ABSTRACT
There has been an increasing concern with the safety of genetically modified (GM) crops. An important modification of GM crops is the expression of Bacillus thuringiensis (Bt) protein, Cry1Ab. Animal exposure to Cry1Ab indicates that the protein is safe, but that it is immunogenic. Whether Cry1Ab is a human immunogen and whether antibody response to this protein can serve as a marker of high exposure to GM crops is unknown. Here we develop an enzyme-linked immunosorbent assay to detect the presence of Cry1Ab-specific IgG in ∼100 individuals living in each of three countries that have varied exposure to GM crops (Papua New Guinea (PNG), low exposure; Kenya, moderate exposure; and the USA, high exposure). Cry1Ab-specific IgG antibodies were detected in individuals living in each region (8%, the USA; 3%, PNG; and 2%, Kenya). Thus, individuals develop anti-Cry1Ab antibodies at a frequency that roughly correlates with the exposure to GM crops expressing this protein.
1. Introduction
As of 2013, genetically modified (GM) crops accounted for approximately 12% (greater than 175 million hectares) of agricultural production worldwide (James, Citation2013). Global GM crop production has increased greater than 100-fold since 1996, and this pronounced rate of increase has established GM as the fastest implemented crop technology in contemporary history (James, Citation2013). Yet despite this continued global expansion, there has been an absence of epidemiological studies regarding human exposure to GM crops.
Bacillus thuringiensis (Bt) is a gram-positive soil-bearing bacteria that generates inclusion bodies during sporulation (Pusztai-CareyCitation, 2016). These spores or inclusion bodies include genetically encoded crystal (Cry) proteins that have broad-spectrum antimicrobial activities, especially against nematodes and insects of agricultural importance (Pigott & Ellar, Citation2007). The genes encoding these proteins have been cloned into a number of important crops such as corn, rice, and soybeans to create GM crops. Cry1Ab percentage of total crop biomass is a metric of interest; however, there is currently no consensus or agreement on measured results. Measured Bt concentrations have varied according to research group and have been documented at means ranging between 0.5 and 2.2 and between 0.1 and 14.8 µg Bt/gram fresh weight of plant (Lorch & Then, Citation2007; Zwahlen, Hilbeck, Gugerli, & Nentwig, Citation2003). These differences suggest that Cry1Ab expression significantly varies among Bt plant species and that methodological monitoring of Cry1Ab expression may need to be standardized.
Though Cry proteins are collectively toxic to many species, each individual Cry protein is generally toxic to only a few species due to receptor specificity (Pigott & Ellar, Citation2007). Though knowledge regarding Cry protein toxicity is still developing, the pore-forming model is the most widely accepted to date. Cadherin protein interactions with Cry proteins in the insect midgut are a part of the pore-forming model of Cry protein toxicity (Bravo, Gill, & Soberon, Citation2007). Cry protein toxicity requires target species ingestion, and subsequent alkaline proteolysis of Cry protoxins (∼130 kDa) to active monomeric toxins (∼70 kDa). Monomeric Cry toxins bind cadherin proteins to then subsequently form oligomers that ultimately form a pore in the apical intestinal epithelial cell membrane (Bravo et al., Citation2004). These pores then allow ions and water to pass freely into the cells, resulting in cell swelling, lysis, and eventually death of the host species (Bravo et al., Citation2004, Citation2007; Vachon, Laprade, & Schwartz, Citation2012).
Cry proteins bind cadherin proteins present on the intestinal epithelial cells of insects and nematodes, but do not bind to the intestinal epithelial cells of mammals (Shimada, Kim, Miyamoto, Yoshioka, & Murata, Citation2003; Shimada, Miyamoto, Kanda, & Murata, Citation2006). Mammalian intestinal epithelial cells lack cadherin protein expression. As a result, Bt corn and soybean expressing Cry proteins have been introduced extensively in the global food system, most notably through their use in animal feed and processed foods.
