ABSTRACT
Food processing affects the bioavailability and immunoreactivity of allergens. However, there was a lack of the combined study of the influence of the processing and gastrointestinal digestion on peanut matrix. Digestibility of peanut matrices was assessed by using an in vitro digestion model and monitored by SDS-PAGE, immunoblotting with polyclonal antibody and serum from peanut-allergic patients. The IgE-binding ability of digested samples will be assessed by immunoassay. Peanut proteins were digested by pepsin after only 2 min which was accompanied by a loss of high molecular weight proteins and enrichment in polypeptides with molecular weight lower than 10 kDa. Ara h 1 and Ara h 3 were partly proteolysis, and Ara h 2/6 containing a digestion-resistant fragment. All soluble fractions after 120 min gastric digestion reduced a higher IgE-binding ability than that after 10 min. Our findings provide a more realistic picture, considering the role of food processing and food matrix.
Introduction
Peanut allergy is a common disease and the cause of fatal food-allergic reactions. According to the data of epidemiological studies, the disease currently affects 1–2% of children in the UK (Nicolaou et al., Citation2010). An increasing trend has been noticed in the USA (Husain & Schwartz, Citation2012). It was reported that there was a significant increase in peanut allergy in children, from 0.4% in 1997 to 0.8% in 2002 to 1.4% in 2008 (Sicherer & Sampson, Citation2014). It is estimated that the prevalence of peanut allergy has nearly doubled in Europe and America over the past two decades (Finkelman, Citation2010). Therefore it is obvious that greater knowledge of the underlying mechanisms and characteristics of food allergens is crucial for a better understanding of this disease.
Each food processing is known to have various effects on the allergenicity of proteins. The extent of the effects depends on food matrix, processing conditions and the biochemical and immunological properties of allergen (Sathe & Sharma, Citation2009; Verhoeckx et al., Citation2015). For example, epitope conformation may be modified by various thermal processing treatments leading to reducing, increasing or non-effect on IgE-binding (Bencharitiwong, van der Kleij, Koppelman, & Nowak-Wegrzyn, Citation2015; Rao et al., Citation2016; Shen et al., Citation2015). Several studies reported that compared to boiling, the roasting of whole peanuts could enhance the IgE-binding capacity of proteins may due to advanced glycation end adducts (Chung & Champagne, Citation2001). However, the animal study observed that roasted peanut did not significantly impact on sensitization potential in Brown Norway (Kroghsbo et al., Citation2014). Other treatments such as acid or enzyme hydrolysis of an allergenic protein may influence the degree of reduction in IgE-binding capacity of some peanut proteins (Koppelman, Hefle, Taylor, & de Jong, Citation2010; Toomer, Do, Pereira, & Williams, Citation2013).
Food intake is the major way for human exposure to a food allergen. Stability during digestion is considered another important feature in determining the allergenic potential of food (Fu, Citation2002). Approximately 70% of the immune cells distributed throughout the gastrointestinal tract. Poor digestibility contributes to maintain the structural integrity of food allergen, which may induce a high sensitizing potential (Moreno, Citation2007).
Several previous studies have investigated digestibility of peanut allergens by in vitro simulated digestion (Burks, Citation2008; Di Stasio et al., Citation2017; Hazebrouck et al., Citation2012). Most of that related to the effects of processing or gastrointestinal digestion on immunoreactivity focused on purified peanut allergens or peanut extracts. Regardless of different digestion model, Ara h 2/6 showed higher pepsin resistance compared to Ara h 1 and Ara h 3 (Apostolovic et al., Citation2016; Prodic et al., Citation2018a). However, the effect of thermal processing on the digestion and immunogenic potential of allergenic proteins in whole peanut matrix is poorly investigated so far. Therefore, the aim of the present study was to assess the bioavailability and IgE reactivity of thermally processed peanuts in the upper GI tract.
Materials and methods
Materials
Baisha peanut was selected from Liaoning Province of China and used for this study. Enzymes used for digestion studies and their activities as provided by the manufacturer are detailed in Supplementary Table 1. Chemicals used for preparing solutions such as simulated salivary fluid (SSF), simulated gastric fluid (SGF) and simulated intestinal fluid (SIF) were from Solarbio (Solarbio Science & Technology Co., Ltd, Beijing, China). NuPAGE Bis-Tris (12%) gel, Mark 12™ marker and SeeBlue™ prestained marker were also from Invitrogen. Anti-Ara h 1, -Ara h 2 and -Ara h 3 were produced by Biotechnology (Beijing, China). Anti-rabbit IgG alkaline phosphatase conjugate, rabbit anti-human IgE-peroxidase conjugate, Tween 20, Bovine serum albumin (BSA) and phosphate-buffered saline (PBS) were purchased from Sigma Chemical Co. (St. Louis, MO). Immobilon-P membrane was obtained from Millipore Corp (Bedford, MA). BeyoECL-Star substrates were purchased from Beyotime Biotechnology (Shanghai, China). Human sera with peanut allergy were obtained from the Fourth Hospital of Hebei, China. Peanut allergy was confirmed in these patients by a clinical team according to physical examination, skin prick testing and objective manifestations observed after peanut ingestion. The protocol was approved by the ethical committee of the China Agricultural University and written informed consent was obtained (approval number 2017001).
