ABSTRACT
Systemic lupus erythematosus (SLE) is a chronic autoimmune disease affecting various organs. Decreased numbers of regulatory T-cells (Treg cells; CD4+CD25highFoxp3+) are associated with the pathogenesis of SLE. A vitamin D deficiency was observed in many lupus patients. In the present study, peripheral blood mononuclear cells were isolated and cultured in the presence or absence of vitamin D, and total Tregs percentage was analyzed by flow cytometry. In addition, the level of expressions of Foxp3, TGFβ, and IL6 genes were analyzed by real-time-PCR. The results indicated that vitamin D treatment increased the percentage of Treg cells, and the expression of Foxp3 and TGFβ, and decreased the expression of IL6 in SLE patients.
Introduction
Systemic lupus erythematosus (SLE) is a chronic inflammatory autoimmune disease with diverse clinical manifestations. Several different cells of the immune system are involved in the pathogenesis of SLE (Davis, Hutcheson, & Mohan, Citation2011)..Regulatory T-cells (Treg cells; CD4+CD25highFoxp3+) are a sub-population of T-cells that modulate immune responses, help a host to maintain self-tolerance, and act to prevent the development of autoimmune diseases (Lee et al., Citation2006; Okamoto, Fujio, Okamura, & Yamamoto, Citation2011). Reduced number or function of Treg cells has been noted during active lupus and it was suggested that enhancing Treg cell functions may have beneficial effects on lupus patients (Okamoto et al., Citation2011). Treg cells express the transcription factor Foxp3 that is required for Treg cells development, maintenance, and function. The presence of Foxp3 is essential for Tregs’ regulatory capacity (Fontenot, Gavin, & Rudensky, Citation2003; Hori, Nomura, & Sakaguchi, Citation2007; Khattri, Cox, Yasayko, & Ramsdell, Citation2003), and controls the expression of surface markers and a number of genes that mediate the suppressive activity of Tregs (Ramsdell, Citation2003). Cytokine milieu play a key role in the development and activity of Treg cells and some of them are affected by the state of SLE in a host. Patients with lupus have low serum levels of transforming growth factor β (TGFβ) (Xing, Su, Cui, & Wang, Citation2012). TGFβ plays a role in maintaining host tolerance to self-antigens and has a dualistic role in promoting Treg cell development (Tackey, Lipsky, & Illei, Citation2004). In addition to helping the switch of naïve T-cells into functional Treg cells (Chen et al., Citation2003), TGFβ induces the expansion, differentiation, and suppressive function of Treg cells (Huber et al., Citation2004). Interleukin 6 (IL6) is another important mediator produced by many cell types (Ohl & Tenbrock, Citation2011) and elevated IL6 levels are often found in the serum and urine of lupus patients (Davis et al., Citation2011). IL6 induces B cell activation and production of IgG antibodies against dsDNA (Tackey et al., Citation2004). IL6 can act to cause a decrease in Treg cell levels by causing a suppression of Foxp3 expression. The presence of IL6 together with TGFβ supports the differentiation of naïve T-cells to Th17 cells (Veldhoen, Hocking, Atkins, Locksley, & Stockinger, Citation2006).
The active form of vitamin D (1,25(OH)2D3) (Lane, Citation2010) is a pleiotropic hormone which regulates calcium hemostasis (Szodoray et al., Citation2008). In recent years, vitamin D deficiency has been reported in lupus patients in the active phase of disease (Chun, Liu, Modlin, Adams, & Hewison, Citation2014) and environmental factors (possibly including vitamin D levels) affect the regulation of the immune system, including differentiation and function of Tregs (Chun et al., Citation2014; Szodoray et al., Citation2008). In order to elucidate the mechanism or mechanisms of this regulation, we sought to examine the in vitro effects of vitamin D on Treg cells and the expression levels of IL6 and TGFβ cytokines that have an impact on Treg cells as well as the intracellular Foxp3 in lupus patients. In particular, this study utilized peripheral blood mononuclear cells (PBMCs) from SLE patients to ascertain if vitamin D could be used as a potential therapy for the treatment of the disease.
