ABSTRACT
Seed germination is regulated by light. Phytochromes (Phys) act as red and far-red light photoreceptors to mediate seed germination. However, the mechanism of this process is not well understood. In this study, we found that the Arabidopsis thaliana mutants vascular plant one-zinc finger 1 (voz1) and voz2 showed higher seed germination percentage than wild type when PhyB was inactivated by far-red light. In wild type, VOZ1 and VOZ2 expression were downregulated after seed imbibition, repressed by PhyB, and upregulated by Phytochrome-interacting factor 1 (PIF1), a key negative regulator of seed germination. Red light irradiation and the voz1voz2 mutation caused increased expression of Gibberellin 3-oxidase 1 (GA3ox1), a gibberellin (GA) biosynthetic gene. We also found that VOZ2 is bound directly to the promoter of GA3ox1 in vitro and in vivo. Our findings suggest that VOZs play a negative role in PhyB-mediated seed germination, possibly by directly regulating GA3ox1 expression.
Graphical abstract
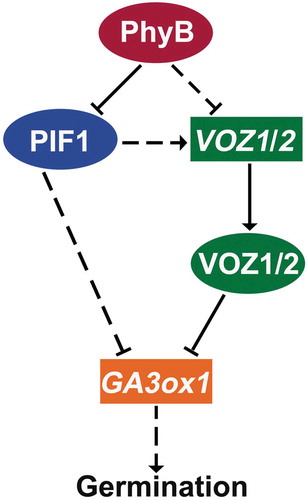
VOZ1 and VOZ2 transcription are repressed by PhyB and upregulated by PIF1, and directly repress GA3ox1 expression in seeds, thereby negatively regulating seed germination.
Seed germination is a critical developmental stage in the plant life cycle; its adaptation allows plants to maintain reproduction through environmental changes [Citation1–Citation3]. Seed germination requires suitable temperature, soil moisture, and light conditions. For photogenic seeds, light is the main factor determining germination or germination percentage. Arabidopsis thaliana seeds are typical light-requiring seeds [Citation4,Citation5]. Light quality, intensity, duration, and direction can be perceived by plant photoreceptors, thus affecting plant development from seed germination to flowering [Citation6–Citation8].
Plants possess at least five different types of photoreceptors: red (R)/far-red (FR) light photoreceptor phytochromes (Phys) [Citation9,Citation10], ultraviolet (UV)-A/blue light photoreceptor cryptochromes (CRYs) [Citation11], blue light receptor phototropins (Phots) [Citation12,Citation13], blue light photoreceptors zeitlupe (ZTL)/lov kelch protein 2 (LKP2)/flavin binding, kelch repeat, F-box protein 1 (FKF1) [Citation14–Citation17], and the UV-B receptor UVR8 [Citation18]. Among these photoreceptor types, Phys are essential for the light promotion of germination [Citation4,Citation19–Citation22]. Phys have two forms, FR light-absorbing (Pfr, λmax = 730 nm) and R light-absorbing forms (Pr, λmax = 660 nm). Pfr is the active light-absorbing form; inactive Pr can be converted to active Pfr upon R light irradiation, which can be reversed by FR light irradiation in a photo-reversible regulation process typical of the Phy light response [Citation23–Citation25]. A. thaliana contains five Phys, i.e., PhyA-E [Citation26–Citation29]; germination is promoted principally by PhyB and PhyA [Citation30]. PhyA mediates a very low fluence response (VLFR) and a high-irradiance response (HIR) to regulate seed germination, whereas PhyB mediates a low fluence response (LFR) to regulate seed germination and triggers R/FR-light-reversible seed germination [Citation30–Citation34].
The light signal perceived by Phys is converted to an internal signal, which in turn regulates seed physiological processes. Gibberellin (GA) and abscisic acid (ABA) are internal signals that play key roles in the regulation of seed germination [Citation34]. In seeds, the action of GA is antagonized by that of ABA; numerous studies have shown that cross-regulation between GA and ABA levels plays a key role in the control of seed germination [Citation35–Citation38]. The seed GA content is increased, and the endogenous ABA level decreased, by imbibition and stratification treatments prior to the onset of the germination [Citation39]. Several studies have revealed interactions between the light, GA, and ABA signaling pathways at the molecular level [Citation34,Citation40,Citation41]. Phytochrome-interacting factor 1 (PIF1, also known as Phytochrome-interacting factor 3-like 5 [PIL5]) is a key negative regulator of Phy-mediated seed germination [Citation42]. Phys promote seed germination by degrading PIF1 protein, which directly regulates the transcription of two DELLA genes and indirectly regulates that of GA and ABA metabolic genes [Citation43,Citation44]. PIF1 indirectly regulates GA levels through the activation of DOF affecting germination 1 (DAG1), which subsequently suppresses transcription of the GA biosynthesis gene Gibberellin 3-oxidase 1 (GA3ox1) [Citation41,Citation45]. PIF1 can also activate the transcription of Somnus (SOM), which in turn represses expression of the GA biosynthetic genes GA3ox1 and GA3ox2 and activates the catabolic gene Gibberellin 2-oxidase 2 (GA2ox2) [Citation41,Citation46]. A recent report indicated that REVEILLE1 (RVE1) and RVE2 transcription factors repress R/FR light-reversible germination downstream of PhyB in Arabidopsis [Citation47,Citation48]. RVE1 directly inhibits GA3ox2 transcription, thereby suppressing GA biosynthesis [Citation47]. RVE1 is also involved in the GA signaling pathway and inhibits germination in an RGA-like2 (RGL2)-dependent manner [Citation48].
