ABSTRACT
Suppressor of cytokine signaling (SOCS) 2, a negative regulator of growth hormone (GH) and insulin-like growth factor 1 (IGF-1), which is associated with acromegaly and cancers, is a promising candidate molecule for treating various diseases. To facilitate its use in protein therapy, we designed and constructed a human SOCS2 protein containing a membrane-permeable peptide sequence and expressed it in an Escherichia coli system. The partially purified recombinant protein was effectively delivered into several cancer cell lines and inhibited cell growth. Biochemical analysis showed that the recombinant SOCS2 protein interacted with growth hormone receptor (GHR) and downregulated GH-STAT5 signaling target genes. Our results suggest that the designed cell-penetrating SOCS2 protein will be useful in intercellular protein therapy to cure cancers.
Abbreviations: SOCS: suppressor of cytokine signaling; GH: growth hormone; GHR: growth hormone receptor; IGF-1: insulin-like growth factor 1; CP: cell-penetrating; STAT: signal transducer and activator of transcription; JAK: Janus kinase; HNF: hepatocyte nuclear factor; MTM: membrane-translocating motif; HIV: human immunodeficiency virus
GRAPHICAL ABSTRACT
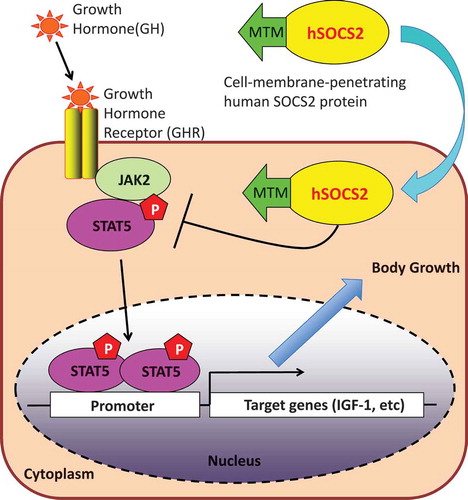
Our produced cell-membrane penetrating human SOCS2 proteins were effectively delivered into several cell lines and inhibited cell growth by suppressing the JAK-STAT5 signal.
Growth hormone (GH), a peptide hormone secreted by somatotropic cells of the anterior pituitary gland, plays important roles in metabolism and body growth [Citation1]. GH promotes the production of insulin-like growth factor (IGF)-1, which is mainly secreted by the liver and exhibits growth-stimulating effects on a wide variety of tissues via the Janus kinase–signal transducer and activator of transcription (JAK–STAT) signaling pathway. The GH/IGF-1 axis has been shown to promote somatic growth and cancer development [Citation2]. Excessive production of GH/IGF-1 by a pituitary adenoma can cause the hormonal disorder acromegaly [Citation3].
Suppressor of cytokine signaling (SOCS) proteins are negative regulators of cytokine and growth factor signaling [Citation4]. Among these proteins, SOCS2 mainly suppresses the GH–JAK2–STAT5 signal transduction pathway, as demonstrated by overgrowth in knockout mice [Citation5,Citation6]. It has been reported that SOCS2 can suppress the carcinogenesis of multiple types of cancers, including liver [Citation7–Citation9], lung [Citation10], breast [Citation11,Citation12] and prostate cancer [Citation13–Citation16]; the level of SOCS2 expression is low in these cancers. Possible explanations for the downregulation of the SOCS2 protein include methylation in its gene promoter region and translation inhibition by non-coding RNA [Citation9,Citation15,Citation16]. Taken together, these results suggest that low SOCS2 protein expression is correlated with tumorigenesis.
Considering its suppressive potential, the SOCS2 protein may be an appropriate candidate molecule for treating acromegaly and cancers. Much effort has been expended to develop methods for its application in protein therapy and iPS cell induction using proteins fused with cell–membrane-penetrating peptides, such as poly-arginine (e.g., 9R or 11R), HIV TAT, and MTM; the two former peptides consist of basic amino acids, whereas the latter contains hydrophobic residues [Citation17–Citation20]. However, there have been no reports on cell membrane-penetrating SOCS2 protein. The objective of the present study was to develop a system to obtain a functional recombinant cell-penetrating SOCS2 protein in an efficient and a time-saving manner. We demonstrate that the recombinant MTM-SOCS2 protein can be readily delivered into various types of cancer cell lines and suppress their proliferation through interaction with growth hormone receptor (GHR), suggesting its usefulness in cancer protein therapy.