Methods
Participants
Thirty SLE patients with a mean age of 34.4 ± 9.3 years (27 women, 3 men) were recruited from the Rheumatology Center of Ghaem Hospital, Mashhad University of Medical Sciences (Mashhad, Iran). All subjects fulfilled at least four of the revised SLE criteria of the American College of Rheumatology's 1997 revised criteria for the classification of SLE (Alvarado-Sánchez et al., Citation2006). Twelve out of the 30 SLE patients were new cases with active SLE and had received no therapeutic intervention prior to the sampling and 18 were in remission cases which received only a maximum dose of 10 mg/day prednisolone and/or 200 mg/day of hydroxychloroquine. Written informed consent was taken from all subjects prior to providing blood samples. The Ethics Council of Mashhad University of Medical Sciences (Mashhad, Iran) approved all protocols used with these subjects in the studies herein.
To assess the effects of vitamin D on Treg cells and related cytokines in SLE patients, PBMCs were cultured in the presence or absence of 50 nM of 1,25(OH)2D3.
Isolation of PBMCs
Blood samples (≈10 ml) from each subject were collected and then diluted 1:1 with phosphate buffered saline (PBS, pH 7.4). PBMCs were then isolated by density gradient centrifugation using Ficoll-Paque (Cedarlane, Toronto, Canada), then were washed twice in PBS, re-suspended at 2 × 106 PBMC/ml in RPMI 1640 medium (BioSera, London, UK) containing 10% heat-inactivated fetal calf serum (BioSera, London, UK) and assessed for viability by trypan blue exclusion.
Cell culture
To optimize vitamin D concentration, PBMCs of three SLE patients were cultured in the presence of 0 (control), 10, 30, 50, and 100 nM of 1,25 (OH)2 D3 (Sigma, St Louis, MO, USA). Then Treg cells were analyzed using flow cytometry (FACS Calibur, Becton Dickinson, San Jose, CA, USA). 50 nM of vitamin D was selected as optimum for further study (data not shown).
For the experiments, sets of 2 × 106 PBMC/patient were placed in sterile polystyrene plates and then 50 nM of either 1,25 (OH)2 D3 or medium without vitamin D was added. All cultures were incubated overnight (16 hours) at 37°C in a humidified atmosphere containing 5% CO2.
Flow cytometry analysis of Treg cells
Treg cell staining was performed using a Foxp3 staining kit (eBioscience, San Diego, CA, USA) according to the manufacturers’ instructions. For the analyses, the PBMCs were first labeled with anti-human CD4(FITC-conjugate) and anti-human CD25(PE-conjugate) (both antibodies from eBioscience, San Diego, CA, USA). For this, 1 × 106cells were first incubated with 10 µl of each antibody at 4°C in the dark for 30 minutes. After being washed in staining buffer, the cells were fixed in 0.5 ml of fixation buffer, permeabilized with 0.5 ml of permeabilization buffer, and then intracellular staining with anti-human Foxp3(PCH101, PE-Cy5-conjugate; eBioscience, San Diego, CA, USA) was performed for 30 minutes at 4°C in the dark. After this step, the samples were immediately analyzed in FACS Calibur system using CELLQuest software (Becton Dickinson). A minimum of 1 × 105cells for each sample were acquired and analyzed.
cDNA synthesis
Total RNA from 5 × 106 cells/patient was isolated using Tripure reagent (Roche, Mannheim, Germany), following the manufacturer's instructions. Then cDNA was synthesized using Reverse transcriptase enzyme (Fermentas, Vilnius, Lithuania). In brief, 1 μg of each extracted RNA sample was transferred to a sterile microtube and 1 µl random hexamer (0.5 µg/ µl) was added. The sample was then chilled on ice, and then 8 µl of reaction solution containing 4 µl of 5× buffer, 2 µl dNTPs (10 mM), 20 units RNase inhibitor, and 1.5 µl H20 was added. The tube was incubated at 25°C for 5 minutes and then 1 µl of reverse transcriptase enzyme (RT) (200 U/μl) was added. The tube was then incubated at 25°C for 10 minutes, 42°C for 60 minutes, and 70°C for 10 minutes.
