ABSTRACT
Pomegranate peel polyphenols (PPPs) extracts presented anti-proliferation and apoptosis-inducing effects in human HepG2 hepatoma cells in our previous studies. This study aimed to investigate the differential anticancer effects of main PPPs components punicalagin (PC) and ellagic acid (EA) on HepG2 cells and their possible action mechanisms. Cell proliferation, morphology, cell cycle and apoptosis were investigated, and the results revealed that PC and EA mediated the cell-cycle arrest at the S-phase and G0/G1-phase and dose-dependently led to apoptosis in HepG2 cells. In addition, apoptosis-related protein activities and the reactive oxygen species levels showed that the activation of apoptosis-related proteins and the elevation of intracellular H2O2 production were aroused. It revealed that both PC and EA could inhibit cell growth and led to apoptosis in HepG2 cells, and PC was more effective than EA. Furthermore, one of the important apoptosis-inducing mechanisms is the participation of them in the mitochondrial apoptosis pathway.
1. Introduction
Pomegranate is widely cultivated in China. As a food and medicine dual-purpose plant, both of its fruit and peel have anti-oxidation and anti-tumour medicinal effects due to their abundant polyphenols contents (Avriam et al., Citation2008; Johanningsmeier & Harris, Citation2011; Tzulker et al., Citation2007). In the pomegranate processing industry, a number of pomegranate products including pomegranate juices, pomegranate beverages, and pomegranate seed oil were popular in the market for their nutritional ingredients and health function. However, large amounts of the pomegranate peels, accounting for 20%–30% of the weight of the pomegranate fruit, are discarded as pomegranate processing wastes, which lead to a waste of resources (Ferrueloa et al., Citation2014). Meanwhile, many studies have shown the efficacy of pomegranate juice and its peel extracts in health care applications, anti-inflammatory (Braga et al., Citation2005; Lansky & Newman, Citation2007) and anti-tumour properties (Aviram & Dornfeld, Citation2001; Syed, Afaq, & Mukhtar, Citation2007). Daily consumption of pomegranate juices is potentially better than apple juice in improving antioxidant function in the elderly. Also, pomegranate juices consumption by diabetic patients could result in anti-oxidative effects on serum and macrophages, without worsen the diabetic parameters (Guo et al., Citation2008; Rosenblat, Hayek, & Aviram, Citation2006). Pomegranate fruit extracts resulted in a significant inhibition in tumour growth concomitant with a significant decrease in serum prostate-specific antigen levels (Malik et al., Citation2005). Particularly, ellagic acid, might be the main polyphenol in pomegranate juice that induced human prostate cancer PC3 cells apoptosis and cell growth inhibition in vitro (Malik, Afaq, Shahid, Akhtar, & Assiri, Citation2011).
At present, the study on the anticancer effects of pomegranate peel polyphenolic extracts and their main active components are rarely reported (Song, Li, & Li, Citation2016). As the main monomers and the beneficial component against various diseases among the pomegranate peel polyphenols, punicalagin (PC) and ellagic acid (EA) were reported elsewhere. We previously reported that pomegranate peel polyphenols extracts can induce apoptosis in human hepatoma cells, and speculated that the main inducing apoptosis function may assumed by their main component, PC and EA (Song et al., Citation2016). In order to provide more details of apoptosis inducing effect of PC/EA on human hepatocellular carcinoma cell line HepG2 cells, further study will conduct to find out different effects by PC and EA, and compare which one is more effective. Therefore, this paper aimed to investigate the differential anticancer effects of the main components PC and EA on human hepatocellular carcinoma cell line HepG2 cells and their possible mechanisms of action.
In the present study, the effects of PC and EA on HepG2 cellular morphology, cell cycle, apoptosis, reactive oxygen species and mitochondrial trans were investigated, respectively. In addition, to get an insight into the mechanisms of action, the activities of caspase-3/9, and apoptosis-related protein expression in HepG2 were also discussed. This study not only provides a basis for the functional mechanism and future application of PC and EA, but also promotes the high value utilisation of pomegranate by-products.
2. Materials and methods
2.1. Materials and reagents
Pomegranate fruits were purchased from local markets in Lintong (Shaanxi, China). The standards of Punicalagin and Ellagic acid were purchased from Sigma (St. Louis, MO, USA). The Roswell Park Memorial Institute-1640 (RPMI-1640) medium was purchased from Life Technologies (Carlsbad, CA, USA). Foetal bovine serum (FBS) was purchased from Tianhang Biological Technology Co. (Zhejiang, China). DMSO, MTT, Hoechst 33258 staining, propidium iodide (PI), and RNase-A were purchased from Sigma (St. Louis, MO, USA). The Annexin V-FITC (fluorescein isothiocyanate)/PI (propidium iodide) kit and DCFH-DA were purchased from Nanjing Jiancheng Biology Co. (Nanjing, China). The caspase-3 and caspase-9 detection kits were purchased from Beyotime Biotechnology Co. (Shanghai, China). 1-antibody was purchased from Abcam Co. (Cambridge, England) and Cell Signaling Technology Co. (Shanghai, China). 2-antibody was purchased from Shenzhen Bioeasy Biotechnology Co. (Shenzhen, China). All the other cell culture reagents were purchased from Sinopharm (Beijing, China).