Materials and methods
Plasmids and cell cultures
Human SOCS2 cDNA was amplified from a human testis cDNA library by polymerase chain reaction (PCR) using specific primers and cloned into the EcoRI site of pcDNA3-Flag, the SalI-NotI sites of pET28a empty, and pET28a-MTM (N-term and C-term) plasmids [Citation21]. Response elements for the lactogenic hormone weregenerated by synthetic oligonucleotides (sense: 5ʹ-TCGAGTGTGGACTTCTTGGAATTAAGGGACTTTTGA-3ʹ, antisense: 5ʹ-GATCTCAAAAGTCCCTTAATTCCAAGAAGTCCACAC-3ʹ) and cloned into the XhoI- BglII sites of the pGL3-control vector (Promega).
MCF-7, HepG2, A549, and PC-3 cells were cultured in low-glucose Dulbecco’s modified Eagle’s medium (DMEM; FUJIFILM Wako Pure Chemical Co.) and SK-MEL-28 cells were cultured in MEM (Gibco). Both media were supplemented with 10% fetal bovine serum (FBS; Biosera) and 1% penicillin-streptomycin (FUJIFILM Wako Pure Chemical Co.).
Purification and labeling of CP-SOCS2
Escherichia coli strain BL21 (DE3) harboring the recombinant pET28a encoding hSOCS2 were grown in lysogeny broth (LB) medium containing 30 μg/mL kanamycin (Kan) at 37°C overnight. The culture was diluted in 1 L fresh LB medium containing Kan and grown to an OD600 of 0.3 at 37°C. Isopropyl β-D-1-thiogalactopyranoside (IPTG) was then added to the culture at a final concentration of 0.1 mM. The bacteria were grown at 37°C for 3 hours and then collected by 10,000 × g centrifugation at room temperature and resuspended in 20 mL buffer A [20 mM Tris-HCl (pH 7.5), 150 mM NaCl, 0.1% Triton X-100, 0.1 mM phenylmethylsulfonyl fluoride (PMSF)] following sonication to disrupt the cells. After centrifugation (10,000 × g, 4°C, 10 min), the precipitates containing the inclusion bodies of the recombinant hSOCS2 proteins were solubilized in 20 mL of buffer B [20 mM sodium phosphate (pH 7.8), 6 M guanidine hydrochloride, 500 mM NaCl]. Solubilized protein fused with a histidine (His) tag was then bound to a Ni-agarose resin. After washing with buffer B, the His-tagged recombinant hSOCS2 protein was eluted with buffer C [20 mM sodium phosphate (pH 8.0), 8 M urea, 500 mM NaCl, 500 mM imidazole]. Renaturation of the partially purified protein was performed by dialysis against buffer D [50 mM sodium phosphate decahydrate (pH 8.0), 500 mM arginine] at 4°C. The protein was then dialyzed against PBS (four times) and low-glucose DMEM. The recombinant hSOCS2 proteins were labeled with fluorescein isothiocyanate (FITC) using the EZ label FITC protein labeling kit (Pierce).
In-vitro transduction and intracellular detection
MCF-7 cells were cultured onto a glass-bottomed dish (Matsunami) and incubated with 0.5 μM of the FITC-labeled SOCS2 protein at 37°C for 1 hour. After incubation, the cells were treated with proteinase K (1 μg/mL) to remove the membrane-bound unpenetrated protein and then fixed with 4% paraformaldehyde–phosphate-buffered saline (PBS) at 25°C for 15 minutes. Fixed cells were observed using an Olympus FV-100 confocal laser scanning microscope. Flow cytometry (FACSCanto; BD Biosciences) and Western blot analysis were conducted to ensure the presence of the FITC-labeled SOCS2 protein in target cells.
Cell proliferation and cell cycle analysis
Using either low-glucose DMEM or MEM supplemented with 10% FBS and 1% penicillin–streptomycin, GHR-expressing cells, i.e., HepG2 (hepatocarcinoma), MCF-7 (breast cancer), A-549 (lung cancer), PC-3 (prostate cancer), and SK-MEL-28 (skin melanoma) cells, were added to 24-well cell culture plates at a density of 5.0 × 104 cells/well and grown at 37°C overnight. The cells were then washed with and grown in fresh medium overnight. The recombinant hSOCS2 proteins were then added to the cell cultures at a final concentration of 0.5 μM and incubated at 37°C for 1 hour. After incubation, the collected cells were washed with fresh medium and then grown in the same medium, which contained either GH (500 ng/mL final concentration) or IGF-1 (10 ng/mL final concentration) at 37°C for the recommended time periods. On days 0, 1, 2, and 3, the cells were harvested after trypsinization and counted using a light microscope and hemocytometer (Burker-Turk types). For MCF-7, cells were collected after 48 hours and the propidium iodide (PI)-stained cells were subjected to flow cytometric analysis (FACSCanto).