Real-time-PCR analyses using TaqMan method
To design specific primers for Foxp3, TGFβ, and IL6 (as well as GAPDH housekeeping) genes, nucleotide sequences of these gene transcript exons were extracted from the database at http://www.ensembl.org and entered into Beacon Designer software (version 7.0, Premier Biosoft, Palo Alto, CA, USA). Appropriate primers and TaqMan Probes were designed and then blasted at NCBI, and the most appropriate primers selected for use in the real-time-PCR (RT-PCR). The primer and probe sequences used here are shown in , respectively.
Table 1. Primers and probes used in this study for RT-PCR analysis.
RT-PCR was then performed on the cDNA samples using the Taq EX Premix (Takara, Shiga, Japan) to measure Foxp3, TGFβ, IL6, and GAPDH gene expression levels. RT-PCR was performed in a Rotor-Gene 6000 thermal cycler (QIAGEN, Hilden, Germany). All data were analyzed using Rotor-Gene 6000 software and a comparative CT method as used to quantify gene expression levels. All data were expressed as mean transcript expression fold-change over untreated samples (controls), normalized to glyceraldehyde-3-phosphate dehydrogenize (GAPDH, internal control).
Statistics
Data are reported as mean ± SD. All statistical analyses were performed using IBM SPSS Statistics 21 (Chicago, IL, USA). Statistical differences between the groups were determined using a paired samples t-test. p<.05 was considered significant.
Results
Thirty SLE patients (12 new cases and 18 in remissions) with a mean age of 34.4 ± 9.3 years participated in this study (27 women and three men). Arthritis was the most common clinical disorder (76.66%), followed by nephritis (20%) and skin involvement (13.33%). Anti-dsDNA was present in 88.4% of the patients, and 94.7% of the patients were positive for anti-nuclear antibody (ANA) ().
Figure 1. Common laboratory findings and clinical disorders observed in SLE patients participated in this study.
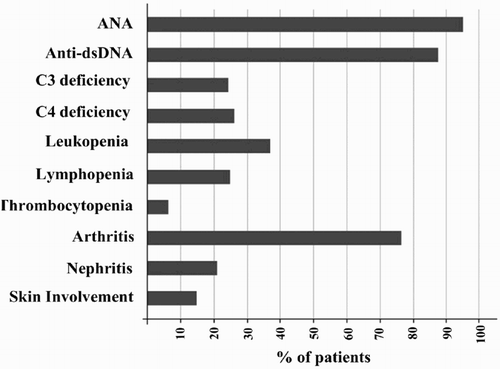
Flow cytometry assessment of Treg cell in the presence of vitamin D
Treatment of PBMCs of SLE patients with 50 nM of 1,25(OH)2D3 significantly increased the percentage of Treg cells (CD4+CD25highFoxp3+) (4.81 ± 2.41%) compared to untreated cells (3.49 ± 1.74%) (p = .006). A representative example of the flow cytometry analysis is shown in .
Expression of Foxp3, TGFβ, and IL6 in the presence of vitamin D
Quantitative RT-PCR analyses of the vitamin D-treated cells revealed that transcript expression of Foxp3 (1.41 ± 0.30), TGFβ (1.38 ± 0.24), and IL6 (0.50 ± 0.09) underwent fold-changes relative to the levels in untreated cells ().This translated to up-regulated expressions of Foxp3 (41%), TGFβ (38%) and down-regulated expressions of IL6 (50%) due to culture in the presence of 50 nM vitamin D.