Preparation of thermally processed peanuts
Whole shelled peanuts were subjected to further processing in 50 g batches by either roasting or boiling. Roasting experiments were conducted in an oven for 20 min at 160°C. Boiling experiments were conducted for 20 min in water. Soluble protein extractions of raw peanut, roasted peanut and boiled peanut which as the control samples were performed for 12 h at 4°C using 50 mM Tris-HCl pH 8.8. The concentration of soluble protein was determined by BCA assay. All samples were aliquoted and stored at −20°C for subsequent allergic protein and IgE-binding analyses.
In vitro oral-gastric-intestinal digestion
Simulated oral-gastric-intestinal digestion of processed peanuts was performed according to the previously reported method (Minekus et al., Citation2014; Smith et al., Citation2015). The concentrations of electrolytes in stock solutions of SSF, SGF, SIF are presented in Table S2.
Briefly, in the oral phase, 500 μL SSF (pH 7) containing human salivary amylase and lysozyme were added to 0.5 g each sample (solid milled raw peanut, roasted peanut and boiled peanut) and mixed by hand for 2 min. The “chew” sample was chilled on ice and kept for protein analysis.
For oral-gastric phase, SGF (pH 3) with pepsin (final concentration 2000 U/mL) was added to each chew sample and the pH was adjusted to 3 the addition of 1 M HCl. All gastric samples were placed in a shaking incubator at 37°C, with a speed of 170 rpm and incubated for 0.3, 2, 10, 40, 70 and 120 min. Digestion was stopped by raising the pH to 7.5 by the addition of 1 M NaOH. The gastric samples were chilled on ice and kept for protein analysis.
Intestinal digestion was performed at 37°C by using the 10 min gastric digestion sample addition of same volume SIF either alone (Intestinal undigested control) or containing enzymes: trypsin (100 U/mL), chymotrypsin (25 U/mL), amylase (200 U/mL), lipase and colipase (2:1 molar ratio with lipase). Samples were incubated in a shaking incubator at 37°C at a speed of 170 rpm over the following time-course: 5, 15, 30 and 60 min. Proteolysis were stopped by addition of 0.3 mL 0.1 M PMSF/mL digest. All the samples were chilled on ice and kept for protein analysis. All the gastric and duodenal digestion samples were centrifuged at 16,000 g for 20 min at 4°C, the supernatant removed and stored the pellet at −20°C until required for further analysis.
Insoluble protein was extracted before use in SDS-PAGE and immunoblot analyses. 10 mg undigested materials or digestion pellets were extracted into 500 μL extraction buffer (7 M urea, 2 M thiourea, 2% CHAPS, 50 mM DTT, pH 8.8) by sonication (3 × 5 min, 60°C). Insoluble material remaining was separated by centrifugation at 16,000 g for 20 min and discarded. Protein concentration was determined using BCA assay (Solarbio Science & Technology Co., Ltd, Beijing, China).
SDS-PAGE
Digested proteins were analysed by SDS-PAGE (under reducing conditions). SDS-PAGE samples were prepared with 50 μL of supernatant and 10 μL of loading buffer (5 × SDS-PAGE Loading Buffer, Solarbio Science & Technology Co., Ltd, Beijing, China) were heated to 90°C for 5 min in a heat block. For electrophoresis separation, 20 μg protein samples were loaded into each well. Electrophoresis was performed for 35 min at 200 V for separating. The gels were stained with Coomassie brilliant blue (R-250) for 30 min and then destained overnight (about 12 h) in washing buffer (50% v/v Methanol, 10% v/v acetic acid). The grey values were visualized with the Electrophoresis Gel Imaging Analysis System (Multi Gauge V3.1, Fujitsu, Japan).