2.2. Extraction and quantification of PC and EA from pomegranate peels
For PC and EA extraction (Their chemical formulas were listed in ), pomegranate peels were firstly manually removed, sun-dried and powdered. PPPs crude extracts were extracted with 60% (v/v) ethanol solution under ultrasonic method. The specific procedures were: the ratio of material to liquid was 1:20, with an extraction time 25 min under 100 W ultrasonic power. The extraction solution was centrifuged at 5000 g for 10 min. After then, the supernatant was collected and concentrated by rotary evaporator at 40°C, crude extracts were then obtained followed by freeze-drying (vacuum degree, 5 mTorr). Resin adsorption and desorption method were used for purification. 25 mg resins was put into 250 mL 1 mg/mL crude extracts solution in conical flasks, then, placed on an orbital shaker at 130 rpm and 25°C for 10 h. After adsorption, resins with pomegranate peel polyphenols were eluted with distilled water twice, then 70% (v/v) ethanol was added to eluent with volume eluent for desorption. Finally, refined PPPs extracts containing PC and EA was obtained after concentrated and freeze-dried.
A 1525 Waters HPLC system (Waters Corp., Milford, Massachusetts, USA) equipped with a binary pump and UV detector (set at 280 nm) was used to quantitatively analyse the pomegranate peel polyphenols. Polyphenols quantification was analysed by a Zorbax SB-C18 column (4.6 mm × 250 mm, 5 μm, Agilent, American) using 1% glacial acetic acid (A) and methanol (B) as mobile phase (0–70 min, 5%–44% B; 70–80 min, 44%–44% B) at a flow rate of 1 mL/min.
2.3. Cell culture and grouping
Human hepatoma cells (HepG2) were purchased from the experimental animal centre of the Fourth Military Medical University (Xi’an, China), and normal human hepatic L-02 cells were purchased from the laboratory animal centre of Sun Yat-Sen University (Nanjing, China). Cells were maintained in RPMI-1640 medium supplemented with 10% FBS, penicillin (100 units/mL) and streptomycin (100 μg/mL) and cultured at 37°C in a humidified incubator with an atmosphere containing 5% CO2.
Three sets of experiments were performed as follows: the test substance treatment group with final concentrations of 50, and 100 μM of PC and EA, the 5-Fu treatment group with a final concentration of 200 μM, and the control group. The test compounds were dissolved in DMSO and diluted by the serum-free culture medium. The final DMSO concentration did not exceed 0.1%. All experiments were repeated three times independently.
2.4. Cell proliferation assessment
The inhibitory effects of PC and EA on the proliferation of HepG2 were measured by MTT assay. Cells were seeded in 96-well culture plates (5 × 103 cells/well). After 24 h, the cells were washed with fresh medium and treated with different concentrations of PC or EA as the sets of experiments for various periods. After the incubation periods of 24 and 48 h, 10 μL of MTT solution (5 mg/mL), which was dissolved in PBS, was added to each well and further incubated for 4 h. Finally, DMSO (150 μL/well) was added, and the formazan crystals that formed were dissolved. The absorbance was measured at 570 nm using a microplate reader (Multiskan Go, Thermo Electron Corporation, USA). Cell viability was calculated by the following formula:
At, Ac and Ab stand for the absorbance of the test substances, the control and the blank, respectively.
2.5. Hoechst 33258 staining analysis
HepG2 cells were cultured in 6-well plates (4 × 105 cells/well) for 24 h and then treated with different concentrations of PC or EA as the sets of experiments for 48 h. PBS was added to wash the wells, and then, the cells were fixed by 4% paraformaldehyde at 4°C. After 15 min, the cells were washed again by PBS and dyed with Hoechst 33258 staining for 10 min. Finally, the morphological changes of the cells were observed under the inverted fluorescence microscope.
2.6. Cell cycle analysis by flow cytometry
The cell cycle distribution was detected by flow cytometry using PI (Marel, Lizard, Izard, Latruffe, & Delmas, Citation2008). HepG2 cells (1 × 106 cells/well) were grown for 24 h and then treated with different concentrations of PC or EA as the sets of experiments for 48 h. Both the suspension cells and the trypsinized adherent cells were collected and then suspended in cold PBS and fixed with 70% ethanol at −20°C overnight. After discarding the supernatant, 1 mL of PBS containing RNase (1.0 mg/mL) and 0.5 mL of PI (50 μg/mL) were added to stain cells for 30 min at room temperature in the dark. Then, the cell cycle was analysed with flow cytometry (Millipore Corporation, Billerica, MA, USA).
