ABSTRACT
Pancreatic cancer (PC), highly malignant, is one of the most lethal cancers. Interferon-induced transmembrane protein 1 (IFITM1) has recently been regarded as a new molecular marker in human cancers. However, the role of IFITM1 in PC remains unclear. In this study, a short hairpin RNA (shRNA) was constructed to assess the effect of IFITM1 on PANC-1 and ASPC-1 cells. The level of IFITM1 was downregulated in cells transfected with shRNA targeting IFITM1 (sh-IFITM1). Silencing of IFITM1 significantly decreased cell viability, downregulated the level of Ki-67, arrested cell at G1/S phase, reduced the number of cells in S phase, and decreased cyclinD1, cyclinE, CDK2, and CDK4 levels. Moreover, Hoechst staining and Western blotting analysis showed that cell apoptosis was induced by IFITM1. IFITM1 knockdown suppressed the MAPK signaling pathway by downregulation of p-ERK, p-P38, and p-JNK levels. These findings suggested that IFITM1 could be considered a potential therapeutic target for PC.
GRAPHICAL ABSTRACT
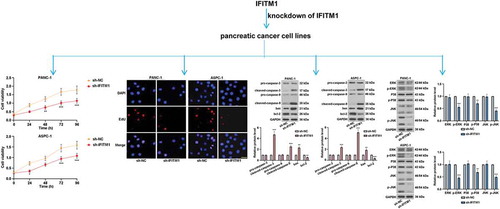
IFITM1 silencing inhibited the proliferation of pancreatic cancer cells, decreased the number of cells, induced cell apoptosis, and suppressed the MAPK signaling pathway.
A series of risk factors such as tobacco use, obesity, diabetes, alcohol use, and chronic pancreatitis concomitant with genetic syndromes are involved in the occurrence and development of pancreatic cancer (PC) [Citation1]. PC, a fatal disease, is one of the most common and aggressive malignant tumors occurring in the digestive system around the world and characterized by rapid and uncontrolled growth [Citation1,Citation2]. Owing to the lack of early detection and effective interventions, PC is usually diagnosed at an advanced stage and has the poorest prognosis in cancer malignancy, with a low 5-year survival rate of 7.7% [Citation3–Citation5]. Some measures including surgery, chemotherapy and targeted therapy, radiotherapy, and traditional Chinese medicine have been taken for the treatment of PC; however, its incidence and mortality rate are still increasing [Citation1,Citation3,Citation5]. Therefore, it is imperative to seek for original therapies for PC.
Interferon-induced transmembrane protein 1 (IFITM1, 17 kDa, alias CD225 or Leu 13 or 9–27) is a member of the interferon-mediated transmembrane protein family and a component of a membrane complex [Citation6,Citation7]. IFITM1 participates in varieties of cellular functions including proliferation, homotypic adhesion in lymphocytes, and metastasis [Citation8]. It also plays an important role in immunity and antiviral activity and is closely associated with inflammatory bowel disease, osteogenesis, and angiogenesis [Citation9]. The study by Yu et al. [Citation10] indicated that inhibition of IFITM1 level via siIFITM1 suppressed proliferation, migration, and invasion of glioma cells. Numerous investigations confirmed that IFITM1 was highly expressed in colorectal cancer, non-small-cell lung cancer, ovarian cancer, and gastric cancer and promoted the migration and invasion of tumor cells [Citation6–Citation8,Citation11]. At the same time, the level of IFITM1 was correlated with a poor prognosis of tumors [Citation7,Citation12]. However, low expression of IFITM1 was observed in cervical cancer. IFITM1 also inhibited the growth and metastasis of cervical cancer [Citation13]. Moreover, IFITM1 had an influence on tumor tolerance to radiotherapy and chemotherapy [Citation14,Citation15]. Rogers et al. [Citation16] reported that IFITM1 was overexpressed in PC tissues. Gene expression profiling interactive analysis (GEPIA) also shows that IFITM1 is highly expressed in pancreatic adenocarcinoma (PAAD). Nevertheless, the role of IFITM1 in PC and the underlying mechanism have not been explored.
In the present study, a short hairpin RNA (shRNA) was constructed to silence the level of IFITM1 in PC cell lines (PANC-1 and ASPC-1), and the effect of IFITM1 knockdown on PC cells was also investigated. It was reported for the first time that knockdown of IFITM1 inhibited the proliferation of PC cell lines, induced cell cycle arrest, facilitated cell apoptosis, and suppressed MAPK signaling pathway.
Materials and methods
Materials
Human PC cell lines (PANC-1 and ASPC-1) were purchased from Procell Life Science & Technology Co., Ltd (Wuhan, China). DMEM and RPMI-1640 medium were provided by Gibco (USA). Fetal bovine serum (FBS) was obtained from Hyclone (USA). 3-(4,5-Dimethylthiazol-2-yl)-2,5-diphenyltetrazolium bromide (MTT) and 5-ethynyl-2ʹ-deoxyuridine (EdU) imaging test kits were obtained from KeyGEN BioTECH (Nanjing, China). Trypsin, dimethyl sulfoxide (DMSO), cell cycle detection kit, Hoechst staining kit, and 4ʹ,6-diamidino-2-phenylindole (DAPI) were provided by Beyotime Biotechnology (Shanghai, China).