Immunoblotting
Unstained SDS-PAGE gels were soaked in transfer buffer (25 mM Tris-HCl, 125 mM glycine, 20% v/v Methanol) for 10 min. Electro-blotting was performed with nitrocellulose membrane using a MiniProtean III system (BioRad) at 300 mA for 80 min. Membranes were blocked 1 h at room temperature with wash buffer containing 5% w/v skimmed milk powder (blocking buffer). Membranes were then washed for 4 × 5 min with wash buffer and dipped into human plasma (1:10 diluted in blocking buffer) or anti-Ara h 1, anti-Ara h 1and anti-Ara h 3 (1:5000 diluted in blocking buffer) and incubated overnight at 4°C. The washing step was repeated before incubating membranes with secondary mouse-anti-human IgE labelled with or goat-anti-rabbit IgG labelled with Horseradish Peroxidase (HRP) (1:10,000 diluted in blocking buffer) for 1 h. Membranes were washed for 4 × 15 min with wash buffer and then incubated with a chemiluminescent BeyoECL-Star for HRP labelled secondary antibody. Images of the separated proteins were taken using a BIO-RAD GelDoc 2000 gel imaging system.
In-gel tryptic digestion
Polyacrylamide gel pieces were manually picked from the protein bands of interest and in-gel trypsin digested at 37°C for 18 h. Each sample was separated by capillary high-performance liquid chromatography and mass spectrometry was carried out by a Q-Exactive mass spectrometer (Thermo Scientific). The analysis was carried out by software Sequest and Proteome Discoverer (Thermo Scientific).
ELISA inhibition
The IgE-binding properties of the liquid phase from the digestion mixtures were analysed using an inhibition ELISA. Flat-bottom 96 well plates were coated with 100 μL per well of 10 μg/mL with raw peanut protein extract, and incubated overnight at 4°C in coating buffer (15 mM Na2CO3, 35 mM NaHCO3 pH 9.6). The plate was washed with ELISA wash buffer (0.05% Tween in 0.01 M PBS, pH 7.4) and blocked with 350 μL per well of blocking buffer (1% BSA, 0.01 M PBS, pH 7.4) for 2 h at 37°C. The plate was washed three times with ELISA wash buffer, and then incubated with 100 μL of each inhibitor (digested raw peanut, digested roasted peanut, digested boiled peanut, final concentration range 100–0.01 µg/mL) and 100 μL diluted pooled sera from peanut-sensitized patients (1:50) in ELISA blocking buffer overnight at 4°C. Both inhibitors and patient serum were diluted with blocking buffer to twice the desired concentration as the concentration would be half when mixed together. The plate was washed with ELISA wash buffer four times to remove any unbound primary antibody, and incubated with diluted 1:10,000 HRP conjugated secondary antibody in ELISA blocking buffer for 1 h at 37°C. After removing any unbound secondary antibody by washing with ELISA wash buffer four times, the plate was incubated with 100 μL per well of TMB substrate for 30 min at room temperature in the dark. After 15 min, the enzymatic reactions were stopped by adding 100 μL per well of 1 M HCl.
Statistical analysis
Statistical analyses were performed using GraphPad Prism 7.01 (GraphPad Software, San Diego, CA, USA).
Results
In vitro digestibility of peanut proteins
Digestibility of raw peanut during simulated oral-gastric-intestinal digestion at physiological condition was assessed at the beginning (). The regions of 67, 35–45 and 14–20 kDa which corresponding to Ara h 1, 3 and 2/6 can be clearly recognized in the gel of non-digested raw peanut. The soluble fraction of chew sample from non-processed peanuts was similar profile as raw peanut extract ((A)). (B) showed that peanut proteins were digested by pepsin after only 2 min which was accompanied by a loss of high molecular weight proteins and enrichment in polypeptides with molecular weight lower than 10 kDa. Although the bands around 67 and 38 kDa which corresponding to Ara h 1 and Ara h 3 acidic were degraded, they seemed to be proteolyzed mainly to other smaller forms, such as 55 kDa form and 23 kDa form (arrow marked in (B)). We analysed the band around 23 kDa, which was increased significantly with time on, using Mass spectrometry. The result () indicated it belonged to Ara h 3 isoforms (Q0GM57, Q647H4). In contrast, Mr ∼14 and ∼9 kDa polypeptides which corresponding to Ara h 2/6 were clearly evident even for 120 min of gastric digestion. Furthermore, still many intact peanut proteins were observed in insoluble fraction even after 120 min ((C)).
Figure 1. SDS-PAGE analysis of simulated oral (A), oral-gastric (B,C) and oral-gastric-intestinal (D,E) digestion of raw peanut. R – undigested raw peanut extract; C – chew sample; G – no enzyme gastric control; I – no enzyme intestinal control; Panels B and C Lanes 1–6 are gastric digestion time points – 0.3, 2, 10, 40, 70 and 120 min; Panels D and E Lanes 1–4 are intestinal digestion time points – 5, 15, 30 and 120 min.
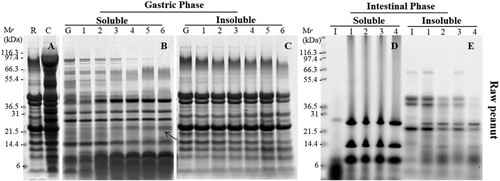
Table 1. Proteins identified in gels by tryptic digestion (bands annotated in (B ) and 3(B)).
