ABSTRACT
We developed a monoclonal antibody (mAb) to detect norfloxacin (NOR) using a novel hapten by introducing an amino group via amido linkage into the piperazine ring of NOR. The sensitivity of an indirect competitive enzyme-linked immunosorbent assay (icELISA) based on this mAb showed an improved IC50 value from 1.18 to 0.12 ng/mL and the limit of detection from 0.37 to 0.05 ng/mL by using a heterologous coating (pazufloxacin coupled to ovalbumin). The recovery rate for NOR in milk ranged from 77.3% to 117.8%. Cross-reactivity (CR) experiments showed good CR with nine common fluoroquinolone analogs: pefloxacin, lomfloxacin, ciprofloxacin, enrofloxacin, dafloxacin, sparfloxacin, ofloxacin, marbofloxacin and flumequine. Furthermore, based on this antigen–antibody combination, an immunochromatographic strip with cut-off value of 1 ng/mL was developed. Given their good sensitivity and CR, the developed icELISA and immunochromatographic strip could be useful for detection of NOR and its analogs in milk.
Introduction
The use of norfloxacin (NOR), a common fluoroquinolone antibiotic, is widespread in livestock for preventing disease and promoting growth due to its broad-spectrum antibacterial properties (Liu, Feng, Qian, Fang, & Wang, Citation2015). However, the long-term accumulation of NOR in the human body caused by eating food containing NOR residues can easily lead to phototoxic side effects (Beberok et al., Citation2015; Hubicka, Zmudzki, Talik, Zuromska-Witek, & Krzek, Citation2013). In addition, the long exposure to antibiotics has contributed to the emergence of resistant bacteria which has resulted in an increased risk of death from common infections and following common medical procedures (Gouvea, Dos, Aquino, & Pereira, Citation2015). Although the maximum residue of NOR has not been limited by the European Union, the China Ministry of Agriculture banned the use of NOR in food animals from December 31, 2015, and its use in animal feed from December 31, 2016 (Agriculture Citation2015). Therefore, the detection of NOR residues in food is particularly significant.
Currently, the methods of monitoring NOR focus on instrumental analytical methods and immunoassays (Annunziata et al., Citation2016; Bucknall, Silverlight, Coldham, Thorne, & Jackman, Citation2003; Chierentin & Salgado, Citation2016; Huang et al., Citation2016; Jiang et al., Citation2013; Liu et al., Citation2015; Mannemala & Kannappan, Citation2016; Mendes et al., Citation2015; Tong, Liu, Xie, & Li, Citation2016; Zhao et al., Citation2014). The most commonly used instrumental analytical methods including high-performance liquid chromatography and liquid chromatography–mass spectrometry are able to detect multiple fluoroquinolones, including NOR, simultaneously to give both quantitative and qualitative results, but the requirements for expensive apparatus and complicated sample preparation limit their application when monitoring samples in bulk (Tao et al., Citation2013). In such cases, immunoassay methods including enzyme-linked immunosorbent assay (ELISA) and immunochromatographic strips provide alternatives with the advantages that they are simple, rapid and have the capacity to deal with numerous samples (Chen et al., Citation2016; Peng, Song, Liu, Kuang, & Xu, Citation2016; Yu, Liu, Song, Kuang, & Xu, Citation2016). Immunoassay methods can be used as a preliminary screening tool for potential positive samples, and the sensitivity of the assay is expected to be low enough to avoid false negative results. Furthermore, along with the ban on NOR residues in animal feed, there is an urgent requirement for more sensitive determination methods. Therefore, a highly sensitive and broad-specificity immunoassay method based on a monoclonal antibody (mAb) was developed to detect fluoroquinolone residues in food.