Animals feeding on crops expressing Cry1Ab develop a humoral response. BALB/c mice exposed to purified Cry1Ab protein, via the intranasal route, produced IgG1 and IgE antibodies (Andreassen et al., Citation2015). Rats fed transgenic rice containing the Cry1Ab protein generated a specific IgG2 response after a 90-day feeding trial of GM rice (Kroghsbo et al., Citation2008). Furthermore, intra-peritoneal injection of either Cry1 toxins (Cry1Aa, Cry1Ab, or Cry1Ac) into mice generated a strong and similar IgG response in both the periphery and mucosal secretions for each of these three toxins (Vázquez-Padrón, Moreno-Fierros, Neri-Bazán, de la Riva, & López-Revilla, Citation1999). Moderate to low levels of Cry1-specific IgM and IgA were also observed (Vázquez-Padrón et al., Citation1999).
Little data exist whether humans develop immune responses to Cry proteins, and whether an immune response is associated with previous GM crop exposure. Japanese patients with food allergies and exposure to GM crops failed to detect IgE specific to Cry1Ab (Nakajima, Teshima, Takagi, Okunuki, & Sawada, Citation2007). Yet another study of farmworkers exposed to topical Bt insecticides developed specific IgE and IgG recognizing Bt spores/vegetative antigens (Bernstein et al., Citation1999). Whether these antibodies were specific for Cry proteins found in the Bt spores was not examined.
Here we hypothesize that Cry proteins expressed in Bt crops are immunogenic, and have the ability to induce the production of Cry-specific IgG antibodies. We believe that regions in the world that produce and import a higher percentage of Bt crops will contain a larger population of individuals testing positive for Cry-specific IgG than those that produce and import Bt crops to a lesser degree. In order to test such hypotheses, we created a novel, sensitive enzyme-linked immunosorbent assay (ELISA) to measure anti-Cry1Ab IgG levels in human sera or plasma originating from countries with varying exposure to GM crops, ranging from high exposure in the USA, to intermediate and low exposure in Kenya and Papua New Guinea (PNG), respectively.
2. Methods
2.1. Study groups and sample size
Archived samples from Papua New Guinean adults were randomly selected for testing from a remote population where food sources are primarily from subsistence farming and hunting and gathering. Imported food is a small part of their diet and no formal GM crop adoption policies are present in PNG (Bourke, Citation2009). Kenyan diets are supplemented to an extent by imported foods. Kenya does not commercially grow Bt crops; however, they import foods from countries that grow GM crops and adopted Cry protein-containing maize for research purposes in 2001 (Mutoni, Citation2014). The USA has one of the highest exposure to GM and contains the highest GM crop hectarage (70.1 million) in the world (U.S. Department of Agriculture: National Agricultural Statistics Service, Citation2006). A low exposure or possible negative control group comprised samples from Kenyan children 6–24 months of age. There is no published data on antibody response to Cry proteins in humans, except for the development of anti-Bt extract IgE and IgG in 10–20% of farmworkers exposed to Bt products in pesticides (Bernstein et al., Citation1999). Thus, we estimated ∼100 samples per group would provide a 95% confidence interval of 5.8 that would be sufficient to show a significant difference in the US population compared to putatively low or nonreactive populations of PNG and Kenya.
Serum samples were obtained from subjects enrolled in other studies with ethical board approval by University Hospitals of Cleveland, OH, and appropriate Institutional Review Boards in PNG and Kenya. The samples were de-identified for use and thus deemed nonhuman research. Only samples were used in which the initial consent form indicated that the individual gave approval for his or her blood samples to be used for other studies. Samples were randomly selected from banks of serum samples.
2.2. Cloning and expression of Cry1Ab
The Cry1Ab gene was cloned from the natural Bacillus thuringiensis kurstaki subspecies HD-1 strain, and was then expressed in Echerichia coli. The 130 kDa protoxins formed inclusion bodies, which were then solubilized in 50 mM N-cyclohexyl-3-aminopropanesulfonic acid (CAPS) buffer, pH 10.5, in the presence of a reducing agent. Protoxins were then digested by commercial trypsin for 4 hours at room temperature. The 67 kDa activated Cry1Ab was then purified by anion exchange HPLC. The purity of the toxin was analyzed by gel-filtration high performance liquid chromatography (HPLC) and SDS-PAGE.
