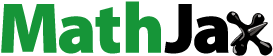
ABSTRACT
In this study, a typical Chinese medicine injection (Mai-luo-ning, MLN) was chosen to study the transformation possibility of its by-products (polysaccharides). What’s more, the basic properties of polysaccharides were also detected and its immunoprotect and anti-inflammatory activity were both proved in vivo and in vitro. The experiment determined that MLN-1 significantly increased the level of anti-inflammatory-related factors and the number of lymphocytes. Acute oral toxicity study established that MLN-1 has a non-toxic effect. The present work successfully showed that MLN-1 has the potential to be developed as a food additive.
Introduction
Polysaccharides are important biological macromolecules. They are usually extracted from plants, animals and microorganisms and are good sources of dietary fibre and prebiotics for immunoregulatory, antioxidative, and anti-tumor activities. Recent studies also found that polysaccharides can affect human health with metabolization and ferment of the gut microbiota. Besides, many plant-derived polysaccharides have drawn attention on their immunoregulatory, non- or less toxicity and few side effects of synthetic drugs (Chen et al., Citation2018; Hao & Zhao, Citation2016; Park et al., Citation2019; Qiao et al., Citation2017). In recent years, the number of mechanism (genomics, proteomics and metabolomics) studies of plant-derived polysaccharides on how to affect human health is increasing.
Mai-luo-ning (MLN), a Traditional Chinese Medicine (TCM) decoction (Peng et al., Citation2012), is made from the Si-miao-yong-an decoction (Yan Fang Xin Bian, volume two, Xiang-ao Bao). MLN consists of four herbs in TCM: Dendrobium nobile Lindl, Achyranthes bidentata, Honeysuckle and Scrophulariaceae. According to the traditional theory, MLN has been widely and efficiently used to treat thrombotic angitis, cerebral thrombosis and its sequelae (Yang et al., Citation2015).
Recent studies have shown that polysaccharides from the above four have immunomodulatory activity. A neutral heteropolysaccharide (DOP-1-1) purified from Dendrobium officinal could significantly increase the levels of TNF-γ and IL-1β in vitro (He et al., Citation2016). A polysaccharide (DTTPS) separated from a new Dendrobium species was able to substantially increase the number of splenic natural killer (NK) cells and enhance macrophage phagocytosis (Yang, Hsieh, Wen, Chiu, & Lin, Citation2017). Moreover, the multiple biological actives of Achyranthes plants could attribute to its various ingredients, including polysaccharides, polypeptides, ketosteroids and triterpenoid saponins (He et al., Citation2017). As other evidence shows that, polysaccharides from the flower buds of Lonicera japonica possess anti-inflammatory activity (Tian, Che, Ha, Wei, & Zheng, Citation2012). The improvement of polysaccharides as the by-products of MLN on immunoprotect and their structures remain unclear.
Thus, the present study aimed to characterize polysaccharides from the MLN, investigate its immunoprotect potential in vivo, and determine its anti-inflammatory activity in vitro. It laid the confirmatory groundwork for future exploration in the food industry.
Materials and methods
Chemicals and materials
RAW 264.7 cell line and Dextran polymers standards were purchased from Chinese Academy of Sciences (Shanghai, China). Dimethyl sulfoxide (DMSO), cyclophosphamide (CY), lipopolysaccharide (LPS), FITC-fluorescent microspheres (2 µm) and concanavalin A (ConA) were purchased from Sigma-Aldrich (St. Louis, MO, USA). Cell counting kit-8 (CCK-8) was purchased from Vazyme Biotech Co., Ltd (Nanjing, China). ELISA Kits for mouse TNF-α, IL-2, IL-6, IFN-γ, SIgA, IgA, IgG and IgM were purchased from Multisciences (Lianke) Biotech Co. Ltd (Hangzhou, China). Nitric oxide (NO) assay kit was purchased from Biyotime Biotechnology (Shanghai, China). D. nobile Lindl, A. bidentata, Honeysuckle and Scrophulariaceae were purchased from Huizhongzhou Ltd. (Bozhou, China).
Preparation of MLN-1
The extraction and purification procedures of total polysaccharide (simulated industrial production) were as follow: Take 300 g of each of four medicinal Chinese herbs in a round-bottomed flask with 9.6 L water and soaked 1 h before heated. Then, heated to reflux them 2.5 h and collected water extract. Repeat the above steps (start from add water) again and combined all water extracts. Next, concentrated water extracts to a density of 1.2 g/mL and added ethanol to a final concentration of 75% (v/v) for 24 h. Then, collected rough sediment and washed by ethanol until the supernatant was clear and colourless. Last, evaporated the surface of ethanol and have a vacuum freeze-drying for storage.
The extracted polysaccharide solution was pretreated through a membrane with the pore size of 0.45 μm to avoid fouling of the ultrafiltration membranes. MLN powder was dissolved at 20 mg/mL with distilled water and passed through a hollow fibre ultrafiltration membrane of which intercepts molecules above 300 KDa (140 cm2, GE Healthcare, USA). Then the total polysaccharide under 300 KDa named MLN-1 was obtained.
The contents of polysaccharides, protein, pectin and tannin in MLN and MLN-1 were detected by the phenol-sulfuric acid method, Coomassie brilliant blue method, calcium pectate method and hide-powder method, respectively (Zhang et al., Citation2012). The content of pigment was measured by ultraviolet spectrophotometry at 450 nm. By comparing the weight before and after passing the membrane, the yield was obtained.