Figure 3. RT-PCR analysis using comparative CT method to quantify gene expression levels of Foxp3, TGFβ, and IL6. Data expressed as mean transcript expression fold-change over untreated samples (controls) normalized to GAPDH. Quantitative RT-PCR analysis of the vitamin D-treated cells revealed that transcript expression of Foxp3 (1.41 ± 0.30), TGFβ (1.38 ± 0.24), and IL6 (0.50 ± 0.09) underwent fold-changes relative to the levels in untreated cells. This translated to up-regulated expressions of Foxp3 (41%), TGFβ (38%) and down-regulated expressions of IL6 (50%) due to culture in the presence of 50 nM vitamin D. Values are shown as mean ± SD.
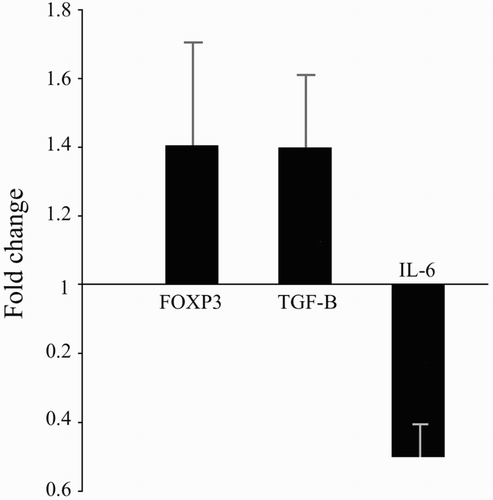
Comparison of results in new cases with SLE patients in remission
Several parameters were compared for new cases and SLE patients in remission. The first comparison showed that vitamin D treatment yielded a not statistically significant increase in Tregs in the remission group (5.18 ± 2.44%) compared to new cases (4.23 ± 2.34%). The second comparison showed that vitamin D down-regulated the expression of IL6 in the remission group (0.35 ± 0.05) more significantly than new cases (0.85 ± 0.12, p = .023).
The level of Foxp3 expression was 1.72 ± 0.36 in new case patients and 1.23 ± 0.26 in the remission group. This difference was significant (p = .038). The levels of TGFβ were also increased in new cases more than in the remission group (2.11 ± 0.21 vs. 1.04 ± 0.28). This difference was highly significant (p = .008) ().
Figure 4. Comparison of gene expression results between new cases and in remission SLE patients. Vitamin D treatment up-regulated the expression levels of Foxp3 (1.72 ± 0.36 vs. 1.23 ± 0.26) (p = .038), and TGFβ (2.11 ± 0.21 vs. 1.04 ± 0.28) (p = .008) in new cases more significantly compared to SLE patients who were in remission. Vitamin D treatment in SLE patients who were in remission down-regulated the expression of IL6 more significantly compared to new cases (0.35 ± 0.05 vs. 0.85 ± 0.12) (p = .023).
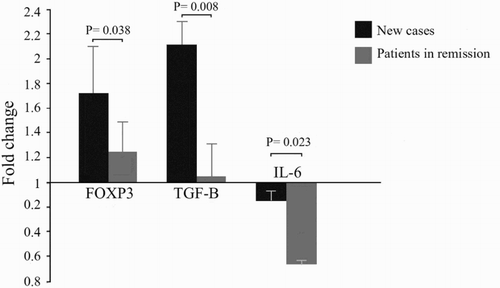
Discussion
Vitamin D as an environmental factor is highly implicated in the pathogenesis of SLE, and the exact immunomodulatory effect of vitamin D has been the subject of different investigations. The findings of our study showed that treatment of PBMCs of SLE patients with1,25(OH)2D3 increases the number of Treg cells and induces the expression of Foxp3 and TGFβ, while it diminishes the gene expression levels of IL6.
