ABSTRACT
Effects of detoxified ginkgo nut powder (DGP) on lipid metabolism of obese mice were evaluated. Mice were divided into four groups: normal group (NC), hyperlipidaemic group (HC, high-fat feed), HC/DGP group (high-fat diet + DGP), and HC/flour group (HC/WF, high-fat diet + flour). After 18 weeks, liver and body fat weights of HC/DGP were significantly lower than those of HC and HC/WF, but similar to those of NC. The serum and hepatic levels of serum triglyceride, total cholesterol, low-density lipoprotein cholesterol, leptin and resistin in HC/DGP were similar to those in NC but significantly lower than those in HC and HC/WF. In addition, the expression levels of PPARα (peroxisome proliferator-activated receptor alpha) in liver and of hormone-sensitive lipase, adipose triglyceride lipase, and monoacylglycerol lipase in epididymal fat were higher in HC/DGP than in other groups. The results revealed that DGP may reduce inflammation and promote normal lipid metabolism.
1. Introduction
Obesity is a significant human health concern. According to World Health Organization, more than 30% of the global population is overweight or obese (Lankford, Hardman, Dankmeyer, & Schmid, Citation2013; Verhaeghe, Greve, & Annemans, Citation2016). Obesity contributes to several health problems, including hypertension, hyperlipidaemia, and diabetes, which increase the risk of heart disease (Shen, Zhang, Dong, Ren, & Chen, Citation2015). Additionally, obesity leads to high blood glucose and abnormalities in gene expression, such as PPARα and peroxisome proliferator-activated receptor gamma (PPARγ), which are strongly associated with lipid metabolism and inflammation (Khalifeh, Awaisheh, Alameri, & Hananeh, Citation2015).
The main causes of obesity are the excessive consumption of calories and the lack of physical activity (Vargas-Robles, Rios, Arellano-Mendoza, Escalante, & Schnoor, Citation2015). Hyperlipidaemia caused by a dysregulation in lipid metabolism is a critical feature of obesity and is strongly associated with metabolic disorders (Vargas-Robles et al., Citation2015). Food intake control and diet are crucial in obesity prevention and treatment (Botezelli et al., Citation2010). A variety of plant-based foods have been widely used in obesity treatment.
Ginkgo biloba extract (GBE) can effectively correct lipid metabolism in hyperlipidaemia (Li et al., Citation2016). G. biloba L., which is commonly referred to as “a living fossil,” is one of the oldest living tree species and is mainly distributed in East Asia. Gingko flavonoids, which play significant roles in human health, are the main component of GBE. GBE neutralizes free-radicals, minimizes cellular apoptosis (Zhou, Qi, & Chen, Citation2017), and improves vascular function (Kanowski, Herrmann, Stephan, Wierich, & Horr, Citation1997). Therefore, GBE has been widely used in weight loss and cardiovascular disease interventions.
Ginkgo nut, rich in GBE, has been used in Traditional Chinese Medicine for thousands of years. The macronutrient composition of ginkgo nut is similar to that of cereal grains; therefore, ginkgo nut can be an excellent substitute for grains. However, ginkgo nut has rarely been added to Chinese foods due to the presence of ginkgolic acids, which cause allergies and other undesirable side effects (Hausen, Citation1998; Koch, Jaggy, & Chatterjee, Citation2000). The maximum permissible level of ginkgolic acids in GBE is 5 ppm (Ahlemeyer, Selke, Schaper, Klumpp, & Krieglstein, Citation2001). Ginkgo nut can be used in food when ginkgolic acids have been removed.
DGP has been prepared in our laboratory by removing ginkgolic acids. DGP is considered to be a suitable dietary supplement due to its safety and biological activity. The objectives of this study were to evaluate the effect of DGP on lipid metabolism and weight loss in obese mice and provide information about the potential applications of DGP.
2. Materials and methods
2.1. Reagents
DGP (14.7% protein, 1.2% fat, 73.8% carbohydrate, 0.67 mg/kg ginkgolic acid, and 701.3 mg/kg gingko flavonoids) and wheat flour (11% protein, 1.6% fat, and 73.5% carbohydrate) were used in this study. DGP was prepared under the following process: Firstly, fresh ginkgo nuts were decorticated, and germs of ginkgo nuts were removed. Then, the ginkgo nuts were soaked in 3 times volume (v/w) of 5 g/l Na2CO2 at 15°C for 3 h. After that, the treated ginkgo nuts were dried at 60°C and grinded. The assay kits for the determination of serum triglyceride (TG), total cholesterol (TC), low-density lipoprotein cholesterol (LDL-C), high-density lipoprotein cholesterol (HDL-C), free fatty acids (FFAs), aspartate aminotransferase (AST), and alanine aminotransferase (ALT) were supplied by Nanjing Jiancheng Bioengineering Institute (Nanjing, Jiangsu province, China). The ELISA (enzyme-linked immuno sorbent assay) kits for serum leptin, adiponectin, and resistin were acquired from Wuhan Boster Biological Technology Co. (Wuhan, Hubei province, China).