Immunoprecipitation (IP)–western blotting and luciferase assays
IP–Western blotting and luciferase assays were performed using protocols described previously [Citation21,Citation22]. Flag-GHR peptides (588–602a.a. and 588-602a.a.; pTyr595) were synthesized (Thermo Fisher Scientific). The antibodies used were anti-GHR (B10; Santa Cruz Biotechnology), SOCS2 (Cell Signaling Technology), pSTAT5 XP mAb. (D47E7; Cell Signaling Technology), STAT5 (Santa Cruz Biotechnology), Actin-β (Abcam), Myc (9E10; Santa Cruz Biotechnology), Flag antibody resin (M2; Sigma Aldrich), horseradish peroxidase (HRP)-conjugated anti-mouse antibody (DAKO), and HRP-conjugated anti-rabbit IgG (Cell Signaling Technology).
Quantitative reverse-transcription PCR (RT-PCR)
HepG2 cells (4.0 × 105 cells/dish) were seeded on a 35-mm dish and cultured for 48 hours. The cells were then incubated with the recombinant SOCS2 proteins (0.25 μM) for 1 hour. After delivery of the SOCS2 proteins, HepG2 cells were stimulated with GH (500 ng/mL) for 6 hours. The cells were harvested and lysed with ISOGEN (NIPPON GENE). Total RNA was isolated according to the manufacturer’s protocol. The RT reaction was performed using Prime Script RT master mix (Takara Bio). To quantify the mRNA levels of GH signaling target genes, quantitative PCR (qPCR) was performed using the Rotor-Gene SYBR Green PCR kit (QIAGEN). The primer sequences used for qPCR were as follows. IGF-1: TGTGGAGACAGGGGCTTTTA (forward); ATCCACGATGCCTGTCTGA (reverse), IGF-2: GCTGGCAGAGGAGTGTCC (forward); GATTCCCATTGGTGTCTGGA (reverse), HNF-3β (FoxA2): CGTTCCGGGTCTGAACTG (forward); ACCGCTCCCAGCATACTTT (reverse), HNF-4α: AGCAACGGACAGATGTGTGA (forward); TCAGACCCTGAGCCACCT (reverse), HNF-6α CCTGGAGCAAACTCAAATCC (forward); TTCTTTCCTTTTGCATGCTG (reverse), Actin-β: CCAACCGCGAGAAGATGA (forward); CCAGAGGCGTACAGGGATAG (reverse). We conducted qPCR at 40 cycles of 95°C for 10 s, followed by 60°C for 30 s. The results were analyzed using Prism 6 software (GraphPad Software, Inc.).
Results and discussion
Expression and purification of CP-hSOCS2 proteins
To simplify the purification procedure, we fused the rhSOCS2 protein with a 6xHis tag at the N-terminus. To introduce the recombinant protein into various cells effectively, we added an MTM sequence to its N- or C-terminus (). As shown in , the production of three recombinant proteins, His-hSOCS2 (HS2), His-MTM-hSOCS2 (HMS2), and His-hSOCS2-MTM (HS2M), in BL21 (DE3) E. coli was very efficient in the presence of IPTG (, lanes 1 and 2). The recombinant proteins were found in the insoluble fraction (), lanes 3 and 4), indicating that the proteins were expressed as inclusion bodies. After solubilizing the His-tagged recombinant proteins with guanidine hydrochloride, affinity purification of the proteins was performed using a Ni-Sepharose resin. The eluted proteins were then dialyzed against an arginine that contained sodium phosphate buffer to remove urea and imidazole, to facilitate folding and solubilization (, lane 7). The purification efficiency is shown in .
Table 1. Purification of recombinant human SOCS2 proteins from E. coli.
Figure 1. Schematic representation, purification and intercellular delivery of recombinant hSOCS2 proteins.