Treg cell dysfunctions are associated with the pathogenesis of lupus and various studies have shown that CD4+CD25highFoxp3+Treg cell development/function is impaired in patients with SLE (Alvarado-Sánchez et al., Citation2006; Khattri et al., Citation2003). Enhancement of Treg cells has beneficial effects in preventing lupus progression and we showed that vitamin D can exert some part of its immunomodulatory effects on lupus patients by increasing the number of these cells. Regulatory function of Treg cells depends on the expression of intracellular Foxp3 (Josefowicz, Lu, & Rudensky, Citation2012). Foxp3 is necessary for Treg cell development, maintenance, and function and controls the expression of a number of genes that mediate Tregs suppressive activity (Ramsdell, Citation2003). Loss of Foxp3 expression was correlated with reduced suppressive function of Treg cells in SLE patients (Suzuki et al., Citation2011). Our results demonstrated that, in addition to enhancing the number of CD4+CD25highFoxp3+Treg cells, vitamin D treatment increased the expression levels of Foxp3 and TGFβ, while it decreased the expression of IL6. These results are compatible with previously published studies (Jeffery et al., Citation2009; Kang, Kim, Lee, Kang, & Lee, Citation2010; Kang et al., Citation2012; Lavi Arab et al., Citation2014). Increased levels of TGFβ can positively affect the expression of Foxp3 (Mahon, Gordon, Cruz, Cosman, & Cantorna, Citation2003). The decreased expression of IL6 we found is consistent with other studies that showed that vitamin D can increase the number of CD4+CD25highFoxp3+Treg cells by reducing the co-factors that direct CD4+ cells into TH17 cells (Stockinger, Citation2007; Terrier et al., Citation2012).
We also observed that the effects of vitamin D on newly diagnosed SLE patients were different from its impact on patients who were in remission. After vitamin D treatment, in the remission group, the expression level of IL6 decreased in comparison to the newly diagnosed group. However, the expression levels of Foxp3 and TGFβ increased more in the newly diagnosed group than in the remission group. The exact mechanisms for differential effects of vitamin D on newly diagnosed SLE patients compared to those in remission proved to be elusive.
Treg cell generation is highly affected by cytokine milieu, and previously published studies showed that the increased expression of TGFβ in the absence or decreased presence of IL6 could shift T helper cell differentiation toward Treg cell generation (Ding-Lei, Zhi-Min, Min-Ning, Xia, & Ling-Yun, Citation2012; Kretschmer et al., Citation2005). IL6 is a critical cytokine in Treg cells generation (Ding-Lei et al., Citation2012). In addition, the presence of IL6 could enhance resistance to the regulatory effects of Treg cells. Thus vitamin D could help patients to overcome this resistance by decreasing the levels of IL6 (Chen et al., Citation2009). Petri, Bello, Fang, & Madger, Citation2013 showed that the stage of SLE could affect the suppressive efficiency of vitamin D, and our results showed that the blockade action of vitamin D on IL6 was more prominent in SLE patients who were in remission. A number of studies showed that IL6 could highly affect the generation of Tregs induced by TGFβ by blocking the expression of foxp3 (Wan, Xia, & Morel, Citation2007; Zheng, Wang, & Horwitz, Citation2008). The drugs used by SLE patients who are in remission could well affect the outcome of vitamin D treatment. Drug effects on the production of interleukin-6 have been documented (Wan et al., Citation2007; Zheng et al., Citation2008). However, presence of Treg cells is not sufficient to prevent disease progression in lupus patients. Activated effector cells become resistant to the suppressive ability of Treg cells as a result of exposure to IL6 which is highly over expressed in SLE patients. IL6 is a proinflammatory cytokine and stimulates inflammatory process in SLE and may have a role in tissue damage as well (Almerighi et al., Citation2009; Correale, Ysrraelit, & Gaitán, Citation2009; Esposito, Balletta, Procino, Postiglione, & Memoli, Citation2009). In addition to the effect on increasing Treg cell generation, decreased expression of IL6 by vitamin D could diminish inflammatory conditions in lupus patients. Regarding our results, it is hypothesized that synchronous presence of vitamin D and conventional drugs can exert more beneficial suppressive activity on SLE patients. Further studies are required to elucidate the differential role of vitamin D in different stages of SLE.
Conclusion
Treatment options of SLE are few, and most of them aim to suppress immune responses, inflammatory conditions, and reestablishment of self-tolerance. Our results show that by increasing the number and functional capacity of Treg cells and by decreasing the inflammatory conditions, the presence of vitamin D can have potential therapeutic benefits in the treatment of SLE patients. Our results show that the stage of disease could influence the suppressive effects of vitamin D in SLE patients.