2.3. Cry1Ab-specific IgG ELISA
Immunolon 4 plates were coated with 5.0 µg/mL of purified Cry1Ab-activated toxin in phosphate-buffered saline (PBS at pH 7.4) 50 µL/well and incubated at 4°C overnight. The plates were washed with PBS containing 0.05% Tween-20, and then blocked with 150 µL PBS containing 5% casein and 0.05% Tween-20 for 1 hour at 37°C. After subsequent washing, 50 µL of serum/plasma (1:200) and 50 µL of rabbit anti-Cry1Ab IgG (AbCam, 1:2000) were added to the respective wells and then incubated at 4°C overnight. Each sample was run in duplicate. The plates were washed and then 50 µL of goat anti-human IgG (Jackson ImmunoResearch, 1:2500) and goat anti-rabbit IgG (Jackson ImmunoResearch, 1:2500) were added to the respective wells. The plates were incubated at 37°C for 1 hour. An alkaline phosphatase substrate tablet (Sigma-Aldrich) was dissolved in 5 mL of p-nitrophenyl phosphate liquid substrate, and 50 µL was added to each well. Colorimetric intensity (optical density (OD)405nm) was measured, and the plates were developed until a Papuan positive control sample reached an OD between 0.8 and 1.0. The reaction was stopped by adding 50 µL of EDTA to each well. Cry1Ab-specific IgG antibody levels were considered positive when the OD was at least three standard deviations (SD) greater than the mean OD of the negative control cohort.
Rabbit anti-Cry1Ab IgG obtained from AbCam was used as a positive control. Likewise, a consistently high-reacting Papuan sample and a consistently low-reacting North American sample were used as internal assay controls to limit plate-to-plate variation. Lastly, two wells containing neither serum/plasma nor anti-Cry1Ab IgG antibody were run on each plate to obtain a background OD. The averaged background OD was then subtracted from each sample OD prior to data/graph representation.
2.4. Western blot
Purified Cry1Ab-activated toxin was separated using an SDS-PAGE gel (4–20% acrylamide). After transferring proteins to a nitrocellulose membrane, the membrane was blocked with 0.5% casein dissolved in PBS-T. Vertical strips of membrane were cut vertically and subsequently incubated in sera/plasma (1:200) (rabbit anti-cry1Ab IgG for specificity control) overnight at 4°C. The following day, the strips were washed with PBS-T and then incubated with goat anti-human IgG (1:2500) (goat anti-rabbit IgG (1:2500) for specificity control) for 1 hour. After washing, the membranes were developed with alkaline phosphatase substrate solution.
3. Results
3.1. Purification of Cry1Ab
shows the purified Cry1 protein and it recognition by polyclonal rabbit anti-Cry1Ab IgG.
3.2. Cry1Ab-specific IgG ELISA
Using mean plus 3 standard deviations of 20 Kenyan children aged 6–24 months as a cutoff, 8 US, 3 Papua New Guinean, and 2 Kenyan samples were considered to be anti-Cry1Ab IgG positive (). The distributions of the Kenyan and PNG groups are similar: appear bimodal with most clustering below an OD of 0.5, while a few cluster above. This bimodal distribution is not seen in sero-reactivity from samples from the USA.
Figure 2. Antibody responses to Cry1 in adults from the USA (N = 100), Kenya (N = 100), and PNG (N = 100). Putative negative controls were from 20 Kenyan children aged 6–24 months. Polyclonal rabbit anti-Cry1Ab IgG (1:2000) served as a positive control. The quantitative cutoff value (mean of negative control cohort +3 SD), which defines the presence or absence of Cry1Ab-specific IgG, is indicated by the dotted line. All sera samples were diluted 1:200.