Materials and methods
Reagents and instruments
NOR and 11 other analogs – pefloxacin (PEF), lomfloxacin (LOM), ciprofloxacin (CIP), enrofloxacin (ENR), dafloxacin (DAF), sparfloxacin (SPX), ofloxacin (OFL), marbofloxacin, flumequine (FLU), pazufloxacin (PAZ) and gatifloxacin (GAT) – were obtained from J&K Scientific Ltd (Shanghai, China). A goat anti-mouse immunoglobulin (IgG) antibody was obtained from Jackson ImmunoResearch Laboratories (West Grove, PA, USA). Keyhole limpet hemocyanin (KLH), bovine serum albumin (BSA), ovalbumin (OVA), horse-radish peroxidase (HRP)-labeled goat anti-mouse IgG, 3,3′,5,5′-tetramethylbenzidine (TMB), Tween-20 and gelatin were purchased from Sigma–Aldrich (St. Louis, MO, USA). Hypoxanthine–aminopterin–thymidine (HAT) and hypoxanthine–thymidine (HT) supplement and other cell culture reagents were purchased from Life Technologies Corporation (Shanghai, China). Other reagents and chemicals were obtained from the National Pharmaceutical Group Chemical Reagent Co., Ltd (Shanghai, China). A microplate reader (Thermo Labsystems, Beverley, MA, USA) was used to measure optical density (OD). Gold nanoparticles (AuNPs) were prepared in our laboratory as described previously (Kong et al., Citation2016).
Synthesis of immunogens and coating antigens
A novel hapten was designed and derived from NOR by introduction of an amino group by amido linkage in the piperazine ring of NOR. Briefly, 357 mg (0.94 mmol) HATU (2-(7-aza-1H-benzotriazole-1-yl)-1,1,3,3-tetramethyluronium hexafluorophosphate) and 243 mg (1.88 mmol) N,N-diisopropylethylamine (DIEA) were added into a solution of 132 mg (0.75 mmol) N-Boc-glycine in 20 mL dichloromethane. The resultant mixture was heated to 30°C and kept stirring for 30 min and then 200 mg (0.63 mmol) NOR was added. The reaction mixture was kept stirring overnight at 30°C. The residue was purified by recrystallization with methyl alcohol to give the intermediate compound (170 mg, 57%) as a white solid. A mixture of intermediate compound (170 mg, 0.357 mmol) in 5 mL HCl was kept stirring overnight at room temperature. Next day, 3000 mL water was added and the mixture was extracted with ethyl acetate. Finally, the solvent was removed under reduced pressure and the residue was purified by HPLC to give 80 mg of the desired hapten NOR-N as a yellow solid: Electronic Spray Ion analysis (positive) 377.1 [M + H+]; 1HNMR (300 MHz, CDCl3): δ 1.42 (t, 3 H), 3.34–3.39 (m, 4 H), 3.61 (m, 3 H), 3.73 (m, 9 H), 3.98 (m, 2 H), 4.60 (q, 2 H), 7.20 (d, 1 H), 7.95 (d, 1 H), 8.10 (m, 3 H), 8.97 (s, 1 H), 15.30 (br, 1 H).
The immunogen was prepared by conjugating NOR-N with KLH by the glutaraldehyde method (Yan, Liu, Xu, Kuang, & Xu, Citation2015). Briefly, 1.2 mM glutaraldehyde solution was added dropwise into a 1 mM solution of hapten dissolved in 200 μL dimethyl formamide. The mixture was reacted for 40 min with stirring in the dark. Subsequently, the solution was gradually added into 0.2 nM KLH diluted in 0.01 M carbonate buffer (CB) solution. Another four hours of reaction time was allowed, then the mixture was dialyzed for three days in 0.01 M phosphate-buffered saline (PBS) to give the immunogen NOR-N–KLH. The entire reaction was carried out at room temperature. The corresponding coating antigen was prepared by replacing 0.2 nM KLH with 10 nM OVA which then formed NOR-N–OVA. Considering the possibility that a heterologous coating antigen could improve the sensitivity of ELISA, the analog PAZ was coupled to OVA forming PAZ–OVA by the glutaraldehyde method described above and GAT–OVA was synthesized by GAT coupling to OVA by the active ester method (Juan et al., Citation2015). Briefly, the mixture of 1 mM GAT, 5 mM carbodiimide (EDC) and 5 mM N-hydroxysuccinimide (NHS) were dissolved in 200 μL PBS and stirred for six hours at room temperature. The mixtures were then added dropwise to 10 nM OVA in 0.01 M CB. After stirring overnight, the reactants were dialyzed for three days in 0.01 M PBS to give the coating GAT–OVA. All the immunogens and coating antigens were stored at 4°C for further experiments.