2.2. Experimental animals
C57BL/6J mice (male, 19–20 g, specific pathogen-free grade, certificate No. SCXK [HU] 2012-0002) were obtained from Shanghai Slac Laboratory Animal Corporation (Shanghai, China) and housed at 24 ± 2°C, 60% ± 10% humidity, and with 12-h light/dark cycles. The mice had ad libitum access to feed and water. Following a one-week adaptation period, the mice were randomly divided into four groups.
2.3. Diets and experimental design
The study was approved by the Animal Care Ethics Committee of Jiangnan University. All procedures were performed in strict accordance with the Guide for the Care and Use of Laboratory Animals. The mice were weight-matched and randomly assigned to one of the four following dietary groups: normal group (n = 20; NC, fed diet AIN-93M; Reeves, Nielsen, & Fahey, Citation1993), hyperlipidaemic group (n = 20; HC, fed a high-fat feed), HC/DGP group (n = 20; fed a high-fat diet supplemented with DGP), and HC/wheat flour group (n = 20; HC/WF, fed a high-fat diet supplemented with wheat flour). The diets were based on AIN-93M. The mice had ad libitum access to their respective diets for 18 weeks. The nutrient composition of the diets is presented in . Weight gain and food consumption were monitored every week.
Table 1. Ingredient composition of the diets fed to mice.
At the end of the feeding trial, mice were fasted overnight. Surgical procedures including orbital eye bleeding and euthanasia were performed under anaesthesia (inhalation of 2% isoflurane via a nose cone). Blood samples were collected and centrifuged at 1000 g for 15 min at 4°C. The resulting supernatant was stored at −80°C for biochemical analysis. Epididymal, intestinal and perirenal fat, liver, and kidney were excised, weighed, and stored at −80°C.
2.4. Measurement of body weight, food intake, and fat mass
Body weight and food intake (five mice per cage) were measured each week. The energy values of each diet were calculated. The amount of food consumed every week per cage was measured by weighing the uneaten food. Total fat mass was calculated by adding the weights of the different collected fat pads (epididymal, intestinal, and perirenal).
2.5. Measurement of liver and epididymal fat parameters
The hepatic and epididymal lipids were extracted according to the procedure of Folch’s methods (Folch, Lees, & Sloan-Stanley, Citation1957). The TG, TC, FFA and lipid content were analysed using the same enzymatic kit as used for the serum analysis.
2.6. Histopathological studies
Liver samples were fixed in 10% formalin neutral buffer for 48 h, dehydrated in ethanol, and embedded in paraffin. Liver sample sections (5 μm thick) were obtained using a microtome. The sections were dewaxed, rehydrated, stained with haematoxylin–eosin (HE), and observed under a XSP-13CC optical microscope (Caikang Optical instrument Co., Ltd., Shanghai, China).
2.7. RNA extraction and gene expression analysis
Total RNA was extracted from liver and epididymal fat samples using an RNA extraction kit (Nanjing Jiancheng Bioengineering Institute, Nanjing, Jiangsu province, China). Total RNA from liver samples was used for the analysis of PPAR-α gene expression, and total RNA from epididymal fat samples was used for the analysis of PPAR-γ, hormone-sensitive lipase (HSL), adipose triglyceride lipase (ATGL), and monoacylglycerol lipase (MGL) expression. Total RNA (3.0 μg) was reverse-transcribed into cDNA using the Revert Aid First Strand cDNA synthesis kit (TaKaRa Bio Inc., Tokyo, Japan). Real-time quantitative PCR was performed using SYBR Premix Ex TaqTM (TaKaRa Bio Inc.. Tokyo, Japan) in a QuantStudioTM 6 Flex Real-Time PCR system (Applied Biosystems, Foster City, CA, USA). The primers are shown in . The ΔΔCt method was used for the relative quantification of gene expression. The ΔΔCt value of each sample was determined by calculating the difference between the Ct value of the target genes and the Ct value of the β-actin reference gene.
Table 2. Nucleotides for real-time RT-PCR.
2.8. Statistical analysis
Data were expressed as mean ± SD and analysed using SPSS software (version 20; SPSS Inc., Chicago, IL, USA). Duncan multiple values test and Student t-test were used to detect differences among groups. Statistical significance was set at p < .05.
3. Results and discussion
3.1. Food intake, body weight, total fat weight, and liver and kidney weights of mice
The high-fat diet effectively induced obesity in mice, based on the increase in body weight (). Liver weight, total fat, and kidney weight were higher in the HC group than in the NC group (). The high-fat diet-induced obesity model had similar pathological features as those of other mouse obesity models (Liu et al., Citation2015).
Figure 1. Body mass of male C57BL/6J mice in each group (g, weekly average, n = 20 mice per group); NC, normal control; HC, hyperlipidaemia control; HC/WF, HC–wheat flour diet; HC/DGP, HC–detoxification ginkgo nut powder diet; values are mean ± S.D; means with different letters (a–d) differ significantly (p < .05).
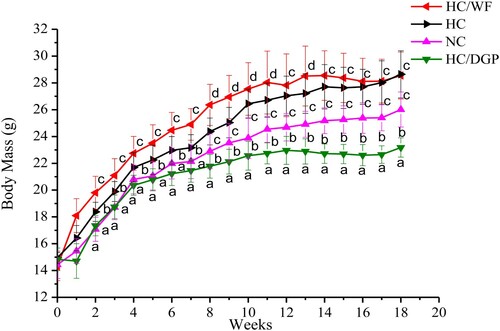
Table 3. Mass of relative viscera samples from the male C57BL/6J mice (g, n = 10 mices per group).