Vascular plant One-Zinc finger protein (VOZ) is a transcription factor unique to higher plants. Arabidopsis contains two members of the VOZ transcription factor family, VOZ1, and VOZ2 [Citation49]. VOZs regulate flowering time [Citation50–Citation53] and respond to biotic and abiotic stresses. VOZs have been suggested to function as negative regulators of the response to abiotic stresses such as freezing and drought stress, and as positive regulators of the response to biotic stresses such as fungal and pathogen infection [Citation54,Citation55]. In Arabidopsis, VOZ2 also functions under heat stress conditions to suppress dehydration-responsive element binding protein 2A (DREB2A), a transcription factor involved in activating gene expression in response to drought or heat stress (HS) [Citation56]. VOZ1 has been reported to function as a negative regulator of HS-inducible DREB2C signaling by blocking access to the AP2 DNA-binding domain of DREB2C [Citation57]. A recent study showed that VOZs also act as positive regulators of several salt-responsive genes and function redundantly in salt resistance [Citation58].
In the present study, we explored the role of VOZs in the repression of PhyB-mediated R/FR photo-reversible germination. The voz1voz2 mutant showed higher seed germination percentage than that of wild type when PhyB was inactivated by FR light (PhyB-off condition). The expression of VOZ1 and VOZ2 was found to be downregulated after imbibition, repressed by PhyB, and upregulated by PIF1. In addition, we showed that R light irradiation and the voz1voz2 mutation caused increased expression of GA3ox1. Moreover, VOZ2 is bound directly to the GA3ox1 promoter, as shown by chromatin immunoprecipitation (ChIP) and electrophoresis mobility shift assay (EMSA). Therefore, we suggest that VOZs play a negative role in PhyB-mediated seed germination, possibly by directly regulating GA3ox1 expression, which provides a new clue for further elucidating the mechanism linking light- and GA-mediated seed germination in Arabidopsis.
Materials and methods
Plant materials and growth conditions
The Arabidopsis used for all experiments described was the Columbia (Col) ecotype. The voz1-1 (CS858345) and voz2-1 (SALK_021718) mutants used in this study as described previously [Citation50] were obtained from Arabidopsis Biological Resource Center (ABRC, www.abrc.osu.edu). The voz1voz2 mutant was voz1-1voz2-1 as described [Citation50]. The phyB mutant used in this study was phyB-9 as described [Citation59]. The mutant pif1 (SALK_072677) described previously [Citation42], was obtained from ABRC. Seeds were sown on half-strength Murashige and Skoog (1/2 MS) agar medium, chilled for 3 d at 4°C under dark, and then transferred to growth chambers under continuous white light for 5 d. Seedlings were then transplanted into the soil and grown in a growth room with a 16 h light/8 h dark cycle at 22–24°C. Seeds were harvested at the same time in each batch.
For generation of VOZ2-Flag transgenic plants, the full-length VOZ2 cDNA was amplified with specific primers (Table S1), cloned into a pCAMBIA1300 vector for expression of a fusion protein bearing Flag tag, and transformed into wild-type Col by using the floral dip method [Citation60]. The T0 seeds were selected on 1/2 MS medium containing 50 µg mL−1 hygromycin, and the hygromycin-resistant plant was transferred to soil 10 d after germination, which was subjected to further genetic and protein analysis. Homozygous lines were selected for the ChIP assay.
Germination assay
Germination assays were performed as described previously [Citation47]. For the PhyB-dependent germination assay, triplicate sets of 50 seeds were surface-sterilized and plated on 0.8% agar (pH 5.8) within one and a half hours under white light, and incubated in the dark, or irradiated with 5 min of FR light (3.2 μmol m−2 s−1) to inactivate PhyB (PhyB-off), or followed by 5 min of R light (20 μmol m−2 s−1) to activate PhyB (PhyB-on), unless specified otherwise. After 4 days’ incubation in darkness, germination was assayed. Seeds with a protruded radical were considered as germinated seeds. All experiments were carried out at least three times with similar results.