Determination of molecular weight
The molecular weight of MLN-1 was determined by high-performance liquid chromatography – evaporative light scattering detector (HPLC-ELSD) on Agilent 1260 system (Agilent, USA) was applied to a TSK-G4000 PWXL (Tosoh Biosep, Japan) column (7.8 mm ID × 30.0 cm) and eluted by 20 mM ammonium acetate for 30 min (40°C evaporator temperature, 30°C nebulizer temperature and 0.7 standard litres per minute nitrogen flow rate). Dextran polymers of 0.180, 4.320, 12.600, 60.600, 110.000, 289.000 and 500.000 KDa were used as calibration standards. With a standard curve, the molecular weight of polysaccharide was detected (Zheng et al., Citation2017).
Fourier transmission infrared spectral analysis
Fourier transform infrared spectroscopy (FT-IR) analysis of MLN-1 in a wavenumber range of 4000–400 cm−1 was recorded by the KBr pellet method with a Tensor 37 FT-IR Spectrometer (Bruker Optics, Ettlingen Germany).
Animal
Male BALB/c mice aged eight weeks with the weight of 18–22 g were obtained from Yangzhou University Comparative Medical Center (Yangzhou, China). The mice were kept in conventional rooms with controlled photoperiod (12:12 L:D, lights on at 07:00 h, approx. 200 lux at 1 m above the floor), temperature (20–22°C), relative humidity (50–60%) and ventilation (15 air changes h−1). All procedures involving animal care were approved by the Ethics Committee of the Chinese Academy of Agricultural Sciences.
Cell culture
RAW264.7, spleen and murine Peyer’s patch (MPP) cells were cultured in RPMI 1640 containing 10% heat-inactivated foetal bovine serum, 100 U/mL penicillin and 100 U/mL streptomycin. Cells were kept at 37°C with 5% CO2 in a fully humid atmosphere. For the measurement of NO and cell viability, 1 × 105 cells were seeded per well in 96-well plate.
Lymphocyte proliferation assay in vitro
According to the method of Chandrashekar (Chandrashekar, Prashanth, & Venkatesh, Citation2011), spleens were aseptically removed from mice and dissociated into single cell suspension at a final density of 5 × 106 cells/mL. One hundred microlitres of cell suspension were seeded into a 96-well plate in each well and then the ConA (1 μg/mL), LPS (2 μg/mL) or MLN-1 (final concentrations at 800, 400, 200, 100, 50, 25, 12.5, 6.25, 3.125 and 1.0625 μg/mL) were added. The splenic cells were cultured for 24 h in the above condition, and then further incubated for 2 h with 10 μL CCK8 per well (Kumar, Prashanth, & Venkatesh, Citation2015). The absorbance was measured at 450 nm by a microplate reader (Thermo Multiskan MK3, USA).
Cell viability assay and secretion NO, acid phosphatase, TNF-α, IFN-γ, and IL-6
RAW264.7 cells were seeded into a 96-well plate with different concentrations of MLN-1 (final concentrations at 100, 50, 25, 12.5, 6.25, 3.125 and 1.0625 μg/mL) to measure the safe concentration. After cultivation for 24 h, CCK8 was added and the absorbance at 450 nm was measured. Repeat above cell culture steps again, 100 μL of supernatant was collected for cytokines (TNF-α, IFN-γ and IL-6) analysis by ELISA kits. Meanwhile, 50 μL of supernatant was translated into another 96-well plate and mixed with 50 μL of Griess I and 50 μL of Griess II reagent. After incubation for 10 min at room temperature, production of NO was determined by measuring the absorbance at 540 nm. And the NO production was calculated according to the regression equation of the standard curve.
After removing all supernatant, 100 μL of para-Nitrophenylphosphate (PNPP, 6.7 mmoL/mL) was added into the 96-well plate and cultured for 30 min (Yu, Kong, Zhang, Sun, & Chen, Citation2016). One hundred microlitres of NaOH (0.1 moL/L) were added into each well and the absorbance at 405 nm was measured.
Assessment of phagocytic activity
Phagocytosis of macrophages was assessed by co-culture of cells with fluorescent beads following the manufacturer’s instructions. Briefly, RAW264.7 cells were pretreated with MLN-1 or LPS for 24 h and then the FITC-labeled fluorescent microspheres (diameter: 2 μm) pre-incubated with 1% BSA for 30 min in dark were added into each well, and a negative group was added 1% BSA. After cultivated for 2 h in dark, every well was washed 3 times by cold PBS. The slides of the cell were observed under the fluorescence microscope at excitation wavelength 485 nm and emission wavelength 530 nm (Zhang, Huang, et al., Citation2016). The cells in the plate without slide were digested with 0.25% trypsin and washed three times by cold PBS. The digestive cells were fixed by 4.0% (w/v) paraformaldehyde at room temperature for 30 min and then re-suspended with PBS. The phagocytic activity was measured by flow cytometer (BD Accuri™ C6 Plus, USA) and evaluated by percentage of the phagocytic cell (PP) and phagocytic index (PI) (Brandhonneur et al., Citation2009). Phagocytic percentage (PP) and phagocytic index (PI) were calculated according to the following formula:
N1 is the number of cells with the fluorescent microsphere, N2 is the number of fluorescent microspheres in the cell, and N is the number of counted cells.