At 18 weeks, mean body weight was higher in the HC and HC/WF groups than in the NC and HC/DGP groups (). The HC/DGP group had the lowest body weight (p < 0.05). Additionally, food intake was lower in the HC/DGP group than in the HC group (p < .05), and the HC group had the highest food intake (). shows that the HC and HC/WF groups had significantly higher total fat values (intestinal fat, epididymal fat, and perirenal fat) than the NC and HC/DGP groups (p < .05). Kidney weight was higher in the HC and HC/WF groups than in the NC and HC/DGP groups (p < 0.05). At 18 weeks, mice from the HC and HC/WF groups had higher liver weight than the NC and HC/DGP groups (p < 0.05).
Table 4. Food intake of C57BL/6J mice in each group (kcal/mouse/day, daily average, n = 20 mice per group).
This long-term feeding study provides justification for the use of DGP as a dietary supplement for the prevention of obesity. Based on the results, DGP contributed to a significant reduction in body, liver, kidney, and total fat weights.
3.2. Effects of DGP on serum lipid levels
Serum lipid levels in mice at 9 and 18 weeks are presented in . At 9 weeks, the HC group had higher serum levels of TG, TC, LDL-C, AST, and ALT and lower serum levels of HDL-C than the NC and HC/DGP groups (p < .05). At 18 weeks, serum TG, TC, and LDL-C levels in HC/DGP were lower than those in HC and HC/WF (p < .05), but similar to those in NC. However, serum HDL-C levels were the higher in HC/DGP than those in HC and HC/WF.
Figure 2. The serum levels of TG, TC, LDL-C, HDL-C, ALT and AST in each group (n = 10 mice per group); A, TG; B, TC; C, LDL-C; D, HDL-C; E, ALT; F, AST; NC, normal control; HC, hyperlipidaemia control; HC/WF, HC–wheat flour diet; HC/DGP, HC–detoxification ginkgo nut powder diet; values are mean ± S.D.; means with different letters (a–d) differ significantly in the 9th week (p < .05). Means within a row with different letters are significantly different (x–z) in the 18th week (p < .05).
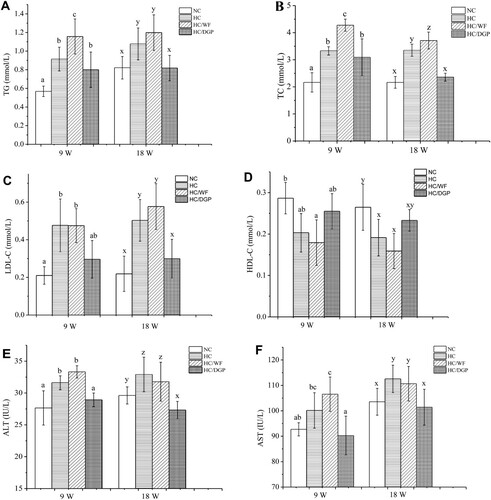
Studies have shown that high serum lipid levels are positively correlated with cardiovascular disease risk (Kunnen & Van, Citation2012). The serum levels of TC, TG, LDL-C, HDL-C, and FFAs are commonly used in the diagnosis of diseases associated with lipid metabolic disorders. TG is the main lipid in human body. Abnormally high or low levels of serum TGs can contribute to cardiovascular diseases and liver dysfunction (Wooton & Melchior, Citation2017). TC, an essential component of cell membranes, can lead to blood vessel damage at high levels (Hoshida et al., Citation1996). LDL and HDL are major lipoproteins. LDL particles deposit cholesterol on arteries and contribute to atherosclerosis (Hazarika, Kalita, Kalita, & Devi, Citation2017), while HDL particles remove lipid molecules from arteries (Ling, Jiang, Wu, & Gao, Citation2017). LDL-C is commonly used to estimate LDL levels. Similarly, HDL-C is used to determine HDL levels. Our study findings showed that DGP decreased serum TC, TG, and LDL-C levels and increased serum HDL-C levels. Opposite results were obtained with HC and HC/WF. WF had no effect on weight gain prevention or lipid metabolism disorders, even though it had the same macronutrient composition as DGP.
3.3. Effects of DGP on leptin, resistin, and adiponectin
Compared to HC and HC/WF, NC and HC/DGP had lower serum FFA, leptin, and resistin levels after 18 weeks of treatment (). In contrast, serum adiponectin levels were higher in NC and HC/DGP than in HC and HC/WF.
Figure. 3. The serum levels of free fatty acid, leptin, resistin and adiponectin in each group (n = 10 mice per group); NC, normal control; HC, hyperlipidaemia control; HC/WF, HC–wheat flour diet; HC/DGP, HC–detoxification ginkgo nut powder diet; values are mean ± S.D.; means with different letters (a–d) differ significantly in the 9th week (p < .05). Means within a row with different letters are significantly different (x–z) in the 18th week (p < .05).