(a) Structure and design of recombinant human suppressor of cytokine signaling (SOCS) 2 proteins containing MTM (AAVLLPVLLAAP, green) and 6 × histidine tag (orange). (b) Expression and purification of recombinant hSOCS2 proteins were analyzed by sodium dodecyl sulfate polyacrylamide gel electrophoresis (SDS-PAGE) and stained with Coomassie Brilliant Blue (CBB, top). (c) Intracellular localization of recombinant hSOCS2 proteins (top) and differential interference contrast (DIC) image (bottom) were analyzed by confocal laser scanning microscopy. C and D, MCF-7 cells were incubated for 1 h in the absence or presence of fluorescein isothiocyanate (FITC)-labeled SOCS2 proteins at 0.5 μM. (d) FITC-labeled cells were analyzed by flow cytometry. (e) Induction efficiency of MTM-SOCS2 was analyzed by Western blotting under concentration-dependent conditions.
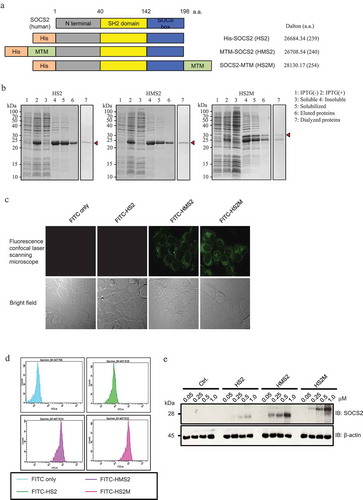
Introduction of rhSOCS2 proteins into MCF-7 cells
To explore whether the soluble rMTM-hSOCS2 proteins could be delivered into cultured cells, we performed immunohistochemical staining of MCF-7 cells using FITC-labeled proteins. As shown in , cells incubated with either the FITC-rHMS2 or FITC-rHS2M protein exhibited a strong green fluorescence signal in the cytoplasmic region, indicating successful introduction of rMTM-hSOCS2 proteins into the cells. We then conducted flow cytometry and Western blot analysis to confirm penetration of the proteins. The results of flow cytometry analysis showed that the cell population exhibited higher FITC fluorescence intensity when either the FITC-rHMS2 or FITC-rHS2M protein was added to the culture ()). Western blot analysis revealed that the amount of rMTM-hSOCS2 proteins detected in the cytoplasmic fraction was dependent on the amount of proteins added to the cells (). These results clearly indicate that the soluble form of the rMTM-hSOCS2 protein can be introduced readily into MCF-7 cells.
CP-SOCS2 proteins inhibit cell proliferation in various cancer cell lines
Using the GHR-expressing cancer cell lines HepG2, MCF-7, A-549, SK-MEL-28, and PC-3, we explored whether the delivered rMTM-SOCS2 proteins could suppress cell proliferation. As shown in , HepG2 cells showed GH- and IGF-1-dependent proliferation (panels A–D). When the rMTM-hSOCS2 proteins were introduced into the cells, significant reduction in cell growth was observed (panel B). In contrast, the recombinant proteins barely inhibited IGF-1-dependent cell proliferation (panel D). The suppressive effect of rMTM-hSOCS2 proteins on GH-dependent cell proliferation was also observed in other cancer cell lines (panels E–H). Cell cycle analysis of MCF-7 cells showed a marked reduction of the transition from the G1 to S phase in the presence of rMTM-hSOCS2 proteins (panel I). In addition to the MTM-tagged SOCS2 proteins, we observed the suppression of cell proliferation by the HS2 protein (SOCS2 protein without MTM sequence) (panels B, E–H). Considering that the SOCS2 protein is rich in the basic and hydrophobic amino acids that can be found in many conventional cell-membrane-penetrating peptides, such as 11R, tat, and MTM, it is possible that a small amount of HS2 protein is taken up by the cells (). These results indicate that the introduced rMTM-hSOCS2 proteins were functional, and that the proteins specifically inhibited the GH signaling pathway.
Figure 2. Suppression of cell proliferation by MTM-SOCS2 in several cancer cell lines.
(a–h) Cell proliferation was measured by counting the number of cells on the indicated number of days after stimulation with either growth hormone (GH) or insulin-like growth factor 1 (IGF-1). (i) The cell-cycle distribution of MCF-7 cells was examined by propidium iodide (PI) staining using a flow cytometer. Percentages of G1, S, and G2/M cells are indicated. Data represent the mean ± standard error; repeated with three independent experiments; *p < 0.05.