Subsequently, the effect of two processed peanuts on allergen digestibility was investigated. Compared with non-digested raw peanut, non-digested roasted peanut showed weaker bands around 67 and 50 kDa, but more aggregations at high molecular weight as Maillard reaction occurred during heat processing ((A)). It seemed no differences of protein profile between non-digested boiled peanut and non-digested raw peanut ((A)). However, when SSF with amylase treated with roasted and boiled peanuts, less peanut proteins expressed in gels compared to raw peanut chew sample. That may because that heat unstable protein has been degrade and more aggregations cannot be release to soluble fractions.
Figure 2. SDS-PAGE analysis of simulated oral (A), oral-gastric (B,C) and oral-gastric-intestinal (D,E) digestion of roasted peanut. R – undigested roasted peanut extract; C – chew sample; G – no enzyme gastric control; I – no enzyme intestinal control; Panels B and C Lanes 1–6 are gastric digestion time points – 0.3, 2, 10, 40, 70 and 120 min; Panels D and E Lanes 1–4 are intestinal digestion time points – 5, 15, 30 and 120 min.
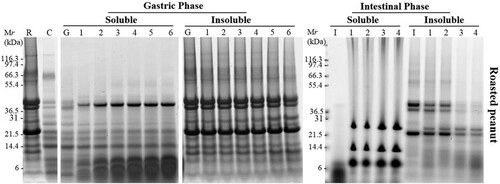
Figure 3. SDS-PAGE analysis of simulated oral (A), oral-gastric (B,C) and oral-gastric-intestinal (D,E) digestion of boiled peanut. R – undigested boiled peanut extract; C – chew sample; G – no enzyme gastric control; I – no enzyme intestinal control; Panels B and C Lanes 1–6 are gastric digestion time points – 0.3, 2, 10, 40, 70 and 120 min; Panels D and E Lanes 1–4 are intestinal digestion time points – 5, 15, 30 and 120 min.
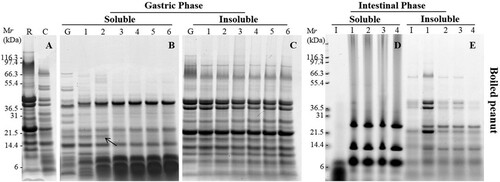
During gastric digestion, the soluble fraction for bands at 67 kDa and 38 kDa corresponding to Ara h 1 and acidic Ara h 3 were rapidly digested. Bands around 14–20 kDa which supposed to be Ara h 2/6 were poorly digested and was still present in the soluble fraction after 120 min of exposure to pepsin in all processed peanuts ((B) and 3(B)) . Low molecular weight proteins (<10 kDa) and digestion fragments were resistant to pepsin digestion. The intensity of bands around 20 kDa after digestion of boiled peanut was degraded more quickly compared to roasted peanuts indicating a slightly faster rate of digestion. The band around 20 kDa (arrow marked in (B)) was hard to seen after digestion for 10 min. We analysed this band from gels using Mass spectrometry () and the result indicated this was isoforms (Q6PSU2) of Ara h 2. Furthermore, significantly more non-digested proteins especially aggregations were observed in an insoluble fraction of roasted peanuts compared to boiled peanuts ((C) and 3(C)).
Samples obtained after 10 min of gastric digestion were submitted to duodenal digestion. Due to the fact that most of the peanut proteins were digested during gastric digestion, SDS-PAGE (after duodenal digestion) of soluble fractions did not show the presence of peanut proteins in any of non-processed and processed peanuts ((D), 2(D), and (D)). The duodenal digestion phase consists of many enzymes, including amylase, trypsin, chymotrypsin, lipase and colipase so the bands that were clear on the gel corresponded to these enzymes. In the insoluble fraction, a very weak band that belonged to Ara h 1 was rapidly digested after 15 min of digestion of all peanut matrices. Acidic and basic Ara h 3 subunits were digested after 0.3 min whereas they were still visible after 60 min of digestion in three matrices especially in raw peanut. Ara h 2/6 was present in the insoluble fraction from peanut flour whereas their abundance was weaker in the insoluble fraction from roasted peanut and boiled peanut.
Immunoblotting analysis of peanut allergens from different processed peanuts
The focus of the further analysis was on the soluble fraction since this is the material presented to epithelial surfaces and likely to play a key role in elicitation of reactions; it traduce bioaccessible allergens or fragments derived thereof. Identification of allergen profiles in both soluble fractions after 10 and 120 min of digestions was achieved using polyclonal rabbit anti-Ara h 1, Ara h 3 and Ara h 2/6 antibodies in immunoblotting (). Ara h 1 can be only detected in non-digested peanut and its isoforms in digested raw peanuts. Ara h 3 were not detected in soluble fraction after gastric digestion of all peanuts. Ara h 2/6 was determined in soluble fraction after digestions but the intensity of the band (mainly at 14 kDa) was higher after digestion for 10 min than for 120 min indicating that Ara h 2/6 can be slightly digested. However, the numerous digestion-resistant fragments belong to Ara h 2/6 still present, even after intestinal digestion.