RNA isolation and qRT-PCR analysis
Total RNA was extracted from seeds using the Spectrum Plant Total RNA kit (Sigma-Aldrich) according to the manufacturer’s protocol, and reverse transcribed using PrimeScript RT reagent Kit with gDNA Eraser (TaKaRa). Real-time fluorescence quantitative PCR (qRT-PCR) analysis was performed as described [Citation61]. The experiment was repeated three times from three independent biological experiments. Expression of ACTIN2 (ACT2) was used for normalization. The relative expression level was analyzed by the CFX96 software. Primer sets used are listed in Table S1, and the primer efficiency is shown in Figure S1.
ChIP assay
ChIP assays were performed as previously described [Citation62,Citation63]. The VOZ2-Flag and wild-type seeds were irradiated with FR light for 5 min followed by R light for 5 min, and then incubated in the dark for 12 h and sampled for ChIP assays. The VOZ2-bound DNAs were immunoprecipitated by Anti-Flag Affinity Gel (bimake) and analyzed by quantitative PCR. The ChIP-qPCR results were calculated as relative binding unit (IP/Input).
EMSA assay
Full-length coding regions of VOZ2 were cloned into the pGEX-4T-3 vector to generate GST-VOZ2 constructs. The primers used for this clone were listed in Table S1. The plasmids were then introduced into Escherichia coli strain BL21 (DE3) to induce protein expression. The E.coli-expressed GST-VOZ2 fusion protein and GST protein were purified by Pierce Glutathione Agarose (Thermo), respectively. GST-VOZ2 protein and GST protein were used for EMSA. The EMSA was carried out as described [Citation62,Citation64], with some modified steps. The primer sequences were synthesized and labeled with FAM fluorescence probe (TsingKe Biological Technology) (Table S1). The GST-VOZ2 protein and FAM-labeled probe or mutant FAM-labeled probe were incubated with or without the amount of unlabeled competitor in binding buffer [20 mM Tris (pH 8.0), 0.5 mM EDTA, 0.05% TritonX-100, 5% glycerol, 1 mM DTT]. The reactions were incubated at room temperature for 30 min and fractioned by electrophoresis in a 4% native polyacrylamide gel (acrylamide:bisacrylamide, 29:1) containing 3% glycerol, 45 mM Tris (pH 8.0), 45 mM boric acid, and 1 mM Na2EDTA. The FAM signal was detected after electrophoresis using KADAK 4000 MM Image Station.
Statistical analysis
The statistical significant difference between samples was analyzed by Student’s t-test using SPSS software.
Results
VOZs negatively regulate PhyB-mediated seed germination
We examined the function of VOZs in light-induced seed germination. The photoreceptor PhyB regulates R/FR reversible seed germination [Citation31,Citation65,Citation66]. We therefore analyzed the germination phenotypes of voz1-1, voz2-1, and voz1voz2 mutants using a PhyB-dependent germination protocol. Seeds without cold stratification were exposed to different light conditions as described previously ()) [Citation47]. The Pfr PhyB present in seeds was inactivated by FR light (PhyB-off condition) or activated by R light (PhyB-on condition), and the seeds were then allowed to germinate in the dark for 4 d before seed germination scoring. Under PhyB-off condition, the voz1voz2 mutant had a higher germination percentage (20.83%) than wild type (6.67%) () and Figure S2), whereas the single voz1-1 and voz2-1 mutants showed no significant differences in phenotype compared to wild type (Figure S3). Under dark or PhyB-on conditions, the germination percentage of voz1voz2 was close to 100%, similar to wild type. A time-course germination assay confirmed that the voz1voz2 mutant seeds germinated faster than wild type under PhyB-off condition, with no significant difference observed under PhyB-on condition ()). As a control, we used pif1 mutant seeds, which germinate well irrespective of their light conditions [Citation43]. These results suggest that VOZs function redundantly and play a negative role in PhyB-mediated seed germination.
VOZ1 and VOZ2 expression are repressed by PhyB
We next examined the expression pattern of VOZ1 and VOZ2 during seed germination. qRT-PCR analysis showed that VOZ1 and VOZ2 expression decreased rapidly during seed imbibition, and disappeared after 48 h of imbibition ()). This result is consistent with data obtained from a public data repository (http://bar.utoronto.ca/efp/cgi-bin/efpWeb.cgi) (Figure S4) [Citation67]. A R light pulse (PhyB-on) greatly reduced the expression of VOZ1 and VOZ2 in wild-type seeds ()). Consistent with this finding, the transcript levels of VOZ1 and VOZ2 were increased in the phyB mutant ()). DOG1, which regulates primary seed dormancy and acts downstream of PhyB [Citation47,Citation68–Citation70], was used as a control. These results suggest that VOZ1 and VOZ2 transcript expression is repressed after imbibition, at least in part by the active form of PhyB.
