in assay buffer solution for 30 min at 27 °C in the dark. Changes in fluorescence intensity (excitation at 530 nm, fluorescence at 560 nm) were monitored using a FlexStation 3 microplate reader (Molecular Devices) at 2 s intervals at 27 °C. After 20 s of baseline reading, an aliquot of assay buffer supplemented with 2× ligand was added, and the scanning continued for an additional 100 s. The response was indicated as delta relative fluorescence units (ΔRFU), calculated as the difference between the maximum and minimum fluorescence values. The activation ability of the sample was calculated according to the following equation: (ΔRFU30 μM sample – ΔRFUassay buffer solution)/(ΔRFU1 μM S3969 – ΔRFUassay buffer solution). Samples showing an activity ratio > 0.18 were selected for the next stage of screening. This procedure is depicted schematically in .
Figure 1. The screening procedure. (a) Cell based assay (1st screening). Compounds were selected where (ΔRFUcompound (30 μM) - ΔRFUassay buffer solution)/(ΔRFUS3969(1 μM) - ΔRFUassay buffer solution) > 0.18. (a) In our electrophysiological assay (2nd screening), compounds (n = 2) were selected where Δ I compound (30 μM)/Δ I amiloride (1 μM) > 0.3. S3969 and amiloride were used as positive and negative controls of the experiment, respectively. See methods for further details.
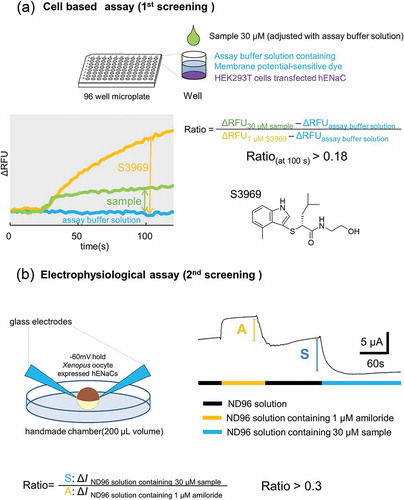
The coefficient of variation (CV) value, Signal/Background ratio (S/B), and Z-factor were used as indicators of the reliability of the plate reader-based measurement system [Citation18]. The equations used to calculate these values are as follows: CV(%) = standard deviation (SD)/average (Av); S/B = Av1 μM S3969/Avassaybuffer solution; Z-factor = 1−(3 × SD1 μM S3969 + 3 × SDassay buffer solution)/(Av1 μM S3969- Av assay buffer solution)
Electrophysiological assay
Because membrane-potential-sensitive dye reacts not only with Na+, but also with other cations such as K+, electrophysiological methods were used for the next screening.
cRNA of hENaCαβγ was transcribed in vitro using linearized plasmid DNA and T7 RNA polymerase (Stratagene, CA, USA) in the presence of the m7G(5ʹ)ppp(5ʹ)G RNA cap structure analog (New England Biolabs, MA, USA) [Citation19]. The transcribed solutions were adjusted to uniform RNA concentration using distilled water (Otsuka Pharmaceutical, Tokyo Japan) and mixed (α: β: γ = 1: 1: 1) for cRNA injection to Xenopus oocyte. Female Xenopus laevis (> 130 g) was purchased from Kato S science (Chiba, Japan) and kept at a water temperature of approximately 16 °C. Oocytes were collected following incision of the lower abdomen and placed in 15 ml collection vials with MBS solution (88 mM NaCl, 1 mM KCl, 2.4 mM NaHCO3, 15 mM HEPES, 0.3 mM Ca(NO3)2 · 4H2O, 0.41 mM CaCl2 · 2H2O, 0.82 mM MgSO4 · 7H2O, adjusted to pH 7.6 with NaOH) containing 30–60 mg collagenase (Sigma-Aldrich) and stirred for 2–4 hours at room temperature (approximately 25 °C). After stirring and washing with MBS solution, oocytes in good condition were selected. Using a puller (PB-7, NARISHIGE, Tokyo Japan), capillary glass (3000-203-G/X, Drummond CO, USA), and micro-injector (NANOJECT II, Drummond), 23.5 nL of cRNA solution (0.05 ng cRNA) was injected per oocyte. Thereafter, oocytes were left in MBS containing 10 μM amiloride at 16 °C for between 1 and 3 days before use.