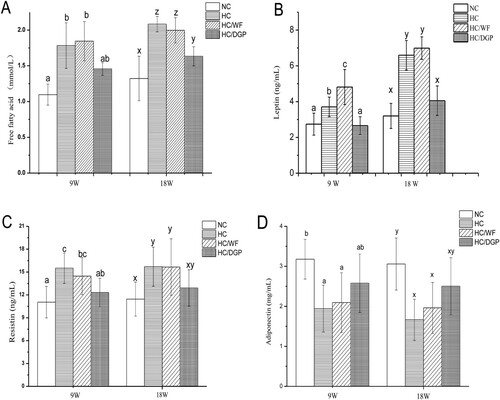
Leptin, resistin, and adiponectin are vital hormones that are indicative of lipid metabolism. Leptin, produced by adipocytes (Majdi et al., Citation2017), regulates the amount of fat stored in the body by decreasing hunger (Arita, Kinoshita, Ushida, Enomoto, & Inagaki-Ohara, Citation2016). Obesity decreases leptin sensitivity. In this study, high serum leptin levels were accompanied by an increase in hepatic steatosis (Kshatriya et al., Citation2011). Resistin is secreted mainly by the adipose tissue and is strongly associated with inflammatory markers (Qi et al., Citation2008). Adiponectin, secreted by adipocytes, has anti-inflammatory properties (Chang et al., Citation2016) and plays important roles in glucolipid metabolism and atherosclerosis prevention (Chang et al., Citation2016). Adiponectin levels are reduced in obese and insulin-resistant animal models. Decreased adiponectin levels have been implicated in the development of hepatic steatosis in obesity and lipodystrophy mouse models.
The results revealed that the HC and HC/WF mice suffered from a lipid metabolic disorder. WF had no protective effects on lipid metabolism. In contrast, DGP restored serum leptin, resistin, and adiponectin to normal levels. Therefore, DGP normalized lipid metabolic disorders in mouse models with high-fat diet-induced obesity.
3.4. Effects of DGP on the liver
There is a close association between obesity and liver damage (e.g. fatty liver). Serum levels of AST and ALT and hepatic levels of TC, TG, and FFAs are important liver indicators (Watts & Burke, Citation1996). (E,F) shows that serum ALT levels were lower in the HC/DGP group than in the HC and HC/WF groups (p < .05). Serum AST levels had similar trends to those of ALT; however, the HC/DGP group had similar AST levels as the NC group. The remaining liver indicators after 9 and 18 weeks are shown in . Liver TG and FFA levels in HC/DGP were lower than those in HC and HC/WF (p < .05), but similar to those in NC. Liver TC levels were lower in HC/DGP than in HC/WF and HC (p < .05), with no significant differences between NC at 9 weeks, but increased at 18 weeks. The HC/DGP group had lower hepatic lipid levels than the HC and HC/WF groups at 9 weeks (p < .05) and reached the lowest levels at 18 weeks (p < .05).
Figure 4. Liver parameters of mice in each group (n = 10 mice per group); A, TG; B, TC; C, free fatty acid; D, lipid content; NC, normal control; HC, hyperlipidaemia control; HC/WF, HC–wheat flour diet; HC/DGP, HC–detoxification ginkgo nut powder diet; values are mean ± S.D.; means with different letters (a–d) differ significantly in the 9th week (p < .05). Means within a row with different letters are significantly different (x–z) in the 18th week (p < .05).
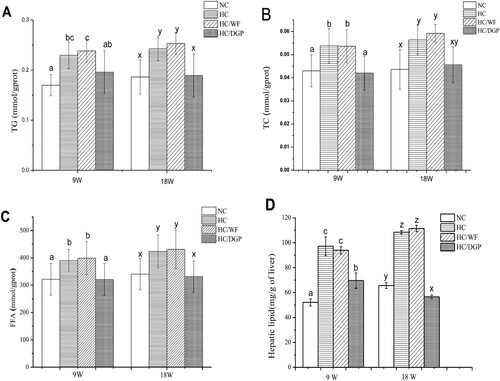
DGP had a positive effect on lipid metabolism and decreased serum TG, TC, and FFA levels when compared to HC. Based on our results, DGP suppressed serum and hepatic lipid levels in HC mice by modulating lipid metabolic enzymes.
Photomicrographs of liver samples stained with HE after 9 and 18 weeks revealed the presence of hepatic steatosis in the groups (). NC and HC/DGP mice had normal hepatocyte structure. HC and HC/WF mice had minimal central zone micro- and macrovesicular steatosis. However, significant micro- and macrovesicular steatosis was observed in HC and HC/WF mice. HC mice had fatty lipid droplets with changes in hepatocyte size and shape. Liver sections from the HC/DGP group had reduced lipid droplets.
Figure 5. Photomicrographs of haematoxylin and eosin stained of mice liver under light magnification. Data shown are representative images (magnification 100×) of each group (n = 10 per group). Typical photographs of liver sections of NC mice (A) HC mice (B) HC/WF mice (C) HC/DGP mice (D) in 9 weeks; NC mice (a) HC mice (b) HC/WF mice (c) HC/DGP mice (d) in 18 weeks.