GEPIA dataset
GEPIA (http://gepia.cancer-pku.cn/) is an interactive web server used for analyzing the RNA sequencing expression, including 9736 tumors and 8587 normal samples from the TCGA and the GTEx projects. GEPIA supplies a series of functions including tumor/normal differential level analysis, survival curves, and gene expression correlation analysis. In this study, we compared the level of IFITM1 gene in tumor and normal PAAD tissues.
Cell culture and transfection
PANC-1 and ASPC-1 cells were incubated in DMEM or RPMI-1640 medium supplemented with 10% FBS in an incubator (37°C, 5% CO2). The cell lines were subcultured by digestion with 0.25% trypsin when they reached about 90% confluency. Cells were transiently transfected with shRNA against IFITM1 (sh-IFITM1) or negative control (sh-NC) or IFITM1 shRNA-2 using Lipofectamine 2000 (11668-019, Invitrogen, USA). The sequences of sh-NC and sh-IFITM1 are shown in . After transfection for 48 h, cells were observed under a fluorescence microscope (BX53, OLYMPUS, Japan). The sequences of sh-NC (NC shRNA) and IFITM1 shRNA-2 were listed in Table S1.
Table 1. The sequences of sh-NC and sh-IFITM1 used for cell transfection.
MTT assay
PANC-1 or ASPC-1 cells were seeded in a 96-well plate (4 × 103 cells/well) and cultured until cell attachment. Then, Cells were transfected and incubated for 0, 24, 48, 72, and 96 h, respectively. After incubation, MTT (150 μL, 0.5 mg/mL) was added to each well and reacted for 4 h at 37°C. 150 μL of DMSO was added to each well. After reaction for 10 min in the dark, the plate was read at 570 nm utilizing a microplate reader (ELX-800, Biotek, USA). Meanwhile, cells were treated with ERK inhibitor (PD98095, 50 μM) or JNK inhibitor (SP600125, 10 μM) or P38 inhibitor (SB203580, 10 μM) and incubated for 0, 24, 48, 72, and 96 h. Cell viability was detected by MTT assay.
Cell cycle analysis
PANC-1 and ASPC-1 cells were cultured in 6-well plates (2 × 106 cells/well), respectively. After transfection for 48 h, cells were collected and washed twice with PBS by centrifugation (310 g, 5 min). Cells were fixed with ice-cold 70% ethanol overnight at 4°C. After centrifugation, cells were collected and washed. Then, 500 μL of staining solution (RNase A:PI = 1:9) was added to cells and mixed. Samples were analyzed by a flow cytometry (NovoCyte, ACEA Biosciences Inc., USA) after incubation at room temperature (RT) in the dark for 40 min.
EdU assay
PANC-1 or ASPC-1 cells (4 × 103 cells/well) were incubated in 96-well plates. After transfection for 48 h, EdU (10 μM, 100 μL) was added to each well, and cells were incubated for 2 h. Cells were fixed with 4% paraformaldehyde at RT for 15 min. After being washed twice with 3% bovine serum albumin (BSA) in PBS, 0.5% Triton X-100 in PBS (0.1 mL) was added to cells and incubated for 20 min. Cells were washed twice and Click-iT (100 μL) was added to each well. After reaction in the dark for 30 min, cells were washed twice and stained with Hoechst (1:2000 in PBS) for 15 min. Cells were visualized by a fluorescence microscope after being washed twice with 3% BSA.
Hoechst staining
PANC-1 and ASPC-1 cells (1 × 105 cells/well) were cultured in 12-well plates, respectively. After transfection or treatment for 48 h, cells were fixed for 10 min and washed with phosphate buffer solution (PBS) twice. Then, Cells were stained with Hoechst (500 μL/well) and incubated for 5 min. After being washed twice, cells were observed using a fluorescence microscope.
Quantitative real-time PCR
After transfection for 48 h, total RNA was extracted from PANC-1 or ASPC-1 cells using an RNA isolation kit (DP419, TianGen Biotech Co., Ltd., China) in accordance with the manufacturer’s recommendation. The cDNA was synthesized utilizing M-MLV reverse transcriptase (NG212, TianGen). RT-PCR was performed using fluorometer (ExicyclerTM 96, BIONEER, Korea), and the reaction mix included cDNA template (1 μL), SYBR Green (0.3 μL, SY1020, Solarbio Science & Technology, China), 2 × Taq PCR MasterMix (10 μL, KT201, TianGen), nuclease-free (7.7 μL), and primers (10 μM, 0.5 μL each, GenScript Co., Ltd., China). The primers were as follows: GAPDH (sense: GACCTGACCTGCCGTCTAG; antisense: AGGAGTGGGTGTCGCTGT) and IFITM1 (sense: GGGCTTCATAGCATTCGC; antisense: CAGATGTTCAGGCACTTGG). IFITM1 mRNA level was measured with the formula: 2−ΔΔCt.