Acute toxicity study
This process was proceeded as reported previously (Yi et al., Citation2013). After overnight fasting, groups of ten male mice were administered MLN-1 in graded doses up to 5000 mg/kg. The normal control group only received water. The groups were observed for 7 days and at the end of the experiment mortality was recorded for each group.
Animal experimental design
Mice were randomly divided into 6 groups (15 mice in each group). One group of mice was used as a vehicle-treated control without any treatment for immunosuppression. The other five groups of mice were subjected to immunosuppression through intraperitoneal treatment with CY (60 mg/kg/day) from days 1 to 3. Among these groups, one group of CY-treated mice was used as an immunosuppressed model group. Astragalus polysaccharide (AP) was used as a positive control. From days 4 to 30, the six different groups of mice were administered the following: vehicle-treated control group, saline; immunosuppressed model group, saline; positive control group, 200 mg/kg body weight of AP; and the MLN-1 groups, 100, 200 or 400 mg/kg body weight of the MLN-1. All of the mice were treated daily by oral administration (Cho et al., Citation2015; Wei et al., Citation2014).
Assay of delayed type hypersensitive (DTH)
On the day of 28, mice (five mice in each group) were intraperitoneally injected with 0.2 mL of 2% (v/v) sheep red blood cells (SRBC). At the last administration, blood was obtained were detected IgA, IgG, IgM in serum by ELISA kits. Meanwhile, the left footpad thickness was measured (D1) and then 20 μL of SRBC (20%) was injected subcutaneously at the measured area on left footpad. After 24 h, the left footpad thickness (D2) was measured at the same area (Zhang, Gao, Pan, Xu, & Jia, Citation2016).
The carbon clearance test
On the day of 28, mice (five mice in each group) were injected with ink (0.01 mL/g body weight) via the tail vein. At the 2 min (OD1, t1) and 10 min (OD2, t2) after injection, 20 μL of blood was obtained from the retinal venous plexuses and added into an EP tube containing 2 mL of Na2CO3 (0.1%). After adequately mixing, 200 μL of the mixture was transferred to a 96-well plate and the absorbance at 600 nm was measured by a microplate reader. The result was shown by the clearance index (K) and the phagocytic index (α).
Immunomodulatory activity
Twenty-four hours after the last administration, blood was collected from the mice (five mice in each group) for IL-2 and INF-γ assay. The mice were subsequently sacrificed by cervical dislocation. The collected spleen samples were used to measure the lymphocyte proliferation activity and the levels of GM-CSF, IL-6, IFN-γ and IL-2. The duodenum samples were used to measure SIgA secretion.
Statistical analysis
Data were analysed by SPSS statistically and presented as means ± SD. Significant differences from control group were indicated by *p < .05, **p < .01 or # p < .05, ## p < .01.
Results
Purification and molecular weight of MLN-1
After membrane filtration and vacuum freeze-drying, brown powder (MLN-1) was collected. Then, the contents of the ingredient in MLN-1 were measured. As shown in , the content of polysaccharide in by-product could be improved and contents of protein, tannin, pectin and pigment were dramatically decreased by hollow fibre ultrafiltration membrane. It suggested hollow fibre ultrafiltration membrane separation was a feasible method of separation, purify of polysaccharide and disposition by-product of MLN.
Table 1. The basic contents of MLN and MLN-1.
The molecular weight of polysaccharide was estimated by HPGPC-ELSD. The equation of the standard curve was as follows: T = −3.1733 lg Mw + 31.627 (R2 = 0.9965, where Mw is molecular weight, and T is retention time). The results of HPGPC illustrated there were three fractions in the MLN-1 and the molecular weights were about 2,322,736, 3209 and 364 Da.
FT-IR analysis of MLN-1
MLN-1 showed bands at 3268.27, 2927.84, 1608.58, 1417.63, 1376.16, 1262.36, 1024.24, 865.04, 819.72 cm−1 and 781.14 (). A band between the regions 1200–1400 cm−1 is related to C–H variable angle vibration. MLN-1 showed bands in the regions of 3628.67, 1608.68 and 2927.84 supposing that it is related to O–H telescopic vibration, C=O asymmetric vibration and submatrix and methylene C–H bonds (Ananthi et al., Citation2010). In addition, the weak absorption peak near 865 cm−1 is the asymmetric retractive vibration of β-pyranose, which indicated the sugar units is β-configuration. It is proved that the glycoside bond of β-configuration is a necessary structural feature for immune stimulation and anti-tumor effect (Kacurakova & Wilson, Citation2001; Lin, Liao, & Ren, Citation2016; Zhang, Huang, et al., Citation2016) ().
Table 2. Qualitative analysis of MLN-1.
Effect assay of MLN-1 in vitro
Lymphocytes play an important role in the acquired immune defense system of hosts, which has various immune regulatory functions (Abbas, Lichtman, & Pillai, Citation2010; Fearon & Locksley, Citation1996; Muraille & Goriely, Citation2017). Therefore, it was important to evaluate the effects of MLN-1 on the viability of splenic lymphocytes at the indicated concentrations of 12.5–800 μg/mL for 24 h (). Results showed that splenic lymphocytes viability was not significantly influenced at the indicated concentrations of 12.5–800 μg/mL (p > .05). Results indicated that MLN-1 were safe up to 800 μg/mL to conduct the assay of anti-inflammatory activity.