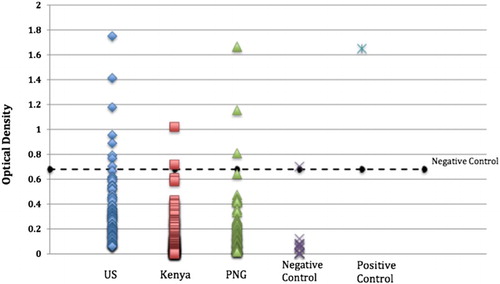
3.3. Western blot confirmation of human anti-Cry1Ab IgG
To confirm that the antibodies specifically recognized Cry1Ab protein, serum from the highest ELISA responder of each population recognized Cry1Ab protein by Western blot analysis (). Cry1Ab was recognized by the sera from individuals living in Kenya and PNG, but not by serum from the individual living in the USA. Rabbit anti-Cry1Ab was used as a positive control for immunoblotting. Staining patterns for the positive control and lanes containing Kenyan and Papua New Guinean serum positive for Cry1Ab-specific IgG responders were similar (). No band at the level of Cry1Ab was shown for the lane containing sera from the individual of US descent. Sera from study subjects that had low OD values in ELISA were used as negative controls.
Figure 3. Cry1Ab-specific IgG Western blotting with sera from the highest ELISA responder of each population. Lane 1, Western Blot of Cry1Ab recognized by alkaline phosphatase-conjugated polyclonal rabbit anti-Cry1Ab IgG. Lanes 2–4, sera with the highest ODs (from ) from PNG, Kenya, and the USA, respectively. Lanes 5–7, sera negative for anti-Cry1Ab IgG (below cutoff threshold in ). Western blots were probed with sera diluted 1:200. Lane 1 is Cry1 probed with rabbit polyclonal and Cry1 antibodies.
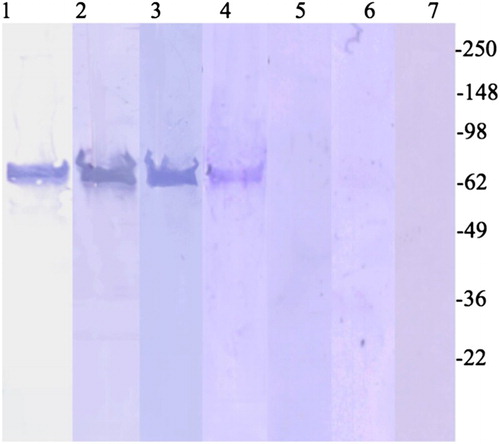
4. Discussion
In this study, we show that certain humans acquire antibody responses to Cry1Ab. We hypothesize that the ingestion of Cry1Ab proteins from GM crops is the most likely source of exposure to Cry1Ab. This is supported by higher serological responses in sera from US individuals where GM crops compose a much larger part of the diet compared to that in PNG and Kenya.
It is possible that Cry protein exposure may occur through means other than the consumption of GM (Bt) crops. Bt has been isolated from soil, leaf surfaces, aquatic environments, insect-rich environments, flourmills, and grain storage facilities (du Rand, Citation2009; Madigan & Martinko, Citation2005; Schnepf et al., Citation1998). Soil-based Cry protein exposure may explain the detection of anti-Cry1Ab antibodies in subjects living in Kenya and PNG, who have frequent exposure to soil, yet have little exposure to Bt crops due to GM agriculture regulations. Although soil-derived Cry1 proteins are not activated and thus conformationally different from the activated version used in the assay, portions of the molecule are likely to be recognized by both, especially linear epitopes. Indeed, sera reactivity denatured Cry1Ab on the SDS-PAGE gel indicates reactivity to linear epitopes.