Western blotting
After transfection for 48 h, PANC-1 and ASPC-1 cells were harvested, respectively. Cells were suspended in RIPA lysis buffer (R0010, Solarbio Science & Technology) supplemented with 1% phenylmethylsulphonyl fluoride (P0100, Solarbio Science & Technology) and reacted on ice for 5 min. After centrifugation for 5 min (10,000 g, 4°C), the supernatant was collected. Protein concentration was detected by BCA kit (PC0020, Solarbio Science & Technology) according to the manufacturer’s instructions. Samples were separated by gel electrophoresis and transferred onto polyvinylidene difluoride (PVDF) membranes (IPVH00010, Millipore, USA). The membranes were blocked with 5% nonfat milk or 5% BSA for 1 h. Then, The membranes were incubated using primary antibody overnight at 4°C and secondary antibody for 60 min at 37°C. After being washed with Tris-buffered saline containing Tween 20 (TBST), the bands were assessed utilizing ECL kit (PE0010, Solarbio Science & Technology) and analyzed by Gel-Pro-Analyzer software. The information on antibodies is listed in .
Table 2. Antibodies used for Western blotting.
Immunofluorescence assay
After transfection for 48 h, PANC-1 or ASPC-1 cells were fixed with 4% paraformaldehyde for 15 min and washed with PBS three times each for 5 min. Next, 0.1% Triton X-100 was added to each well and incubated for 30 min at RT. After washing thrice, cells were cultured with goat serum (SL038, Solarbio Science & Technology) for 15 min and then incubated with Ki-67 (1:200 in PBS, A11005, Abclonal, China) at 4°C overnight. Cells were washed three times and incubated with goat anti-rabbit IgG (1:300, A0516, Beyotime Biotechnology) for 1 h in the dark. Finally, cells were stained with DAPI and visualized with a fluorescence microscope.
Data analysis
The results were expressed as mean ± SD. The statistical significance of differences among the three groups was analyzed by one-way ANOVA followed by Tukey’s multiple comparisons test, and the difference between the two groups was analyzed by unpaired t-test. The results of MTT assay were analyzed by two-way ANOVA followed by Tukey’s multiple comparisons test. *p < 0.05 was considered significant.
Results
The expression of IFITM1 was upregulated in PAAD
To assess differences of IFITM1 expression in tumor and normal PAAD tissues, the IFITM1 mRNA level was analyzed utilizing the GEPIA dataset. As displayed in , the expression of IFITM1 was prominently higher in PAAD tissues than that of normal tissues, which provided a theoretical basis for the following experiments.
Figure 1. Interferon-induced transmembrane protein 1 (IFITM1) expression level in pancreatic adenocarcinoma (PAAD) compared with TCGA normal and GTEx data utilizing gene expression profiling interactive analysis (GEPIA) webserver. It was found that IFITM1 was significantly upregulated in the tumor. For TCGA tumor (red), its matched normal and GTEx data (blue) were given. X-axis: left, patients with PAAD; right, normal tissue adjacent to PAAD; T, tumor; N, normal; num, number. Y-axis: TPM, transcript per million. *p < 0.05 vs. normal tissue.
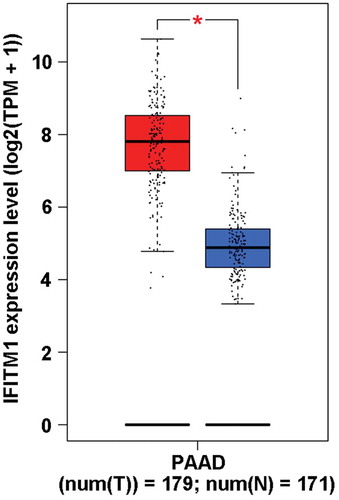
IFITM1 knockdown inhibited the proliferation of PC cells
) shows that the transfection effect of IFITM1 on PANC-1 or ASPC-1 cells was obvious in sh-NC and sh-IFITM1 groups compared with the parental group. As depicted in ), there were no marked changes in IFITM1 mRNA level between the parental and sh-NC groups. Meanwhile, IFITM1 mRNA level was reduced in PANC-1 or ASPC-1 cells transfected with sh-IFITM1. Consistently, the expression of IFITM1 was downregulated in sh-IFITM1 groups ()). Besides, MTT assay was used to detect cell proliferation. It can be seen from ) that silencing of IFITM1 decreased cell viability compared to cells transfected with sh-NC. In PANC-1 cells, IFITM1 silencing resulted in a significant decrease in cell viability at 48 or 72 or 96 h. Similarly, IFITM1 knockdown apparently reduced the viability of ASPC-1 cells at 24 or 72 or 96 h. In order to exclude the possibility of off-target effects of the shRNA, IFITM1 shRNA-2 was also used in this study. The level of IFITM1 and cell viability were significantly lowered in PANC-1 or ASPC-1 cells transfected with IFITM1 shRNA-2 (Figure S1). These results suggested that the efficiency of IFITM1 shRNA-2 was similar to that of sh-IFITM1. Apart from these, the immunofluorescence assay was performed to evaluate the level of Ki-67. As illustrated in ), shRNA against IFITM1 remarkably inhibited the nuclear translocation of Ki-67 and reduced the ratio of Ki-67-positive cells to total cells in PANC-1 or ASPC-1 cells in comparison with sh-NC groups. These results indicated that knockdown of IFITM1 prominently suppressed the proliferation of PC cells.