Figure 4. Immunoblotting analysis of the soluble fraction of digested peanut and processing peanut. Immunoblots were developed using polyclonal antibody preparations to Ara h1, Ara h3 and Ara h 2/6. The order of the samples in lane 1: non-digested raw peanut; lanes 2, 4 and 6: soluble fractions after 10 min gastric digestion of raw peanut, roasted peanut and boiled peanut, respectively; lanes 3, 5 and 7: soluble fractions after 120 min gastric digestion of raw peanut, roasted peanut and boiled peanut, respectively.
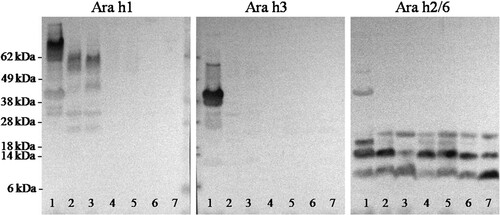
To determine whether digestion changed immunoreactivity of proteolytic fragments of allergenic proteins, soluble fractions of digestion products obtained from non-processed peanut, roasted peanut and boiled peanut after 10 and 120 min of oral-gastric digestion were analysed by IgE-immunoblotting using plasma from two peanut-allergic patients (). In the non-digested control sample (lane 1), bands around 67, 38 and 13–22 kDa were identified as IgE-reactive in patient 1 who was the highly sensitized to multiple allergens. In the soluble fraction of processed peanut mainly Ara h 2/6/7 was. Boiled peanut showed the lowest immune-reactive compare to raw and roasted peanut. clearly shown the presence of peptides lower than 8 kDa after digestion of all digested peanuts, but they were not IgE reactive (). Similar allergen profiles were detected when plasma was used from patients 2.
Figure 5. IgE immunoreactivity of digested peanut and processing peanut using plasma from peanut-allergic patients. The order of the samples in lane 1: non-digested raw peanut; lanes 2, 4 and 6: soluble fractions after 10 min gastric digestion of raw peanut, roasted peanut and boiled peanut, respectively; lanes 3, 5 and 7: soluble fractions after 120 min gastric digestion of raw peanut, roasted peanut and boiled peanut, respectively.
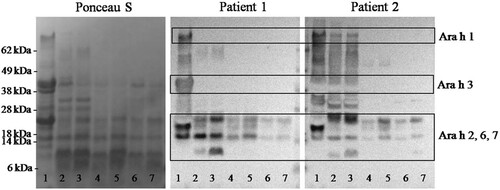
IgE-binding analysis of different digests
Inhibition ELISAs were also performed to assess the binding capacity of digested peanuts compared to non-digested raw peanut extract. The (a) showed that binding capacity was reduced after digestion, and processed peanut even lower than a raw peanut. The largest decreasing in binding capacity was observed in the extensively digested of boiled peanuts. The IC50 values were determined as 2.61, 4.44 and 5.56 μg/mL for the raw, roasted and boiled peanuts after digested for 10 min. When the raw, roasted and boiled peanuts were digested for 120 min, the IC50 values were changed to 3.43, 4.83 and 8.47 μg/mL.
Discussion
Most of the food we consume in our society have been processed. These process-induced food protein conformational changes may further affect the digestion/absorption of proteins/peptides and their immune function (Netting, Makrides, Gold, Quinn, & Penttila, Citation2013; Rahaman, Vasiljevic, & Ramchandran, Citation2016; Teodorowicz, van Neerven, & Savelkoul, Citation2017). Furthermore, the sensitivity of food proteins to enzymatic hydrolysis appears to be an important factor determining their allergenicity (Bogh & Madsen, Citation2016; Peram, Loveday, Ye, & Singh, Citation2013). However, the effect of thermal processing on the digestion and immunogenic potential of allergenic proteins in whole peanut matrix is poorly investigated so far. Previous literature has demonstrated that roasting increases and boiling decreases the IgE-binding capacity of peanut (Beyer et al., Citation2001; Turner et al., Citation2014). Purified Ara h 1 and Ara h 2 were more resistant to digestive enzymes and heat than the untreated allergens (Maleki, Chung, Champagne, & Raufman, Citation2003). Compared to these existing works (Mandalari et al., Citation2014; Moreno, Citation2007; Prodic et al., Citation2018b), the whole peanut matrix which contains around 20% protein, 50% lipids and dietary fibre may affect allergen conformation during heating and digestibility during the digestion process.