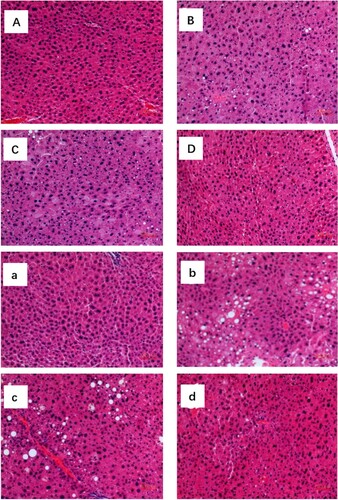
The ingestion of a high-fat diet stimulates de novo lipogenesis, which increases the fatty acid availability to the liver and potentially increases TG overproduction (Postic & Girard, Citation2008). In hepatocytes, the increased retention of lipids in the form of TC, TG, and FFAs leads to fatty liver (Te et al., Citation2004). HC diets can cause steatosis, and in the present study, we demonstrated that mice fed the HC diet had hepatic steatosis. The liver metabolizes FFAs by two pathways, and these pathways are strongly associated with hepatic steatosis (Browning & Horton, Citation2004).
Even though WF (11% protein, 1.6% fat, and 73.5% carbohydrate) and DGP (14.7% protein, 1.2% fat, and 73.8% carbohydrate) have similar macronutrient composition, WF did not improve lipid metabolism in obese mice. In contrast, DGP reduced lipid metabolic indicators to the levels observed in the control group. Therefore, DGP has a hepatoprotective role in models of high-fat diet-induced obesity.
3.5. Effects of DGP on epididymal fat
shows the levels of TG and TC in epididymal fat. Compared to HC and HC/WF, NC and HC/DGP had lower TG and TC levels in epididymal fat at 9 weeks (p < 0.05). At 18 weeks, HC/DGP had the lowest TG levels among all groups (p < .05), while HC/WF had the highest TG levels. The HC/DGP group had lower TG and TC levels in epididymal fat than the HC/WF group after 18 weeks of treatment (p < .05). Therefore, DGP decreased both epididymal fat weight and lipid and cholesterol levels.
Figure 6. The epididymal fat levels of triglyceride and total cholesterol in each group (n = 10mice per group); NC, normal control; HC, hyperlipidaemia control; HC/WF, HC–wheat flour diet; HC/DGP, HC–detoxification ginkgo nut powder diet; values are mean ± S.D.; means with different letters (a–d) differ significantly in the 9th week (p < .05). Means within a row with different letters are significantly different (x, y, z, k) in the 18th week (p < .05).
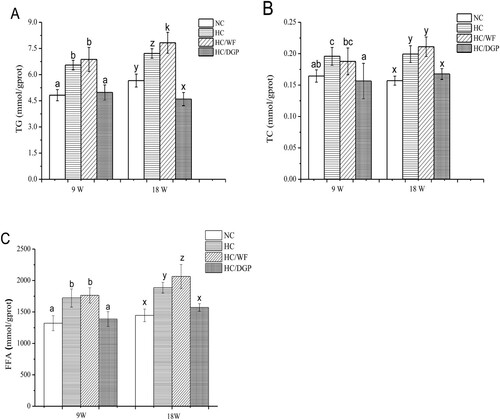
3.6. Transcription of PPARα, ATGL, HSL, MGL, and PPARγ
To better understand the reduced weight gain observed in the HC/DGP group, we explored lipolysis in mice at 18 weeks. Hepatic PPARα mRNA levels were significantly higher in the HC/DGP group than in other groups (A). As shown in (B–E), HC/DGP had the highest mRNA levels of ATGL, HSL, and MGL and the lowest mRNA levels of PPARγ in epididymal fat.
Figure 7. Expression of lipolytic genes in adipose tissue. Activated receptor (PPARα) mRNA concentrations (A) in liver. ATGL, MGL, HSL and activated receptor γ (PPAR γ) mRNA concentrations (B, C, D and E). NC, normal control; HC, hyperlipidaemia control; HC/WF, HC–wheat flour diet; HC/DGP, HC–detoxification ginkgo nut powder diet; values are mean ± S.D.; means with different letters (a–c) differ significantly in the 18th week (p < .05).
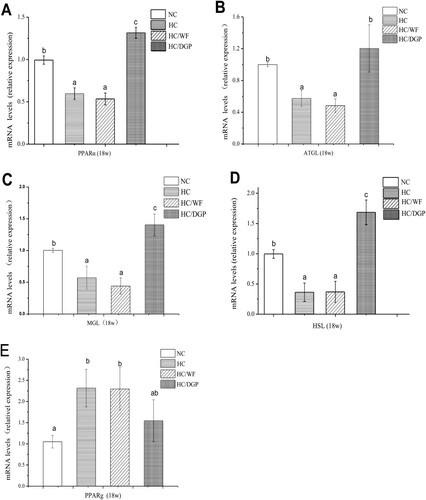
PPARs, which include PPARα, PPARβ and PPARγ, are steroid hormone receptors (Imran et al., Citation2017). PPARs play a role in lipid metabolism, obesity, and cardiovascular diseases (Eckel, Daniels, Jacobs, & Robertson, Citation2005). PPARs have hypolipidaemic effects (Staels et al., Citation1998). PPARα regulates the expression of genes involved in fatty acid beta oxidation in the liver. Downregulation or deficient expression of PPARα is associated with the development of non-alcoholic fatty liver disease. PPARα agonists prevent and inhibit the development of non-alcoholic fatty liver disease (Ahmed & Byrne, Citation2007). In this study, the HC group had low hepatic PPARα mRNA levels, while the control group and the DGP-supplemented group had high hepatic PPARα mRNA levels.