Acknowledgements
The authors wish to thank the Vice President of the Research Council of Mashhad University of Medical Sciences (MUMS).
Disclosure statement
No potential conflict of interest was reported by the authors.
Funding
This study was supported by the Research Council of Mashhad University of Medical Sciences (MUMS) by grant number 88472.
Notes on contributors
Mohsen Ghoryani, MSc, Ph.D Student in Immunology, Mashhad University of Medical Sciences.
Maryam Sahebari, Assistant Professor in Rheumatology, MD in Mashhad University of Medical Sciences.
Mahmoud Mahmoudi, Professor in Immunology, MD, Ph.D in Mashhad University of Medical Sciences.
Nafise Abdollahi, Rheumatologist, MD in Mashhad University of Medical Sciences.
Hadi Reihani, MSc in Immunology, Mashhad University of Medical Sciences.
Shahrzad Zamani Taghizadeh Rabe, MSc in Immunology, Researcher, Mashhad University of Medical Sciences.
Nafise Tabasi, MSc in Immunology, Researcher in Mashhad University of Medical Sciences.
Maryam Rastin, Assistant Professor in Immunology, Ph.D, in Mashhad University of Medical Sciences.
References
- Almerighi, C., Sinistro, A., Cavazza, A., Ciaprini, C., Rocchi, G., & Bergamini, A. (2009). 1Alpha,25-dihydroxyvitamin D3 inhibits CD40L-induced pro-inflammatory and immunomodulatory activity in human monocytes. Cytokine, 45(3), 190–197. doi: 10.1016/j.cyto.2008.12.009
- Alvarado-Sánchez, B., Hernández-Castro, B., Portales-Pérez, D., Baranda, L., Layseca-Espinosa, E., & Abud-Mendoza, C. (2006). Regulatory T cells in patients with systemic lupus erythematosus. Journal of Autoimmunity, 27(2), 110–118. doi: 10.1016/j.jaut.2006.06.005
- Chen, W., Jin, W., Hardegen, N., Lei, K. J., Li, L., Marinos, N., … Wahl, S. M. (2003). Conversion of peripheral CD4+CD25− naive T cells to CD4+CD25+ regulatory T cells by TGF-β induction of transcription factor Foxp3. Journal of Experimental Medicine, 198, 1875–1886. doi: 10.1084/jem.20030152
- Chen, X., Das, R., Komorowski, R., Beres, A., Hessner, M. J., Mihara, M., & Drobyski, W. R. (2009). Blockade of interleukin-6 signaling augments regulatory T-cell reconstitution and attenuates the severity of graft-versus-host disease. Blood, 114, 891–900. doi: 10.1182/blood-2009-01-197178
- Chun, R. F., Liu, P. T., Modlin, R. L., Adams, J. S., & Hewison, M. (2014). Impact of vitamin D on immune function: Lessons learned from genome-wide analysis. Frontiers in Physiology, 5, 1–15. doi: 10.3389/fphys.2014.00151
- Correale, J., Ysrraelit, M. C., & Gaitán, M. I. (2009). Immunomodulatory effects of Vitamin D in multiple sclerosis. Brain, 132(5), 1146–1160. doi: 10.1093/brain/awp033
- Davis, L. S., Hutcheson, J., & Mohan, C. (2011). The role of cytokines in the pathogenesis and treatment of systemic lupus erythematosus. Journal of Interferon and Cytokine Research, 31(10), 781–789. doi: 10.1089/jir.2011.0047
- Ding-Lei, S., Zhi-Min, L., Min-Ning, S., Xia, L., & Ling-Yun, S. (2012). Roles of pro-and anti-inflammatory cytokines in the pathogenesis of SLE. Journal of Biomedicine and Biotechnology, 2012 , 1–15.
