ABSTRACT
Celiac disease is one of the most prevalent food intolerances worldwide, with a gluten-free diet as the only effective therapy. Therefore, searching for new alternative approaches is a priority, and one of them is the idea of using proteinases for degradation of gluten proteins. The aim of the study was a trial of enzymatic cleavage of prolamins extracted from wheat, barley, rye, triticale and oat. The proteolysis was performed by microbial proteases from Bacillus stearothermophilus, Bacillus licheniformis, Bacillus thermoproteolyticus and Streptomyces griseus. The reaction was performed up to 60 min, stopped by addition of appropriate synthetic inhibitor and products of limited proteolysis were analyzed by SDS-PAGE. Most of the prolamins were susceptible to proteolysis by the examined microbial enzymes and as the result of hydrolysis the molecules of lower molecular weight and peptides occurred (<30,000 Da). The immunoreactivity of proteolysis products was evaluated using the reaction with polyclonal and monoclonal antibodies by Western blot and ELISA analysis, respectively. Immunochemical analyses confirmed that celiac-active sequences of amino acids in prolamins were at least partially destroyed and have lost their “toxicity”, so application of microbial proteases seems to be an alternative method for decreasing of celiac activity of prolamins.
Introduction
Cereals, most importantly wheat, play an essential role in determining both nutritional and functional properties of cereal-derived foods. Generally, based on different solubilities, cereal proteins can be classified into water/salt-soluble albumins and globulins, alcohol-soluble prolamins and high-molecular-weight glutelins soluble in diluted acid/base solutions (Mamone, Picariello, Addeo, & Ferranti, Citation2011). Prolamins and glutelins, the storage fractions of the endosperm of cereal grains, represent the most abundant proteins accounting approximately up to 90% in wheat (Cavazos & de Mejia, Citation2013). The prolamins are monomeric polypeptides with molecular weights between 28 and 50,000 Da (van Eckert et al., Citation2010; Vaccino, Becker, Brandolini, Salamini, & Kilian, Citation2009; Wieser, Citation2007). They are rich in glutamine (35%) and proline (15%), which makes them highly resistant to proteolytic degradation within the gastrointestinal tract (Gregorini, Colomba, Ellis, & Cicltira, Citation2009). As a result, many long peptides (>20-mers) persist during digestion in the small intestinal lumen and are only partially hydrolyzed by brush-border membrane exopeptidases. These peptides (especially α2-gliadin fragment of 33 amino acids LQLQPFPQPQLPYPQPQLPYPQPQLPYPQPQPF) are deamidated by transglutaminase 2 (TG2), resulting in Th1 cell proliferation in the gut mucosa and causing the development of celiac disease (Shan et al., Citation2002; Siegel et al., Citation2006). Thus, eliminating the amount of Pro- and Gln-rich immunogenic peptides in the small intestine can have a significant therapeutic potential.
Currently the only and effective treatment for celiac disease is a strict life-long gluten-free diet, which forbids any foods containing wheat prolamins and corresponding proteins from rye, barley and prophylactically oat, which safety for celiac patients is still questionable (Londono et al., Citation2013; Zingorne, Capone, & Cicacci, Citation2010). Alternative methods for decreasing celiac activity of prolamins are under investigation (see review Makharia, Citation2014), from which protease therapy has been proposed as one of the future therapeutic options to reduce or even eliminate the celiac-active properties of prolamins. Bacterial and fungal proline peptidases are very useful for degradation of celiac activity of wheat gliadins, eg prolyl-endopeptidase (PEP) from Flavobacterium meningosepticum and Myxococcus xanthus (Shan, Marti, Sollid, Gray, & Khosla, Citation2004; Stepniak et al., Citation2006) or PEP from Aspergillus niger and Aspergillus oryzae (Ehren et al., Citation2009). Unfortunately, most PEPs are irreversibly inactivated by gastric enzymes and acidic pH, so they are unable to cleave Pro-rich peptides, such as the 33-mer from α-gliadin, in vivo. Although PEP activity could simplify gluten structure and texture, it would need protection, for example encapsulation (Solid & Khosla, Citation2005). Despite the fact that mentioned endopeptidases are degraded inside the digestive tract, they can be useful during food processing and, therefore, it is desirable to search for other sources of such microbial enzymes. Another approach exploited degradation of wheat gliadins with naturally evolved glutenases (Stenman, Linfors, et al., Citation2010; Stenman, Venäläinen, et al., Citation2009) from germinating cereals evolutionarily selected for their cleavage of Glu-residues, which are resistant to digestion with pepsin and trypsin, and active over a large pH range.
Peptidase systems of selected lactic acid bacteria strains (eg Lactobacillus alimentarius, Lactobacillus brevis, Lactobacillus sanfranciscensis, Lactobacillus hilgardii) known for their partial hydrolysis of prolamin proteins contained in wheat and rye flours were also studied (Di Cagno et al., Citation2002; Rizello, Curiel, et al., Citation2014; Rizello, De Angelis, et al., Citation2007). It was shown that wheat and rye breads or pasta with durum flours treated using selected sourdough lactic acid bacteria markedly decreased the celiac activity of wheat/rye prolamins (Di Cagno et al., Citation2004). Unfortunately, in the last decades, cereal baked goods are manufactured by processes using chemical leavening agents in combination with baker yeasts instead of traditional technology exploited sourdough fermentations using proteolytic lactic acid bacteria. Under these conditions, cereal proteins are not degraded (or only partially) and can lead to the development of celiac disease (Greco et al., Citation2011). Therefore, it is worthwhile to evaluate the effect of sourdough lactic acid bacteria with appropriate PEPs to hydrolyze celiac-active sequences in cereal proteins.