Figure 2. Effect of MLN-1 on proliferation of splenic lymphocytes in vitro, measured by CCK8. According to the concentration of MLN-1, experiments were divided into seven treated groups, such as “M-12.5” (MLN-1 12.5 μg/mL). Control group including NC (PBS), ConA (1 μg/mL) and LPS (2 μg/mL). “MLN-1” was simple stimulated by MLN-1 and NC group as blank control, ConA and LPS as positive control. “MLN-1 + ConA” and “MLN-1 + LPS” were co-stimulated ConA or LPS with MLN-1, ConA and LPS as blank control. Data are presented as means ± SD (n = 3). Significant differences from blank control are indicated by *p < .05, **p < .01.
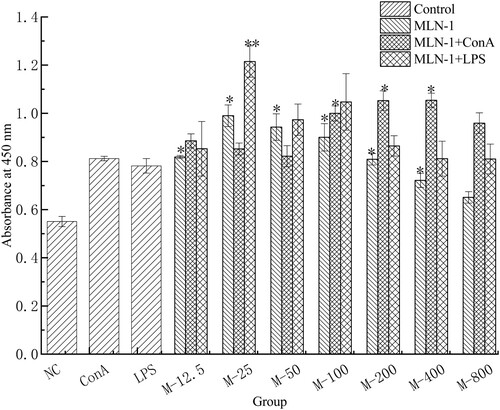
As shown in , the proliferations in groups treated with 12.5–400 μg/mL MLN-1 were significantly higher than that in the NC group (p < .05). Co-stimulation with ConA, the proliferations in M-100, M-200 and M-400 group also markedly increased compared to ConA group (p < .05). Moreover, co-stimulation with LPS, the proliferation in M-25 was remarkably higher than the LPS group (p < .01).
We also investigated the effects of MLN-1 on three representative functions of macrophages NO synthesis, cytokine production and phagocytosis. As shown in (A), the levels of NO in groups treated with MLN-1 or LPS were higher than that in NC group, especially MLN-1 at 50 and 100 μg/mL (p < .05). At the concentrations of 3.125–25 μg/mL, the productions of TNF-α in MLN-1 treated groups dramatically enhanced compared with NC group (p < .05), especially at 6.25 and 12.5 μg/mL (p < .01) ((B)). And, after treatment with MLN-1 at 3.125–50 μg/mL, the levels of IFN-γ and IL-6 significantly improved (p < .05) ((C)). Furthermore, treatment with MLN-1 was capable of not only significantly enhancing acid phosphatase activity (p < .05), but markedly increased the phagocytic activity of neutral red (p < .01) ((D)).
Figure 3. Effects of the MLN-1 on the levels of NO (A), TNF-α (B), IFN-γ and IL-6(C) in RAW264.7. Absorbance at 540 nm (D) represented the phagocytic activity of neutral red. Absorbance at 405 nm (D) represented acid phosphatase activity. According to the concentration of MLN-1, experiments were divided into six MLN-1-treated groups, and NC was used as blank control, LPS as positive control. Data are presented as means ± SD (n = 3). Significant differences from NC group are indicated by *P < .05, **P < .01.
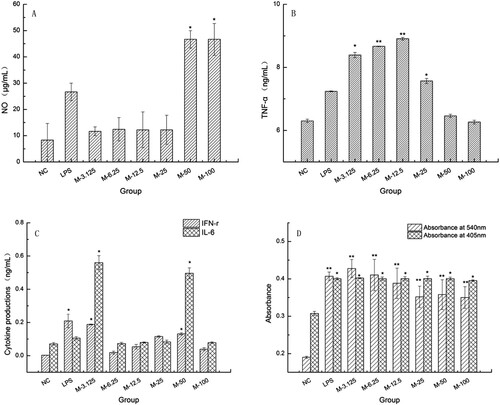
The phenomenon of phagocytosis in macrophage was shown in and data of flow cytometer were presented in . Phagocytic cells (PP) and phagocytic indexes (PI) in MLN-1 treated groups were significantly higher than that in CY (p < .01). It suggested MLN-1 could increase both the number of macrophages with fluorescent microspheres and the number of fluorescent microspheres in the macrophage.
Table 3. Phagocytic activity of fluorescence microscope in vitro.
Indeed, the basic mechanisms of anti-tumor, bactericidal and other beneficial effects of plant polysaccharides confirmed that they stimulate T and B lymphocytes cells, NK cells and macrophages, which secrete cytokines and immunoglobulin (Beutler, Citation2004). In addition, T and B lymphocytes could be activated by ConA and LPS, respectively. In this study, MLN-1 treatment could improve the proliferation of splenic lymphocytes co-stimulated with LPS or ConA both in vitro and in vivo. It suggested that MLN-1 could improve the proliferation of splenic lymphocytes not only B cells but also T cells in vitro and in vivo.