Production of mAb
Five female BABL/c mice obtained from the Shanghai Laboratory Animal Center (Shanghai, China) were immunized by continuous multipoint subcutaneous injection with the immunogen (Guo et al., Citation2015). The first immunization was performed with 100 µg/mouse emulsified in Freund’s complete adjuvant. After a month, another five booster immunizations were administered, one every 21 days with 50 µg/mouse emulsified in Freund’s incomplete adjuvant. Seven days after the last immunization, mice were tail-bled and the sera were evaluated by indirect competitive ELISA (icELISA) with the corresponding coating antigen. The mouse exhibiting good affinity to and inhibition of the most fluoroquinolones received a last intraperitoneal injection with 25 μg immunogen. Three days later, the mouse was sacrificed and cell fusion procedures were carried out. Briefly, the spleen was removed, splenocytes were isolated and fused with SP2/0 myeloma cells. After seven days of culture in HAT and HT solutions, supernatants of hybridoma cells were screened by icELISA and the selected hybridoma cells were cloned thrice by limiting dilution prior to ascites production. Antibodies were purified by ammonium sulfate precipitation and stored at 4°C for further experiments.
ELISA procedure
icELISA was performed to evaluate the affinity and specificity of the antibodies to the analyte in serum or supernatants of hybridoma cells as described previously (Li et al., Citation2014; Peng et al., Citation2013). Briefly, 100 µL/well coating antigen diluted in 0.01 M CB was added to the 96-well plates and incubated for 2 h at 37°C. The plates were next washed three times using 0.01 M PBS with 0.05% Tween 20 and blocked with 100 µL/well of 0.01 M CB with 0.2% (w/v) gelatin for 2 h at 37°C. After washing, 50 μL of each standard in PBS and 50 μL of mAb at certain concentration in antibody dilution solution (0.01 M PBS containing 0.05%, v/v, Tween 20 and 0.1%, w/v, gelatin) were added and incubated for 30 min at 37°C. The plates were then washed three times and 100 μL/well of HRP-conjugated goat anti-mouse IgG (diluted 1:3000 with antibody dilution buffer) was added. After incubation for 30 min at 37°C, the plates were washed four times and 100 µL/well of TMB solution (2 mL of 0.06%, w/v, TMB in glycol with 10 mL of 0.1 M citrate phosphate buffer, pH 5.0, containing 1.8 μL of 30% hydrogen peroxide) were added. Finally, 50 μL of 2 M H2SO4 were added to stop the reaction after incubation for 15 min at 37°C, and the OD was read at 450 nm in a microplate reader.
Sensitivity and specificity evaluation
To evaluate the icELISA, sigmoid curves were created using OriginPro 8.6 software (OriginLab Corp., Northampton, MA, USA). Analyte concentrations that provide a tracer binding inhibition in OD values at 50% and 20% were defined as the IC50 value and the limit of detection (LOD), respectively (Wang et al., Citation2007). Cross-reactivity (CR) values were calculated as follows: CR% = (IC50 of analyte/IC50 of analog) × 100. All nine fluoroquinolones were tested.