VOZ1 and VOZ2 expression are positively regulated by PIF1
Because PIF1 is a key repressor of seed germination and is negatively regulated by PhyB [Citation42,Citation43], we wondered whether VOZs are regulated by PIF1. To verify this hypothesis, we analyzed VOZ1 and VOZ2 expression in wild-type and pif1 mutant seeds grown under PhyB-dependent germination conditions. Interestingly, the level of VOZ1 and VOZ2 expression in pif1 mutant seeds was downregulated compared with the wild type under PhyB-off condition (). Similar results were reported for RVE1 and RVE2, which are activated by PIF1 at the transcriptional level [Citation47]. These data suggest that VOZ1 and VOZ2 are likely to be positively regulated by PIF1.
We next tested whether VOZ1 and VOZ2 regulate PIF1 expression. PIF1 expression in wild-type and voz1voz2 mutant seeds grown under PhyB-dependent germination conditions was analyzed using qRT-PCR. As shown in ), the expression level of PIF1 in voz1voz2 mutant seeds was similar to that of wild type both under PhyB-on and PhyB-off conditions. Together, these findings suggest that VOZ1 and VOZ2 are likely to function downstream of PIF1 in PhyB-mediated seed germination.
The mutation of VOZ1 and VOZ2 result in upregulated GA3ox1 expression
It has been reported that GA3ox1, GA3ox2, and GA2ox2 play prominent roles in PhyB-mediated seed germination [Citation43,Citation71]. To investigate the molecular mechanism of VOZs in regulating PhyB-mediated seed germination, we first detected GA3ox1, GA3ox2, and GA2ox2 expression in voz1voz2 mutant seeds grown under PhyB-dependent germination conditions. R light irradiation and the voz1voz2 mutation caused increased expression of GA3ox1 (,). However, the transcript levels of GA3ox2 and GA2ox2 in voz1voz2 mutant seeds were comparable to that in wild type ()). These results suggest that VOZs negatively regulate GA3ox1, but not GA3ox2 or GA2ox2, in seeds.
The mutation of VOZ1 and VOZ2 does not affect the expression of DELLA genes or ABA metabolic genes
Phy-mediated light signaling promotes seed germination by increasing the level of bioactive GA and by inhibiting expression of the DELLA genes GA-insensitive (GAI) and Repressor of GA1-3 (RGA), which are key negative regulators of GA signaling [Citation44,Citation72]. We therefore tested whether VOZs regulate transcript expression of these DELLA genes using the PhyB-dependent germination protocol. GAI and RGA transcript levels in wild-type seeds are decreased by R light [Citation44,Citation46]. Similarly, the R light irradiation greatly reduced the levels of the GAI and RGA transcript in both wild-type and voz1voz2 mutant seeds ()). However, voz1voz2 mutation had no significant effect on RGA or GAI expression ()). These results indicate that VOZs do not regulate transcript expression of the DELLA genes RGA and GAI in seeds.
ABA metabolic genes are regulated by Phys during photo-reversible seed germination [Citation35]. Exposure to R light decreases expression of the ABA anabolic genes ABA-deficient1 (ABA1), Nine-cis-epoxycarotenoid dioxygenase6 (NCED6), and NCED9 and increases expression of the ABA catabolic gene Cytochrome P450, Family 707, Subfamily A, Polypeptide 2 (CYP707A2) in wild-type seeds [Citation35,Citation44]. Similarly, the expression of ABA1, NCED6, and NCED9 was decreased while that of CYP707A2 was increased by a R light irradiation (PhyB-on condition) in both the voz1voz2 mutant and wild type. However, there was no significant difference in the expression of these genes between the voz1voz2 mutant and wild type under our experimental conditions ()). These results indicate that VOZs do not regulate the ABA metabolic genes ABA1, NCED6, NCED9, and CYP707A2 in seeds.
VOZ2 protein binds to the GA3ox1 promoter
VOZ1 and VOZ2 are transcription factors that regulate target genes either directly or indirectly. VOZ2 has been reported to bind as a dimer to the specific palindromic sequence GCGTNx7ACGC, with Domain-B, which functions in both DNA-binding and dimerization domains [Citation49]. The GA3ox1 promoter region contains two potential VOZ2 binding sites within a 3-kb region upstream of the transcription initiation site: one forward sequence, GA3ox1-A, is located between −2800 and −2786 bp, and the other reverse sequence, GA3ox1-B, is located between −1298 and −1284 bp ()). To determine whether VOZ2 binds to these sites, we performed a ChIP assay using VOZ2-Flag transgenic seeds that expressed Flag-tagged VOZ2 protein (Figure S5). Unlike the wild-type samples, the a, b, d, and e fragments of the promoter region of GA3ox1 were substantially enriched in the VOZ2-Flag samples ()), suggesting that these two sites are likely to be VOZ2 binding sites.