Acute oral toxicity study
The results of the acute toxicity study indicated that a single oral administration MLN-1 (5000 mg/kg) did not trigger any mortality, behavioural changes (gait, posture, fur, depression, and panting) in the mice as compared to the control group. Similarly, no significant changes were recorded in serum biochemical (total bilirubin, creatinine, triglycerides, SGOT, and glucose) as well as haematology parameters (RBCs, WBCs) of the treated group when compared to the control group.
Effects assay of MLN-1 in vivo
Under the stimulation by supernatant of MPP cells obtained from MLN-1 treated mice, except CY-M-100 (MPP-NC) group, the proliferations of bone marrow significantly increased (p < 0.05) ((A)). And the proliferation of CY-AP (MPP-NC) group was higher than MLN-1 treated group.
Figure 5. Intestinal immunomodulating activity in vivo was researched. The proliferation of bone marrow cell from NC or CY group stimulated by MPP cell (A), the levels of cytokine in PP cell (B, C), the contents of SIgA in duodenum (D). Except NC group, all groups were treated with CYC. NC, CY group was as normal control and blank control, CY-AP was used as positive control with 200 mg/kg AP; CY-M-100, CY-M-200, CY-M-400 was respectively treated with 100, 200, 400 mg/kg MLN-1. Data are presented as means ± SD (n = 5). Significant differences from CY group are indicated by *P < .05, **P < .01.
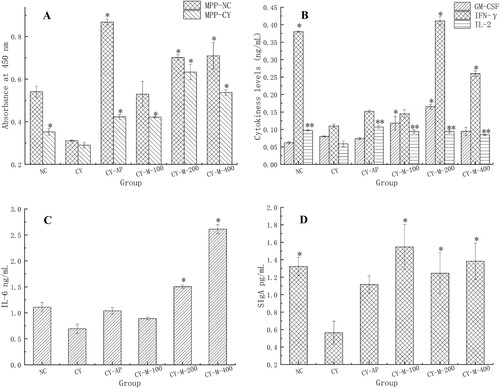
To further investigate reasons for MLN-1 stimulating the proliferation of bone marrow, the levels of cytokines in MPP were detected. Results present in , under the administration of MLN-1, the levels of GM-CSF, IL-2, IFN-γ and IL-6 were significantly improved in the CY-M-200 group (p < .05) ((B,C)). Moreover, the level of IL-2 was markedly enhanced (p < .01). In addition, no significant difference on the levels of cytokine or proliferations had recorded between the groups treated with MLN-1 and AP (p > .05). The results confirmed that both AP and MLN-1 could stimulate the immune activity of MPP by selectively boosting production of various cytokines. More importantly, it indicated MLN-1 has stronger effects than AP on enhancement immune activity of MPP.
(D) shown that MLN-1 was capable of reversing the secretion of SIgA in the duodenum in all CY-treated groups (p > .05). Especially, the secretion of SIgA in CY-M-100 group (1.5470 pg/mL) was highest, it was higher than CY-AP group (1.1161 pg/mL) ((D)).
Administration of 200, 400 mg/kg MLN-1 could dramatically enhance the proliferation of splenic lymphocytes (p < .01). Moreover, the compared to NC, the proliferations in CY-M-200 and CY-M-400 group observably improved (p < .01), but no difference was found between CY-AP, CY-M-100 and CY group (p > .05) ().
Figure 6. Effect of MLN-1 on proliferation of splenic lymphocytes in vivo, measured by CCK8. LPS (2 μg/mL) and ConA (1 μg/mL) were added in splenic lymphocytes from mouse of different groups. Data are presented as means ± SD (n = 5). Significant differences from CY group are indicated by *p < .05, **p < .01. Significant differences from NC group are indicated by #P < .05, ##P < .01, as same in total paper.
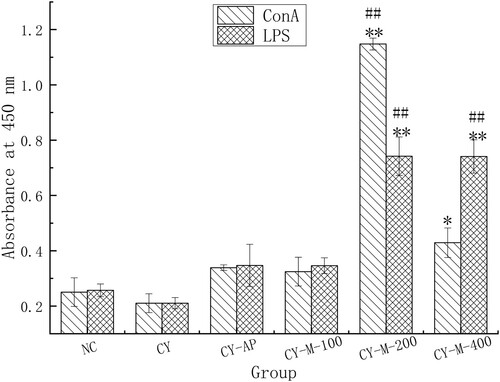
The results () of DTH shown that MLN-1 could improve the DTH in CY-treated groups, especially in CY-M-100 and CY-M-400 group (p < .01). At the same time, compared to the NC group, the DTH in CY-M-400 group observably improved (p < .01), but there had no significant difference between CY-AP and CY group.
Figure 7. DTH (n = 5) in mice were measured, treated with MLN-1 or AP for 28 days. NC, CY and CY-AP group were used as normal, blank and positive control, respectively. Data are presented as means ± SD.
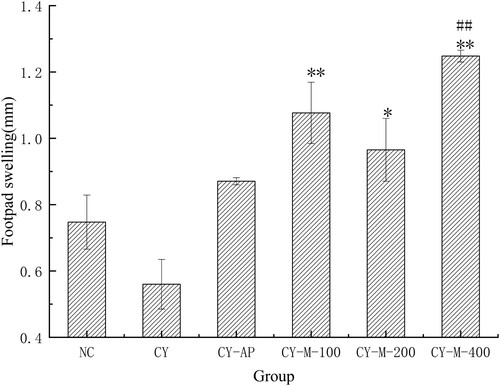
As shown in , the phagocytic indexes (K and α) in AP and MLN-1 treated groups improved, and CY-M-100 group was highest in all groups (p < .05).