Additionally, other specific endopeptidases with cleavage activity for Pro-residues of different substrates have been reported, eg endopeptidase from Lactobacillus helveticus CNRZ32 (Chen, Christensen, Broadbent, & Steele, Citation2003), metalloendoproteinase from Penicillium citricum (Doi et al., Citation2004) and prolyl-endoprotease from A. niger (Stepniak et al., Citation2006). The latter one is a promising tool for celiac disease therapy due to the optimal pH activity in the range 4–5 with strong stability at pH 2 and complete resistance to digestion with pepsin (Gobetti, Rizello, Di Cagno, & De Angelis, Citation2007).
As presented above, the idea of using proteases for degradation of gluten protein nowadays is explored and different sources of proteases and their possible applications are tested. Based on our preliminary study on wheat gliadin proteolysis by microbial enzymes (Socha, Mickowska, Urminská, & Kačmárová, Citation2015), we examined proteases from Bacillus and Streptomyces for hydrolysis of different cereal prolamins (wheat, barley, rye, triticale and oat). The degree of proteolysis was evaluated using reaction with polyclonal and monoclonal antibodies by Western blot and ELISA analysis, respectively. Using this approach we were able to assess the potential capability of examined enzymes to decrease (at least partially) celiac activity of prolamins.
Materials and methods
Chemicals and biological material
Bacterial proteases from Bacillus stearothermophilus, Bacillus thermoproteolyticus and Streptomyces griseus were purchased from Fluka (Switzerland); Bacillus licheniformis protease was from Sigma-Aldrich (St. Louis, MO, USA). All commercially available enzymes were selected for their proteolytic activity to cleave peptide bonds in various substrates. Specific conditions for each enzyme were determined according to product information provided by the manufacturer and partially modified by on-line enzyme database BRENDA (http://www.brenda-enzymes.org/).
Sample collections of selected varieties of wheat, rye, triticale, barley and oat grains (common wheat, cultivar Saxana, spring form; common wheat, cultivar Blava, winter form; durum wheat, cultivar Soldur; spelt wheat, cultivar Rubiota; rye, cultivar Dankowskie Nowe; triticale, cultivar Wanad; barley, cultivar Ludan; oat, cultivar Detvan) were from gene bank of seed's species Slovak Agricultural Research Centre, Research Institute of Plant Production, Piestany (Slovakia).
Polyclonal anti-wheat gluten antibody was from US Biological (USA), anti-rabbit antibody conjugated with horseradish peroxidase was from BD Pharmingen (USA), ELISA kits were RIDASCREEN® Gliadin (R-Biopharm, Germany) and AgraQuant®Gluten (Romer Labs UK Ltd., England). All other chemicals were analytical grade and were from Sigma-Aldrich (St. Louis, MO, USA) or Applichem (Darmstadt, Germany).
Extraction of proteins
Cereal proteins were extracted according to Osborne's (Citation1924) procedure with modifications. Grains were milled and the proteins were extracted by magnetic stirring with 15 ml of solvent/g meal for 1 hour at room temperature. Extraction was carried out with the following series of solvents: 0.5 mol/l NaCl to extract salt-soluble albumins and globulins (twice), distilled water to remove NaCl, and 70% (v/v) ethanol to extract prolamins and alcohol-soluble proteins. The extracted alcohol-soluble proteins were lyophilized and used for further analyses. The protein content in extracts was calculated on the basis of amino acid analysis performed according to the method of Moore and Stein (Smith, Citation2003) using an AAA400 chromatographic amino acid analyzer (Ingos, Czech Republic).
Proteolysis
Lyophilized prolamins (1.5 mg) were dissolved in 150 µl 0.1 mol/l Tris-HCl with pH optimal for each enzyme. Enzymes were prepared in a concentration of 0.5 mg/ml in 50 mmol/l acetate buffer (pH 5.5). Proteolysis was performed in enzyme–prolamins ratio 1:250 for B. licheniformis protease and 1:200 for other proteases, in temperature conditions appropriate for each enzyme. In case of metallopeptidase from B. thermoproteolyticus and S. griseus proteases mixture, coenzyme Zn2+ in the form of zinc acetate was added to the reaction mixture. Proteolysis was stopped after 15, 30 and 60 min with suitable synthetic inhibitor. Proteolysis conditions are listed in .
Table 1. Summary of proteolytic conditions.
Electrophoresis
Aliquots of 10 µl (approx. 100 µg of proteins/lane) of extracted prolamins and proteolysis products were analyzed by Tris-Tricine SDS-PAGE electrophoresis under reducing conditions according to the Schägger–von Jagow method (Schägger & von Jagow, Citation1987).
Immunochemical analyses
After SDS-PAGE, proteins were electrotransferred onto PVDF membranes ImmobilonPSQ under conditions of 1.5 hour at 170 mA using 10 mmol/l CAPS with 10% methanol, pH 11 transfer buffer, according to the protocol of the manufacturer (Millipore, Billerica, USA). Membranes were blocked in 1% BSA in TBS buffer, pH 7.6, overnight and washed with TBS buffer containing 0.1% Tween 20 4-times/15 min. Incubation with the anti-gluten wheat antibody solution (dilution 1:1000 in blocking solution) was carried out for 1.5 hour, followed by washing with TBS buffer containing 0.1% Tween 20 5-times/15 min. After incubation with the primary antibody, membranes were subjected to incubation with anti-rabbit horseradish peroxidase-conjugated antibody (dilution 1:2,000 in blocking solution) for 1 hour, followed by washing with TBS buffer containing 0.1% Tween 20 6-times/15 min. Finally, blots were developed by chromogenic detection using diaminobenzidine (Sigma, St. Louis, MO, USA).