Preparation of AuNP-labeled mAb
The mAb labeled with AuNPs was prepared as described previously (Xing et al., Citation2014, Citation2015). Briefly, 40 μL 0.2 mg/mL purified antibody was added dropwise to 1 mL AuNP solution which was adjusted pH to 8 with 0.1 M K2CO3. The mixture was stirred for 30 min and 50 μL 10% (w/v) BSA was added dropwise to block any unreacted sites. Then the mixture was incubated at room temperature for 2 h and centrifuged twice at 8000 × g for 20 min. Finally, the AuNP-labeled mAb was resuspended in 0.002 M borate solution.
Preparation of immunochromatographic strips
The immunochromatographic strip was assembled in our laboratory as described previously (Chen et al., Citation2015). Briefly, goat anti-mouse IgG at the concentration of 0.5 mg/mL and the coating antigen PAZ–OVA at the concentration of 0.5 mg/mL were sprayed onto a nitrocellulose (NC) membrane to form the control and test lines (C line and T line, respectively) at an interval of 5 mm. After drying at 37°C for 30 min, the NC membrane was attached to the middle of a polyvinyl chloride support plate. Then the sample pad and absorbent pad were glued to the bottom and upper section of the plate, respectively, with 2 mm overlaps with the NC membrane. Finally, the plate was cut into 3 mm × 60 mm strips and stored in a desiccator for further experiments.
Immunochromatographic strip assay
A 150 μL aliquot of sample solution was added to 50 μL of AuNP-labeled mAb in the wells of a microtiter plate and the mixtures were allowed to react for 5 min at RT. The ends of the strips with the sample pad were then inserted into the mixtures. With the assistance of capillary action, the mixtures migrated from the sample pad, passing through the NC membrane where they reacted with the coating antigen on the T line and the goat anti-mouse IgG on the C line, finally reaching the absorption pad. The whole process took 5 min. In the NOR-negative sample, the AuNP-labeled mAb migrated and was captured by the coating antigen forming a deep color. In the NOR-positive sample, the AuNP-labeled mAb would combine with the NOR in the sample. Therefore, less AuNP-labeled mAb was available to combine with the coating antigen on the T line, resulting in a lighter color of T line. When the concentration of NOR increased beyond a certain amount, no color would develop at the T line. This critical concentration was defined as the cut-off value of the strip. In any case, the AuNP-labeled mAb or the NOR-AuNP-labeled mAb mixtures would continue to migrate and react with the goat-anti-mouse IgG antibody on the C line forming a deep red color.
Recovery test
A negative milk sample confirmed by LC/MS/MS was obtained from Jiangsu Entry-Exit Inspection and Quarantine Bureau. The milk samples were spiked with NOR standard solution at different concentrations. Spiked milk samples were diluted 10 times with 0.01 M PBS and analyzed by the developed ELISA. Each test was repeated three times.
Results and discussion
Hapten
As is well known, antibodies play an important role in any immunoassay, while the hapten design is the key step in obtaining a satisfactory antibody. In this study, we designed a novel hapten by introducing an amino group via the amido linkage into the piperazine ring of NOR to generate a sensitive antibody. depicts the synthesis of the hapten NOR-N, the immunogen NOR-N–KLH and the coating protein NOR-N–OVA by the glutaraldehyde method. depicts the synthesis of heterologous coating PAZ–OVA by glutaraldehyde method and GAT–OVA by the active ester method.