Unlike PPARα, PPARγ is highly expressed in the adipose tissue. PPARγ binds to NF-κB and TNFα (Croasdell et al., Citation2015), which are associated with inflammation and necrosis (Olefsky & Glass, Citation2010). shows that the HC/DGP group had the lower PPARγ expression in epididymal fat than the HC and HC/WF groups, but similar to the NC group. Therefore, DGP reduced inflammation.
TGs are hydrolysed by ATGL, HSL, and MGL (Lafontan & Langin, Citation2009). DGP increased mRNA ATGL, MGL, and HSL levels in epididymal fat (p < 0.05), suggesting that the upregulation of lipase is part of the mechanism of PPARγ action on lipolysis. Our findings revealed that DGP may be an effective therapeutic strategy for the treatment of HC-induced hypercholesterolaemia and related cardiovascular diseases.
4. Conclusions
Although ginkgo nut was an important traditional food and medicine source for Chinese, it was seldom applied widely because of its toxicity (Kobayashi, Yoshimura, Johno, Sasaki, & Wada, Citation2011). There were several studies about identification of ginkgo nut protein and starch (Deng et al., Citation2011; Miao et al., Citation2012), but no application reports about detoxified ginkgo nut or DGP. This study confirmed that DGP has preventive effects on obesity and hyperlipidaemia. DGP significantly reduced body weight, liver and fat weights, and serum lipid indicators in obese mice. DGP downregulates the expression of PPAR-γ and upregulates the expression of ATGL, HSL, MGL, and PPAR-α. The results not only showed that DGP is a good ingredient for diet food and healthy food, but also suggested a theoretical basis for DGP consumption in large quantities.
Disclosure statement
No potential conflict of interest was reported by the authors.
Additional information
Funding
Notes on contributors
Haiying Liu
Dr. Haiying Liu, associate professor of School of Food Science and Technology at Jiangnan University. His research is focused uponin functional food, collagen and grain.
Xinli Pei
Xinli Pei, graduate student of School of Food Science and Technology at Jiangnan University. His is interested in grain nutrition.
Kaiwen Shi
Kaiwen Shi, graduate student of School of Food Science and Technology at Jiangnan University. His research is focused uponin functional food.
Jing Wang
Jing Wang, graduate student of School of Food Science and Technology at Jiangnan University. Her research is focused uponin functional food.
Fei Han
Dr. Fei Han, associate professor of Academy of State Administration of Grain (ASAG). Her research interests include the human health.
Aike Li
Dr. Aike Li, professor of Academy of State Administration of Grain (ASAG). His is interested in animal nutrition.
References
- Ahlemeyer, B., Selke, D., Schaper, C., Klumpp, S., & Krieglstein, J. (2001). Ginkgolic acids induce neuronal death and activate protein phosphatase type-2C. European Journal of Pharmacology, 430(1), 1–7. doi: https://doi.org/10.1016/S0014-2999(01)01237-7
- Ahmed, M. H., & Byrne, C. D. (2007). Modulation of sterol regulatory element binding proteins (SREBPs) as potential treatments for non-alcoholic fatty liver disease (NAFLD). Drug Discovery Today, 12(17–18), 740–747. doi: https://doi.org/10.1016/j.drudis.2007.07.009
- Arita, S., Kinoshita, Y., Ushida, K., Enomoto, A., & Inagaki-Ohara, K. (2016). High-fat diet feeding promotes stemness and precancerous changes in murine gastric mucosa mediated by leptin receptor signaling pathway. Archives of Biochemistry and Biophysics, 610, 16–24. doi: https://doi.org/10.1016/j.abb.2016.09.015
- Botezelli, J. D., Mora, R. F., Dalia, R. A., Moura, L. P., Cambri, L. T., Ghezzi, A. C., Mello, M. A. (2010). Exercise counteracts fatty liver disease in rats fed on fructose-rich diet. Lipids in Health and Disease, 9(1), 116. doi:https://doi.org/10.1186/1476-511X-9-116
- Browning, J. D., & Horton, J. D. (2004). Molecular mediators of hepatic steatosis and liver injury. Journal of Clinical Investigation, 114(2), 147–152. doi: https://doi.org/10.1172/JCI200422422
- Chang, C. S., Lu, Y. J., Chang, H. H., Hsu, S. H., Kuo, P. H., Shieh, C. C., … Tsai, Y. S. (2016). Role of adiponectin gene variants, adipokines and hydrometry-based percent body fat in metabolically healthy and abnormal obesity. Obesity Research & Clinical Practice, In Press. doi:https://doi.org/10.1016/j.orcp.2016.05.003
- Croasdell, A., Duffney, P. F., Kim, N., Lacy, S. H., Sime, P. J., & Phipps, R. P. (2015). PPARγ and the innate immune system mediate the resolution of inflammation. Ppar Research, Article ID 54691. doi:https://doi.org/10.1155/2015/549691
- Deng, Q. C., Wang, L., Wei, F., Xie, B. J., Huang, F. H., Huang, W., … Xue, S. (2011). Functional properties of protein isolates, globulin and albumin extracted from Ginkgo biloba seeds. Food Chemistry, 124(4), 1458–1465. doi: https://doi.org/10.1016/j.foodchem.2010.07.108
- Eckel, R. H., Daniels, S. R., Jacobs, A. K., & Robertson, R. M. (2005). America’s children: A critical time for prevention. Circulation, 111, 1866–1868. doi: https://doi.org/10.1161/01.CIR.0000163655.15190.FB
- Folch, J., Lees, M., & Sloan-Stanley, G. H. (1957). A simple method for isolation and purification of total lipids from animal tissues. The Journal of Biological Chemistry, 226, 497–509.