For sandwich ELISA analysis, a commercial kit RIDASCREEN® Gliadin based on the monoclonal antibody R5 and AgraQuant®Gluten based on the monoclonal antibody G12 were used according to the instructions of the manufacturers (R-Biopharm, Germany and Romer Labs UK Ltd., England, respectively).
Results
Electrophoretic analysis of proteolysis products
The main fractions of wheat prolamins (gliadins) have molecular weights of range 30–55,000. Rye prolamins (secalins) comprised two main fractions of approximately 35 and 66,000. The electrophoretic profile of triticale prolamins consists of fractions characteristic for wheat (35–55,000) as well as for rye (66,000). Molecular weights of barley prolamins (hordeins) were similar to gliadins (35–45,000) and only oat prolamins (avenins) are formed from proteins of molecular weight below 30,000. Generally, even during shortest proteolysis time (15 min) (data not shown) all prolamin fractions were at least partially degraded and low-molecular fragments of proteins (<25,000) appeared.
All prolamins were susceptible to proteolysis by subtilisin from B. licheniformis and most of them were significantly or almost completely hydrolyzed, resulting in small protein fragments with molecular weight <15,000. Only the winter form of common wheat and barley showed a moderate degree of proteolysis ((a)). Serine peptidase from B. stearothermophilus activity to wheat, rye, triticale and barley prolamins resulted in substantial cleavage into protein fragments with molecular weights below 35,000 while oat prolamins were only slightly digested (main protein fractions of 20–30,000 presented on the electrophoregram) ((a)). All cereal prolamins have been almost totally degraded to low-molecular products below 15,000 by cleavage of B. thermoproteolyticus peptidase. Only oat prolamins were quite resistant to proteolysis and the rest of the main fractions of approximately 20–30,000 were still observed ((a)). S. griseus protease behaved as the most active enzyme, as it hydrolyzed very effectively prolamins of all examined cereals ((a)).
Figure 1. Proteolysis of prolamins detected by SDS-PAGE (a) and Western blot (b) after 60 min of hydrolysis using protease from Bacillus licheniformis: CW1 – common wheat, cultivar Saxana, spring form; CW2 – common wheat, cultivar Blava, winter form; DW – durum wheat, cultivar Soldur; SW – spelt wheat, cultivar Rubiota; R – rye, cultivar Dankowskie Nowe; T – triticale, cultivar Wanad; B – barley, cultivar Ludan; O – oat, cultivar Detvan; lane 1 – prolamins treated using protease; lane 2 – untreated prolamins; M – molecular marker; arrows indicate fragments after proteolysis.
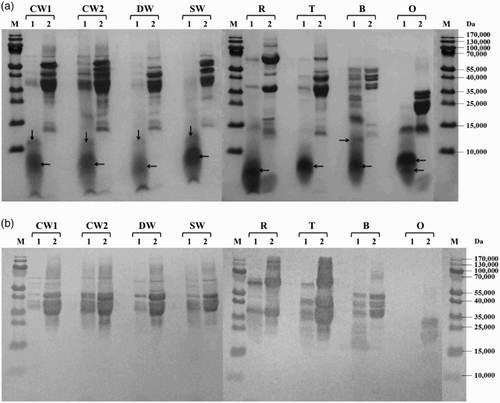
Figure 2. Proteolysis of prolamins detected by SDS-PAGE (a) and Western blot (b) after 60 min of hydrolysis using protease from B. stearothermophilus: CW1 – common wheat, cultivar Saxana, spring form; CW2 – common wheat, cultivar Blava, winter form; DW – durum wheat, cultivar Soldur; SW – spelt wheat, cultivar Rubiota; R – rye, cultivar Dankowskie Nowe; T – triticale, cultivar Wanad; B – barley, cultivar Ludan; O – oat, cultivar Detvan; lane 1 – prolamins treated using protease; lane 2 – untreated prolamins; M – molecular marker; arrows indicate fragments after proteolysis.
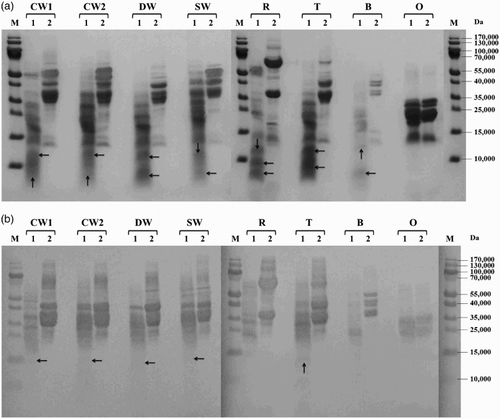
Figure 3. Proteolysis of prolamins detected by SDS-PAGE (a) and Western blot (b) after 60 min of hydrolysis using protease from Bacillus thermoproteolyticus: CW1 – common wheat, cultivar Saxana, spring form; CW2 – common wheat, cultivar Blava, winter form; DW – durum wheat, cultivar Soldur; SW – spelt wheat, cultivar Rubiota; R – rye, cultivar Dankowskie Nowe; T – triticale, cultivar Wanad; B – barley, cultivar Ludan; O – oat, cultivar Detvan; lane 1 – prolamins treated using protease; lane 2 – untreated prolamins; M – molecular marker; arrows indicate fragments after proteolysis.