Antibody production and ELISA evaluation
After antisera screening using icELISA, the mouse with the best titer and greatest inhibition of NOR was sacrificed for fusion. Ultimately, the corresponding antibody marked mAb-1C9 was obtained after cell screening and antibody purification. Different concentration standards (0, 0.04, 0.12, 0.37, 1.11, 3.33 and 10 ng/mL) were prepared, and icELISA was developed to evaluate the sensitivity of mAb-1C9 with a coating antigen concentration of 0.1 μg/mL and mAb concentration of 0.02 μg/mL. Each point represents the mean ± SD of three replicates. As shown in , a sigmoid curve was created using NOR-N–OVA as the coating antigen which indicated an IC50 value of 1.18 ng/mL and LOD of 0.37 ng/mL. Some studies previously indicated that a heterologous coating could improve the sensitivity of immunoassay. Here, two heterologous coating antigens GAT–OVA and PAZ–OVA were synthesized to evaluate the IC50 of ELISA (). The heterologous coatings GAT–OVA and PAZ–OVA resulted in an IC50 as low as 0.38 and 0.12 ng/mL, respectively, for NOR which improved the sensitivity of the immunoassay by about 3 and 10 times, respectively (). Accordingly, LOD values of 0.17 and 0.05 ng/mL, respectively, were obtained as shown in . Likewise, the sensitivity of immunoassay for other FQ analogs was improved with heterologous coating. To evaluate the broad specificity, the CR of nine common FQ analogs was tested. As shown in , for mAb-1C9 using PAZ–OVA as the heterologous coating, as many as seven FQ analogs showed good CR exceeding 10%, including ENR, PEF, danofloxacin (DAN), CIP, OFL, marbofloxacin (MAR) and LOM. Meanwhile, weak CR was observed for FLU and SPX.
Figure 3. Sigmoid curves for NOR detection by icELISA using NOR-N–OVA, GAT–OVA and PAZ–OVA as coating antigen.
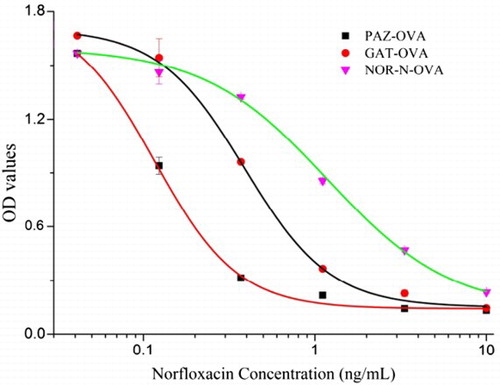
Table 1. The IC50 and cross-reactivities (CR) of 10 FQs using different coating antigens by icELISA.
Recovery test
Matrix interferences were eliminated by diluting spiked milk 10 times. As shown in , the average recovery of NOR in milk samples was 73.3–117.8% which indicated the developed ELISA was effective in the detection of NOR in milk.
Table 2. Recoveries of NOR in milk by icELISA.
Immunochromatographic strip assay
The test strip was tested in both the PBS matrix and in milk samples. A NOR stock solution of 1 mg/mL dissolved in 0.05 M Na2CO3 was prepared and NOR standards of different concentrations (0, 0.1, 0.25, 0.5, 1 ng/mL) were prepared by diluting with 0.01 M PBS. As shown in , a deep color was observed on the T line by the naked eye when 0 ng/mL NOR was added. As the concentration of NOR increased, the color on the T line became weaker. The color disappeared completely when the concentration of NOR reached 1 ng/mL. This concentration of 1 ng/mL was defined as the cut-off value when evaluating the sensitivity of the strip. Furthermore, several representative FQs were tested as shown in .