Under an SZ-40 upright microscope (Olympus, Tokyo, Japan), Membrane currents were recorded using a two-electrode voltage clamping method with an OC-725C amplifier (Warner Instruments, CA, USA), and digitized using a Digidata 1550 signal converter (Axon Instruments, CA, USA). For data acquisition, we used pCLAMP 10.2 software (Axon Instruments). The bath solution (ND96 solution) contained 96 mM NaCl, 2 mM KCl, 1 mM MgCl2, 1.8 mM CaCl2 and 5 mM HEPES (pH 7.5 with NaOH). Two glass electrodes were made from capillary glass (GC150TF-10 HARVARD, CA, USA) using the puller described above. Filled with pipette solution containing 3 M KCl, they had a resistance of approximately 2 MΩ. All electrophysiological experiments were performed at room temperature (approximately 25 °C) using an acrylic handmade chamber with a volume of approximately 200 μL. Bath solution was perfused at approximately 2000 μL/min flow speed. In the bath solution, the membrane potential of the oocyte was fixed at −60 mV, and the current (ΔI) was measured based on the amount of Na+ flowing in through the hENaCαβγ before and after addition of the sample. Since the expression level of hENaCαβγ per oocyte varied, the ΔI was corrected using the ENaCαβγ inhibitor 1 μM amiloride, and evaluation scores were calculated using the following formula:
│ΔI 30 μM sample │/│ΔI 1 μM amiloride│. Solutions with scores > 0.3 were finally selected. A schematic drawing of our experimental procedures is shown in .
Data analysis
The results were expressed as mean ± standard error of the mean (SEM). All statistical analyses were conducted with JMP software (SAS, Tokyo Japan). Multiple comparisons were performed with the Tukey-Kramer test. Half-maximal effective concentration (EC50) and maximum effect value (Emax) for the ligand-receptor interactions were determined from the concentration-response curves generated using Clampfit ver.10.2 (Molecular Devices) by fitting the data to Hill’s equation. For all analyses, differences with p-values < 0.05 were considered significant.
Results
Assay system for screening hENaCαβγ activators
First, we constructed an assay system to screen hENaCαβγ activators efficiently. The first screening was a cell-based assay using HEK293T cells. This screening, performed using a plate reader, set the following target values for its parameters: CV ≦ 10%, S/B ≧ 2, and Z-factor ≧ 0.5. CV is an indicator of signal variation and S/B value indicates the background signal intensity. Z-factor is used as an index to evaluate the quality of the assay system. The calculation methods of CV, S/B and Z-factor are shown in materials and methods section. To enhance the Z-factor and S/B value, it was desirable to reduce background noise, probenecid was added to lower non-specific influx of cations. As a result, the parameters of the current cell-based assay were approximately as follows: CV ≦ 9%, S/B ≧ 1.82, and Z-factor ≧ 0.51. In general, 1 > Z-factor≧0.5 is considered to be “an excellent assay” [Citation18]. In this study, the Z-factor≧0.51 was guaranteed showing that the assay satisfied a certain level.
Identification of hENaCαβγ activators containing indole and benzothiophene rings
A total of 6100 compounds were subjected to two-step screening using cell-based and electrophysiological assays (). First, we screened 5343 compounds, 4800 from DDI’s core library and 634 from natural library. Two compounds, A-1001 and B-1001, registered significant activity. We then screened chemical libraries structurally related to A-1001 (319 compounds), and to B-1001 (347 compounds). Finally, 11 compounds were obtained: A-1001, A-1002, A-1003, A-1004, A-1005, A-1006, A-1007, B-1001, B-1002, B-1003, and B-1004 (). Among 11 compounds, five were subjected further analysis. The structure of remaining six compounds are shown in Supplementary Figure 1.