Figure 2. IFITM1 knockdown inhibited the proliferation of pancreatic cancer cells. (a) PANC-1 and ASPC-1 cells were transfected with negative control (sh-NC) or short hairpin RNA targeting IFITM1 (sh-IFITM1), respectively. After transfection for 48 h, the transfection efficiency was observed by a fluorescence microscope. Scale bar: 200 μm. (b) After transfection for 48 h, IFITM1 mRNA level was detected via quantitative real-time PCR in PANC-1 and ASPC-1 cells, respectively. GAPDH was used as an internal reference. (c) After transfection for 48 h, the expression of IFITM1 was determined by Western blotting in PANC-1 or ASPC-1 cells. GAPDH was used as an internal reference. (d) After transfection and the replacement of the cell medium, MTT assay was utilized to evaluate the viability of PANC-1 or ASPC-1 cells at 0, 24, 48, 72, and 96 h, respectively. (e) After transfection for 48 h, the level of Ki-67 was detected with immunofluorescence assay in PANC-1 and ASPC-1 cells. Scale bar: 50 μm. The ratio of Ki-67-positive cells to total cells was calculated in PANC-1 or ASPC-1 cells. Results were expressed as mean ± SD (n = 3). *p < 0.05, **p < 0.01, and ***p < 0.001 vs. sh-NC group.
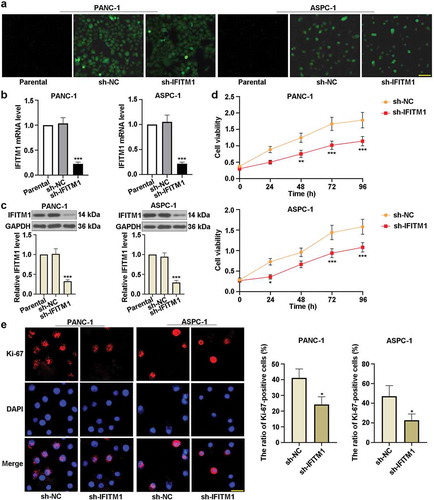
IFITM1 knockdown induced cell cycle arrest in G1/S phase
Flow cytometry was utilized to visualize the effect of IFITM1 knockdown on cell cycle. It was found that the percentage of cells in G1 phase was obviously increased but significantly decreased at S phase in PANC-1 or ASPC-1 cells transfected with sh-IFITM1 ()). EdU assay results revealed that IFITM1 silencing markedly reduced the number of PANC-1 or ASPC-1 cells in S phase compared with cells transfected with sh-NC ()). Moreover, the expressions of cyclinD1, cyclinE, CDK2, and CDK4 were assessed by Western blotting after transfection for 48 h. As displayed in ), an obvious reduction in cyclinD1, cyclinE, CDK2, and CDK4 levels was observed in PANC-1 and ASPC-1 cells by silencing of IFITM1. These findings suggested that cell cycle arrest was induced by IFITM1 knockdown in PC cells.
Figure 3. IFITM1 knockdown induced cell cycle arrest in G1/S phase. (a) PANC-1 and ASPC-1 cells were transfected with sh-NC or sh-IFITM1, respectively. After transfection for 48 h, cell cycle was detected using flow cytometry. (b) After transfection for 48 h, cell proliferation was assessed by EdU assay in PANC-1 and ASPC-1 cells, respectively. Scale bar: 50 μm. (c) After transfection for 48 h, the expressions of cyclinD1, cyclinE, CDK2, and CDK4 were evaluated with Western blotting in PANC-1 or ASPC-1 cells. GAPDH was used as an internal reference. Data were present as mean ± SD (n = 3). *p < 0.05, **p < 0.01, and ***p < 0.001 vs. sh-NC group.
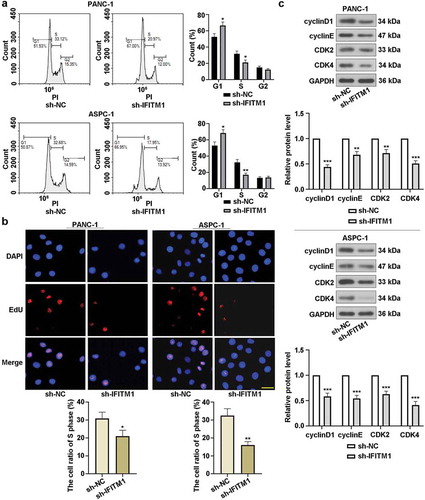
IFITM1 knockdown mediated cell apoptosis
To confirm the role of IFITM1 knockdown in cell apoptosis, PANC-1 or ASPC-1 cells were stained with Hoechst after transfection for 48 h. There were no obvious morphological changes in sh-NC groups. However, nuclear condensation and apoptotic bodies were visualized in PANC-1 and ASPC-1 cells transfected with sh-IFITM1, respectively ()). Furthermore, Western blotting was carried out to detect changes in the levels of proteins involved in apoptosis. As exhibited in ), IFITM1 silencing upregulated cleaved-caspase-3, cleaved-caspase-9, and bax levels, whereas the expression of bcl-2 was downregulated in cells transfected with IFITM1. Meanwhile, no differences in the levels of pro-caspase-3 and pro-caspase-9 were seen in sh-NC and sh-IFITM1 groups. These results showed that silencing of IFITM1 could prompt PC cell apoptosis.