Table 4. Effects of MLN-1 on macrophage phagocytosis.
As shown in (A), the levels of IgA in serum among all groups did not have a significant difference (p > .05). But when compared with CY group, the levels of IgM in MLN-1 and AP treated groups significantly enhanced (p < .05), especially in CY-M-200 and CY-M-400 group (p < .01). Moreover, there was a prominent difference between CY-M-200 and NC group (p < .01). In addition, the levels of IgG in CY-AP and CY-M-100 group were significantly higher than that in the CY group (p < .05).
AP and MLN-1 could prominently increase the levels of INF-γ in the CY treated group (p < .05) in (B). AP and MLN-1 could also improve the levels of IL-2 in CY treated group for CY-AP group was highest (p < .05) but MLN-1 treated group had a slight boost (p > .05).
When comparing CY with NC group, the different reductions of DTH, the phagocytic ability of macrophages and the remarkable decrease in the levels of IgG, IgM, INF-γ in serum and SIgA in duodenum proved an immunosuppressive mouse model, which was successfully established by CY.
Discussion
Macrophages play an irreplaceable role in the defense system of hosts for they can not only initiate innate immune responses but also be effector cells that contribute to fight foreign invaders and inflammation. Moreover, the macrophage is a bridge between innate and adaptive immunity (Juarez-Vazquez, Alonso-Castro, & Garcia-Carranca, Citation2013; Schepetkin & Quinn, Citation2006).
Macrophages can differentiate into two kinds of activated cells (M1, M2) through stimulation by LPS or some cytokines such as INF-γ and TNF-α. M1 macrophages were capable of secreting cytokines (INF-γ, TNF-α, IL-6) and NO to enhance the ability of phagocytosis and lethality (Lee et al., Citation2015; Lin et al., Citation2016). Based on experimental findings, Dendrobium huoshanense polysaccharide (DHP) could stimulate macrophages to secrete NO, TNF-α and IL-1β (Xie et al., Citation2016). Other studies showed that a polysaccharide from Astragali (AP) was able to enhance the production of NO, TNF-α and IL-6 in RAW264.7 cells (Huang et al., Citation2016). In this study, experiments performed in vitro with cultured RAW264.7 cells showed that MLN-1 had evident improvement of the phagocytic ability and the levels of NO, INF-γ and IL-6, which demonstrated macrophages differentiate into M1 were triggered by MLN-1 through cytokines. Furthermore, the assay of carbon clearance and DTH in mice also confirmed that MLN-1 could indirectly improve the immune activity of macrophage in vivo.
Due to large molecular weight, polysaccharides may be absorbed a little through the intestinal epithelium, but it can interact with MPP cells to initiate the mucosal immune system. In mucosal immunity, SIgA plays a critical role. Evidence suggests that polysaccharides could improve the mucosal immune response (Liang et al., Citation2013). Lavender pectin had activated the MPP-mediated bone marrow cell proliferation to enhancing the intestinal mucosal immunity (Georgiev et al., Citation2017). Likewise, our results demonstrated via activating the MPP-mediated bone marrow cell proliferation and enhancing the productions of cytokine from MPP cells, AP and MLN-1 were improved the intestinal mucosal immunity. Furthermore, the level of SIgA in duodenum was also enhanced after treatment with MLN-1. According to report, the levels of IgA, IgG and IgM in serum were remarkably improved by Dietary litchi pulp polysaccharides (Huang et al., Citation2016). While our results showed AP and MLN-1 could increase the levels of INF-γ, IgG and IgM rather than IgA in serum.
As a typical TCM, MLN have been manufactured more than 12,600 tons every year. With it comes to a new problem, its by-products can’t be deal with an environmentally friendly way. In this study, membrane technology was adopted to separated and purified polysaccharides for many advantages including energy-saving, convenient operation and maintenance.
In summary, MLN-1 shown a strong immune-enhancing activity by increasing the levels of cytokines, immunoglobulin and the phagocytic activity in vitro and in vivo. Our study provided a new insight into reusing the by-product of natural medicine.
Supplemental Material
Download ()Disclosure statement
No potential conflict of interest was reported by the authors.
Additional information
Funding
References
- Abbas, A. K., Lichtman, A. H., & Pillai, S. (2010). Cellular and molecular immunology (6th ed.). Philadelphia, PA: Saunders/Elsevier.