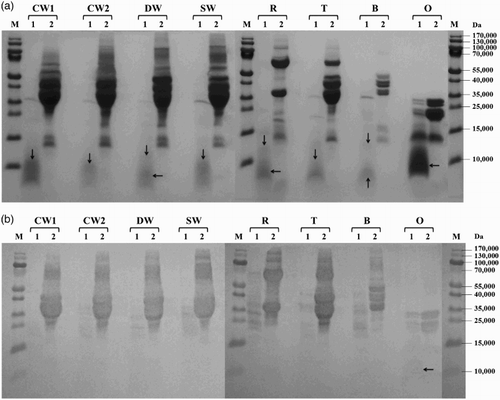
Figure 4. Proteolysis of prolamins detected by SDS-PAGE (a) and Western blot (b) after 60 min of hydrolysis using protease from Streptomyces griseus: CW1 – common wheat, cultivar Saxana, spring form; CW2 – common wheat, cultivar Blava, winter form; DW – durum wheat, cultivar Soldur; SW – spelt wheat, cultivar Rubiota; R – rye, cultivar Dankowskie Nowe; T – triticale, cultivar Wanad; B – barley, cultivar Ludan; O – oat, cultivar Detvan; lane 1 – prolamins treated using protease; lane 2 – untreated prolamins; M – molecular marker; arrows indicate fragments after proteolysis.
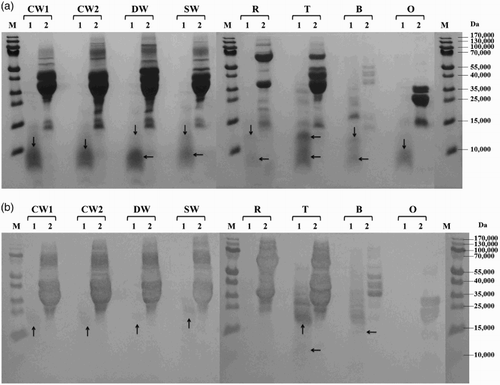
Immunochemical analysis of proteolysis products
Immunological features of cereal prolamins from SDS-PAGE gels were firstly investigated by reaction with polyclonal anti-wheat gluten antibody using Western blotting. However, results from Western blotting comprise only qualitative characteristics, so we also employed quantitative ELISA analysis with R5 and G12 monoclonal antibodies.
Western blot analysis showed that new small protein fragments appearing after proteolysis (molecular weight <15,000) were generally not recognized by the antibody. In case of B. stearothermophilus and S. griseus proteases, slight reaction with wheat and triticale protein fragments of 15–20,000 was observed ((b) and 4(b)). The rest of the un-cleaved wheat prolamin fractions (35–55,000) were reliably recognized after proteolysis with all tested microbial enzymes. Proteolysis using S. griseus protease resulted in almost complete disappearance of rye immunoreactive proteins while barley and triticale fragments with molecular weights ranging from 15 to 35,000 revealed a slight reaction ((b)). Hydrolyzed oat prolamins showed the highest variability among all cereal proteins. Anti-gluten polyclonal antibody recognized not only un-cleaved avenins, but also a slight band of approximately 12,000 after hydrolysis using B. thermoproteolyticus protease ((b)) and only typical un-cleaved fractions of approximately 20–35,000 after B. stearothermophilus peptidase proteolysis ((b)). Neither avenins nor their cleavage products were detected by immunoblotting after B. licheniformis ((b)) and S. griseus ((b)) protease digestion.
The quantification of immunoreactivity of prolamins and proteolysis products was performed by sandwich ELISA method based on the monoclonal R5 and G12 antibodies. R5 antibody was developed against secalin epitopes and G12 antibody is raised against 33-mer from α2-gliadin; so applying two antibodies with different specificity allows to get complementary results. ELISA measurements were done before proteolysis on native prolamins and after proteolytic reaction had been stopped. The relative residual immunochemical reactivity of cleaved prolamins detected by R5 antibody is summarized in . Barley prolamins were the most resistant to proteolytic degradation; 22–80% of celiac-active epitopes still existed after proteolysis. This phenomenon occurred possibly due to poor solubility of barley prolamins; therefore, proteases were less effective in digestion compared to other cereals. Prolamins from different wheat species (except durum wheat) contained substantial amounts of celiac-active sequences in their native state while the lowest residual activity with R5 antibody was observed after S. griseus and B. thermoproteolyticus protease treatment of common wheat samples. In case of rye and triticale prolamin hydrolysis, both proteinases mentioned above acted very effectively as well, only 0.34%–2.64% residual activity was observed in comparison to 4.48%–9.69% and 5.05%–6.55% residual activity after B. licheniformis and B. stearothermophilus protease treatment, respectively. Oat avenins is believed to be nonreactive with R5 antibody due to the different epitopes presented, although cross-reactivity is possible. In our experiment celiac-active epitopes of oat were detected at a very low level, also in their native state (). Regarding S. griseus protease the quantity of avenin proteolysis products was even below the limit of detection ( and ).