- Hausen, B. M. (1998). The sensitizing capacity of ginkgolic acids in Guinea pigs. American Journal of Contact Dermatitis, 9(3), 146–148.
- Hazarika, A., Kalita, H., Kalita, M. C., & Devi, R. (2017). Withdrawal from high-carbohydrate, high-saturated-fat diet changes saturated fat distribution and improves hepatic low-density-lipoprotein receptor expression to ameliorate metabolic syndrome in rats. Nutrition, 38, 95–101. doi: https://doi.org/10.1016/j.nut.2017.01.005
- Hoshida, S., Nishida, M., Yamashita, N., Igarashi, J., Hori, M., Kamada, T., … Tada, M. (1996). Amelioration of severity of myocardial injury by a nitric oxide donor in rabbits fed a cholesterol-rich diet. Journal of the American College of Cardiology, 27(4), 902–909. doi: https://doi.org/10.1016/0735-1097(95)00538-2
- Imran, M., Nadeem, M., Saeed, F., Imran, A., Khan, M. R., & Khan, M. A. (2017). Immunomodulatory perspectives of potential biological spices with special reference to cancer and diabetes. Food and Agricultural Immunology, 28(4), 543–572. doi: https://doi.org/10.1080/09540105.2016.1259293
- Kanowski, S., Herrmann, W. M., Stephan, K., Wierich, W., & Horr, R. (1997). Proof of efficacy of the Ginkgo biloba special extract EGb 761 in outpatients suffering from mild to moderate primary degenerative dementia of the Alzheimer type or multiinfarct dementia. Phytomedicine, 4(1), 3–13. doi: https://doi.org/10.1016/S0944-7113(97)80021-9
- Khalifeh, M. S., Awaisheh, S. S., Alameri, O. H., & Hananeh, W. M. (2015). Small intestine mucosal immune system response to high-fat-high-cholesterol dietary supplementation in male rats. Food and Agricultural Immunology, 26(2), 293–304. doi: https://doi.org/10.1080/09540105.2014.914467
- Kobayashi, D., Yoshimura, D., Johno, A., Sasaki, K., & Wada, K. (2011). Toxicity of 4’- o-methylpyridoxine-5’- o-glucoside in Ginkgo biloba seeds. Food Chemistry, 126(3), 1198–1202. doi: https://doi.org/10.1016/j.foodchem.2010.12.001
- Koch, E., Jaggy, H., & Chatterjee, S. S. (2000). Evidence for immunotoxic effects of crude Ginkgo biloba L. Leaf extracts using the popliteal lymph node assay in the mouse. International Journal of Immunopharmacology, 22(3), 229–236. doi: https://doi.org/10.1016/S0192-0561(99)00080-6
- Kshatriya, S., Liu, K., Salah, A., Szombathy, T., Freeman, R. H., Reams, G. P., & Villarreal, D. (2011). Obesity hypertension: The regulatory role of leptin. International Journal of Hypertension, Article ID: 270624. doi:https://doi.org/10.4061/2011/270624
- Kunnen, S., & Van, E. M. (2012). Lecithin: Cholesterol acyltransferase: Old friend or foe in atherosclerosis?. Journal of Lipid Research, 53, 1783–1799. doi: https://doi.org/10.1194/jlr.R024513
- Lafontan, M., & Langin, D. (2009). Lipolysis and lipid mobilization in human adipose tissue. Progress in Lipid Research, 48(5), 275–297. doi: https://doi.org/10.1016/j.plipres.2009.05.001
- Lankford, T., Hardman, D., Dankmeyer, C., & Schmid, T. (2013). Analysis of state obesity legislation from 2001 to 2010. Journal of Public Health Management and Practice, 19, S114–S118. doi: https://doi.org/10.1097/PHH.0b013e3182847f2d
- Ling, Y., Jiang, J. J., Wu, B. J., & Gao, X. (2017). Serum triglyceride, high-density lipoprotein cholesterol, apolipoprotein B, and coronary heart disease in a Chinese population undergoing coronary angiography. Journal of Clinical Lipidology, 11(3), 646–656. doi: https://doi.org/10.1016/j.jacl.2017.02.017
- Liu, Z., Patil, I. Y., Jiang, T., Sancheti, H., Walsh, J. P., Stiles, B. L., & Cadenas, E. (2015). High-fat diet induces hepatic insulin resistance and impairment of synaptic plasticity. PLoS One, 10(5), e0128274. doi:https://doi.org/10.1371/journal.pone.0128274
- Li, H. T., Zhou, X. Q., Gao, P., Li, Q. Y., Li, H. S., Huang, R., & Wu, M. (2016). Inhibition of lipid oxidation in foods and feeds and hydroxyl radicaltreated fish erythrocytes: A comparative study of Ginkgo biloba leaves extracts and synthetic antioxidants. Animal Nutrition, 2, 234–241. doi: https://doi.org/10.1016/j.aninu.2016.04.007