Figure 4. IFITM1 knockdown mediated cell apoptosis. (a) PANC-1 and ASPC-1 cells were transfected with sh-NC or sh-IFITM1, respectively. After transfection for 48 h, cells were stained with Hoechst and observed via a fluorescence microscope. Scale bar: 50 μm. (b) After transfection for 48 h, pro-caspase-3, cleaved-caspase-3, pro-caspase-9, cleaved-caspase-9, bax, and bcl-2 levels were detected by Western blotting in PANC-1 and ASPC-1 cells, respectively. GAPDH was used as an internal reference. Results were expressed as mean ± SD (n = 3). **p < 0.01 and ***p < 0.001 vs. sh-NC group.
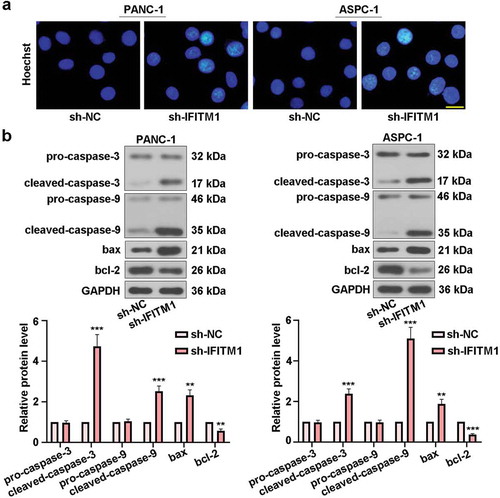
IFITM1 knockdown suppressed MAPK signaling pathway
It can be seen from ) that IFITM1 silencing led to significant decreases in p-ERK, p-P38, and p-JNK levels. At the same time, in PANC-1 cells transfected with sh-IFITM1 or sh-NC, there were no obvious changes in the expressions of ERK, P38, and JNK. The results of ) in ASPC-1 cells were similar to that of ). The above findings indicated that IFITM1 knockdown inhibited MAPK signaling pathway in PC cells.
Figure 5. IFITM1 knockdown suppressed MAPK signaling pathway. PANC-1 and ASPC-1 cells were transfected with sh-NC or sh-IFITM1, respectively. After transfection for 48 h, the levels of ERK, p-ERK, P38, p-P38, JNK, and p-JNK were assessed via Western blotting in PANC-1 (a) or ASPC-1 (b) cells. GAPDH was used as an internal reference. Data were present as mean ± SD (n = 3). **p < 0.01 and ***p < 0.001 vs. sh-NC group.
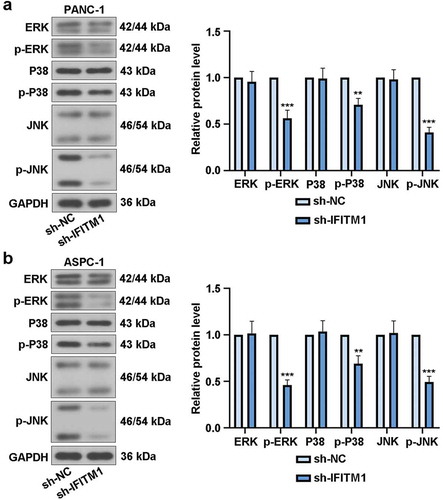
The causality between MAPK inhibitors and cell viability/apoptosis
To assess the causality between MAPK inhibitors and cell viability/apoptosis, PD98095 or SP600125 or SB203580 was used as a positive control. As shown in ), knockdown of IFITM1 significantly reduced cell viability in PANC-1 and ASPC-1 cells compared to cells transfected with sh-NC. Meanwhile, compared with parental group, PD98095 or SP600125 or SB203580 treatment decreased cell viability, which was similar to that of IFITM1 knockdown. Besides, Hoechst staining was utilized to assess cell apoptosis. As depicted in ), compared with sh-NC group, IFITM1 silencing obviously mediated cell apoptosis, accompanied by elevated apoptotic rate in PANC-1 and ASPC-1 cells. At the same time, the administration of PD98095 or SP600125 or SB203580 induced cell apoptosis in comparison with control cells, which was in agreement with that of IFITM1 silencing. These results indicated that inhibition of the MAPK signaling pathway could lower cell viability and induce apoptosis in PC cells.