- Esposito, P., Balletta, M. M., Procino, A., Postiglione, L., & Memoli, B. (2009). Interleukin-6 release from peripheral mononuclear cells is associated to disease activity and treatment response in patients with lupus nephritis. Lupus, 18(14), 1329–1330. doi: 10.1177/0961203309106183
- Fontenot, J. D., Gavin, M. A., & Rudensky, A. Y. (2003). Foxp3 programs the development and function of CD4+CD25+ regulatory T cells. Nature Immunology, 4(4), 330–336. doi: 10.1038/ni904
- Hori, S., Nomura, T., & Sakaguchi, S. (2007). Control of regulatory T cell development by the transcription factor Foxp3. Science, 299(5609), 1057–1061. doi: 10.1126/science.1079490
- Huber, S., Schramm, C., Lehr, H. A., Mann, A., Schmitt, S., Becker, C., … Blessing, M. (2004). Cutting edge: TGF-β signalling is required for the in vivo expansion and immunosuppressive capacity of regulatory CD4+CD25+ T cells. Journal of Immunology, 173, 6526–6531. doi: 10.4049/jimmunol.173.11.6526
- Jeffery, L. E., Burke, F., Mura, M., Zheng, Y., Qureshi, O. S., Hewison, M., & Walker, L. S. (2009). 1,25-Dihydroxyvitamin D3 and IL-2 combine to inhibit T cell production of inflammatory cytokines and promote development of regulatory T cells expressing CTLA-4 and Foxp3. Journal of Immunology, 183(9), 5458–5467. doi: 10.4049/jimmunol.0803217
- Josefowicz, S. Z., Lu, L. F., & Rudensky, A. Y. (2012). Regulatory T cells: Mechanisms of differentiation and function. Annual Review of Immunology, 30, 531–564. doi: 10.1146/annurev.immunol.25.022106.141623
- Kang, I., Kim, S. H., Lee, W. W., Kang, S. W., & Lee, J. S. (2010). 1,25-dihyroxyvitamin D3 enhances the activity of the Foxp3 gene in human CD4+ T cells through directly binding to putative VDRE sites in the Foxp3 gene. Journal of Immunology, 1(2), 84–89.
- Kang, S. W., Kim, S. H., Lee, N., Lee, W. W., Hwang, K. A., & Shin, M. S. (2012). 1,25-Dihyroxyvitamin D3 promotes Foxp3 expression via binding to vitamin D response elements in its conserved non-coding sequence region. Journal of Immunology, 188(11), 5276–5282. doi: 10.4049/jimmunol.1101211
- Khattri, R., Cox, T., Yasayko, S. A., & Ramsdell, F. (2003). An essential role for Scurfin in CD4+CD25+ T regulatory cells. Nature Immunology, 4(4), 337–342. doi: 10.1038/ni909
- Kretschmer, K., Apostolou, I., Hawiger, D., Khazaie, K., Nussenzweig, M. C., & von Boehmer, H. (2005). Inducing and expanding regulatory T cell populations by foreign antigen. Nature Immunology, 6(12), 1219–1227. doi: 10.1038/ni1265
- Lane, N. E. (2010). Vitamin D and systemic lupus erythematosus: Bones, muscles, and joints. Current Rheumatology Reports, 12(4), 259–263. doi: 10.1007/s11926-010-0106-1
- Lavi Arab, F., Rastin, M., Faraji, F., Zamani Taghizadeh Rabe, S., Tabasi, N., Khazaee, M., … Mahmoudi, M. (2014). Assessment of 1,25-D3 effects on Treg cells and their related molecules in active chromatin lupus induced Balb/c mice. Immunopharmacology and Immunotoxicology. Advance online publication. doi:10.3109/08923973.2014.968255
- Lee, J. H., Wang, L. C., Lin, Y. T., Yang, Y. H., Lin, D. T., & Chiang, B. L. (2006). Inverse correlation between CD4+ regulatory T-cell population and autoantibody levels in paediatric patients with systemic lupus erythematosus. Immunology, 117(2), 280–286. doi: 10.1111/j.1365-2567.2005.02306.x