Correspondingly, (Bt) Cry proteins have been used as bio-pesticides for mosquito control in malaria endemic regions (Goldberg & Margalit, Citation1977; Insell & Fitz-James, Citation1985; Klowden, Held, & Bulla, Citation1983). Bacillus thuringiensis serotype israelensis has been used in tropical disease control efforts in Kenya (Fillinger, Knols, & Becker, Citation2003) and South Eastern Asia (Brown et al., Citation2001), and further validates our detection of Cry-specific antibodies in individuals living in regions where Bt crops are not formally grown. Such nonfood-based Bt exposure is less likely to explain the development of Cry1Ab-specific IgG in study subjects from the USA because individuals living in the USA have much less environmental exposure compared to individuals from Kenya and PNG, yet have higher antibody levels. Likewise, due to the general structural homology among the Cry protein family (López-Pazos & Cerón, Citation2007), we also suggest that other Cry proteins used in agriculture, such as Cry1Ac and Cry1F, are also immunogenic in humans.
In order to stimulate an immune response following eating Bt GM crops, Cry proteins would need to override homeostatic oral tolerance mechanisms in humans. Future research investigating the immune response to Cry proteins specifically upon oral consumption, however, would be required in order to test this hypothesis. Because the dietary status of the individuals was unknown, the development of biomarkers for GM crop exposure is restricted. It is possible that individuals may have inhaled aerosolized Cry proteins from plate debris, dust, etc. In a BALB/c mouse model of airway allergy, inhalation of the activated Cry1Ab toxin has been shown to generate specific antibody responses (Andreassen, Citation2015).
Antibodies to specific Cry proteins may not be able to serve as epidemiological markers of previous GM crop exposure. Bt and its respective Cry proteins are ubiquitous in nature, and ethnographic research suggests that Cry protein exposure may occur through several other means outside of consumption of GM crops. This observation indicates that future attempts to measure GM crop exposure must focus on proteins only expressed in common GM crops themselves. Likewise, it is also important to develop standardized methods for comparable quantitative Cry protein determination in such crops (Székács et al., Citation2012). Developing biomarkers that may accomplish this role are of interest due to the largely unknown long-term physiological consequences of consuming or being exposed to novel proteins expressed in GM crops.
This preliminary study highlights the importance of developing future studies to understand how exposure to GM proteins induces immune responses in humans. Our work underlines the need to approach GM crop/food topics from an integrative perspective, one that includes environmental, food science, and medical disciplines, in order to study physiological food-mediated reactions.
Supplier information
Cry1Ab purified protein (Case Western Reserve University, Department of Biochemistry, Dr. Marianne Carey Laboratory) (http://www.case.edu/med/biochemistry/faculty/pusztai.html).
Anti-Bacillus thuringiensis Cry1Ab Toxin antibody (http://www.abcam.com/bacillus-thuringiensis-cry1ab-toxin-antibody-9-ab113679.html).
Anti-human IgG, Anti-rabbit IgG (https://www.jacksonimmuno.com/catalog/1).
Acknowledgements
We would like to thank CWRU professor Professor Mary Holmes for her everlasting support and encouragement; her novel academic and community work has inspired us to pursue food research avenues as they relate to individual and public health.
Disclosure statement
No potential conflict of interest was reported by the authors.
Notes on contributors
Alexander Razavi is currently pursuing his doctor of medicine (M.D.) and masters of public health (M.P.H) degrees from Tulane University School of Medicine.
Indu Malhotra (Ph.D) is a Research Scientist and Lab Manager at Case Western Reserve University School of Medicine's Center for Global Health and Diseases.
Anil Ghosh (Ph.D) was a Sr. Research Associate at Case Western Reserve University School of Medicine's Center for Global Health and Diseases during the time of the study and he is currently a Research Scientist at University of Washington's Center for Innate Immunity and Immune Disease.
Marianne Pusztai-Carey (Ph.D) is an Associate Professor in the Department of Biochemistry at Case Western Reserve University.
Jeffrey Marks (M.D.) is a Professor of Surgery at Case Western Reserve University School of Medicine and the Director of Surgical Endoscopy at University Hospitals Cleveland Medical Center.
Christopher King (M.D.), Ph.D is a Faculty researcher and Professor of international health, medicine, and pathology at Case Western Reserve University School of Medicine.