Figure 6. IFITM1 knockdown lowered cell viability and induced cell apoptosis. PANC-1 and ASPC-1 cells were transfected with sh-NC or sh-IFITM1, respectively. Meanwhile, cells were treated with PD98095 or SP600125 or SB203580. (a) MTT assay was utilized to evaluate cell viability at 0, 24, 48, 72, and 96 h, respectively. (b) After transfection or treatment for 48 h, cells were stained with Hoechst and observed via a fluorescence microscope. Scale bar: 50 μm. The apoptotic rate was calculated in PANC-1 or ASPC-1 cells. Results were expressed as mean ± SD (n = 3). *p < 0.05, **p < 0.01, and ***p < 0.001 vs. sh-NC group; #p < 0.05, ##p < 0.01, and ###p < 0.001 vs. parental group.
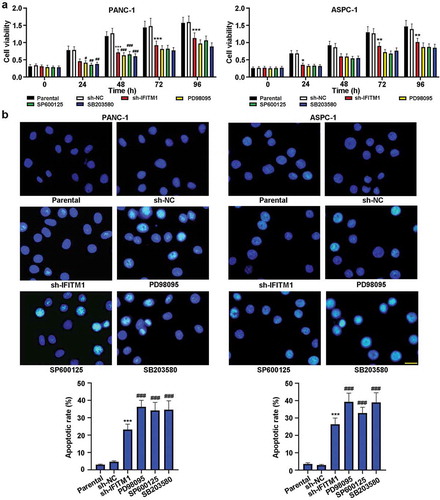
Discussion
This study provided evidence for the role of IFITM1 in PC cells’ proliferation and apoptosis for the first time. It was confirmed that the level of IFITM1 in PANC-1 and ASPC-1 cells transfected with sh-IFITM1 was decreased, indicating successful transfection efficiency. In addition, a series of assays validated that knockdown of IFITM1 inhibited cell proliferation, induced cell cycle arrest, promoted apoptosis, and suppressed the MAPK signaling pathway.
Cell proliferation and apoptosis play vital roles in the oncogenesis and development of PC [Citation17,Citation18]. It has been reported that IFITM1 gene is an essential factor regulating cell growth [Citation13]. For example, Sari et al. [Citation12] pointed out that the proliferation of colorectal cancer cell lines (SW620 and HT29) was modestly impaired in the absence of IFITM1. IFITM1 overexpression ameliorated breast cancer cell survival and aggression in estrogen-deprived situations [Citation19]. Besides, Ki-67 protein was considered a marker of cell proliferation [Citation20]. In the present study, a decrease in cell viability was induced by knockdown of IFITM1 in PANC-1 and ASPC-1 cells and IFITM1 silencing significantly repressed the nuclear translocation of Ki-67. These findings were similar to those reported by previous studies [Citation9,Citation10,Citation14,Citation21]. Moreover, Ki-67 is existed in all phases of cell cycle except G0 phase [Citation22]. Cell cycle process is vital for replicating cells accomplished by a variety of checkpoints, which can be activated via DNA damage. Under the above circumstance, cell cycle arrest can contribute to the recovery of damage [Citation23]. Furthermore, the previous report showed that silencing of long noncoding RNA EPIC1 significantly induced cell cycle arrest in PC cells [Citation24]. In the current study, IFITM1 knockdown blocked cell cycle progression at G1/S phase and decreased the number of PANC-1 or ASPC-1 cells in S phase. What is more, cyclinD1, cyclinE, and CDK4 are mostly expressed at G1 phase, whereas CDK2 is especially existed in S phase [Citation23,Citation25]. The results revealed that the expressions of cyclinD1, cyclinE, CDK2, and CDK4 were downregulated by IFITM1 silencing, which was in line with the previous report [Citation26].
Hoechst staining was carried out to visualize the morphological changes in PANC-1 and ASPC-1 cells. When cells were transfected with sh-IFITM1, cells with chromatin condensation and apoptotic bodies were significantly increased compared with sh-NC groups. Apoptosis can be induced by extrinsic and/or intrinsic pathways [Citation20]. The bcl-2 family maintains the balance between proapoptotic and antiapoptotic proteins such as bax and bcl-2. If the balance breaks, apoptosis may be mediated [Citation27]. Besides, the caspase family including caspase-3, caspase-7, and caspase-9 was closely related to apoptotic cell death [Citation28,Citation29]. Caspase-3 is rarely muted in PC and regarded as a marker for evaluating apoptosis [Citation28]. In the current study, the expressions of caspase-3, caspase-9, bax, and bcl-2 were detected by Western blotting. The results revealed that knockdown of IFITM1 increased the levels of cleaved-caspase-3, cleaved-caspase-9, and bax but decreased the expression of bcl-2, indicating that IFITM1 knockdown induced PC cell apoptosis. Huang et al. [Citation18] reported that baicalin markedly promoted apoptosis of PC SW1990 cells. Nevertheless, as we all know, this study was the first finding that IFITM1 silencing could mediate PANC-1 and ASPC-1 cell apoptosis.