- Mahon, B. D., Gordon, S. A., Cruz, J., Cosman, F., & Cantorna, M. T. (2003). Cytokine profile in patients with multiple sclerosis following vitamin D supplementation. Journal of Neuroimmunology, 134(1–2), 128–132. doi: 10.1016/S0165-5728(02)00396-X
- Ohl, K., & Tenbrock, K. (2011). Inflammatory cytokines in systemic lupus erythematosus. Biomedicine and Biotechnology, 2011, 1–14. doi: 10.1155/2011/432595
- Okamoto, A., Fujio, K., Okamura, T., & Yamamoto, K. (2011). Regulatory T-cell-associated cytokines in systemic lupus erythematosus. Journal of Biomedicine and Biotechnology, 2011, 1–9. doi: 10.1155/2011/463412
- Petri, M., Bello, K. J., Fang, H., & Madger, L. S. (2013). Vitamin D in systemic lupus erythematosus: Modest association with disease activity and the urine protein-to-creatinine ratio. Arthritis & Rheumatology, 65(7), 1865–1871. doi: 10.1002/art.37953
- Ramsdell, F. (2003). Foxp3 and natural regulatory T cells: Key to a cell lineage? Immunity, 19, 165–168. doi: 10.1016/S1074-7613(03)00207-3
- Stockinger, B. (2007). Th17 cells: An orphan with influence. Immunology & Cell Biology, 85(2), 83–84. doi: 10.1038/sj.icb.7100035
- Suzuki, K., Setoyama, Y., Yoshimoto, K., Tsuzaka, K., Abe, T., & Takeuchi, T. (2011). Decreased mRNA expression of two FOXP3 isoforms in peripheral blood mononuclear cells from patients with rheumatoid arthritis and systemic lupus erythematosus. International Journal of Immunopathology and Pharmacology, 24, 7–14.
- Szodoray, P., Nakken, B., Gaal, J., Jonsson, R., Szegedi, A., & Zold, E. (2008). The complex role of vitamin D in autoimmune diseases. Scandinavian Journal of Immunology, 68(3), 261–269. doi: 10.1111/j.1365-3083.2008.02127.x
- Tackey, E., Lipsky, P. E., & Illei, G. G. (2004). Rationale for interleukin-6 blockade in systemic lupus erythematosus. Lupus, 13(5), 339–343. doi: 10.1191/0961203304lu1023oa
- Terrier, B., Derian, N., Schoindre, Y., Chaara, W., Geri, G., & Zahr, N. (2012). Restoration of regulatory and effector T cell balance and B cell homeostasis in systemic lupus erythematosus patients through vitamin D supplementation. Arthritis Research & Therapy, 14(5), 1–10. doi: 10.1186/ar4060
- Veldhoen, M., Hocking, R. J., Atkins, C. J., Locksley, R. M., & Stockinger, B. (2006). TGF beta in the context of an inflammatory cytokine milieu supports de novo differentiation of IL 17-producing T cells. Immunity, 24, 179–189. doi: 10.1016/j.immuni.2006.01.001
- Wan, S., Xia, C., & Morel, L. (2007). IL-6 produced by dendritic cells from lupus-prone mice inhibits CD4+CD25+ T cell regulatory functions. Journal of Immunology, 178, 271–279. doi: 10.4049/jimmunol.178.1.271
- Xing, Q., Su, H., Cui, J., & Wang, B. (2012). Role of Treg cells and TGF-β1 in patients with systemic lupus erythematosus: A possible relation with lupus nephritis. Immunological Investigations, 41(1), 15–27. doi: 10.3109/08820139.2011.578189
- Zheng, S. G., Wang, J., & Horwitz, D. A. (2008). Cutting edge: Foxp3+CD4+CD25+ regulatory T cells induced by IL-2 and TGF-β are resistant to Th17 conversion by IL-6. Journal of Immunology, 180, 7112–7116. doi: 10.4049/jimmunol.180.11.7112