Additional information
Funding
References
- Andreassen, M. (2015). Immune properties of cry1Ab transgenic maize studied in mouse models of airway and food allergy. Scandinavian Journal of Immunology, 81(3), 192–200. doi: 10.1111/sji.12269
- Andreassen, M., Rocca, E., Bohn, T., Wikmark, O. G., van den Berg, J., Lovik, M., & Nygaard, U. C. (2015). Humoral and cellular immune responses in mice after airway administration of Bacillus thuringiensis Cry1Ab and MON810 cry1Ab-transgenic maize. Food and Agricultural Immunology, 26(4), 521–537. doi: 10.1080/09540105.2014.988128
- Bernstein, I. L., Bernstein, J. A., Miller, M., Tierzieva, S., Bernstein, D. I., Lummus, Z., & Seligy, V. L. (1999). Immune responses in farm workers after exposure to Bacillus thuringiensis pesticides. Environmental Health Perspectives, 107(7), 575–582. doi: 10.1289/ehp.99107575
- Bourke, R. M. (2009). History of agriculture in Papua New Guinea. Food and Agriculture in Papua New Guinea, 10–26.
- Bravo, A., Gill, S. S., & Soberon, M. (2007). Mode of action of Bacillus thuringiensis Cry and Cyt toxins and their potential for insect control. Toxicon, 49(4), 423–435. doi: 10.1016/j.toxicon.2006.11.022
- Bravo, A., Gomez, I., Conde, J., Munoz-Garay, C., Sanchez, J., Miranda, R., … Soberon, M. (2004). Oligomerization triggers binding of a Bacillus thuringiensis Cry1Ab pore-forming toxin to aminopeptidase N receptor leading to insertion into membrane microdomains. Biochimica et Biophysica Acta (BBA)-Biomembranes, 1667(1), 38–46. doi: 10.1016/j.bbamem.2004.08.013
- Brown, M. D., Carter, J., Watson, T. M., Thomas, P., Santagulianna, G., Purdie, D. M., & Kay, B.H. (2001). Evaluation of liquid Bacillus thuringiensis var. israelensis products for control of Australian Aedes arbovirus vectors. Journal of the American Mosquito Control Association, 17(1), 8–12. .
- du Rand, N. (July 2009). Isolation of entomopathogenic gram positive spore forming bacteria effective against coleoptera (PhD Thesis). University of KwaZulu-Natal, Pietermaritzburg.
- Fillinger, U., Knols, B. G., & Becker, N. (2003). Efficacy and efficiency of new Bacillus thuringiensis var. israelensis and Bacillus sphaericus formulations against Afrotropical anophelines in Western Kenya. Tropical Medicine & International Health, 8(1), 37–47. doi: 10.1046/j.1365-3156.2003.00979.x
- Goldberg, L. J., & Margalit, J. (1977). A bacterial spore demonstrating rapid larvicidal activity against Anopheles sergentii, Uranotaenia unguiculata, Culex univittatus, Aedes aegypti and Culex pipiens. Mosquito News, 37(3), 355–358.
- Insell, J. P., & Fitz-James, P. C. (1985). Composition and toxicity of the inclusion of Bacillus thuringiensis subsp. israelensis. Applied and Environmental Microbiology, 50(1), 56–62.
- James, C. (2013). Global status of commercialized biotech/GM crops: 2013. Ithaca, NY: International Service for the Acquisition of Agri-biotech Applications (ISAAA).
- Klowden, M. J., Held, G. A., & Bulla, L. A. (1983). Toxicity of Bacillus thuringiensis subsp. israelensis to adult Aedes aegypti mosquitoes. Applied and Environmental Microbiology, 46(2), 312–315.
- Kroghsbo, S., Madsen, C., Poulsen, M., Schrøder, M., Kvist, P. H., Taylor, M., … Knudsen, I. (2008). Immunotoxicological studies of genetically modified rice expressing PHA-E lectin or Bt toxin in Wistar rats. Toxicology, 245, 24–34. doi: 10.1016/j.tox.2007.12.005
- Lorch, A., & Then, C. (2007). How much Bt toxin do genetically engineered MON810 maize plants actually produce: Bt concentrations in field plants from Germany and Spain. Hamburg: Greenpeace.
