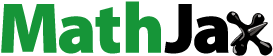
ABSTRACT
Anti-idiotypic antibody technology has been widely developed. Here, we prepared the F(ab’)2 fragments of the Cry1F toxin polyclonal antibody as Ab1and screened an anti-idiotype nanobody (VHH) for Bt Cry1F toxin from the camelid naive antibody phage display library. After three cycles of panning, six clones were isolated and subjected to indirect competitive enzyme-linked immunosorbent assay (IC-ELISA) for the detection of the Cry1F toxin. A competition inhibition rate of 47.00% exists between the anti-idiotype nanobody with the highest activity (5B) and Cry1F toxin for F(ab’)2 fragments. The binding abilities between the anti-idiotypic nanobody 5B and brush border membrane vesicles (BBMV) of Ostrinia furnacalis (Guenée) are also good. The anti-idiotypic nanobody 5B expressed through the prokaryotic system has the same activity as in phage form, and mortality compared to controls could reach 23.3% (P < 0.05). The material has the potential to simulate the Cry1F toxin to a certain extent.
1. Introduction
Cry1F, one of the second-generation Bacillus thuringiensis toxins, plays an important role in enhancing insecticidal activity, managing resistance and expanding the pesticidal spectrum. Maize and cotton expressing the cry1F gene have potential to control aboveground lepidopteran pests, including the larvae of the European corn borer (Ostrinia nubilais (Hübner)), Asian corn borer (O. furnacalis (Guenée)), fall armyworm (Spodoptera frugiperda), beet armyworm (S. exigua (Hübner)) and cotton bollworm (Helicoverpa zea) (Carrière et al., Citation2018; Vélez, Vellichirammal, Jurat-Fuentes, & Siegfried, Citation2016). Transgenic (GE) maize event TC1507 expressing the Cry1F toxin protein has been widely grown in the Americas, such as in Brazil and Argentina, since initially commercially grown in the United States in 2003 (Farias et al., Citation2014; Grimi et al., Citation2018). In 2006, reports of potential S. frugiperda resistance to TC1507 maize in Puerto Rico were received (Storer et al., Citation2010). Investigation revealed that pest populations showed high insensitivity to the Cry1F protein in bioassays, with resistance ratios likely in excess of 1000-fold (Storer, Kubiszak, Ed King, Thompson, & Santos, Citation2012). It took only three to four years for resistance to be detected in S. frugiperda, since the 2008 GE maize event TC1507 was introduced into Brazil (Farias et al., Citation2014). In recent years, it has been confirmed that Cry1F exhibits cross-resistance to a series of Bt Cry toxins. The Cry1F-resistant strain of the Asian corn borer showed moderate cross-resistance to Cry1Ab and Cry1Ac of 22.8- and 26.9-fold, respectively, under laboratory conditions (Wang et al., Citation2016). Shabbir et al. (Citation2018) verified that a Cry1Ah-resistant colony of the Asian corn borer showed high cross-resistance to Cry1F (464-fold). A high-dose/refuge strategy is still widely adopted to delay evolution of resistance of Cry1F and other Bt Cry toxins to GE crops, and applicable strategies for Bt pyramided plants should be carefully implemented (Shabbir et al., Citation2018; Vélez et al., Citation2016). In addition, it is necessary to seek a substitute of the Cry1F toxin for resistance management.
Anti-idiotypic antibodies (AId/Ab2) have the capacity to simulate antigen spatial structures and biological activity according to the immunological network theory formulated by Jerne (Citation1974). Ab2β, as one of the subtypes of Ab2, carrying an internal image of the original antigen, which could recognize the variable region of idiotypic antibodies, mimics the three-dimensional structure of original antigens, thereby interfering with antigen–antibody (Ab1) binding that is able to mimic the function of antigens. AId is employed as a vaccine, as potential serodiagnosis reagents in immunoassays and substations of highly toxic substances (Deyev & Lebedenko, Citation2009; Hu et al., Citation2017; Koprowski, Herlyn, Lubeck, DeFreitas, & Sears, Citation1984; Ruffini et al., Citation2014). In recent years, anti-idiotypic antibody technology has been used to prepare vaccines for hepatitis A, B, C and various diseases (Davtyan, Poghosyan, Sukiasyan, & Grant, Citation2013; Grant, Citation2002; Kiyohara et al., Citation2009; Yildirim, Başalp, Yücel, Manav, & Sezen, Citation2004). Eger et al. (Citation2016) also reported that a new human/mouse chimeric anti-idiotype Ab ganglidiximab may have the potential to tailor immune responses to the paratope regions mimicking GD2 overexpressed in neuroblastoma (NB) as well.
The selection of Ab2 from a phage display library is an alternative approach that is capable of physical association of the phenotype and genotype compared with traditional immune responses. As one form of display, nanobodies provide better research screening of anti-idiotypic antibodies from those libraries, due to the advantages of high solubility, high thermal stability, and ease of production. Additionally, nanobodies serve as substitutes of highly toxic and carcinogenic substances, such as fumonisin B1, aflatoxin B1, citrinin, ochratoxin and other toxins (Ren et al., Citation2019; Shu et al., Citation2019; Xu et al., Citation2015; Zhang, Zhang, Tang, Zhang, & Li, Citation2019).