It can be seen in the comparison of thermally processed peanuts that not all food allergens are resistant to heating. Because of allergen degradation or aggregation, the simulated salivary or SGF could not release as much protein as raw peanut did. Roasted and boiled peanut proteins in the soluble fractions were digested very fast that Ara h 1 and Ara h 3 can be hardly seen in the gels, even not detected by polyclonal antibodies. However, Ara h 1, Ara h 3 or other allergens which broken down by gastric-intestinal digestion did not mean them to lose allergenic potential in the absence of the intact protein. The Ara h 3 isoforms were even identified in raw and roasted peanut after 120 min digestion processing by mass spectrometry. This finding coincides with the previous report that Ara h 3 contains a core region predicted to be preserved upon digestive enzyme attack in the gastrointestinal tract (Koppelman et al., Citation2010; Prodic et al., Citation2018a).
Binding of IgE antibodies is essential to elicit an allergic reaction. The degree of protein hydrolysis increased with digestion time on. All soluble fractions after 120 min gastric digestion reduced a higher IgE-binding ability than that after 10 min. Boiled peanuts were more digestible than raw and roasted peanuts which we can conclude from SDS-PAGE result. After 2 h digestion, the allergen species and content were less than raw and roasted peanuts. This phenomenon could help us explain the IgE-binding capacity of boiled peanut was reduced after digestion. In addition, it has been found that boiling decreased the allergenicity of Ara h 2 due to the structural modification that can affect IgE-binding epitopes. Maillard reaction is usually considered as one of the reasons for increased sensitization of roasted peanut (Teodorowicz et al., Citation2017). However, the aggregations induced by Maillard reaction were difficult to gastric digest, which can be seen in the insoluble fraction. Proteins in the soluble fractions digested into low molecular weight peptides which lead to its lower IgE-binding capacity than that in raw peanut.
Although peanut matrix was digested rapidly, there was still a great deal of intact and partly proteolysis allergens even after gastric-intestinal digestion. This would provide a source of peanut allergens for the gastrointestinal mucosal immune system. Compared with the results of in vitro digestion of purified protein under similar conditions, the digestibility of protein in the real food matrix decreased significantly. Processed peanuts, especially boiled peanut, could reduce this effect to a certain extent. Our findings provide a more realistic picture, considering the role of food processing and food matrix.
Acknowledgments
H.R. designed the study, oversaw all aspects of the research, analysed and interpreted the data; W.X. principal investigator, conceived research project and obtained funding. All authors were involved in revising the manuscript and all read and approved the final manuscript.
Disclosure statement
No potential conflict of interest was reported by the authors.
Additional information
Funding
References
- Apostolovic, D., Stanic-Vucinic, D., de Jongh, H. H., de Jong, G. A., Mihailovic, J., Radosavljevic, J., … Koppelman, S. J. (2016). Conformational stability of digestion-resistant peptides of peanut conglutins reveals the molecular basis of their allergenicity. Scientific Reports, 6, 29249. doi:10.1038/srep29249 Retrieved from http://www.ncbi.nlm.nih.gov/pubmed/27377129
- Bencharitiwong, R., van der Kleij, H. P., Koppelman, S. J., & Nowak-Wegrzyn, A. (2015). Effect of chemical modifications on allergenic potency of peanut proteins. Allergy and Asthma Proceedings, 36(3), 185–191. doi:10.2500/aap.2015.36.3840 Retrieved from http://www.ncbi.nlm.nih.gov/pubmed/25976435
- Beyer, K., Morrowa, E., Li, X. M., Bardina, L., Bannon, G. A., Burks, A. W., & Sampson, H. A. (2001). Effects of cooking methods on peanut allergenicity. Journal of Allergy and Clinical Immunology, 107(6), 1077–1081. doi:10.1067/mai.2001.115480 Retrieved from http://www.ncbi.nlm.nih.gov/pubmed/11398088