Figure 2. Chemical structure of the identified compounds. (a) Scheme of this screening. Two compounds (A-1001 and B-1001) were selected from 5434, and nine (six and three) compounds were selected from 666 (319 for A-1001 and 347 for B-1001) compounds having structures similar to the first two compounds. Marks show the compounds used for further experiments. (b) Structures of potential hENaCαβγ activators exhibited to the further experiment (, ). Structures of unmarked compounds were shown in Supplementary Figure 1. Score shows average in screening respectively (cell means 1st screening using cell based assay, oocyte means 2nd screening using electrophysiological assay).
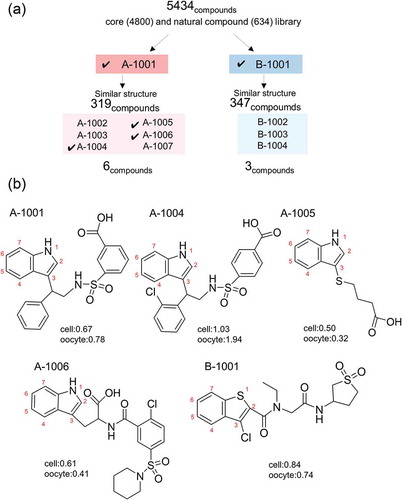
Figure 3. Dose-dependent activation of human ENaCαβγ channel by the compounds. (a) Dose-response relationships for the five selected compounds using a cell-based assay. Each point represents the mean ± S.E. from six independent measurements. Responses are indicated as ΔRFU. (b) Half- maximal effective concentration (EC50) and maximum effect value (Emax) of the five compounds were obtained by fitting the data to Hill’s equation. Colors indicate five compounds (A-1001; red, A-1004; black, A-1005; green, A-1006; yellow, B-1001; blue and S3969; gray).
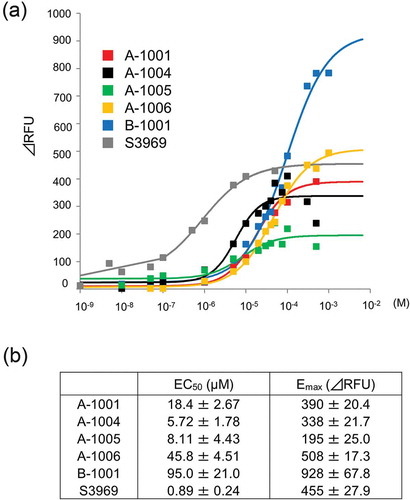
Figure 4. Effect of ENaCαβγ subunit composition on the modulatory activity of compounds. Each chimeric ENaC activation level shown was calculated as a ratio to the response value as follows:ΔRFUchimeric ENaCαβγ each compound(30 μM)/ΔRFUhENaCαβγ each compound(30 μM) Each value represents the mean ± S.E. Same letters indicate non-significant values according to the Tukey-Kramer test (p < 0.05; n = 4 for each). Colors indicate chimeric trimer combination (αHβHγH shows human ENaC(hENaC)αβγ; black, αMβHγH mouse ENaC(mENaC)α, hENaCβ and γ; yellow, αHβMγH shows hENaCα, γ and mENaCβ; blue, αHβHγM hENaCα,β and mENaCγ; green, αMβMγM mENaCαβγ; white).
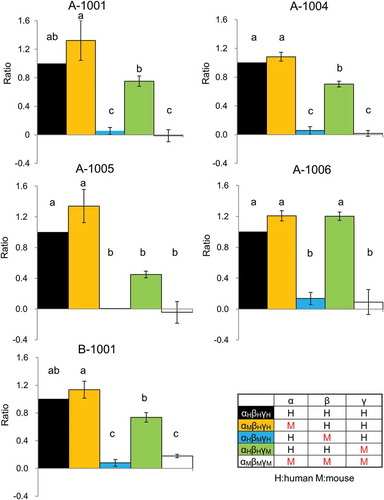
Structural characteristics of the identified compounds
Due to the limited quantity of compounds for further analysis, we focused on five compounds containing an indole ring (A-1001, A-1004, A-1005, and A-1006) and one compound containing a benzothiophene ring (B-1001). illustrates the chemical structure of the five compounds identified in this screening. All of them possess a nitrogen or sulfur atom in a heterocyclic ring. Side chains were added at position 3 (indole ring), or position 2 (benzothiophene ring). Nitrogen or sulfur atoms, which are able to construct polar groups, were present in the side chain. Hydrophilic residues were found at the other end of indole or benzothiophene ring.