2.7. Apoptosis measurement by flow cytometry
The apoptosis was quantified by an Annexin V-FITC/PI double staining assay using an Annexin V-FITC detection kit. Approximately 1 × 106 cells/well in 6-well plates were incubated overnight and then treated with different concentrations of PC or EA as the sets of experiments for 48 h. Thereafter, all the cells were collected and suspended in 400 μL of binding buffer. Next, 5 μL of Annexin V-FITC and 10 μL of PI were added to stain for 10 min in the dark. The early apoptotic cells and late apoptotic/necrotic cells (annexin+/PI− and annexin+/PI+) were measured by the flow cytometry.
2.8. Detection of caspase-3 and caspase-9 activities
Analyses of the activities of caspase-3 and caspase-9 were carried out with a Caspase Activity Assay kit (Beyotime Biotechnology Co., Shanghai, China) according to the manufacturer’s instructions. Cells in the control group and in each treatment group were collected and lysed in cold lysis buffer. After 15 min in ice, protein was obtained from the supernatant by centrifugation and was mixed with detection buffer and catalytic substrate (AcDEVD-pNA and Ac-LEHD-pNA) in a 96-well plate. The mixtures were incubated for 2 h at 37 °C. The activity of the caspases, which was represented by the absorbance, was measured at 405 nm with a microplate reader (Multiskan Go, Thermo Electron Corporation, USA).
2.9. Intracellular reactive oxygen species assay in HepG2 cells
The production of intracellular reactive oxygen species (ROS) was assessed by a DCFH-DA probe. Briefly, HepG2 cells were seeded at a density of 1 × 106 cells/mL in 6-well plates and treated with different concentrations of PC or EA for 24 h. Then, the cells were collected and exposed to 0.2 mL PBS containing the final concentration of 10 μM DCFH-DA. After 30 min of incubation at 37 °C, the fluorescence intensity, which represents the production of ROS, was measured by the flow cytometer.
2.10. Apoptosis-related protein expression detected by western-blot
Cells of control group and treatment group were collected and lysed with protein extraction reagent and proteinase inhibitors. The lysates were centrifuged at 15,000 g for 30 min, draw the supernatant and determined the protein concentration by Bradford assay (Li et al., Citation2013). After being separated by 15% SDS-PAGE, proteins were transferred to PVDF membranes and use 5% fat-free dry milk to block for 1 h. Using 1-antibody (1:1000–3000) to closed overnight at 4°C. Later, 2-antibody (1:10000), P53, Bax, Bcl-2, Cyt-c, Caspase-3/9, PARP and Actin are incubated for 1 h under the condition of 37°C. Using alkaline phosphatase colour kit to colouring, then take and preserve pictures. The experiment repeated three times.
3. Results
3.1. Analysis of PC and EA in pomegranate peels
Polyphenols compounds in purified pomegranate peel extracts were identified, and the components and quantification were showed in . There were seven main polyphenolic substances successfully separation and identification. Among these compounds, PC with isomer structures (two peaks, 15.22 and 22.49 min) was the major component, with the total content of 65.38 mg/100 mg. Followed with catechin, EA, and gallic acid, with the content of 12.66, 2.93, and 2.53 mg/100 mg, respectively. The results showed that PC, catechin, EA, and gallic acid were the mainly components in PPPs.
3.2. Effects of PC and EA on antiproliferation of HepG2 cells
As shown in the results of the MTT method in , PC and EA inhibited the survival rate of HepG2 cells (hepatoma cells) in a dose-dependent and time-dependent manner but had no significant effect on L-02 cells (normal hepatic cell) ((B)). The inhibitory rates of HepG2 cells treated with PC and EA at 25, 50 and 100 μM for 24 h was 16.4%, 26.3%, and 40.2% and 13.1%, 25.7%, and 37.8%, respectively. When treated with PC at 25, 50 and 100 μM for 48 h, the inhibitory effects were significantly increased to 36.2%, 47.3%, and 59.9%, respectively, which were higher than those of EA (29.1%, 44.4%, 52.8%), ((A)). Compared to the control, the PC and EA treatments had a significant influence on the survival rate of HepG2 cells (P < 0.01). After 48 h of treatment, the IC50 values reached 94.70 μM for EA and 83.47 μM for PC. Thus, the results demonstrated that the inhibition of HepG2 cell proliferation induced by PC was stronger than that induced by EA. This was similar to another study, Raji lymphoma cell line was inhibited by Cuscuta extracts (Aribi, Zerizer, Kabouche, Screpanti, & Palermo, Citation2016; Ghazanfari, Naseri, Shams, & Rahmati, Citation2013), it suggested that many natural plant extracts could inhibit cancer cells proliferating without affecting normal cells.