Figure 4. Images of NOR and nine representative FQs detection using the immunochromatographic strip assay in 0.01 M PBS. The concentrations of NOR were 0, 0.1, 0.25, 0.5 and 1 ng/mL (1 = 0 ng/mL, 2 = 0.1 ng/mL, 3 = 0.25 ng/mL, 4 = 0.5 ng/mL and 5 = 1 ng/mL). LOM (1 = 0 ng/mL, 2 = 0.1 ng/mL, 3 = 0.25 ng/mL, 4 = 0.5 ng/mL, 5 = 1 ng/mL and 6 = 2.5 ng/mL). PEF (1 = 0 ng/mL, 2 = 0.1 ng/mL, 3 = 0.25 ng/mL, 4 = 0.5 ng/mL and 5 = 1 ng/mL). CIP (1 = 0 ng/mL, 2 = 0.1 ng/mL, 3 = 0.25 ng/mL, 4 = 0.5 ng/mL, 5 = 1 ng/mL, 6 = 2.5 ng/mL and 7 = 5 ng/mL). OFL (1 = 0 ng/mL, 2 = 0.25 ng/mL, 3 = 0.5 ng/mL, 4 = 1 ng/mL, 5 = 2.5 ng/ mL and 6 = 5 ng/mL). DAN (1 = 0 ng/mL, 2 = 0.25 ng/mL, 3 = 0.5 ng/mL, 4 = 1 ng/mL and 5 = 2.5 ng/mL). ENR (1 = 0 ng/mL, 2 = 0.25 ng/mL, 3 = 0.5 ng/mL, 4 = 1 ng/mL, 5 = 2.5 ng/mL and 6 = 5 ng/mL). MAR (1 = 0 ng/mL, 2 = 0.25 ng/mL, 3 = 0.5 ng/mL, 4 = 1 ng/mL, 5 = 2.5 ng/mL and 6 = 5 ng/mL). SPX (1 = 0 ng/mL, 2 = 0. 5 ng/mL, 3 = 1 ng/mL, 4 = 2.5 ng/mL, 5 = 5 ng/mL and 6 = 10 ng/mL). FLU (1 = 0 ng/mL, 2 = 0.5 ng/mL, 3 = 1 ng/mL, 4 = 2.5 ng/mL, 5 = 5 ng/mL, 6 = 10 ng/mL and 7 = 20 ng/mL).
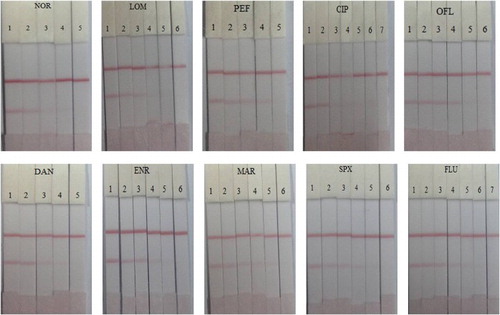
Conclusions
In this study, a NOR mAb with an IC50 of 1.18 ng/mL was prepared by introducing an amino group via amido linkage into the piperazine ring of NOR as the hapten. The resulting IC50 of 0.12 ng/mL showed that sensitivity was improved nearly 10 times by using PAZ–OVA as the heterologous coating. CR experiments for mAb-1C9 using a PAZ–OVA heterologous coating indicated good CR with up to seven FQ analogs which were ENR, PEF, DAN, CIP, OFL, MAR and LOM. Unfortunately, weak CR was observed for FLU and SPX. Based on this sensitive and class-specific mAb, we developed an icELISA with LOD of 0.05 ng/mL and an immunochromatographic strip with a cut-off value of 1 ng/mL for NOR detection in milk using PAZ–OVA as the heterologous coating.
Disclosure statement
No potential conflict of interest was reported by the authors.
Notes on contributors
Juan Peng is now a Ph.D. candidate at Jiangnan University. Her research is focused on the development of monoclonal antibodies and biosensors of veterinary drug.
Liqiang Liu obtained his Ph.D. in food science in 2014 from Jiangnan University, Wuxi, China, and then became a faculty in College of Food Science and Technology of Jiangnan University. His research interests are immunochromatographic strip design and application.
Hua Kuang obtained her Ph.D. from China Agricultural University in 2009 and then began to work as a faculty in College of Food Science and Technology of Jiangnan University. She is currently a full professor in food safety. Her research interests are biosensor development.
Gang Cui is a professor of Food Science and Technology of Jiangnan University. He obtained his Ph.D. in food science in 2007. His research interests are fast detection technology and food safety evaluation.
Chuanlai Xu is a full professor of Food Science and Technology of Jiangnan University. He obtained his Ph.D. in food science in 2002. His research interests are fast detection technology and food safety evaluation.
References
- Annunziata, L., Visciano, P., Stramenga, A., Colagrande , M. N., Campana, G., Scortichini, G., … Compagnone, D. (2016). Development and validation of a method for the determination of quinolones in muscle and eggs by liquid chromatography-tandem mass spectrometry. Food Analytical Methods, 9(8), 2308–2320.