Figure 5. Proteolysis of prolamins detected by R5 ELISA after 60 min of hydrolysis using different microbial proteases; *ppm values lower than 20 mg/kg (Codex Alimentarius limit); †inconclusive results; ‡<LOD (limit of detection).
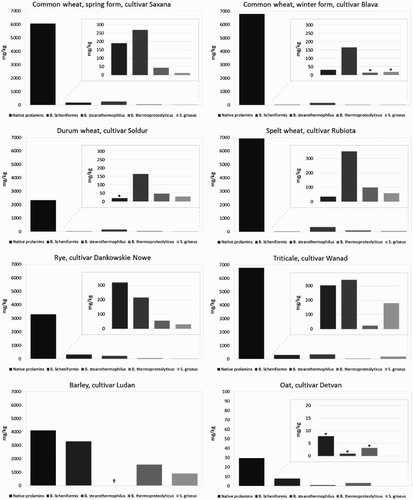
Table 2. Relative residual immunoreactivity of extracted prolamins after proteolysis (R5 ELISA).
Relative residual immunochemical reactivity of cleaved prolamins detected by G12 antibody is summarized in . Generally, G12 antibody detected higher residual immunochemical activity compared to R5 antibody but from the point of view of proteolytic activity of used proteinases the obtained results are compatible. Immunochemical analysis based on G12 as well as R5 antibodies revealed that protease from S. griseus acted the most effectively from examined enzymes, resulting in the most significant reduction of amounts of celiac-active epitopes. Similarly, the lowest proteolytic activity of proteinase from B. stearothermophilus was confirmed (also corresponding with SDS-PAGE and Western blot results).
Table 3. Relative residual immunoreactivity of extracted prolamins after proteolysis (G12 ELISA).
Discussion
The enzymatic degradation of gluten proteins can be possibly applied not only in the therapy of celiac patients, but also in technological processing of raw materials to decrease gluten “toxicity”. Therefore, different sources of proteases with such an activity are currently explored. The aim of the work was to examine the susceptibility of prolamins to cleavage by proteolytic enzymes of microbial origin. The activity of proteases from Bacillus and Streptomyces was investigated on wheat, rye, triticale and barley grains as these cereals contain main prolamin fractions with celiac-active sequences of amino acids. Additionally, oat was included although gliadin-like epitopes do not occur in oat avenins, but other potentially harmful epitopes are quite common (Ballabio et al., Citation2011; Londono et al., Citation2013).
The proteolytic reactions were performed on extracted prolamins dissolved in solutions to mimic conditions optimal for enzymes, although prolamin concentrations were not comparable with natural prolamin content in cereals and the yield of the extraction process can vary among samples. Serine proteases from B. licheniformis and B. stearothermophilus are called subtilisins, and are nonspecific peptidases with broad affinity for a large uncharged residue in P1 (P1 is amino acid residue in a substrate undergoing cleavage in the N-terminal direction from the cleaved peptide bond, and the residue in C-terminal direction is designated P1′). Metallopeptidase from B. thermoproteolyticus (thermolysin) has a high specificity for cleavage of peptide bonds on the N-terminal side of hydrophobic amino acid residues, and hydrolyses between residues P1 and P1′, where P1′ is the residue derived from hydrophobic amino acids: Ala, Phe, Leu, Ile, Val or Met. Protease form Streptomyces griseus (called pronase E or actinase E) is a mixture of several proteases: five serine-type proteases, two zinc endopeptidases, two zinc-leucine aminopeptidases and one zinc carboxypeptidase, so it has a very wide range of substrate specificity and was very effective in digestion of proteins. Although cereal prolamins are rich in Pro and Gln amino acids and used enzymes do not exhibit specific activity for these residues, the broad specificity for cleavage of peptide bonds makes them potentially effective tools to degrade complex cereal proteins.
Bacterial prolyl-endopeptidases with the capability to eliminate Pro-rich peptides of wheat gliadins have been extensively investigated (Ehren et al., Citation2009; Shan et al., Citation2004; Stepniak et al., Citation2006). These enzymes include peptidase from F. meningosepticum with preference for Pro–Gln bonds; peptidase from Sphingomonas capsulate cleaved both Pro–Gln and Pro–Tyr bonds with comparable efficiency; and peptidase from Myxococcus xanthus with modest preference for Pro–(Tyr/Phe) sites over Pro–Gln sites (Shan et al., Citation2004). The microbial enzymes isolated from Bacillus and Streptomyces investigated here showed powerful proteolytic activity under basic conditions. Moreover, all proteases of genus Bacillus used exhibit their activity at high temperature (see “Materials and Methods”). Although conditions of such proteolysis do not allow their direct application to celiac patients' therapy, they do not eliminate the possibility of using them in technology of raw materials and food processing after detailed research.
Evaluation of celiac activity of cereal prolamins and their proteolysis products was performed by two immunochemical methods, Western blot and ELISA, involving polyclonal and monoclonal antibodies, respectively. Generally, anti-wheat antibodies (either polyclonal or monoclonal) recognize not only wheat gliadins but also barley hordeins, rye secalins and triticale prolamins. In comparison to polyclonal antibodies, very narrow specificity of monoclonal antibodies only against given epitopes eliminates the cross-reactivity. Western blot analysis was performed with polyclonal antibody against wheat gluten and obtained results showed that prolamins cleaved by tested microbial enzymes to small peptides (<15,000) were generally not recognized by the antibody. The ELISA based on monoclonal antibody R5 was developed against secalin extract with capability to recognize celiac-active epitopes (QQPFP, QQQFP, LQPFP and QLPFP) in wheat, rye and barley prolamins (van Eckert et al., Citation2010; Thompson & Méndez, Citation2008). Triticale is a cross-breed between wheat and rye containing celiac-active epitopes which can also be recognized by the R5 antibody. Despite the fact that R5 antibody is nonspecific against epitopes of oat prolamins, Ellis, Rosen-Bronson, O'Reilly, and Ciclitra (Citation1998) reported that QQQPF amino acid sequence in avenins comprises cross-reactivity and thus false-positive results. After proteolysis performed by all tested microbial proteases, the recognition of digested oat avenins did not differ significantly either with anti-wheat polyclonal or R5 monoclonal antibody.