3.3. Effects of PC and EA on the morphology of HepG2 cells
The morphological change was an index used to evaluate apoptosis. The morphology of the cells after Hoechst 33258 staining is shown in . Compared with the control group, the cells in the treatment group were deformed and deeply stained. Punctate fluorescence was increased and enhanced. It could be seen that there was nuclear shrinkage, and chromatin margination appeared. With an increase in the concentration of the test substance, the cell deformation and hyperchromatic phenomenon became more obvious.
3.4. Effects of PC and EA on cell cycle arrest
To determine whether the cell growth inhibition of PC and EA was due to cell cycle arrest, we examined the cycle phases of HepG2 cells that were treated with PC and EA for 48 h and stained by PI. When PC acted on HepG2 cells for 48 h at 50 and 100 μM, the DNA histogram results of cell cycle analysis indicated that the number of cells in the S phase was enhanced from 36.78% to 50.25% (P < 0.01) ((A)) and was accompanied by a decrease of the percentage of cells in the G0/G1 phase from 51.58% to 43.79%. Nevertheless, after continuing the role of EA on HepG2 cells for 48 h at the same concentration, the accumulation of cell population in the G0/G1 phase was increased from 60.53% to 68.61% (P < 0.01), and the quantity of cells in the G2/M phase was reduced simultaneously ((B)). These results indicated that both PC and EA could inhibit the growth of HepG2 cells, since they could arrest HepG2 cells in the S phase and G0/G1 phase, respectively.
Figure 5. Effects of PC and EA on cell cycle arrest in HepG2 cells. (A) Representative histograms of DNA content in the cells incubated with different concentrations of PC or EA (0, 50 and 100 μM) for 48 h, 5-Fu was set as positive control group; (B) Percentage of cell populations in G0/G1, S and G2/M phases. *p < 0.05, **p < 0.01.
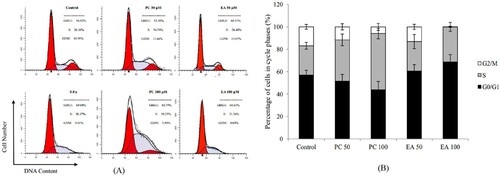
3.5. Effects of PC and EA on apoptosis induction
To elucidate whether the death of HepG2 cells was due to apoptosis induced by PC and EA, we performed an apoptotic characterisation of the cells treated with PC and EA for 48 h. The results of Annexin V-FITC and PI double staining and flow cytometry analysis are shown in (A). In contrast with the control group, when HepG2 cells were treated with PC and EA at 50 and 100 μM, the percentage of late apoptosis cells was elevated to 23.30% and 35.05% for PC (P < 0.01) and 16.13% and 23.80% for EA (P < 0.01), respectively ((B)). Moreover, PC and EA caused a variation in early apoptotic rates, although the change is small. The results suggested that both PC and EA induced cell apoptosis in a dose-dependent manner, and the effect of apoptosis induced by PC was stronger than that of EA (P < 0.05).
3.6. Effects of PC and EA on caspase-9 and caspase-3 activities in HepG2 cells
The caspase protease family plays a central role in coordinating apoptotic responses (Son et al., Citation2010). To investigate the apoptotic pathway, we examined caspase-9 and caspase-3 activities. (A and B) show the significant changes in the activities of caspase-9 and caspase-3 in cells treated with PC and EA at 50 and 100 μM for 24 h. Compared to the control, the activities of caspase-9 in the cells of treatment groups were increased 1.3-fold (P < 0.01) and 1.9-fold (P < 0.01) for PC, while EA caused 1.1-fold (P < 0.05) and 1.5-fold (P < 0.01) increases ((A)). Similarly, the intracellular caspase-3 activity was also increased ((B)). The activity was increased as the concentrations of test substance increased, and PC had a better effect than EA under the same conditions. The expression of caspase-3 and caspase-9 proteins were conducted by western blotting. As shown in , caspase-3 and caspase-9 proteins were significantly expressed in drug-treated group compared with those in the control, indicating that apoptosis did occur in HepG2 cells in drug-treated groups.