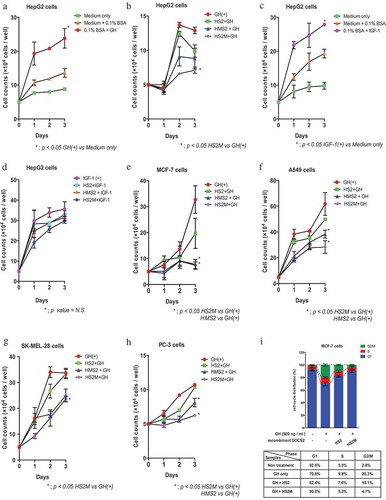
CP-SOCS2 interacted with GHR and suppressed JAK2–STAT5 signaling
SOCS2 mainly suppresses the GH–JAK2–STAT5 signal transduction pathway. To determine whether rMTM-hSOCS2 proteins can suppress this pathway, we first examined the interaction between the 6xMyc-tagged SOCS2 protein expressed stably by HEK293T cells and the Tyr-595-phosphorylated peptide of GHR (588–602) by performing IP using an anti-Flag antibody. Interaction experiments using this phosphorylated peptide of GHR and the SH2 domain of SOCS2 in vitro have been previously reported [Citation23]. As shown in the upper panel of , the 6xMyc-tagged SOCS2 protein was detected on a blotted membrane using an anti-Myc tag antibody, demonstrating the interaction between the 6xMyc-tagged SOCS2 protein and the Tyr-595-phosphorylated peptide of GHR. Using the same approach, we found that the three recombinant proteins, HS2, HMS2, and HS2M, were also able to interact with the Tyr-595-phosphorylated peptide of GHR (). Among the three recombinant proteins, the C-terminus MTM-fused SOCS2 (HS2M) protein seemed to interact weakly with the peptide (). It is possible that the feeble interaction between the Tyr-595-phosphorylated peptide of GHR and the SOCS2 protein is due to a conformational change of the SH2 domain of the SOCS2 protein in vitro when the MTM sequence is present at the C-terminal end. Because the C-terminal MTM-fused SOCS2 (HS2M) protein exhibits stronger inhibition of cell proliferation () and GH target gene expression () than the N-terminal MTM-fused SOCS2 (HMS2) protein, we presume that the permeability of the HS2M protein is higher than that of the HMS2 protein (,)).
Figure 3. Interaction of CP-SOCS2 with growth hormone receptor (GHR).
(a) Interaction of synthesized Flag-tagged Tyr-595-phosphorylated GHR peptides with HEK293T cell-expressed SOCS2 was analyzed by an immunoprecipitation (IP)–Western blotting assay (upper panel). The expression of 6xMyc-tagged SOCS2 in HEK293T cells was analyzed by Western blotting (lower panel). (b) Interaction between the synthesized GHR peptides (un-phosphorylated, left panel; phosphorylated, right panel) and the recombinant SOCS2 proteins was analyzed by an IP–Western blotting assay. (c) Interaction of the recombinant SOCS2 proteins with HEK293T cells expressing GHR-Myc-Flag were analyzed by an IP–Western blotting assay (upper panel). Immunoprecipitated GHR (middle panel) and actin-β expression (lower panel, as a control) were also examined by Western blotting.
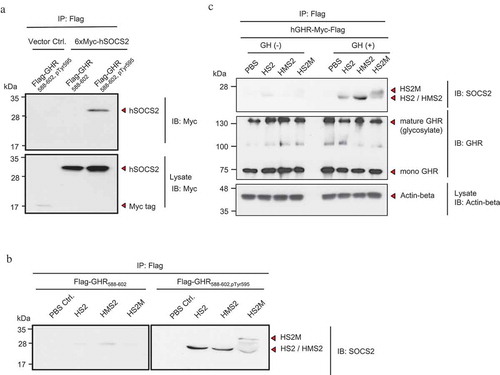
Figure 4. Inhibition of the GH target gene expression by MTM-SOCS2 proteins.
(a) Lysates of recombinant SOCS2-treated and GH-stimulated HepG2 cells were prepared and phosphorylation of STAT5 at Tyr-694(5A)/699(5B) was analyzed by an IP-Western blotting assay. (b) Suppression of Janus kinase–signal transducer and activator of transcription (JAK2–STAT) signaling by MTM-SOCS2 proteins in HepG2 cells was analyzed by a luciferase assay. (c–f) The mRNA levels of indicated GH target genes were analyzed by quantitative reverse-transcription polymerase chain reaction (qRT-PCR). All experiments were performed independently, in triplicate. Data are expressed as means ± standard error, *p < 0.05.