To further confirm whether VOZ2 protein can recognize the core sequence of these two sites in the promoter region of GA3ox1, we purified VOZ2 recombinant protein from Escherichia coli and performed an EMSA. VOZ2 recombinant protein was incubated with a FAM-labeled probe (30mer) containing the GCGTTAATCTTAAGG core sequence of site A, or the GTGTAGTTTTAACGC core sequence of site B ()). As shown in ), incubation with the GST-VOZ2 protein caused probe mobility shift bands for sites A and B; however, incubation with GST protein did not, indicating that VOZ2 can bind to these two sequences. As the concentration of the unlabeled probe increased, the binding capacity gradually decreased. The addition of a mutant-labeled probe (5ʹ-GCGTTAATCTTAAGG-3ʹ changed to 5ʹ-aaaaaAAaaaaAAaa-3ʹ in A, and 5ʹ-GTGTAGTTTTAACGC-3ʹ changed to 5ʹ-aaaaAaaaaaAAaaa-3ʹ in B) ()) did not cause mobility shift bands ()), further confirming that VOZ2 binds directly to these two sequences in the A and B sites of GA3ox1. Together, these results suggest that VOZ2 can bind to the GA3ox1 promoter in vivo and in vitro.
Discussion
VOZs play a negative role in PhyB-mediated seed germination
Previous studies have demonstrated that Arabidopsis VOZ1 and VOZ2 are involved in regulating PhyB-dependent flowering [Citation50–Citation53] and biotic and abiotic stress responses [Citation54–Citation58]. In this study, we found that VOZ1 and VOZ2 regulate seed germination by analyzing voz1voz2 mutants. Under dark and PhyB-on conditions, the germination frequency of voz1voz2 was similar to that of wild-type plants, whereas that of voz1voz2 was higher than that of wild-type plants under PhyB-off condition (). Similar but strong phenotypes have been reported for pif1 mutant seeds and rve1-null mutant seeds, which negatively regulate Phy-mediated seed germination [Citation42,Citation43,Citation47]. Additionally, similar to RVE1 [Citation47], VOZ1 and VOZ2 expression was repressed by PhyB (). We also found that VOZ1 and VOZ2 expression were subjected to regulation by PIF1, a key negative regulator of Phy-mediated seed germination [Citation40,Citation42], whereas VOZs did not regulate PIF1 expression under our experimental conditions (). We therefore hypothesized that VOZs are likely to function downstream of PIF1 and play a negative role in PhyB-mediated seed germination (Figure S6), which needs to be further confirmed by additional genetic data.
Possible mechanism for VOZs in regulating PhyB-mediated seed germination
Recent studies have demonstrated that Phys modulate endogenous levels of GA and ABA, as well as GA responsiveness to trigger R/FR light-reversible seed germination [Citation34]. Several transcription factors have been identified that link light perception and the modulation of hormone levels and responsiveness. PIF1 negatively regulates Phy-mediated seed germination by regulating the transcription of ABA and GA metabolic genes either directly or indirectly [Citation40,Citation73]. RVE1 binds directly to the GA3ox2 promoter to inhibit its transcription, thus suppressing the biosynthesis of bioactive GA and repressing R/FR light-reversible germination downstream of PhyB [Citation47]. RVE1 has also been reported to interact with both RGL2 and its E3 ubiquitin ligase SLEEPY1 (SLY1), and to modulate seed germination by inhibiting RGL2 degradation through preventing the interaction of RGL2 with SLY1 [Citation48]. Our results show that VOZ1 and VOZ2 repressed the expression of GA3ox1, but not that of the seed DELLA genes and ABA metabolic genes examined in this study (). VOZ2 was reported to function as a transcriptional repressor of DREB2A under heat stress and non-heat stress conditions [Citation56]. VOZs also act as positive regulators of several salt-responsive genes and function redundantly in salt resistance [Citation58]. Additionally, VOZs directly repress the expression of modifier of snc1,3 (MOS3)/suppressor of auxin resistance 3 (SAR3), which is required for proper flowering timing, by binding to its promoter [Citation51]. We therefore speculated that VOZs might regulate GA3ox1 expression by binding directly to its promoter. Our ChIP and EMSA results showed that VOZ2 is capable of binding to core sequences in the promoter region of GA3ox1 (). Together, our findings suggest that VOZs might directly repress GA3ox1 expression, thereby negatively regulating PhyB-mediated seed germination (Figure S6). However, additional genetic and molecular experiments are required to verify this possibility.
In conclusion, the results of the present study demonstrate that VOZ1 and VOZ2 function in PhyB-mediated seed germination, possibly by directly regulating GA3ox1 expression, and suggest a new clue for further elucidating the mechanism linking light- and GA-mediated seed germination in Arabidopsis.
Author contribution
DL, LQ, XL and XZ designed the research; DL, LQ, MZ and XL performed the experiments; HW and JM provided technical assistance to DL and LQ; DL, LQ, XL and XZ analyzed the data; DL, XL and XZ supervised and completed the writing.
VOZ-supplementary_data.pdf
Download PDF (514.4 KB)Acknowledgments
We thank Dr. Takayuki Kohchi for providing voz1-1voz2-1 mutant seeds.
Disclosure statement
No potential conflict of interest was reported by the authors.