3.7. ROS production of HepG2 cells
According to recent studies, the increase in ROS represents one signal prior to cancer cell apoptosis; evidence has also proved that some phytochemicals can stimulate the cancer cells to produce more ROS to their toxicity threshold and then induce apoptosis (Trachootham, Alexandre, & Huang, Citation2009). We conjectured that the cell growth inhibition of PC and EA is partly due to the increased levels of ROS in cells. Therefore, we detected intracellular ROS with a DCF probe to confirm this suspicion. The results of changes in the ROS level in HepG2 cells are shown in (C). Both PC and EA at different concentrations on HepG2 cells lasted for 24 h; the intracellular ROS were all significantly higher, in contrast with control cells. Furthermore, 50 and 100 μM of PC and EA promoted the H2O2 production by 20.40% and 44.17% and by 13.80% and 32.90%, respectively, dose-dependently. The above investigation indicated that PC and EA induced the generation of H2O2 in HepG2 cells. Therefore, the level of ROS was higher than baseline, and the effect of PC was more intense than that of EA.
3.8. Analysis of expression of apoptosis-related proteins
The results of western-blot were shown in . Compared with the control group, the expression of apoptosis-related protein P53 was significantly increased in PC, EA and PPPs treatment groups. The expression of pro-apoptotic protein Bax was increased with the increase of the concentration of test substance. At the same time, the expression of the pro-apoptotic protein Cyt-c was also significantly increased. Moreover, the DNA repair enzyme PARP in treatment groups were cleavage into three fragments, but the control group of PARP was still in single band. These series of changes in apoptosis-related protein expression in HepG2 cells suggests that PC and EA could induce HepG2 cells apoptosis and its apoptosis pathway may be via the intracellular mitochondrial pathway.
4. Discussion and conclusion
Under the natural state, pomegranate peel polyphenols are mainly made up of PC, which accounts for 65.75% of the total phenol in the pomegranate peel (He et al., Citation2015; Li, Zhao, Yu, & Li, Citation2009). Studies have shown that the major form of internal metabolism and absorption for PC is pomegranate EA, the hydrolysate of PC (Castonguay et al., Citation1997; Larrosa, Tomas-Barberan, & Espin, Citation2006; Seeram et al., Citation2007). Consequently, there are generally two forms of pomegranate peel polyphenol extract products on the market: one is a natural extract of pomegranate peel polyphenols that is mainly made up of PC, and the other is a type of pomegranate peel polyphenols extract in which PC is hydrolysed to EA (which accounts for more than 90%) in the extraction process. However, it is not yet clear whether both PC and EA are bioactive, or which is more active in HepG2 cells. To explore this question, the study was conducted in an effort to investigate the in vitro anti-hepatoma activity of PC and EA using the human hepatocellular carcinoma cell HepG2 as a model cell.
Although it is widely recognised that PC is the main monomer of pomegranate peel polyphenols, there are few reports concerning its anti-tumour activity. A previous study demonstrated the effect of PC on human glioma cell U87MG, and the results revealed that PC could induce cell apoptosis and autophagy and finally resulted in cell death (Wang et al., Citation2013). While there were reports on the anti-tumour effect of EA, the other major monomers of pomegranate peel polyphenols, such as in prostate cancer and cerebral cancer (Kim et al., Citation2010; Malik et al., Citation2011). However, there are few reports on the effects of PC and EA for inducing apoptosis of hepatoma cells and their mechanism. In the present study, we separately examined the potential effects of PC and EA on cell viability and morphology, cell-cycle distribution, apoptosis, caspase-3 and caspase-9 activities, and intracellular ROS generation in HepG2 cells, and we discovered that both PC and EA could significantly inhibit the proliferation of HepG2 cells and induce cell apoptosis. Furthermore, the roles of PC and EA might be related to participation in the mitochondrial apoptotic pathway. Comparing the anti-tumour activities of PC and EA, we found that the activity of PC was stronger than that of EA. This may be due to the different molecular structures of them. EA is hydrolysis product of PC and has a smaller molecular structure (Larrosa et al., Citation2006). Maybe they have different receptors or targets, which need a further study. Regarding to the influence of PC and EA on cell cycle, our study demonstrated that they both have a retardation effect on HepG2 cells. PC mainly arrested the cell cycle at the S phase and EA did so at the G0/G1 phase. Currently, there is a theory that the phytochemical contribution is through various pathways by recognising different targets (Wagner, Citation2006). The regulation of cell cycle is theoretically through the G1 phase of the retention. The G0 phase is when cell is temporarily out of cycle and stops division (Alberts et al., Citation2013). Our study reached an agreement with this argument. The results showed that EA significantly blocked cancer cells during the G0/G1 phase, indicating that EA probably inhibited the HepG2 cell proliferation by retention the cell at G1 phase and stopping the cell division in G0 phase, this inhibition effect was identified with the effect of acylated blueberry anthocyanin on H22 murine tumours (Liu, Chen, Li, & Sun, Citation2016). Furthermore, PC significantly blocked cancer cells during S phase when DNA synthesis, suggesting that PC maybe prevented the cancer cell DNA synthesis. It was consistent with the results of FCM determination. What’s more, PC could be degraded and transformed into EA in some extent, which revealing a functional overlap towards PC and EA although they contributed to pathways individually during cell cycle. However, the accurate mechanism of PC and EA on cancer cell cycle remains further exploration.