Detection of highly immunogenic epitopes of α2-gliadin 33-mer was performed by ELISA based on the G12 antibody. The G12 antibody specifically recognizes the sequence QPQLPY located in repetitions in that immunogenic peptide. The G12 antibody detects also similar peptides of rye (QPQQPY), barley (QPQLPF) and oat (Hammer, Citation2012). In our experiment, ELISA analysis based on the G12 antibody gives information on quantities of undigested 33-mer or its residues containing these immunogenic epitopes, eg shorter peptides containing sequence QPQLPY. Results indicated that QPQLPY peptides from wheat and similar peptides from other cereals were more resistant to proteolysis of tested microbial enzymes than epitopes recognized by the R5 antibody ( and ). The G12 antibody is capable of detecting specifically potential immunogenic peptides of oats as well, in contrast to rather nonspecific cross-reactivity of the R5 antibody with oats avenins. Also in our study, G12 ELISA delivered more reliable results for the oat sample than ELISA based on the R5 antibody – the presence of not cleaved avenins after treatment with B. stearothermophilus and B. thermoproteolyticus was confirmed by detection with the G12 antibody (un-cleaved avenins bands are visible on corresponding SDS-PAGE gel and Western blot membrane).
Quantitative ELISA analyses based on R5 and G12 antibodies confirmed Western blot results, that at least substantial part or the majority of celiac activity of prolamins was destroyed after proteolysis, even in samples containing a huge amount of typical celiac-active sequences like wheat or triticale prolamins (). Prolamins were cleaved into fragments less than 300 amino acids long (molecular weight <35,000) which probably lacked celiac-active epitopes, as the residual reactivity was very low. Exceptionally, barley hordeins after proteolysis using protease from B. stearothermophilus resulted in measurements impossible to interpret () probably due to the nonspecific reaction with the antibody (measurement signal independent of sample dilution). Generally, barley hordeins showed very poor solubility in buffers possibly due to the simultaneous extraction of various hydrophobic substances, not only protein/peptide fragments that can affect analytical data. It should be noted that native prolamins (used as control) and prolamins appointed to protease treatment were dissolved in the same buffers under specific conditions appropriate for each enzyme as referred in . Moreover, incomplete solubility of barley hordeins (35–45,000) could be the reason of worsened electrophoretic profile ((a)–4(a)), indicating only 5–6 main fragments with less visibility on coomassie-stained gels. Amino acid composition of hordeins showed higher content of proline (approx. 24%–27%) compared to wheat gliadins (approx. 17%–18%) (Mickowska, Socha, Urminská, & Cieślik, Citation2012) probably causing lower degree of proteolysis, especially in the case of B. licheniformis protease treatment ((a)). Immunochemical analyses showed that approximately 22%–80% celiac-active sequences were still detected by R5 ELISA and 18%–34% by G12 ELISA ( and ) and hordein fragments of 20–45,000 effectively reacted with the polyclonal antibody ((a)). It should be worth mentioning that sandwich R5 ELISA is criticized of overestimation of barley hordeins (Kanerva, Sontag-Strohm, Ryöppy, Alho-Lehto, & Salovaara, Citation2006).
Immunoreactivity of oat prolamins was very low in the sample of native avenins while after proteolysis it was additionally reduced. However, the reaction with monoclonal antibody confirmed oat safety in a gluten-free diet; several studies (Ballabio et al., Citation2011; Halbmayr-Jech et al., Citation2012) proved that content of celiac-active sequences depends strongly on oat variety. Unfortunately, the majority of oat raw material and products are contaminated by wheat, barley and rye, which was proved by the PCR method (Størsrud, Yman, & Lenner, Citation2003). The oat variety tested here was obtained from the gene bank of seed species, which means that possible contamination by other cereals can be excluded. In consequence, even reduced immunoreactivity of oat does not guarantee safety for celiac patients unless carefully tested and oat products prepared in a special way.
Conclusions
Proteolytic cleavage of cereal prolamins using microbial proteases from Bacillus and Streptomyces showed good susceptibility of these proteins to proteolysis. Immunochemical analyses after protease treatment of examined prolamins confirmed the efficiency of the digestion process. Quantitative analysis revealed that the amount of corresponding products was on a low level compared to prolamins in their native state. All enzymes used in this study belong to the broad-specificity proteases, which make them potentially effective tools for decreasing (at least partially) celiac activity of the examined prolamins. Commercially available protease from S. griseus is a mixture of several proteases with different specificity, and therefore acted the most effectively among the tested enzymes. Although prolamins were susceptible to degradation by nonspecific proteases, further analyses are still needed to assess the more detailed structural and biochemical features of proteolysis products.
Acknowledgments
The authors thank Juraj Missik for technical assistance.