- Beberok, A., Wrzesniok, D., Otreba, M., Milinski, M., Rok, J., & Buszman, E. (2015). Effect of norfloxacin and moxifloxacin on melanin synthesis and antioxidant enzymes activity in normal human melanocytes. Molecular and Cellular Biochemistry, 401(1–2), 107–114.
- Bucknall, S., Silverlight, J., Coldham, N., Thorne, L., & Jackman, R. (2003). Antibodies to the quinolones and fluoroquinolones for the development of generic and specific immunoassays for detection of these residues in animal products. Food Additives and Contaminants, 20(3), 221–228.
- Chen, Y. N., Kong, D. Z., Liu, L. Q., Song, S. S., Kuang, H., & Xu, C. L. (2016). Development of an ELISA and immunochromatographic assay for tetracycline, oxytetracycline, and chlortetracycline residues in milk and honey based on the class-specific monoclonal antibody. Food Analytical Methods, 9(4), 905–914.
- Chen, Y. N., Wang, Y. W., Liu, L. Q., Wu, X. L., Xu, L. G., Kuang, H., … Xu, C. L. (2015). A gold immunochromatographic assay for the rapid and simultaneous detection of fifteen beta-lactams. Nanoscale, 7(39), 16381–16388.
- Chierentin, L., & Salgado, H. R. N. (2016). Review of properties and analytical methods for the determination of norfloxacin. Critical Reviews in Analytical Chemistry, 46(1), 22–39.
- The China Ministry of Agriculture. (2015). Gazette of the Ministry of Agriculture of the People’s Republic of China, no. 2292.
- Gouvea, R., Dos, S. F. F., Aquino, M., & Pereira, V. L. D. (2015). Fluoroquinolones in industrial poultry production, bacterial resistance and food residues: A review. Brazilian Journal of Poultry Science, 17(1), 1–10.
- Guo, L. L., Song, S. S., Liu, L. Q., Peng, J., Kuang, H., & Xu, C. L. (2015). Comparsion of an immunochromatographic strip with ELISA for simultaneous detection of thiamphenicol, florfenicol and chloramphenicol in food samples. Biomedical Chromatography, 29(9), 1432–1439.
- Huang, W. H., Kong, Y., Yang, W. M., Ni, X. N., Wang, N. W., Lu, Y., & Xu, W. Z. (2016). Preparation and characterization of novel thermosensitive magnetic molecularly imprinted polymers for selective recognition of norfloxacin. Journal of Polymer Research, 23(5), 1–11.
- Hubicka, U., Zmudzki, P., Talik, P., Zuromska-Witek, B., & Krzek, J. (2013). Photodegradation assessment of ciprofloxacin, moxifloxacin, norfloxacin and ofloxacin in the presence of excipients from tablets by UPLC-MS/MS and DSC. Chemistry Central Journal, 7(1), 1–12.
- Jiang, W., Wang, Z., Beier, R. C., Jiang, H., Wu, Y., & Shen, J. (2013). Simultaneous determination of 13 fluoroquinolone and 22 sulfonamide residues in milk by a dual-colorimetric enzyme-linked immunosorbent assay. Analytical Chemistry, 85(4), 1995–1999.
- Juan, P., Dezhao, K., Liqiang, L., Shanshan, S., Hua, K., & Chuanlai, X. (2015). Determination of quinoxaline antibiotics in fish feed by enzyme-linked immunosorbent assay using a monoclonal antibody. Analytical Methods, 7(12), 5204–5209.
- Kong, D., Liu, L., Song, S., Suryoprabowo, S., Li, A., Kuang, H., … Xu, C. (2016). A gold nanoparticle-based semi-quantitative and quantitative ultrasensitive paper sensor for the detection of twenty mycotoxins. Nanoscale, 8(9), 5245–5253.