There are also some in-depth studies on the anti-tumour activity of EA towards different cancer cells, such as human bladder cancer T24 (Li et al., Citation2005), human cervical cancer CaSki (Narayanan, Geoffroy, Willingham, Re, & Nixon, Citation1999), and colon cancer (Narayanan & Re, Citation2001). What is consistent with this research result was that EA could induce apoptosis in these cells arrested human bladder cancer T24 and human cervical cancer CaSki cells in the G0/G1 phase, and led to changes in caspase and ROS levels in T24 cells. Meanwhile, these studies explored the genes related to apoptosis regulation. In our study, PC and EA raised the ROS level and the activity of caspase-3/9. Moreover, All PARP in the treatment groups were divided into 3 bands, while only 1 band in the control group. This may means that the DNA repair enzyme PARP in treatment groups of HepG2 cells maybe cleavage into fragments by Caspase-3/9, due to the PARP is the substrate of Caspase-3/9. In our experiment we found that increased the expression of Caspase-3/9, we therefore speculate that the DNA repair enzyme PARP lost activity after being cut by Caspase-3/9. This process further accelerates the cancer cell apoptosis. In other words, the results of protein expression of PARP suggest that PC and EA may block the DNA repair in cancer cell and therefore accelerating the apoptosis of HepG2 cells.
Besides, the expressions of apoptotic-related proteins which including P53, Bax, Cyt-c in treatmnet group cells were significantly increased compared with the control group. The changes of gene expression show that the mechanism of apoptosis in HepG2 cells induced by PC and EA maybe it is related to their participation in the mitochondrial apoptotic pathway (Kwon et al., Citation2014; Li, Shao, Fu, Han, & Gao, Citation2010; van Delft & Huang, Citation2006).
In the comprehensive view, we can draw a conclusions as follows, the substances were adopted in this paper, PC and EA as well as its originated extracts PPPs, all of them have good inhibitory and proapoptotic effects on human hepatoma HepG2 cells, it seems PC was more stronger effect on HepG2 cells than EA, PC and EA-rich extracts PPPs show a better effect than any of them single use, this may be due to the possible synergistic effect of the two of them, the mechanism of apoptosis in HepG2 cells induced by PC, EA and PPPs maybe it is related to their participation in the mitochondrial apoptotic pathway.
Of course, It should be pointed out that in vitro experiment, one advantage is the fact that it can exclude the effects of complex factors resulting from the internal metabolic biotransformation of test substances, and we can intuitively and accurately observe and analyse the effects of test substances on cancer cells and their mechanisms. But it cannot be denied that the in vitro experiments have some limitation. This experiment cannot reflect the internal absorption, metabolism, and activity of test substances, which need further study in vivo.
Acknowledgements
The Project was supported by the National Natural Science Foundation of China (Grant No. 31871801) and by the Fundamental Research Funds for the Central Universities (Project No. GK201706003; GK201703066; and No. GK201803068), and also Supported by the Science and Technology Research of Shaanxi Province (Project No. 2019NY-136).
Disclosure statement
No potential conflict of interest was reported by the authors.
Additional information
Funding
References
- Alberts, B., Bray, D., Hopkin, K., Johnson, A., Lewis, J., Raff, M., Roberts, K., & Walter, P. (2013). Essential cell biology (4th ed., pp. 605–607, 613–614). NY: Garland Science, Taylor & Francis Group.
- Aribi, B., Zerizer, S., Kabouche, Z., Screpanti, I., & Palermo, R. (2016). Effect of Argania spinosa oil extract on proliferation and Notch1 and ERK1/2 signaling of T-cell acute lymphoblastic leukemia cell lines. Food and Agricultural Immunology, 27(3), 350–357. doi: 10.1080/09540105.2015.1104654
- Aviram, M., & Dornfeld, L. (2001). Pomegranate juice consumption inhibits serum angiotensin converting enzyme activity and reduces systolic blood pressure. Atherosclerosis, 158, 195–198. doi: 10.1016/S0021-9150(01)00412-9
- Avriam, M., Volkova, N., Coleman, R., Dreher, M., Reddy, M. K., Ferreira, D., & Rosenblt, M. (2008). Pomegranate phenolics from the peels, arils, and flowers are antiatherogenic: Studies in vivo in atherosclerotic apolipoprotein E-deficient (E0) mice and in vitro in cultured macrophages and lipoproteins. Journal of Agricultural and Food Chemistry, 56, 1148–1157. doi: 10.1021/jf071811q
- Braga, L. C., Shupp, J. W., Cummings, C., Jett, M., Takahashi, J. A., Carmo, L. S., … Nascimento, A. M. A. (2005). Pomegranate extract inhibits staphylococcus aureus growth and subsequent enterotoxin production. Journal of Ethnopharmacology, 96, 335–339. doi: 10.1016/j.jep.2004.08.034
- Castonguay, A., Gali, H. U., Perchellet, E. M., Gao, X. M., Boukharta, M., Jalbert, G., … Perchellet, J. (1997). Antitumorigenic and antipromoting activities of ellagic acid, ellagitannins and oligomeric anthocyanin and procyanidin. International Journal of Oncology, 10, 367–373.