- Ananthi, S., Raghavendran, H. R. B., Sunil, A. G., Gayathri, V., Ramakrishnan, G., & Vasanthi, H. R. (2010). In vitro antioxidant and in vivo anti-inflammatory potential of crude polysaccharide from Turbinaria ornata (Marine Brown Alga). Food and Chemical Toxicology, 48(1), 187–192. doi: 10.1016/j.fct.2009.09.036
- Beutler, B. (2004). Innate immunity: An overview. Molecular Immunology, 40(12), 845–859. doi: 10.1016/j.molimm.2003.10.005
- Brandhonneur, N., Chevanne, F., Vie, V., Frisch, B., Primault, R., Le Potier, M. F., & Le Corre, P. (2009). Specific and non-specific phagocytosis of ligand-grafted PLGA microspheres by macrophages. European Journal of Pharmaceutical Sciences, 36(4–5), 474–485. doi: 10.1016/j.ejps.2008.11.013
- Chandrashekar, P. M., Prashanth, K. V. H., & Venkatesh, Y. P. (2011). Isolation, structural elucidation and immunomodulatory activity of fructans from aged garlic extract. Phytochemistry, 72(2–3), 255–264. doi: 10.1016/j.phytochem.2010.11.015
- Chen, Q., Qi, C., Peng, G., Liu, Y., Zhang, X., & Meng, Z. (2018). Immune-enhancing effects of a polysaccharide PRG1-1 from Russula griseocarnosa on RAW264.7 macrophage cells via the MAPK and NF-κB signalling pathways. Food and Agricultural Immunology, 29, 833–844. doi: 10.1080/09540105.2018.1461198
- Cho, C. W., Han, C. J., Rhee, Y. K., Lee, Y. C., Shin, K. S., Shin, J. S., … Hong, H. D. (2015). Cheonggukjang polysaccharides enhance immune activities and prevent cyclophosphamide-induced immunosuppression. International Journal of Biological Macromolecules, 72, 519–525. doi: 10.1016/j.ijbiomac.2014.09.010
- Fearon, D. T., & Locksley, R. M. (1996). The instructive role of innate immunity in the acquired immune response. Science, 272(5258), 50–53. doi: 10.1126/science.272.5258.50
- Georgiev, Y. N., Paulsen, B. S., Kiyohara, H., Ciz, M., Ognyanov, M. H., Vasicek, O., … Kratchanova, M. G. (2017). The common lavender (Lavandula angustifolia Mill.) pectic polysaccharides modulate phagocytic leukocytes and intestinal Peyer’s patch cells. Carbohydrate Polymers, 174, 948–959. doi: 10.1016/j.carbpol.2017.07.011
- Hao, L. X., & Zhao, X. H. (2016). Immunomodulatory potentials of the water-soluble yam (Dioscorea opposita Thunb) polysaccharides for the normal and cyclophosphamide-suppressed mice. Food and Agricultural Immunology, 27(5), 667–677. doi: 10.1080/09540105.2016.1148666
- He, T. B., Huang, Y. P., Yang, L., Liu, T. T., Gong, W. Y., Wang, X. J., … Hu, J. M. (2016). Structural characterization and immunomodulating activity of polysaccharide from Dendrobium officinale. International Journal of Biological Macromolecules, 83, 34–41. doi: 10.1016/j.ijbiomac.2015.11.038
- He, X. R., Wang, X. X., Fang, J. C., Chang, Y., Ning, N., Guo, H., … Huang, X. Q. (2017). The genus Achyranthes: A review on traditional uses, phytochemistry, and pharmacological activities. Journal of Ethnopharmacology, 203, 260–278. doi: 10.1016/j.jep.2017.03.035
- Huang, F., Zhang, R. F., Liu, Y., Xiao, J., Liu, L., Wei, Z. C., … Liu, D. (2016). Dietary litchi pulp polysaccharides could enhance immunomodulatory and antioxidant effects in mice. International Journal of Biological Macromolecules, 92, 1067–1073. doi: 10.1016/j.ijbiomac.2016.08.021
- Juarez-Vazquez, M. D., Alonso-Castro, A. J., & Garcia-Carranca, A. (2013). Kaempferitrin induces immunostimulatory effects in vitro. Journal of Ethnopharmacology, 148(1), 337–340. doi: 10.1016/j.jep.2013.03.072
- Kacurakova, M., & Wilson, R. H. (2001). Developments in mid-infrared FT-IR spectroscopy of selected carbohydrates. Carbohydrate Polymers, 44(4), 291–303. doi: 10.1016/S0144-8617(00)00245-9
- Kumar, V. P., Prashanth, K. V. H., & Venkatesh, Y. P. (2015). Structural analyses and immunomodulatory properties of fructo-oligosaccharides from onion (Allium cepa). Carbohydrate Polymers, 117, 115–122. doi: 10.1016/j.carbpol.2014.09.039
- Lee, S. G., Jung, J. Y., Shin, J. S., Shin, K. S., Cho, C. W., Rhee, Y. K., … Lee, K. T. (2015). Immunostimulatory polysaccharide isolated from the leaves of Diospyros kaki Thumb modulate macrophage via TLR2. International Journal of Biological Macromolecules, 79, 971–982. doi: 10.1016/j.ijbiomac.2015.06.023
- Liang, M. F., Liu, G. H., Zhao, Q. Y., Yang, S. F., Zhong, S. X., Cui, G. L., … Zhu, R. L. (2013). Effects of Taishan Robinia pseudoacacia polysaccharides on immune function in chickens. International Immunopharmacology, 15(4), 661–665. doi: 10.1016/j.intimp.2013.02.026