- López-Pazos, S. A., & Cerón, J. (2007). Three-dimensional structure of Bacillus thuringiensis toxins: A review. Acta Biológica Colombiana, 12, 19–32.
- Madigan M. T., & Martinko, J. M. (Eds.). (2005). Brock biology of microorganisms (11th ed.) Boston: Prentice Hall. ISBN 978-0-13-144329-7.
- Mutoni, C. K. (2014). Assessment of maize food products in the Kenyan market for presence of genetic elements and proteins from genetically modified organisms (Doctoral Dissertation). Jomo Kenyatta University of Agriculture and Technology, Juja, Kenya.
- Nakajima, O., Teshima, R., Takagi, K., Okunuki, H., & Sawada, J. I. (2007). ELISA method for monitoring human serum IgE specific for Cry1Ab introduced into genetically modified corn. Regulatory Toxicology and Pharmacology, 47(1), 90–95. doi: 10.1016/j.yrtph.2006.08.003
- Pigott, C. R., & Ellar, D. J. (2007). Role of receptors in Bacillus thuringiensis crystal toxin activity. Microbiology and Molecular Biology Reviews, 71(2), 255–281. doi: 10.1128/MMBR.00034-06
- Pusztai-Carey, M. (2016). Research Interests. Department of Biochemistry, Case Western Reserve University School of Medicine. Retrieved from http://www.case.edu/medbiochemistry/faculty/pusztai.html
- Schnepf, E., Crickmore, N., Van Rie, J., Lereclus, D., Baum, J., Feitelson, J., & Dean, D. H. (1998). Bacillus thuringiensis and its pesticidal crystal proteins. Microbiology and Molecular Biology Reviews, 62(3), 775–806.
- Shimada, N., Kim, Y. S., Miyamoto, K., Yoshioka, M., & Murata, H. (2003). Effects of Bacillus thuringiensis Cry1Ab toxin on mammalian cells. Journal of Veterinary Medical Science, 65(2), 187–191. doi: 10.1292/jvms.65.187
- Shimada, N., Miyamoto, K., Kanda, K., & Murata, H. (2006). Bacillus thuringiensis insecticidal crylab toxin does not affect the membrane integrity of the mammalian intestinal epithelial cells: An in vitro study. In Vitro Cellular & Developmental Biology-Animal, 42(1–2), 45–49. doi: 10.1007/s11626-006-0011-0
- Székács, A., Weiss, G., Quist, D., Takács, E., Darvas, B., Meier, M., & Hilbeck, A. (2012). Inter-laboratory comparison of Cry1Ab toxin quantification in MON 810 maize by enzyme-immunoassay. Food and Agricultural Immunology, 23(2), 99–121. doi: 10.1080/09540105.2011.604773
- U.S. Department of Agriculture: National Agricultural Statistics Service (2006, 3 January). Ohio State Agriculture Overview, 2004. Retrieved January 12, 2006, from http://www.nass.usda.gov/Statistics_by_State/Ag_Overview/AgOverview_OH.pdf
- Vachon, V., Laprade, R., & Schwartz, J. L. (2012). Current models of the mode of action of Bacillus thuringiensis insecticidal crystal proteins: A critical review. Journal of Invertebrate Pathology, 111(1), 1–12. doi: 10.1016/j.jip.2012.05.001
- Vázquez-Padrón, R. I., Moreno-Fierros, L., Neri-Bazán, L., de la Riva, G. A., & López-Revilla, R. (1999). Intragastric and intraperitoneal administration of Cry1Ac protoxin from bacillus thuringiensis induce systemic and mucosal antibody response in mice. Life Sciences, 64, 1897–1912. doi: 10.1016/S0024-3205(99)00136-8
- Zwahlen, C., Hilbeck, A., Gugerli, P., & Nentwig, W. (2003). Degradation of the Cry1Ab protein within transgenic Bacillus thuringiensis corn tissue in the field. Molecular Ecology, 12(3), 765–775. doi: 10.1046/j.1365-294X.2003.01767.x