- Ferrueloa, A., Romerob, I., Cabrerab, P. M., Aranceb, I., Andresb, G., & Angulob, J. C. (2014). Effects of resveratrol and other wine polyphenols on the proliferation, apoptosis and androgen receptor expression in LNCaP cells. Actas Urologicas Espanlas, 38(6), 397–404. doi: 10.1016/j.acuroe.2014.05.002
- Ghazanfari, T., Naseri, M., Shams, J., & Rahmati, B. (2013). Cytotoxic effects of Cuscuta extract on human cancer cell lines. Food and Agricultural Immunology, 24(1), 87–94. doi: 10.1080/09540105.2011.648608
- Guo, C. J., Wei, J. Y., Yang, J. J., Xu, J., Pang, W., & Jiang, Y. G. (2008). Pomegranate juice is potentially better than apple juice in improving antioxidant function in elderly subjects. Nutrition Research, 28, 72–77. doi: 10.1016/j.nutres.2007.12.001
- He, X. Y., Li, M. Y., Zhao, W., Liu, L., Kong, X. H., & Li, J. K. (2015). Chemical fingerprint and quantitative analysis for quality control of polyphenols extracted from pomegranate peel by HPLC. Food Chemistry, 176, 7–11. doi: 10.1016/j.foodchem.2014.12.040
- Johanningsmeier, S. D., & Harris, G. K. (2011). Pomegranate as a functional food and nutraceutical source. Annual Review of Food Science and Technology, 2, 181–201. doi: 10.1146/annurev-food-030810-153709
- Kim, S., Nishimoto, S. K., Bumgardner, J. D., Haggard, W. O., Gaber, M. W., & Yang, Y. Z. (2010). A chitosan/beta-glycerolphosphate thermo-sensitive gel for the delivery of ellagic acid for the treatment of brain cancer. Biomaterials, 31(14), 4157–4166. doi: 10.1016/j.biomaterials.2010.01.139
- Kwon, S. J. K., Lee, J. H., Moon, K. D., Jeong, I. Y., Yee, S. T., Lee, M. K., & Seo, K. I. (2014). Isoegomaketone induce apoptosis in SK-MEL-2 human melanoma cells through mitochondrial apoptotic pathway via activating the PI3K/Akt pathway. International Journal of Oncology, 45, 1969–1976. doi: 10.3892/ijo.2014.2598
- Lansky, E. P., & Newman, R. A. (2007). Punica granatum (pomegranate) and its potential for prevention and treatment of inflammation and cancer. Journal of Ethnopharmacology, 109, 177–206. doi: 10.1016/j.jep.2006.09.006
- Larrosa, M., Tomas-Barberan, F. A., & Espin, J. C. (2006). The dietary hydrolysable tannin punicalagin releases ellagic acid that induces apoptosis in human colon adenocarcinoma Caco-2 cells by using the mitochondrial pathway. The Journal of Nutritional Biochemistry, 17, 611–625. doi: 10.1016/j.jnutbio.2005.09.004
- Li, T. M., Chen, G. W., Su, C. C., Lin, J. G., Yeh, C. C., Cheng, K. C., & Chung, J. G. (2005). Ellagic acid induced p53/p21 expression, G1 arrest and apoptosis in human bladder cancer T24 cells. Anticancer Research, 25, 971–980.
- Li, X. Q., Shao, S. H., Fu, G. L., Han, X. H., & Gao, H. (2010). Study on norcantharidin-induced apoptosis in SMMC-7721 cells through mitochondrial pathways. Chinese Journal of Integrative Medicine, 16, 448–452. doi: 10.1007/s11655-010-0538-5
- Li, J. T., Zhang, J. L., He, H., Ma, Z. L., Nie, Z. K., Wang, Z. Z., & Xu, X. G. (2013). Apoptosis in human hepatoma HepG2 cells induced by corn peptides and its anti-tumor efficacy in H22 tumor bearing mice. Food and Chemical Toxicology, 51, 297–305. doi: 10.1016/j.fct.2012.09.038
- Li, G. X., Zhao, Y. H., Yu, C. Z., & Li, J. K. (2009). Composition of pomegranate peel polyphones and its antioxidant activities. Scientia Agricultura Sinica, 42, 4035–4041.