Supplementary material
Supplemental data for this article can be accessed here.
Additional information
Funding
References
- Bentsink L, Koornneef M. Seed dormancy and germination. Arab B. 2008;6:e0119.
- Rajjou L, Duval M, Gallardo K, et al. Seed germination and vigor. Annu Rev Plant Biol. 2012;63(1):507–533.
- Penfield S. Seed dormancy and germination. Curr Biol. 2017;27(17):R874–R878.
- Shinomura T. Phytochrome regulation of seed germination. J Plant Res. 1997;110(1):151–161.
- Yamauchi Y, Ogawa M, Kuwahara A, et al. Activation of gibberellin biosynthesis and response pathways by low temperature during imbibition of Arabidopsis thaliana seeds. Plant Cell. 2004;16(2):367–378.
- Ryu JS, Il KJ, Kunkel T, et al. Phytochrome-specific type 5 phosphatase controls light signal flux by enhancing phytochrome stability and affinity for a signal transducer. Cell. 2005;120(3):395–406.
- Neff MM, Fankhauser C, Chory J. Light: an indicator of time and place. Genes Dev. 2000;14(3):257–271.
- Quail PH. Phytochrome photosensory signalling networks. Nat Rev Mol Cell Biol. 2002;3(2):85–93.
- Quail PH. Photosensory perception and signalling in plant cells: new paradigms? Curr Opin Cell Biol. 2002;14(2):180–188.
- Chen M, Chory J. Phytochrome signaling mechanisms and the control of plant development. Trends Cell Biol. 2011;21(11):664–671.
- Chaves I, Pokorny R, Byrdin M, et al. The cryptochromes: blue light photoreceptors in plants and animals. Annu Rev Plant Biol. 2011;62(1):335–364.
- Briggs WR, Christie JM. Phototropins 1 and 2: versatile plant blue-light receptors. Trends Plant Sci. 2002;7(5):204–210.
- Christie JM. Phototropin blue-light receptors. Annu Rev Plant Biol. 2007;58(1):21–45.
- Nelson DC, Lasswell J, Rogg LE, et al. FKF1, a clock-controlled gene that regulates the transition to flowering in Arabidopsis. Cell. 2000;101(3):331–340.
- Somers DE, Schultz TF, Milnamow M, et al. ZEITLUPE encodes a novel clock-associated PAS protein from Arabidopsis. Cell. 2000;101(3):319–329.
- Schultz TF, Kiyosue T, Yanovsky M, et al. A role for LKP2 in the circadian clock of Arabidopsis. Plant Cell. 2001;13(12):2659–2670.
- Suetsugu N, Wada M. Evolution of three LOV blue light receptor families in green plants and photosynthetic stramenopiles: phototropin, ZTL/FKF1/LKP2 and aureochrome. Plant Cell Physiol. 2013;54(1):8–23.
- Rizzini L, Favory -J-J, Cloix C, et al. Perception of UV-B by the Arabidopsis UVR8 protein. Science. 2011;332(6025):103–106.
- Donohue K, Heschel MS, Butler CM, et al. Diversification of phytochrome contributions to germination as a function of seed-maturation environment. New Phytol. 2008;177(2):367–379.
- Poppe C, Schafer E. Seed germination of Arabidopsis thaliana phyA/phyB double mutants is under phytochrome control. Plant Physiol. 1997;114(4):1487–1492.
- Bae G, Choi G. Decoding of light signals by plant phytochromes and their interacting proteins. Annu Rev Plant Biol. 2008;59(1):281–311.
- Hennig L, Stoddart WM, Dieterle M, et al. Phytochrome E controls light-induced germination of Arabidopsis. Plant Physiol. 2002;128(1):194–200.
- Sineshchekov VA. Photobiophysics and photobiochemistry of the heterogeneous phytochrome system. Biochim Biophys Acta - Bioenergy. 1995;1228(2–3):125–164.
- Rockwell NC, Su Y-S, Lagarias JC. Phytochrome structure and signaling mechanisms. Annu Rev Plant Biol. 2006;57(1):837–858.
- Kircher S. Nucleocytoplasmic partitioning of the plant photoreceptors phytochrome A, B, C, D, and E is regulated differentially by light and exhibits a diurnal rhythm. Plant Cell Online. 2002;14(7):1541–1555.
- Mathews S, Sharrock RA. Phytochrome gene diversity. Plant Cell Environ. 1997;20(6):666–671.
- Sharrock RA, Quail PH. Novel phytochrome sequences in Arabidopsis thaliana: structure, evolution, and differential expression of a plant regulatory photoreceptor family. Genes Dev. 1989;3(11):1745–1757.
- Clack T, Mathews S, Sharrock RA. The phytochrome apoprotein family in Arabidopsis is encoded by five genes: the sequences and expression of PHYD and PHYE. Plant Mol Biol. 1994;25(3):413–427.