- Bogh, K. L., & Madsen, C. B. (2016). Food allergens: Is there a correlation between stability to digestion and allergenicity? Critical Reviews in Food Science and Nutrition, 56(9), 1545–1567. doi:10.1080/10408398.2013.779569 Retrieved from http://www.ncbi.nlm.nih.gov/pubmed/25607526
- Burks, A. W. (2008). Peanut allergy. The Lancet, 371(9623), 1538–1546. doi: https://doi.org/10.1016/S0140-6736(08)60659-5
- Chung, S., & Champagne, E. T. (2001). Association of end-product adducts with increased IgE binding of roasted peanuts. Journal of Agricultural and Food Chemistry, 49, 3911–3916. doi: https://doi.org/10.1021/jf001186o
- Di Stasio, L., Picariello, G., Mongiello, M., Nocerino, R., Berni Canani, R., Bavaro, S., … Mamone, G. (2017). Peanut digestome: Identification of digestion resistant IgE binding peptides. Food and Chemical Toxicology, 107(Pt A), 88–98. doi:10.1016/j.fct.2017.06.029 Retrieved from http://www.ncbi.nlm.nih.gov/pubmed/28634114
- Finkelman, F. D. (2010). Peanut allergy and anaphylaxis. Current Opinion in Immunology, 22(6), 783–788. doi:10.1016/j.coi.2010.10.005 Retrieved from http://www.ncbi.nlm.nih.gov/pubmed/21051210
- Fu, T. (2002). Digestion stability as a criterion for protein allergenicity assessment. Annals of the New York Academy of Sciences, 964, 99–110. doi: https://doi.org/10.1111/j.1749-6632.2002.tb04135.x
- Hazebrouck, S., Guillon, B., Drumare, M. F., Paty, E., Wal, J. M., & Bernard, H. (2012). Trypsin resistance of the major peanut allergen Ara h 6 and allergenicity of the digestion products are abolished after selective disruption of disulfide bonds. Molecular Nutrition & Food Research, 56(4), 548–557. doi:10.1002/mnfr.201100614 Retrieved from http://www.ncbi.nlm.nih.gov/pubmed/22495983
- Husain, Z., & Schwartz, R. A. (2012). Peanut allergy: An increasingly common life-threatening disorder. Journal of the American Academy of Dermatology, 66(1), 136–143. doi:10.1016/j.jaad.2011.02.031 Retrieved from http://www.ncbi.nlm.nih.gov/pubmed/21820205
- Koppelman, S. J., Hefle, S. L., Taylor, S. L., & de Jong, G. A. (2010). Digestion of peanut allergens Ara h 1, Ara h 2, Ara h 3, and Ara h 6: A comparative in vitro study and partial characterization of digestion-resistant peptides. Molecular Nutrition & Food Research, 54(12), 1711–1721. doi:10.1002/mnfr.201000011 Retrieved from http://www.ncbi.nlm.nih.gov/pubmed/20603832
- Kroghsbo, S., Rigby, N. M., Johnson, P. E., Adel-Patient, K., Bogh, K. L., Salt, L. J., … Dileepan, K. N. (2014). Assessment of the sensitizing potential of processed peanut proteins in brown Norway rats: Roasting does not enhance allergenicity. PLoS One, 9(5), e96475. doi:10.1371/journal.pone.0096475 Retrieved from http://www.ncbi.nlm.nih.gov/pubmed/24805813
- Maleki, S. J., Chung, S., Champagne, E. T., & Raufman, J. (2003). The effects of roasting on the allergenic properties of peanut proteins. Journal of Allergy and Clinical Immunology, 112, 190–195. doi:10.067/mai.2000.109620 doi: https://doi.org/10.1067/mai.2003.1551
- Mandalari, G., Rigby, N. M., Bisignano, C., Lo Curto, R. B., Mulholland, F., Su, M., … Sathe, S. K. (2014). Effect of food matrix and processing on release of almond protein during simulated digestion. LWT - Food Science and Technology, 59(1), 439–447. doi: https://doi.org/10.1016/j.lwt.2014.05.005
- Minekus, M., Alminger, M., Alvito, P., Ballance, S., Bohn, T., Bourlieu, C., … Brodkorb, A. (2014). A standardised static in vitro digestion method suitable for food – an international consensus. Food & Function, 5(6), 1113–1124. doi: https://doi.org/10.1039/C3FO60702J
- Moreno, F. J. (2007). Gastrointestinal digestion of food allergens: Effect on their allergenicity. Biomedicine & Pharmacotherapy, 61(1), 50–60. doi:10.1016/j.biopha.2006.10.005 Retrieved from http://www.ncbi.nlm.nih.gov/pubmed/17188456
- Netting, M., Makrides, M., Gold, M., Quinn, P., & Penttila, I. (2013). Heated allergens and induction of tolerance in food allergic children. Nutrients, 5(6), 2028–2046. doi:10.3390/nu5062028 Retrieved from http://www.ncbi.nlm.nih.gov/pubmed/23739144
- Nicolaou, N., Poorafshar, M., Murray, C., Simpson, A., Winell, H., Kerry, G., … Custovic, A. (2010). Allergy or tolerance in children sensitized to peanut: Prevalence and differentiation using component-resolved diagnostics. Journal of Allergy and Clinical Immunology, 125(1), 191–197.e13. doi: https://doi.org/10.1016/j.jaci.2009.10.008