- Lin, Z. H., Liao, W. Z., & Ren, J. Y. (2016). Physicochemical characterization of a polysaccharide fraction from Platycladus orientalis (L.) Franco and its macrophage immunomodulatory and anti-hepatitis B virus activities. Journal of Agricultural and Food Chemistry, 64(29), 5813–5823. doi: 10.1021/acs.jafc.6b01387
- Muraille, E., & Goriely, S. (2017). The nonspecific face of adaptive immunity. Current Opinion in Immunology, 48, 38–43. doi: 10.1016/j.coi.2017.08.002
- Park, Y. M., Lee, H. Y., Kang, Y. G., Park, S. H., Lee, B. G., Park, Y. J., … Lee, Y. R. (2019). Immune-enhancing effects of Portulaca oleracea L.-based complex extract in cyclophosphamide-induced splenocytes and immunosuppressed rats. Food and Agricultural Immunology, 30(1), 13–24. doi: 10.1080/09540105.2018.1540552
- Peng, L., Li, M., Xu, Y. Z., Zhang, G. Y., Yang, C., Zhou, Y. N., … Zhang, J. P. (2012). Effect of Si-Miao-Yong-An on the stability of atherosclerotic plaque in a diet-induced rabbit model. Journal of Ethnopharmacology, 143(1), 241–248. doi: 10.1016/j.jep.2012.06.030
- Qiao, D. L., Wei, C. B., Chen, N. D., Min, Y. J., Xu, H. J., & Chen, R. (2017). Influences of Hyriopsis cumingii polysaccharides on mice immunosignaling molecules and T lymphocyte differentiation. Food and Agricultural Immunology, 28(4), 586–598. doi: 10.1080/09540105.2017.1306494
- Schepetkin, I. A., & Quinn, M. T. (2006). Botanical polysaccharides: Macrophage immunomodulation and therapeutic potential. International Immunopharmacology, 6(3), 317–333. doi: 10.1016/j.intimp.2005.10.005
- Tian, J., Che, H. L., Ha, D., Wei, Y. P., & Zheng, S. Y. (2012). Characterization and anti-allergic effect of a polysaccharide from the flower buds of Lonicera japonica. Carbohydrate Polymers, 90(4), 1642–1647. doi: 10.1016/j.carbpol.2012.07.044
- Wei, J. T., Wang, S. X., Liu, G., Pei, D., Liu, Y. F., Liu, Y., & Di, D. L. (2014). Polysaccharides from Enteromorpha prolifera enhance the immunity of normal mice. International Journal of Biological Macromolecules, 64, 1–5. doi: 10.1016/j.ijbiomac.2013.11.013
- Xie, S. Z., Hao, R., Zha, X. Q., Pan, L. H., Liu, J., & Luo, J. P. (2016). Polysaccharide of Dendrobium huoshanense activates macrophages via toll-like receptor 4-mediated signaling pathways. Carbohydrate Polymers, 146, 292–300. doi: 10.1016/j.carbpol.2016.03.059
- Yang, L. C., Hsieh, C. C., Wen, C. L., Chiu, C. H., & Lin, W. C. (2017). Structural characterization of an immunostimulating polysaccharide from the stems of a new medicinal Dendrobium species: Dendrobium Taiseed Tosnobile. International Journal of Biological Macromolecules, 103, 1185–1193. doi: 10.1016/j.ijbiomac.2017.05.185
- Yang, W. M., Shi, Z. B., Yang, H. Q., Teng, J. F., Zhao, J., & Xiang, G. L. (2015). Mailuoning for acute ischaemic stroke. Cochrane Database of Systematic Reviews. doi: 10.1002/14651858.CD007028.pub3
- Yi, J., Ye, X. L., Wang, D. Z., He, K., Yang, Y., Liu, X. J., & Li, X. G. (2013). Safety evaluation of main alkaloids from Rhizoma Coptidis. Journal of Ethnopharmacology, 145(1), 303–310. doi: 10.1016/j.jep.2012.10.062
- Yu, Z. D., Kong, M. L., Zhang, P. Y., Sun, Q. J., & Chen, K. S. (2016). Immune-enhancing activity of extracellular polysaccharides isolated from Rhizopus nigricans. Carbohydrate Polymers, 148, 318–325. doi: 10.1016/j.carbpol.2016.04.068
- Zhang, J. J., Gao, X., Pan, Y. G., Xu, N., & Jia, L. (2016). Toxicology and immunology of Ganoderma lucidum polysaccharides in Kunming mice and Wistar rats. International Journal of Biological Macromolecules, 85, 302–310. doi: 10.1016/j.ijbiomac.2015.12.090
- Zhang, J., Hu, Y. L., Wang, D. Y., Qin, T., Liu, C., Liu, X., … Nguyen, T. L. (2012). The optimization of sulfation modification conditions for ophiopogonpolysaccharide based on antiviral activity. International Journal of Biological Macromolecules, 51(4), 657–662. doi: 10.1016/j.ijbiomac.2012.06.023
- Zhang, W. J., Huang, J., Wang, W., Li, Q., Chen, Y., Feng, W. W., … Wu, X. Y. (2016). Extraction, purification, characterization and antioxidant activities of polysaccharides from Cistanche tubulosa. International Journal of Biological Macromolecules, 93, 448–458. doi: 10.1016/j.ijbiomac.2016.08.079
- Zheng, P. M., Fan, W. T., Wang, S. H., Hao, P., Wang, Y., Wan, H. Y., … Zhao, X. N. (2017). Characterization of polysaccharides extracted from Platycodon grandiflorus (Jacq.) A.DC. affecting activation of chicken peritoneal macrophages. International Journal of Biological Macromolecules, 96, 775–785. doi: 10.1016/j.ijbiomac.2016.12.077