The MAPK signaling pathway, mainly including ERK, P38, and JNK, regulates cell proliferation, migration, and apoptosis [Citation27,Citation30]. For instance, the previous study indicated that ITGA6 and RPSA enhanced the invasive and metastatic properties of PC cells by activating the MAPK/ERK pathway [Citation31]. P38 is an essential protein during intracellular signal transduction [Citation32] and expressed in a series of cell types [Citation33]. The previous research reported that JNK activation resulted in different responses. Transient JNK activation promoted cell survival, whereas the prolonged activation of JNK led to cell death [Citation30]. As reported by Kim et al. [Citation34] in THP-1 cells, the expression of IFITM1 could be induced by the activation of CD147 with cyclophilin through upregulation of ERK, PI3K, and NF-κB. The previous research revealed that the interaction between IFITM1 and caveolin-1 (CAV-1) could elevate the inhibitory role of CAV-1 in ERK activation [Citation35]. Nevertheless, the effects of IFITM1 knockdown on ERK, P38, and JNK are unknown. This study revealed that the levels of p-ERK, p-P38, and p-JNK were downregulated in cells transfected with sh-IFITM1, demonstrating that IFITM1 knockdown suppressed the MAPK pathway in PC cells. To the best of our knowledge, this finding has not been reported so far. Our results in this study suggested that silencing of IFITM1 was possibly through inhibiting the activation of the MAPK signaling pathway to repress cell proliferation and induce cell cycle arrest and apoptosis in PC cells. Moreover, Yan et al. [Citation9] found that silencing of IFITM1 inhibited Wnt/β-catenin signaling pathway in lung cancer cells and tumor tissues. Therefore, Wnt/β-catenin pathway can be worthy of study in PC in future.
In summary, silencing of IFITM1 suppressed the proliferation of PANC-1 and ASPC-1 cells. Moreover, knockdown of IFITM1 arrested cell in G1/S phase, reduced the number of cells at S phase, and downregulated the expressions of proteins involved in cell cycle. Hoechst staining and Western blotting results suggested that IFITM1 knockdown promoted cell apoptosis. It was further found that IFITM1 silencing had a suppressive effect on the MAPK signaling pathway. Therefore, IFITM1 could be acted as a novel molecular target for the treatment of PC.
Author contribution
WC was responsible for the research design and participated in the paper. LZ drafted the manuscript and reviewed the literature. WC, ZW, DK, XZ, and XC participated in the qRT-PCR, Western blotting, and cellular function experiments. All authors were responsible for the data analysis and figure format.
Supplementary_File.pdf
Download PDF (368.3 KB)Disclosure statement
No potential conflict of interest was reported by the authors.
Supplementary data
Supplemental material for this article can be accessed here.
References
- Lin QJ, Yang F, Jin C, et al. Current status and progress of pancreatic cancer in China. World J Gastroenterol. 2015;21:7988–8003.
- Yan X, Hui Y, Hua Y, et al. EG-VEGF silencing inhibits cell proliferation and promotes cell apoptosis in pancreatic carcinoma via PI3K/AKT/mTOR signaling pathway. Biomed Pharmacother. 2019;109:762–769.
- Mohammed A, Rao CV. Pancreatic cancer chemoprevention: challenges and opportunities. Curr Med Chem. 2018;25:2532–2534.
- Karakas Y, Lacin S, Yalcin S. Recent advances in the management of pancreatic adenocarcinoma. Expert Rev Anticancer. 2018;18:51–62.
- Liu F, Xia Z, Zhang M, et al. SMARCAD1 promotes pancreatic cancer cell growth and metastasis through Wnt/beta-catenin-mediated EMT. Int J Biol Sci. 2019;15:636–646.
- Lee J, Goh SH, Song N, et al. Overexpression of IFITM1 has clinicopathologic effects on gastric cancer and is regulated by an epigenetic mechanism. Am J Pathol. 2012;181:43–52.
- Yang YG, Koh YW, Sari IN, et al. Interferon-induced transmembrane protein 1-mediated EGFR/SOX2 signaling axis is essential for progression of non-small cell lung cancer. Int J Cancer. 2019;144:2020–2032.
- Kim NH, Sung HY, Choi EN, et al. Aberrant DNA methylation in the IFITM1 promoter enhances the metastatic phenotype in an intraperitoneal xenograft model of human ovarian cancer. Oncol Rep. 2014;31:2139–2146.
- Yan J, Jiang Y, Lu J, et al. Inhibiting of proliferation, migration, and invasion in lung cancer induced by silencing interferon-induced transmembrane protein 1 (IFITM1). Biomed Res Int. 2019;2019:9085435.
- Yu F, Ng SS, Chow BK, et al. Knockdown of interferon-induced transmembrane protein 1 (IFITM1) inhibits proliferation, migration, and invasion of glioma cells. J Neuro-oncol. 2011;103:187–195.
- Yu F, Xie D, Ng SS, et al. IFITM1 promotes the metastasis of human colorectal cancer via CAV-1. Cancer Lett. 2015;368:135–143.
- Sari IN, Yang YG, Phi LT, et al. Interferon-induced transmembrane protein 1 (IFITM1) is required for the progression of colorectal cancer. Oncotarget. 2016;7:86039–86050.