- Franklin KA, Quail PH. Phytochrome functions in Arabidopsis development. J Exp Bot. 2010;61(1):11–24.
- Ibarra SE, Auge G, Sánchez RA, et al. Transcriptional programs related to phytochrome A function in Arabidopsis seed germination. Mol Plant. 2013;6(4):1261–1273.
- Botto JF, Sánchez RA, Casal JJ. Role of phytochrome B in the induction of seed germination by light in Arabidopsis thaliana. J Plant Physiol. 1995;146(3):307–312.
- Botto JF, Sanchez RA, Whitelam GC, et al. Phytochrome A mediates the promotion of seed germination by very low fluences of light and canopy shade light in Arabidopsis. Plant Physiol. 1996;110(2):439–444.
- Shinomura T, Nagatani A, Hanzawa H, et al. Action spectra for phytochrome A- and B-specific photoinduction of seed germination in Arabidopsis thaliana. Proc Natl Acad Sci. 1996;93(15):8129–8133.
- Seo M, Nambara E, Choi G, et al. Interaction of light and hormone signals in germinating seeds. Plant Mol Biol. 2009;69(4):463–472.
- Seo M, Hanada A, Kuwahara A, et al. Regulation of hormone metabolism in Arabidopsis seeds: phytochrome regulation of abscisic acid metabolism and abscisic acid regulation of gibberellin metabolism. Plant J. 2006;48:354–366.
- Piskurewicz U, Jikumaru Y, Kinoshita N, et al. The gibberellic acid signaling repressor RGL2 inhibits Arabidopsis seed germination by stimulating abscisic acid synthesis and ABI5 activity. Plant Cell Online. 2008;20(10):2729–2745.
- Kendall SL, Hellwege A, Marriot P, et al. Induction of dormancy in Arabidopsis summer annuals requires parallel regulation of DOG1 and hormone metabolism by low temperature and CBF transcription factors. Plant Cell. 2011;23:2568–2580.
- Piskurewicz U, Turečková V, Lacombe E, et al. Far-red light inhibits germination through DELLA-dependent stimulation of ABA synthesis and ABI3 activity. Embo J. 2009;28(15):2259–2271.
- Shu K, Liu XD, Xie Q, et al. Two faces of one seed: hormonal regulation of dormancy and germination. Mol Plant. 2016;9(1):34–45.
- Lau OS, Deng XW. Plant hormone signaling lightens up: integrators of light and hormones. Curr Opin Plant Biol. 2010;13(5):571–577.
- de Wit M, Galvão VC, Fankhauser C. Light-mediated hormonal regulation of plant growth and development. Annu Rev Plant Biol. 2016;67:513–537.
- Oh E. PIL5, a phytochrome-interacting basic Helix-Loop-Helix protein, is a key negative regulator of seed germination in Arabidopsis thaliana. Plant Cell Online. 2004;16:3045–3058.
- Oh E, Yamaguchi S, Kamiya Y, et al. Light activates the degradation of PIL5 protein to promote seed germination through gibberellin in Arabidopsis. Plant J. 2006;47:124–139.
- Oh E, Yamaguchi S, Hu J, et al. PIL5, a phytochrome-interacting bHLH protein, regulates gibberellin responsiveness by binding directly to the GAI and RGA promoters in Arabidopsis seeds. Plant Cell Online. 2007;19:1192–1208.
- Gabriele S, Rizza A, Martone J, et al. The Dof protein DAG1 mediates PIL5 activity on seed germination by negatively regulating GA biosynthetic gene AtGA3ox1. Plant J. 2010;61:312–323.
- Kim DH, Yamaguchi S, Lim S, et al. SOMNUS, a CCCH-Type zinc finger protein in Arabidopsis, negatively regulates light-dependent seed germination downstream of PIL5. Plant Cell Online. 2008;20:1260–1277.
- Jiang Z, Xu G, Jing Y, et al. Phytochrome B and REVEILLE1/2-mediated signalling controls seed dormancy and germination in Arabidopsis. Nat Commun. 2016;7(1):1–10.
- Yang L, Jiang Z, Liu S, et al. Interplay between REVEILLE1 and RGA‐LIKE2 regulates seed dormancy and germination in Arabidopsis. New Phytol. Epub ahead of print. 2019. DOI:10.1111/nph.16236.
- Nobutaka M, Toru H, Kunio T, et al. VOZ; isolation and characterization of novel vascular plant transcription factors with a one-zinc finger from Arabidopsis thaliana. Plant Cell Physiol. 2004;45:845–854.
- Yasui Y, Mukougawa K, Uemoto M, et al. The phytochrome-interacting VASCULAR PLANT ONE-ZINC FINGER1 and VOZ2 redundantly regulate flowering in Arabidopsis. Plant Cell. 2012;24(8):3248–3263.
- Celesnik H, Ali GS, Robison FM, et al. Arabidopsis thaliana VOZ (Vascular plant One-Zinc finger) transcription factors are required for proper regulation of flowering time. Biol Open. 2013;2:424–431.