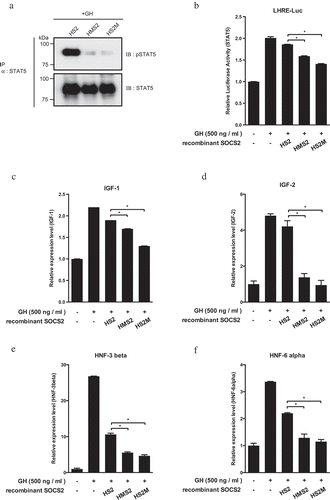
To confirm that the introduced MTM-SOCS2 proteins are able to interact with GHR in the cells, we added functional recombinant proteins to a culture of HEK293T cells that stably expressed the human GHR-Myc-Flag protein. Western blot analysis of the product obtained by IP indicated interaction between the HS2M and HMS2 proteins with the GHR protein in the presence of GH; a low level of the recombinant protein without MTM sequence (HS2) was also detected in the absence and presence of GH ().
Based on these results, we then investigated the inhibitory effect of recombinant SOCS2 proteins on the JAK2–STAT5 signaling pathway using HepG2 cells. The hepatocarcinoma-derived cells gave the highest response to GH among all of the cell lines tested in the present study (Supplemental ). As shown in , the level of phosphorylated STAT5 at Tyr-694(5A)/699(5B) was extremely low when the HMS2 and HS2M proteins were added to the cell culture, indicating that the two recombinant SOCS2 proteins containing the MTM sequence were successfully introduced into the cells and suppressed the phosphorylation of STAT5. Using a reporter containing lactogenic hormone response elements, a luciferase assay was performed and the inhibitory effect of the recombinant proteins on the transcriptional activity of STAT5 was confirmed (). The GH-dependent transcriptional activity of STAT5 was significantly reduced in the presence of the MTM-tagged HMS2 and HS2M proteins.
Using RT-qPCR, we also examined the steady-state mRNA levels of the four GH target genes, IGF-1, IGF-2, HNF-3β, and HNF-6α. As shown in (panels C–F), the mRNA levels of the four genes were extremely low when either the HMS2 or HS2M protein was added to the cell culture, demonstrating the suppression of GH target gene transcription. The HS2 protein, which has no MTM sequence, also exhibited a slight inhibitory effect on the transcription of the four target genes. Our results clearly show that the recombinant SOCS2 proteins were able to suppress the JAK2–STAT5 signaling transduction pathway.
In conclusion, we developed a highly productive E. coli expression system that can express cell-penetrating SOCS2 proteins (MTM-SOCS2) at the mg level; the quantity of proteins is comparable to that of the MTM-fused SOCS3 protein reported by Dr. Hawiger’s research group (17). We also established a method that allows insoluble recombinant SOCS2 proteins to be purified and refolded into functional proteins; the obtained soluble proteins, which can be readily introduced into various cancer cells, can suppress cell proliferation through interference with the GH–JAK2–STAT5 signal transduction pathway. Using our system, a large quantity of functional cell-penetrating SOCS2 proteins can be obtained within a relatively short period of time, suggesting that these recombinant proteins can be usefully applied in protein therapy for treating acromegaly and cancers.
Author contributions
A.M., H.S., and Y.M. designed and conducted the experiments. T.S. and S.H. supervised cell proliferation and cell cycle analyses. Summary of the research was mainly carried out by H.S. and Y.M. Preparation and approval of the manuscript were carried out by A.M., H.S., Y.M., T.S., and S.H.
Suppl._data_20181012final_miyamoto.pdf
Download PDF (288.8 KB)Acknowledgments
We are grateful to Dr. Kyoko Fujiwara (Nihon University) for providing cell lines A-549 and SK-MEL-28.
Disclosure statement
No potential conflict of interest was reported by the authors.
Additional information
Funding
References
- Goldenberg N, Barkan A. Factors regulating growth hormone secretion in humans. Endocrinol Metabolism Clinics North America. 2007;36:37–55.
- Boguszewski CL, Boguszewski MC, Kopchick JJ. Growth hormone, insulin-like growth factor system and carcinogenesis. Endokrynol Pol. 2016;67:414–426.