- Zheng W, Zhao Z, Yi X, et al. Down-regulation of IFITM1 and its growth inhibitory role in cervical squamous cell carcinoma. Cancer Cell Int. 2017;17:88.
- Yang J, Li L, Xi Y, et al. Combination of IFITM1 knockdown and radiotherapy inhibits the growth of oral cancer. Cancer Sci. 2018;109:3115–3128.
- Fumoto S, Shimokuni T, Tanimoto K, et al. Selection of a novel drug-response predictor in esophageal cancer: a novel screening method using microarray and identification of IFITM1 as a potent marker gene of CDDP response. Int J Oncol. 2008;32:413–423.
- Rogers CD, Fukushima N, Sato N, et al. Differentiating pancreatic lesions by microarray and QPCR analysis of pancreatic juice RNAs. Cancer Biol Ther. 2006;5:1383–1389.
- Liu C, Zhang H, Zang X, et al. The influence of SnoN gene silencing by siRNA on the cell proliferation and apoptosis of human pancreatic cancer cells. Diagn Pathol. 2015;10:30.
- Huang Q, Zhang J, Peng J, et al. Effect of baicalin on proliferation and apoptosis in pancreatic cancer cells. Am J Transl Res. 2019;11:5645–5654.
- Lui AJ, Geanes ES, Ogony J, et al. IFITM1 suppression blocks proliferation and invasion of aromatase inhibitor-resistant breast cancer in vivo by JAK/STAT-mediated induction of p21. Cancer Lett. 2017;399:29–43.
- Katary MA, Abdelsayed R, Alhashim A, et al. Salvianolic acid B slows the progression of breast cancer cell growth via enhancement of apoptosis and reduction of oxidative stress, inflammation, and angiogenesis. Int J Mol Sci. 2019;20:5653.
- Yang G, Xu Y, Chen X, et al. IFITM1 plays an essential role in the antiproliferative action of interferon-gamma. Oncogene. 2007;26:594–603.
- Meyer HJ, Gundermann P, Surov A. Associations between FDG-PET and Ki 67-index in head and neck cancer: a meta-analysis. Medicine (Baltimore). 2019;98:e17472.
- Kim SA, Kang OH, Kwon DY. Cryptotanshinone induces cell cycle arrest and apoptosis of NSCLC cells through the PI3K/Akt/GSK-3beta pathway. Int J Mol Sci. 2018;19:2739.
- Xia P, Liu P, Fu Q, et al. Long noncoding RNA EPIC1 interacts with YAP1 to regulate the cell cycle and promote the growth of pancreatic cancer cells. Biochem Biophys Res Commun. 2020;522:978-985.
- Zhong ZQ, Song MM, He Y, et al. Knockdown of Ezrin by RNA interference reverses malignant behavior of human pancreatic cancer cells in vitro. Asian Pac J Cancer Prev. 2012;13:3781–3789.
- Jin B, Jin H, Wang J. Silencing of interferon-induced transmembrane protein 1 (IFITM1) inhibits proliferation, migration, and invasion in lung cancer cells. Oncol Res. 2017. DOI:10.3727/096504017X14844360974116
- Yoo ES, Choo GS, Kim SH, et al. Antitumor and apoptosis-inducing effects of piperine on human melanoma cells. Anticancer Res. 2019;39:1883–1892.
- Wang Y, Sun L, Luo Y, et al. Knockdown of KDM1B inhibits cell proliferation and induces apoptosis of pancreatic cancer cells. Pathol Res Pract. 2019;215:1054–1060.
- Tassone P, Tagliaferri P, Viscomi C, et al. Zoledronic acid induces antiproliferative and apoptotic effects in human pancreatic cancer cells in vitro. Br J Cancer. 2003;88:1971–1978.
- Mohammad J, Singh RR, Riggle C, et al. JNK inhibition blocks piperlongumine-induced cell death and transcriptional activation of heme oxygenase-1 in pancreatic cancer cells. Apoptosis. 2019;24:730–744.
- Wu Y, Tan X, Liu P, et al. ITGA6 and RPSA synergistically promote pancreatic cancer invasion and metastasis via PI3K and MAPK signaling pathways. Exp Cell Res. 2019;379:30–47.
- Wang Y, Bu C, Wu K, et al. Curcumin protects the pancreas from acute pancreatitis via the mitogen-activated protein kinase signaling pathway. Mol Med Rep. 2019;20:3027–3034.
- Chen Q, Yang C, Chen L, et al. YY1 targets tubulin polymerisation-promoting protein to inhibit migration, invasion and angiogenesis in pancreatic cancer via p38/MAPK and PI3K/AKT pathways. Br J Cancer. 2019;121:912–921.
- Kim JY, Kim H, Suk K, et al. Activation of CD147 with cyclophilin A induces the expression of IFITM1 through ERK and PI3K in THP-1 cells. Mediators Inflammation. 2010;2010:821940.
- Xu Y, Yang G, Hu G. Binding of IFITM1 enhances the inhibiting effect of caveolin-1 on ERK activation. Acta Biochim Biophys Sin. 2009;41:488–494.