Modulation of hENaCαβγ channel activity by the five identified compounds
Dose-dependent activation of hENaCαβγ by each compound was analyzed by measuring ΔRFU in HEK293 cells (). All five compounds exhibited relatively higher EC50 (5–100 µM) than S3969 (0.89 µM)(). However, B-1001, which contains a benzothiophene ring, has an approximately two-fold higher Emax value than the other compounds. This indicates that the extent of hENaCαβγ activation is maximal in the B-1001-treated cells.
Selective activation of human and mouse ENaC αβγ subunits by the compounds
We analyzed the activation mechanism of hENaCαβγ using chimeric human and mouse ENaC with various subunit combinations (). None of the five selected compounds activated mENaCαβγ. We then replaced each of the subunits from human to mouse and further examined the activation levels of the five compounds. No compound’s activity exhibited any dependency on the human α subunit, but all showed strong dependency on the human β subunit. The activation level in the αHβHγH combination was equal to that in the αMβHγH combination, and negligible in the αHβMγH combination. Interestingly, all of the compounds except A-1006 showed weak dependency on the human γ subunit, with standard activation in the αHβHγH combination and significantly less activation in the αHβHγM combination.
Discussion
We have identified structurally related compounds that show differential modulatory activity against human and mouse ENaCαβγ subunits. Our primary objective was to find a potent activator of the hENaCαβγ channel and to obtain information about its mode of action. We identified five compounds with significant activity, but all of them showed higher EC50 than the known activator S3969. This indicates that the compounds require higher concentration to obtain half-maximal activation. However, one benzothiophene-related compound, B-1001, showed approximately two-fold higher Emax value than the average of the other compounds, suggesting its potency as an hENaCαβγ enhancer. Since only one benzothiophene derivative was examined in this study, it may be useful to examine other benzothiophene derivatives. The other notable feature of the compounds we identified is an indole ring, common partial structure of S3969. Previous studies have also suggested that the indole ring is an important partial structure for activation of hENaCαβγ [Citation16]. It seems possible that these ring structures play a crucial role in hENaCαβγ-modulating activity.
The subunit selectivity we observed may also provide useful information for future chemical design efforts. The alignment of human and mouse ENaC subunits is shown in Supplementary Figure 2. Our findings relating to the sensitivity of four of the compounds to human/mouse substitution of ENaCγ subunits indicated that these compounds can modulate hENaCαβγ activity not only via the β subunit but also via the γ subunit. Whether this sensitivity can be attributed to certain amino acid differences between the human and mouse γ subunits is an interesting question. From the viewpoint of chemical structure, one obvious difference between the four sensitive compounds and the insensitive compound A-1006 is a carboxylic acid residue located close to the indole ring. It is possible that this carboxylic acid supports the specific interaction of A-1006 with the human β subunit but not with the γ subunit. Regarding the amino acid sequences of the ENaCαβγ subunits, it was shown that V348 in the human β subunit is critical for ENaC activation by S3969 [Citation16]. Other residues important for ENaC activation, include M90 and K350 [Citation20]. These residues are conserved in the human and mouse β subunits, but those in the corresponding position in human and mouse γ subunits are different (L for M90, L for V348 and E for K350). Further study using human/mouse substitution mutants of the γ subunit may provide clues to the mechanism underlying this γ subunit-dependency, and insight to aid chemical design of hENaCαβγ channel activators.
Author contributions
YK, TS, TM, KA, and TA designed the project. YK, RM, and TS conducted experiments and analyzed data. TS, NM and HW synthesized S3969.YK, MN, AY, KA, and TA interpreted the data. TO and HK provided samples and advice. YK, MN, AY, and TA wrote the manuscript. All authors critically read and contributed to the manuscript.
Supplementary_figure1_and_figure2.pdf
Download PDF (256.4 KB)Acknowledgments
This research was (partially) supported by the Platform Project for Supporting Drug Discovery and Life Science Research from AMED under Grant Number JP17am0101086.