- Peram, M. R., Loveday, S. M., Ye, A., & Singh, H. (2013). In vitro gastric digestion of heat-induced aggregates of β-lactoglobulin. Journal of Dairy Science, 96(1), 63–74. doi:10.3168/jds.2012-5896 Retrieved from http://www.sciencedirect.com/science/article/pii/S0022030212007849
- Prodic, I., Stanic-Vucinic, D., Apostolovic, D., Mihailovic, J., Radibratovic, M., Radosavljevic, J., … Cirkovic Velickovic, T. (2018a). Influence of peanut matrix on stability of allergens in gastric-simulated digesta: 2S albumins are main contributors to the IgE reactivity of short digestion-resistant peptides. Clinical and Experimental Allergy. doi:10.1111/cea.13113 Retrieved from http://www.ncbi.nlm.nih.gov/pubmed/29412488
- Prodic, I., Stanic-Vucinic, D., Apostolovic, D., Mihailovic, J., Radibratovic, M., Radosavljevic, J., … Cirkovic Velickovic, T. (2018b). Influence of peanut matrix on stability of allergens in gastric-simulated digesta: 2S albumins are main contributors to the IgE reactivity of short digestion-resistant peptides. Clinical and Experimental Allergy, 48(6), 731–740. doi:10.1111/cea.13113 Retrieved from http://www.ncbi.nlm.nih.gov/pubmed/29412488
- Rahaman, T., Vasiljevic, T., & Ramchandran, L. (2016). Effect of processing on conformational changes of food proteins related to allergenicity. Trends in Food Science & Technology, 49, 24–34. doi: https://doi.org/10.1016/j.tifs.2016.01.001
- Rao, H., Tian, Y., Tao, S., Tang, J., Li, X., & Xue, W.-T. (2016). Key factors affecting the immunoreactivity of roasted and boiled peanuts: Temperature and water. LWT - Food Science and Technology, 72, 492–500. doi: https://doi.org/10.1016/j.lwt.2016.05.014
- Sathe, S. K., & Sharma, G. M. (2009). Effects of food processing on food allergens. Molecular Nutrition & Food Research, 53(8), 970–978. doi:10.1002/mnfr.200800194 Retrieved from http://www.ncbi.nlm.nih.gov/pubmed/19603400
- Shen, L.-L., Zhu, Q.-Q., Huang, F.-W., Xu, H., Wu, X.-L., Xiao, H.-F., … Liu, Z.-G. (2015). Effect of heat treatment on structure and immunogenicity of recombinant peanut protein Ara h 2.01. LWT - Food Science and Technology, 60(2), 964–969. doi: https://doi.org/10.1016/j.lwt.2014.10.044
- Sicherer, S. H., & Sampson, H. A. (2014). Food allergy: Epidemiology, pathogenesis, diagnosis, and treatment. Journal of Allergy and Clinical Immunology, 133(2), 291–307.e5. doi:10.1016/j.jaci.2013.11.020 Retrieved from http://www.ncbi.nlm.nih.gov/pubmed/24388012
- Smith, F., Pan, X., Bellido, V., Toole, G. A., Gates, F. K., Wickham, M. S., … Mills, E. N. (2015). Digestibility of gluten proteins is reduced by baking and enhanced by starch digestion. Molecular Nutrition & Food Research, 59(10), 2034–2043. doi:10.1002/mnfr.201500262 Retrieved from http://www.ncbi.nlm.nih.gov/pubmed/26202208
- Teodorowicz, M., van Neerven, J., & Savelkoul, H. (2017). Food processing: The influence of the maillard reaction on immunogenicity and allergenicity of food proteins. Nutrients, 9(8), 835. doi:10.3390/nu9080835 Retrieved from http://www.ncbi.nlm.nih.gov/pubmed/28777346
- Toomer, O. T., Do, A., Pereira, M., & Williams, K. (2013). Effect of simulated gastric and intestinal digestion on temporal stability and immunoreactivity of peanut, almond, and pine nut protein allergens. Journal of Agricultural and Food Chemistry, 61(24), 5903–5913. doi:10.1021/jf400953q Retrieved from http://www.ncbi.nlm.nih.gov/pubmed/23742710
- Turner, P. J., Mehr, S., Sayers, R., Wong, M., Shamji, M. H., Campbell, D. E., & Mills, E. N. (2014). Loss of allergenic proteins during boiling explains tolerance to boiled peanut in peanut allergy. Journal of Allergy and Clinical Immunology, 134(3), 751–753. doi:10.1016/j.jaci.2014.06.016 Retrieved from http://www.ncbi.nlm.nih.gov/pubmed/25065723
- Verhoeckx, K. C., Vissers, Y. M., Baumert, J. L., Faludi, R., Feys, M., Flanagan, S., … Kimber, I. (2015). Food processing and allergenicity. Food and Chemical Toxicology, 80, 223–240. doi:10.1016/j.fct.2015.03.005 Retrieved from http://www.ncbi.nlm.nih.gov/pubmed/25778347