Disclosure statement
No potential conflict of interest was reported by the authors.
Additional information
Funding
References
- Ballabio, C., Uberti, F., Manferdelli, S., Vacca, E., Boggini, G., Redaelli, R., … Restani, P. (2011). Molecular characterisation of 36 oat varieties and in vitro assessment of their suitability for coeliacs’ diet. Journal of Cereal Science, 54, 110–115. doi: 10.1016/j.jcs.2011.04.004
- Cavazos, A., & de Mejia, E. G. (2013). Identification of bioactive peptides from cereal storage proteins and their potential role in prevention of chronic diseases. Comprehensive Reviews in Food Sciences and Food Safety, 12, 364–380. doi: 10.1111/1541-4337.12017
- Chen, Y.-S., Christensen, J. E., Broadbent, J. R., & Steele, J. L. (2003). Identification and characterization of Lactobacillus helveticus PepO2, an endopeptidase with post-proline specificity. Applied and Environmental Microbiology, 69, 1276–1282. doi: 10.1128/AEM.69.2.1276-1282.2003
- Di Cagno, R., De Angelis, M., Auricchio, S., Greco, L., Clarke, C., De Vincenzi, M., … Gobetti, M. (2004). Sourdough bread made from wheat and nontoxic flours and started with selected lactobacilli is tolerated in celiac sprue patients. Applied and Environmental Microbiology, 70, 1088–1096. doi: 10.1128/AEM.70.2.1088-1096.2004
- Di Cagno, R., De Angelis, M., Lavermicocca, P., De Vincenzi, M., Giovannini, C., Faccia, M., & Gobetti, M. (2002). Proteolysis by sourdough lactic acid bacteria: Effects on wheat flour protein fractions and gliadin peptides involved in human cereal intolerance. Applied and Environmental Microbiology, 68, 623–633. doi: 10.1128/AEM.68.2.623-633.2002
- Doi, Y., Akiyama, H., Yamada, Y., Ee, C. H. E., Lee, B. R., Ikeguchi, M., & Ichishima, E. (2004). Thermal stabilization of penicillolysin, a thermo-labile 19 kDa Zn2+-protease, obtained by site-directed mutagenesis. Protein Engineering Design and Selection, 17, 261–266. doi: 10.1093/protein/gzh034
- van Eckert, R., Bond, J., Rawson, P., Klein, C. L., Stern, M., & Jordan, T. W. (2010). Reactivity of gluten detecting monoclonal antibodies to a gliadin reference material. Journal of Cereal Science, 51, 198–204. doi: 10.1016/j.jcs.2009.11.012
- Ehren, J., Morón, B., Martin, E., Bethune, M. T., Gray, G. M., & Khosla, C. (2009). A food-grade enzyme preparation with modest gluten detoxification properties. PLoS ONE, 4, e6313. doi: 10.1371/journal.pone.0006313
- Ellis, H. J., Rosen-Bronson, S., O'Reilly, N., & Ciclitra, P. J. (1998). Measurement of gluten using a monoclonal antibody to a coeliac toxic peptide of a gliadin. Gut, 43, 190–195. doi: 10.1136/gut.43.2.190
- Gobetti, M., Rizello, C. G., Di Cagno, R., & De Angelis, M. (2007). Sourdough lactobacilli and celiac disease. Food Microbiology, 24, 187–196. doi: 10.1016/j.fm.2006.07.014
- Greco, L., Gobetti, M., Auricchio, R., Di Mase, R., Landolfo, F., Paparo, F., … Aurichio, S. (2011). Safety for patients with celiac disease of baked goods made of wheat flour hydrolyzed during food processing. Clinical Gastroenterology and Hepatology, 9, 24–29. doi: 10.1016/j.cgh.2010.09.025
- Gregorini, A., Colomba, M., Ellis, H. J., & Cicltira, P. J. (2009). Immunogenicity characterization of two ancient wheat α-gliadin peptides related to coeliac disease. Nutrients, 1, 276–290. doi: 10.3390/nu1020276
- Halbmayr-Jech, E., Hammer, E., Fiedler, R., Coutts, J., Rogers, A., & Cornish, M. (2012). Characterization of G12 sandwich ELISA, a next-generation immunoassay fot gluten toxicity. Journal of AOAC International, 95, 372–376. doi: 10.5740/jaoacint.SGE_Halbmayr-Jech
- Hammer, E. (2012). Gluten-free—How can you prove it? International Food Hygiene, 23, 9–10.
- Kanerva, P., Sontag-Strohm, T. S., Ryöppy, P. H., Alho-Lehto, P., & Salovaara, H. O. (2006). Analysis of barley contamination in oats using R5 and ω-gliadin antibodies. Journal of Cereal Science, 44, 347–352. doi: 10.1016/j.jcs.2006.08.005
- Londono, D. M., van't Westende, W. P. C., Goryunova, S., Salentijn, E. M. J., van den Broeck, H. C., van der Meer, I. M., … Smulders, M. J. M. (2013). Avenin diversity analysis of the genus Avena (oat). Relevance for people with celiac disease. Journal of Cereal Science, 58, 170–177. doi: 10.1016/j.jcs.2013.03.017
- Makharia, G. K. (2014). Current and emerging therapy for celiac disease. Frontiers in Medicine, 1, 1–11. doi: 10.3389/fmed.2014.00006
- Mamone, G., Picariello, G., Addeo, F., & Ferranti, P. (2011). Proteomic analysis in allergy and intolerance to wheat products. Expert Review of Proteomics, 8, 95–115. doi: 10.1586/epr.10.98
- Mickowska, B., Socha, P., Urminská, D., & Cieślik, E. (2012). The comparison of prolamins extracted from different varieties of wheat, barley, rye and triticale species: amino acid composition, electrophoresis and immunodetection. Journal of Microbiology, Biotechnology and Food Sciences, 1, 742–752.