Disclosure statement
No potential conflict of interest was reported by the authors.
Supplemental Material
Supplemental data for this article can be accessed here.
Additional information
Funding
References
- Canessa CM, Horisberger JD, Rossier BC. Epithelial sodium channel related to proteins involved in neurodegeneration. Nature. 1993;361(6411):467.
- Canessa CM, Schild L, Buell G, et al. Amiloride-sensitive epithelial Na+ channel is made of three homologous subunits. Nature. 1994;367(6462):463.
- Kellenberger S, Gautschi I, Schild L. An external site controls closing of the epithelial Na+ channel ENaC. J Physiol. 2002;543(2):413–424.
- Waldmann R, Champigny G, Bassilana F, et al. Molecular cloning and functional expression of a novel amiloride-sensitive Na+ channel. J Biol Chem. 1995;270(46):27411–27414.
- Yamamura H, Ugawa S, Ueda T, et al. Protons activate the δ-subunit of the epithelial Na+ channel in humans. J Biol Chem. 2004;279(13):12529–12534.
- Staruschenko A, Adams E, Booth RE, et al. Epithelial Na+ channel subunit stoichiometry. Biophys J. 2005;88(6):3966–3975.
- Kretz O, Barbry P, Bock R, et al. Differential expression of RNA and protein of the three pore-forming subunits of the amiloride-sensitive epithelial sodium channel in taste buds of the rat. J Histochem Cytochemistry. 1999;47(1):51–64.
- Lin W, Finger TE, Rossier BC, et al. Epithelial Na+ channel subunits in rat taste cells: localization and regulation by aldosterone. J Comp Neurol. 1999;405(3):406–420.
- Brand JG, Teeter JH, Silver WL. Inhibition by amiloride of chorda tympani responses evoked by monovalent salts. Brain Res. 1985;334(2):207–214.
- Ninomiya Y, Funakoshi M. Amiloride inhibition of responses of rat single chorda tympani fibers to chemical and electrical tongue stimulations. Brain Res. 1988;451(1–2):319–325.
- Chandrashekar J, Kuhn C, Oka Y, et al. The cells and peripheral representation of sodium taste in mice. Nature. 2010;464(7286):297.
- Geller DS, Rodriguez-Soriano J, Boado AV, et al. Mutations in the mineralocorticoid receptor gene cause autosomal dominant pseudohypoaldosteronism type I. Nat Genet. 1998;19(3):279.
- Kellenberger S, Gautschi I, Rossier BC, et al. Mutations causing Liddle syndrome reduce sodium-dependent downregulation of the epithelial sodium channel in the Xenopus oocyte expression system. J Clin Invest. 1998;101(12):2741–2750.
- Mall M, Grubb BR, Harkema JR, et al. Increased airway epithelial Na+ absorption produces cystic fibrosis-like lung disease in mice. Nat Med. 2004;10(5):487.
- Baker E, Jeunemaitre X, Portal AJ, et al. Abnormalities of nasal potential difference measurement in Liddle’s syndrome. J Clin Invest. 1998;102(1):10–14.
- Lu M, Echeverri F, Kalabat D, et al. Small molecule activator of the human epithelial sodium channel. J Biol Chem. 2008;283(18):11981–11994.
- Chang, SS, Grunder, S, et al. Mutations in subunits of the epithelial sodium channel cause salt wasting with hyperkalaemic acidosis, pseudohypoaldosteronism type 1. Nat Genet. 1996;12(3):248.
- Zhang JH, Chung TD, Oldenburg KR. A simple statistical parameter for use in evaluation and validation of high throughput screening assays. J Biomol Screen. 1999;4(2):67–73.
- Michael. L, Eleanor W, Sommerville J. Xp54, the Xenopus homologue of human RNA helicase p54, is an integral component of stored mRNP particles in oocytes. Nucleic Acids Res. 1997;25(5):965–973.
- Rauh R, Soell D, Haerteis S, et al. A mutation in the β-subunit of ENaC identified in a patient with cystic fibrosis-like symptoms has a gain-of-function effect. Am J Physiology-Lung Cell Mol Physiol. 2012;304(1):L43–L55.