- Majdi, M. A., Mohammadzadeh, N. A., Lotfi, H., Mahmoudi, R., Alipour, F. G., Shool, F., … Zarghami, N. (2017). Correlation of resistin serum level with fat mass and obesity-associated gene (FTO) rs9939609 polymorphism in obese women with type 2 diabetes. Diabetes & Metabolic Syndrome: Clinical Research & Reviews, In Press. doi:https://doi.org/10.1016/j.dsx.2017.05.004
- Miao, M., Jiang, H., Jiang, B., Cui, S. W., Jin, Z. Y., & Zhang, T. (2012). Structure and functional properties of starches from Chinese ginkgo (Ginkgo biloba L.) nuts. Food Research International, 49(1), 303–310. doi: https://doi.org/10.1016/j.foodres.2012.07.038
- Olefsky, J. M., & Glass, C. K. (2010). Macrophages, inflammation, and insulin resistance. Annual Review of Physiology, 72, 219–246. doi: https://doi.org/10.1146/annurev-physiol-021909-135846
- Postic, C., & Girard, J. (2008). Contribution of de novo fatty acid synthesis to hepatic steatosis and insulin resistance: Lessons from genetically engineered mice. Journal of Clinical Investigation, 118(3), 829–838. doi: https://doi.org/10.1172/JCI34275
- Qi, Q., Wang, J., Li, H., Yu, Z., Ye, X., Hu, F. B., & Lin, X. (2008). Associations of resistin with inflammatory and fibrinolytic markers, insulin resistance, and metabolic syndrome in middle-aged and older Chinese. European Journal of Endocrinology, 159(5), 585–593. doi:https://doi.org/10.1530/EJE-08-0427
- Reeves, P. G., Nielsen, F. H., & Fahey, G. C. (1993). AIN-93 purified diets for laboratory rodents: Final report of the American institute of nutrition ad hoc writing committee on the reformulation of the AIN-76 A rodent diet. Journal of Nutrition, 123(11), 1939–1951. doi: https://doi.org/10.1093/jn/123.11.1939
- Shen, R. L., Zhang, W. L., Dong, J. L., Ren, G. X., & Chen, M. (2015). Sorghum resistant starch reduces adiposity in high-fat diet-induced overweight and obese rats via mechanisms involving adipokines and intestinal flora. Food and Agricultural Immunology, 26(1), 120–130. doi: https://doi.org/10.1080/09540105.2013.876976
- Staels, B., Dallongeville, J., Auwerx, J., Schoonjans, K., Leitersdorf, E., & Fruchart, J. C. (1998). Mechanism of action of fibrates on lipid and lipoprotein metabolism. Circulation, 98, 2088–2093. doi: https://doi.org/10.1161/01.CIR.98.19.2088
- Te, S. K., Bourass, I., Sels, J. P., Driessen, A., Stockbrugger, R. W., & Koek, G. H. (2004). Non-alcoholic steatohepatitis: Review of a growing medical problem. European Journal of Internal Medicine, 15(1), 10–21. doi:https://doi.org/10.1016/j.ejim.2003.12.008
- Vargas-Robles, H., Rios, A., Arellano-Mendoza, M., Escalante, B. A., & Schnoor, M. (2015). Antioxidative diet supplementation reverses high-Fat diet-induced increases of cardiovascular risk factors in mice. Oxidative Medicine and Cellular Longevity, Article ID 467471, 2015, 1–9. doi:https://doi.org/10.1155/2015/467471
- Verhaeghe, N., Greve, O. De., & Annemans, L. (2016). The potential health and economic effect of a body mass Index decrease in the overweight and obese population in Belgium. Public Health, 134, 26–33. doi: https://doi.org/10.1016/j.puhe.2016.01.015
- Watts, G., & Burke, V. (1996). Lipid-lowering trials in the primary and secondary prevention of coronary heart disease: New evidence, implications and outstanding issues. Current Opinion in Lipidology, 7, 341–355. doi: https://doi.org/10.1097/00041433-199612000-00002
- Wooton, A. K., & Melchior, L. M. (2017). Obesity and type 2 diabetes in our youth: A recipe for cardiovascular disease. The Journal for Nurse Practitioners, 13(3), 222–227. doi: https://doi.org/10.1016/j.nurpra.2016.08.035
- Zhou, X. M., Qi, Y. L., & Chen, T. (2017). Long-term pre-treatment of antioxidant Ginkgo biloba extract EGb-761 attenuates cerebral-ischemia-induced neuronal damage in aged mice. Biomedicine & Pharmacotherapy, 85, 256–263. doi: https://doi.org/10.1016/j.biopha.2016.11.013