- Yasui Y, Kohchi T. VASCULAR PLANT ONE-ZINC FINGER1 and VOZ2 repress the FLOWERING LOCUS C clade members to control flowering time in Arabidopsis. Biosci Biotechnol Biochem. 2014;78(11):1850–1855.
- Kumar S, Choudhary P, Gupta M, et al. VASCULAR PLANT ONE-ZINC FINGER1 (VOZ1) and VOZ2 interact with CONSTANS and promote photoperiodic flowering transition. Plant Physiol. 2018;176(4):2917–2930.
- Nakai Y, Nakahira Y, Sumida H, et al. Vascular plant one-zinc-finger protein 1/2 transcription factors regulate abiotic and biotic stress responses in Arabidopsis. Plant J. 2013;73:761–775.
- Nakai Y, Fujiwara S, Kubo Y, et al. Overexpression of VOZ2 confers biotic stress tolerance but decreases abiotic stress resistance in Arabidopsis. Plant Signal Behav. 2013;8:2–5.
- Koguchi M, Yamasaki K, Hirano T, et al. Vascular plant one-zinc-finger protein 2 is localized both to the nucleus and stress granules under heat stress in Arabidopsis. Plant Signal Behav. 2017;12(3):1–7.
- Song C, Lee J, Kim T, et al. VOZ1, a transcriptional repressor of DREB2C, mediates heat stress responses in Arabidopsis. Planta. 2018;247:1439–1448.
- Prasad KVSK, Xing D, Reddy ASN. Vascular plant one-zinc-finger (VOZ) transcription factors are positive regulators of salt tolerance in Arabidopsis. Int J Mol Sci. 2018;19(12):3731. Epub ahead of print.
- Reed JW, Nagpal P, Poole DS, et al. Mutations in the gene for the red/far-red light receptor phytochrome B alter cell elongation and physiological responses throughout Arabidopsis development. Plant Cell. 1993;5(2):147–157.
- Clough SJ, Bent AF. Floral dip: a simplified method for Agrobacterium-mediated transformation of Arabidopsis thaliana. Plant J. 1998;16(6):735–743.
- Peng J, Yu D, Wang L, et al. Arabidopsis F-box gene FOA1 involved in ABA signaling. Sci China Life Sci. 2012;55(6):497–506.
- Liu H, Yu X, Li K, et al. Photoexcited CRY2 interacts with CIB1 to regulate transcription and floral initiation in Arabidopsis. Science. 2008;322(5907):1535–1539.
- He R, Li X, Zhong M, et al. A photo-responsive F-box protein FOF2 regulates floral initiation by promoting FLC expression in Arabidopsis. Plant J. 2017;91:788–801.
- Li C, Liu X, Qiang X, et al. EBP1 nuclear accumulation negatively feeds back on FERONIA-mediated RALF1 signaling. PLOS Biology. 2018;16(10):e2006340. Epub ahead of print.
- Shinomura T, Nagatani A, Chory J, et al. The induction of seed germination in Arabidopsis thaliana is regulated principally by phytochrome B and secondarily by phytochrome A. Plant Physiol. 1994;104(2):363–371.
- Shinomura T, Hanzawa H, Schäfer E, et al. Mode of phytochrome B action in the photoregulation of seed germination in Arabidopsis thaliana. Plant J. 1998;13(5):583–590.
- Winter D, Vinegar B, Nahal H, et al. An ‘electronic fluorescent pictograph’ browser for exploring and analyzing large-scale biological data sets. PLoS One. 2007;2(8):1–12.
- Alonso-Blanco C, Bentsink L, Hanhart CJ, et al. Analysis of natural allelic variation at seed dormancy loci of Arabidopsis thaliana. Genetics. 2003;164(2):711–729.
- Bentsink L, Jowett J, Hanhart CJ, et al. Cloning of DOG1, a quantitative trait locus controlling seed dormancy in Arabidopsis. Proc Natl Acad Sci. 2006;103(45):17042–17047.
- Nakabayashi K, Bartsch M, Xiang Y, et al. The time required for dormancy release in Arabidopsis is determined by DELAY OF GERMINATION1 protein levels in freshly harvested seeds. Plant Cell. 2012;24(7):2826–2838.
- Yamaguchi S, Smith MW, Brown RGS, et al. Phytochrome regulation and differential expression of gibberellin 3β-hydroxylase genes in germinating Arabidopsis seeds. Plant Cell. 1998;10:2115–2126.
- Sun T. Molecular mechanism of gibberellins signalling in plants. Annu Biol. 2004;55:197–223.
- Oh E, Kang H, Yamaguchi S, et al. Genome-wide analysis of genes targeted by PHYTOCHROME INTERACTING FACTOR 3-LIKE5 during seed germination in Arabidopsis. Plant Cell. 2009;21(2):403–419.