- Li, Z., Liu, L., Song, S., Guo, S., Kuang, H., & Xu, C. (2014). Development of an enzyme-linked immunosorbent assay for octylphenol. Food and Agricultural Immunology, 25(3), 397–410.
- Liu, C. C., Feng, X., Qian, H. L., Fang, G. Z., & Wang, S. (2015). Determination of norfloxacin in food by capillary electrophoresis immunoassay with laser-induced fluorescence detector. Food Analytical Methods, 8(3), 596–603.
- Mannemala, S. S., & Kannappan, V. (2016). Multiple response optimization of a liquid chromatographic method for determination of fluoroquinolone and nitroimidazole antimicrobials in serum and urine. Clinical Biochemistry, 49(7–8), 587–595.
- Mendes, C., Buttchevitz, A., Kruger, J. H., Bernardi, L. S., Oliveira, P. R., & Silva, M. A. S. (2015). Quantitative analysis of norfloxacin in beta-cyclodextrin inclusion complexes – development and validation of a stability-indicating HPLC method. Analytical Sciences, 31(10), 1083–1089.
- Peng, J., Song, S., Xu, L., Ma, W., Liu, L., Kuang, H., & Xu, C. (2013). Development of a monoclonal antibody-based sandwich ELISA for peanut allergen Ara h 1 in food. International Journal of Environmental Research and Public Health, 10(7), 2897–2905.
- Peng, S., Song, S. S., Liu, L. Q., Kuang, H., & Xu, C. L. (2016). Rapid enzyme-linked immunosorbent assay and immunochromatographic strip for detecting ribavirin in chicken muscles. Food and Agricultural Immunology, 27(4), 449–459.
- Tao, X., Chen, M., Jiang, H., Shen, J., Wang, Z., Wang, X., … Wen, K. (2013). Chemiluminescence competitive indirect enzyme immunoassay for 20 fluoroquinolone residues in fish and shrimp based on a single-chain variable fragment. Analytical and Bioanalytical Chemistry, 405(23), 7477–7484.
- Tong, L., Liu, H., Xie, C., & Li, M. J. (2016). Quantitative analysis analysis of antibiotics in aquifer sediments by liquid chromatography coupled to high resolution mass spectrometry. Journal of Chromatography A, 1452, 58–66.
- Wang, Z., Zhu, Y., Ding, S., He, F., Beier, R. C., Li, J., … , Shen J. (2007). Development of a monoclonal antibody-based broad-specificity ELISA for fluoroquinolone antibiotics in foods and molecular modeling studies of cross-reactive compounds. Analytical Chemistry, 79(12), 4471–4483.
- Xing, C., Kuang, H., Hao, C., Liu, L., Wang, L., & Xu, C. (2014). A silver enhanced and sensitive strip sensor for cadmium detection. Food and Agricultural Immunology, 25(2), 287–300.
- Xing, C., Liu, L., Song, S., Feng, M., Kuang, H., & Xu, C. (2015). Ultrasensitive immunochromatographic assay for the simultaneous detection of five chemicals in drinking water. Biosensors & Bioelectronics, 66, 445–453.
- Yan, H. J., Liu, L. Q., Xu, N. F., Kuang, H., & Xu, C. L. (2015). Development of an immunoassay for carbendazim based on a class-selective monoclonal antibody. Food and Agricultural Immunology, 26(5), 659–670.
- Yu, L., Liu, L. Q., Song, S. S., Kuang, H., & Xu, C. L. (2016). Development of an immunochromatographic test strip and ic-ELISA for tetrabromobisphenol: A detection in lake water and rice pudding samples. Food and Agricultural Immunology, 27(4), 460–470.
- Zhao, S., Zhao, H., Zhu, H., Li, C., Lu, X., & Bi, S. (2014). Determination of norfloxacin and ciprofloxacin in chicken meat based on matrix solid-phase dispersion extraction and capillary zone electrophoresis. Journal of Chemistry, 2014, 610120.