- Chanson P, Salenava S, Kamenicky P. Acromegaly. Handb Clin Neurol. 2014;124:197–219.
- Ahmed SF, Farquharson C. The effect of GH and IGF1 on linear growth and skeletal development and their modulation by SOCS proteins. J Endocrinol. 2010;206:249–259.
- Greenhalgh CJ, Bartolino P, Asa SL, et al. Growth enhancement in suppressor of cytokine signaling 2 (SOCS-2)-deficient mice is dependent on signal transducer and activator of transcription 5b (STAT5b). Mol Endocrinol. 2002;16:1394–1406.
- Greenhalgh CJ, Rico-Bautista E, Lorentzon M, et al. SOCS2 negatively regulates growth hormone action in vitro and in vivo. J Clin Invest. 2005;115:397–406.
- Chen M, Wei L, Law C-T, et al. RNA N6-methyladenosine methyltransferase-like 3 promotes liver cancer progression through YTHDF2-dependent posttranscriptional silencing of SOCS2. Hepatology. 2018;67:2254–2270.
- Cui M, Sun J, Hou J, et al. The suppressor of cytokine signaling 2 (SOCS2) inhibits tumor metastasis in hepatocellular carcinoma. Tumour Biol. 2016;37:13521–13531.
- Jiang C, Long J, Liu B, et al. miR-500a-3p promotes cancer stem cells properties via STAT3 pathway in human hepatocellular carcinoma. J Exp Clin Cancer Res. 2017;36:99.
- Zhou Y, Zhang Z, Wang N, et al. Suppressor of cytokine signalling-2 limits IGF1R-mediated regulation of epithelial-mesenchymal transition in lung adenocarcinoma. Cell Death Dis. 2018;9:429.
- Haffner MC, Petridou B, Peyrat JP, et al. Favorable prognostic value of SOCS2 and IGF-I in breast cancer. BMC Cancer. 2007;7:136.
- Farabegoli F, Ceccarelli C, Santini D, et al. Suppressor of cytokine signalling 2 (SOCS-2) expression in breast carcinoma. J Clin Pathol. 2005;58:1046–1050.
- Iglesias-Gato D, Chuan YC, Wikström P, et al. SOCS2 mediates the cross talk between androgen and growth hormone signaling in prostate cancer. Carcinogenesis. 2014;35:24–33.
- Hendriksen PJ, Dits NF, Kokame K, et al. Evolution of the androgen receptor pathway during progression of prostate cancer. Cancer Res. 2006;66:5012–5020.
- Misawa A, Takayama K, Urano T, et al. Androgen-induced long noncoding RNA (lncRNA) SOCS2-AS1 promotes cell growth and inhibits apoptosis in prostate cancer cells. J Biol Chem. 2016;291:17861–17880.
- Das R, Gregory PA, Fernandes RC, et al. MicroRNA-194 promotes prostate cancer metastasis by inhibiting SOCS2. Cancer Res. 2017;77:1021–1034.
- Jo D, Liu D, Yao S, et al. Hawiger J Intercellular protein therapy with SOCS3 inhibits inflammation and apoptosis. Nat Med. 2005;11:892–898.
- Ohno Y, Suzuki-Takedachi K, Yasunaga S, et al. Manipulation of cell cycle and chromatin configuration by means of cell-penetrating geminin. PLoS One. 2016;11:e0155558.
- Choi JM, Shin JH, Sohn MH, et al. Cell-permeable Foxp3 protein alleviates autoimmune disease associated with inflammatory bowel disease and allergic airway inflammation. Proc Natl Acad Sci USA. 2010;107:18575–18580.
- Zhou H, Wu S, Joo JY, et al. Generation of induced pluripotent stem cells using recombinant proteins. Cell Stem Cell. 2009;4:381–384.
- Oho M, Nakano R, Nakayama R, et al. TIPE2 (tumor necrosis factor α-induced protein 8-like 2) is a novel negative regulator of TAK1 signal. J Biol Chem. 2016;291:22650–22660.
- Masuhiro Y, Kayama K, Fukushima A, et al. SOCS-3 inhibits E2F/DP-1 transcriptional activity and cell cycle progression via interaction with DP-1. J Biol Chem. 2008;283:31575–31583.
- Greenhalgh CJ, Metcalf D, Thaus AL, et al. Biological evidence that SOCS-2 can ACT either as an enhancer or suppressor of growth hormone signaling. J Biol Chem. 2002;277:40181–40184.