- Liu, W., Chen, J., Li, Q., & Sun, A. (2016). Inhibitory effects of acylated blueberry anthocyanin on H22 murine tumors. Food and Agricultural Immunology, 27(4), 509–522. doi: 10.1080/09540105.2015.1129599
- Malik, A., Afaq, F., Sarfaraz, S., Adhami, V. M., Syed, D. N., & Mukhtar, H. (2005). Pomegranate fruit juice for chemoprevention and chemotherapy of prostate cancer. Proceedings of the National Academy of Sciences, 102, 14813–14818. doi: 10.1073/pnas.0505870102
- Malik, A., Afaq, S., Shahid, M., Akhtar, K., & Assiri, A. (2011). Influence of ellagic acid on prostate cancer cell proliferation: A caspase-dependent pathway. Asian Pacific Journal of Tropical Medicine, 4, 550–555. doi: 10.1016/S1995-7645(11)60144-2
- Marel, A. K., Lizard, G., Izard, J. C., Latruffe, N., & Delmas, D. (2008). Inhibitory effects of trans-resveratrol analogs molecules on the proliferation and the cell cycle progression of human colon tumoral cells. Molecular Nutrition & Food Research, 52, 538–548. doi: 10.1002/mnfr.200700185
- Narayanan, B. A., Geoffroy, O., Willingham, M. C., Re, G. G., & Nixon, D. W. (1999). P53/p21(WAF1/CIP1) expression and its possible role in G1 arrest and apoptosis in ellagic acid treated cancer cells. Cancer Letters, 136, 215–221. doi: 10.1016/S0304-3835(98)00323-1
- Narayanan, B. A., & Re, G. G. (2001). IGF-II down regulation associated cell cycle arrest in colon cancer cells exposed to phenolic antioxidant ellagic acid. Anticancer Research, 21, 359–364.
- Rosenblat, M., Hayek, T., & Aviram, M. (2006). Anti-oxidative effects of pomegranate juice (PJ) consumption by diabetic patients on serum and on macrophages. Atherosclerosis, 187, 363–371. doi: 10.1016/j.atherosclerosis.2005.09.006
- Seeram, N. P., Aronson, W. J., Zhang, Y., Henning, S. M., Moro, A., Lee, R. P., … Heber, D. (2007). Pomegranate ellagitannin-derived metabolites inhibit prostate cancer growth and localize to the mouse prostate gland. Journal of Agricultural and Food Chemistry, 55, 7732–7737. doi: 10.1021/jf071303g
- Son, Y. O., Hitron, J. A., Wang, X., Chang, Q. S., Pan, J. J., & Zhang, Z. (2010). Cr(VI) induces mitochondrial-mediated and caspase-dependent apoptosis through reactive oxygen species-mediated p53 activation in JB6 Cl41 cells. Toxicology and Applied Pharmacology, 245, 226–235. doi: 10.1016/j.taap.2010.03.004
- Song, B. B., Li, J., & Li, J. K. (2016). Pomegranate peel polyphenols extract induced apoptosis in human hepatoma cells by mitochondrial pathway. Food and Chemical Toxicology, 93, 158–166. doi: 10.1016/j.fct.2016.04.020
- Syed, D. N., Afaq, F., & Mukhtar, H. (2007). Pomegranate derived products for cancer chemoprevention. Seminars in Cancer Biology, 17, 377–385. doi: 10.1016/j.semcancer.2007.05.004
- Trachootham, D., Alexandre, J., & Huang, P. (2009). Targeting cancer cells by ROS-mediated mechanisms: A radical therapeutic approach? Nature Reviews Drug Discovery, 8, 579–591. doi: 10.1038/nrd2803
- Tzulker, R., Glazer, I., Bar-Ilan, I., Holland, D., Aviram, M., & Amir, R. (2007). Antioxidant activity, polyphenol content, and related compounds in different fruit juices and homogenates prepared from 29 different pomegranate accessions. Journal of Agricultural and Food Chemistry, 55, 9559–9570. doi: 10.1021/jf071413n
- van Delft, M. F., & Huang, D. S. (2006). How the Bcl-2 family of proteins interact to regulate apoptosis. Cell Research, 16, 203–213. doi: 10.1038/sj.cr.7310028
- Wagner, H. (2006). Multitarget therapy – the future of treatment for more than just functional dyspepsia. Phytomedicine, 13, 122–129. doi: 10.1016/j.phymed.2006.03.021
- Wang, S. G., Huang, M. H., Li, J. H., Lai F.-I, Lee, H. M., & Hsu, Y. U. (2013). Punicalagin induces apoptotic and autophagic cell death in human U87MG glioma cells. ACTA Pharmacologica Sinica, 34(11), 1411–1419. doi: 10.1038/aps.2013.98