- Osborne, T. B. (1924). The vegetable proteins (2nd ed). London: Longmans Green. 154 pp.
- Rizello, C. G., Curiel, J. A., Nionelli, L., Vincentini, O., Di Cagno, R., Silano, M., … Coda, R. (2014). Use of fungal proteases and selected sourdough lactic acid bacteria for making wheat bread with an intermediate content of gluten. Food Microbiology, 37, 59–68. doi: 10.1016/j.fm.2013.06.017
- Rizello, C. G., De Angelis, M., De Cagno, R., Camarca, A., Silano, M., Losito, I., … Palmisano, F. (2007). Highly efficient gluten degradation by lactobacilli and fungal proteases during food processing: New perspectives for celiac disease. Applied and Environmental Microbiology, 73, 4499–4507. doi: 10.1128/AEM.00260-07
- Schägger, H., & von Jagow, G. (1987). Tricine-sodium dodecyl sulfate-polyacrylamide gel electrophoresis for the separation of proteins in the range from 1 to 100 kDa. Analytical Biochemistry, 166, 368–379. doi: 10.1016/0003-2697(87)90587-2
- Shan, L., Marti, T., Sollid, L. M., Gray, G. M., & Khosla, C. (2004). Comparative biochemical analysis of three bacterial prolyl endopeptidases: Implications for coeliac sprue. Biochemical Journal, 383, 311–318. doi: 10.1042/BJ20040907
- Shan, L., Molberg, Ø, Parrot, I., Hausch, F., Filiz, F., Gray, G. M., … Khosla, C. (2002). Structural basis for gluten intolerance in celiac sprue. Science, 297, 2275–2279. doi: 10.1126/science.1074129
- Siegel, M., Bethune, M. T., Gass, J., Ehren, J., Xia, J., Johannsen, A., … Khosla, C. (2006). Rational design of combination enzyme therapy for celiac sprue. Chemistry & Biology, 13, 649–658. doi: 10.1016/j.chembiol.2006.04.009
- Smith, B. J. (2003). Protein sequencing protocols (Methods in Molecular Biology), (Vol. 211, p. 508). New Jersey: Humana Press.
- Socha, P., Mickowska, B., Urminská, D., & Kačmárová, K. (2015). The use of different proteases to hydrolyze gliadins. Journal of Microbiology, Biotechnology and Food Sciences, 4(special issue 2), 101–104. doi: 10.15414/jmbfs.2015.4.special2.101-104
- Solid, L. M., & Khosla, C. (2005). Future therapeutic options for celiac disease. Nature Clinical Practice Gastroenterology & Hepatology, 2, 140–147. doi: 10.1038/ncpgasthep0111
- Stenman, S. M., Linfors, K., Venäläinen, J. I., Hautala, A., Männistö, P. T., Garcia-Horsman, J. A., … Kaukinen, K. (2010). Degradation of coeliac disease-inducing rye secalin by germinating cereal enzymes: Diminishing toxic effects in intestinal epithelial cells. Clinical and Experimental Immunology, 161, 242–249. doi: 10.1111/j.1365-2249.2010.04119.x
- Stenman, S. M., Venäläinen, J. I., Lindfors, K., Auriola, S., Mauriala, T., Kaukovirta-Norja, A., … Mäki, M. (2009). Enzymatic detoxification of gluten by germinating wheat proteases: Implications for new treatment of celiac disease. Annals of Medicine, 41, 390–400. doi: 10.1080/07853890902878138
- Stepniak, D., Spaenij-Dekking, L., Mitea, C., Moester, M., de Ru, A., Baak-Pablo, R., … Koning, F. (2006). Highly efficient gluten degradation with a newly identified prolyl endoprotease: Implications for celiac disease. American Journal of Physiology–Gastrointestinal and Liver Physiology, 291, G621–G629. doi: 10.1152/ajpgi.00034.2006
- Størsrud, S., Yman, I. M., & Lenner, R. A. (2003). Gluten contamination in oat products and products naturally free from gluten. European Food Research and Technology, 217, 481–485. doi: 10.1007/s00217-003-0786-0
- Thompson, T., & Méndez, E. (2008). Commercial assays to assess gluten content of gluten-free foods: Why they are not created equal. Journal of the American Dietetic Association, 108, 1682–1687. doi: 10.1016/j.jada.2008.07.012
- Vaccino, P., Becker, H. A., Brandolini, A., Salamini, F., & Kilian, B. (2009). A catalogue of Triticum monococcum genes encoding toxic and immunogenic peptides for celiac disease patients. Molecular Genetics and Genomics, 281, 289–300. doi: 10.1007/s00438-008-0412-8
- Wieser, H. (2007). Chemistry of gluten proteins. Food Microbiology, 24, 115–119. doi: 10.1016/j.fm.2006.07.004
- Zingorne, F., Capone, P., & Cicacci, C. (2010). Celiac disease: Alternatives to a gluten free diet. World Journal of Gastrointestinal Pharmacology and Therapeutics, 1, 36–39. doi: 10.4292/wjgpt.v1.i1.36