There have been few studies on an anti-idiotypic antibody for Cry toxins. Qiu et al. (Citation2018) have developed a phage-mediated competitive chemiluminescent immunoassay (c-CLIA) for determination of the Cry1Ab toxin using anti-idiotypic camel nanobodies that were highly specific for Cry1Ab recognition. Liu et al. (Citation2018) screened anti-idiotypic single-chain variable fragments (ScFvs) of the Bt Cry2Aa toxin using the chain shuffling method, which showed superior competitive inhibition existed between anti-idiotypic scFvs and Cry2Aa. The binding abilities between anti-idiotypic scFvs with brush border membrane vesicles (BBMV) of Plutella xylostella larvae demonstrated that the anti-idiotypic scFvs could be used to map the binding epitope of the receptors in target insects instead of the Cry2Aa toxin.
In this study, anti-idiotypic nanobodies that specifically bind to F(ab’)2 fragments of anti-Cry1F polyclonal antibodies (pAbs) were successfully screened from a naive phage display nanobody library. Subsequently, competitive inhibition and binding activities of anti-idiotypic nanobodies were determined by ELISA. Expression of highly active anti-idiotypic nanobodies was used to further confirm the insecticidal activity.
2. Experimental section
2.1. Materials
Cry1F toxin standard was purchased from Envirologix Inc. (Portland, ME, USA). Freund's adjuvants (complete and incomplete) were obtained from Sigma (St. Louis, MO, USA). A Pierce™ F(ab’)2 Preparation Kit (44988) was purchased from Thermo Scientific (Rockford, IL, USA). HiTrap Protein A HP(1 mL) was purchased from GE Healthcare (Uppsala, Sweden). Horseradish peroxidase (HRP)-goat anti-rabbit IgG(H+L) was purchased from KPL (Milford, MA, USA). Horseradish peroxidase (HRP)-goat anti-rabbit IgG Fc (ab97196) was obtained from Abcam. The camelid naive single-domain antibody phage display library was provided by NB Biolab (Chengdu, Sichuan, China). E.coli TG1 was provided by our lab. T4 DNA ligase, Nco I and Not I were purchased from NEB. 2×High-Fidelity (Pfu) Master Mix was purchased from Tsingke Biological Technology (Beijing, China). DNA gel extraction spin kits, 6-well plates and 96-well plates were purchased from Corning (USA). Skim milk powder was obtained from Solabio (Beijing, China). All reagents were of analytical grade.
2.2. Production and purification of F(ab’)2 fragments of anti-Cry1F IgG from rabbits
Two female New Zealand white rabbits were employed for generating the immune response. One week before immunization, each rabbit was pre-bled to obtain control serum. The rabbits were inoculated with Cry1F toxin protein (0.8 mg) diluted in 0.8 mL carbonate buffered saline (CBS, 50 mM, pH 9.6) mixed with 0.8 mL complete Freund's adjuvant for the primary immunization and incomplete Freud's adjuvant for subsequent booster with an interval of two weeks. The serum containing polyclonal anti-Cry1F IgG was centrifuged at 5000 g for 10 min at 4°C, and stored at −80°C. The work was conducted with the formal approval of the Laboratory Animal Management Committee of Jiangsu Province (SCXK (Su) 2015–0020).
Anti-Cry1F IgG in antiserum was deposited by saturated ammonium sulfate for preliminary purification followed by application with 1 mL HiTrapTM Protein A HP and then purified pAbs was tested by indirect ELISA to evaluate the titers. The concentration of the purified antibodies was measured at 280 nm by use of Ultra-micro spectrophotometer (Thermo SMA2000, USA), and samples were stored in aliquots at −20°C for further use.
Production and purification of F(ab’)2 fragments of anti-Cry1F IgG were performed referring to the introduction of the F(ab’)2 Preparation Kit. Immobilized pepsin digested IgG to generate F(ab’)2 fragments. The NAb™ Protein A Plus Spin Column bound the large Fc fragments and undigested IgG, allowing the F(ab’)2 fragments to pass through the column for efficient purification. The purity and affinity of F(ab’)2 fragments were identified by sodium dodecyl sulfate polyacrylamide gel electrophoresis (SDS-PAGE) and indirect non-competitive ELISA, respectively (Beatty, Beatty, & Vlahos, Citation1987).
2.3. Panning of the VHH phage display library
Six-well plates were coated with 1 mL of 100 μg/mL of F(ab’)2 fragments (the concentrations of each subsequent round were cut in halved) in CBS buffer at 4°C overnight. The plates were washed three times with phosphate-buffered saline (PBS, 50 mmol/L, pH 7.4) containing 0.05% Tween (PBST,V/V) and were blocked with 3% skim milk powder in PBS (MPBS) followed by incubation at 37°C for 2 h. After washing three times, 1 mL of 1.023×108 cfu/mL camelid naive VHH phage display library in 3% MPBS were added to the wells and incubated at 37°C for 2 h with gentle rocking motion. Then, the plates were washed 10 times with 10 mL PBST buffer (20 times with 20 mL PBST buffer in each subsequent round). After the washing step, 100 µg/mL trypsin solution was added to elute the phage followed by incubation with gentle agitation for one hour at room temperature. The subsequent expanding culture, purification of phage by PEG6000/NaCl, and polyclonal and monoclonal phage ELISA were done according to the protocols of the Human Domain antibody (DAb) library.
2.4. Competitive phage ELISA for VHH activity
Two µg/mL of the Cry1F toxin diluted with CBS was coated in 96-well plates overnight at 4°C. On the second day, the plates were washed three times with PBST and were blocked by 3% MPBS and incubated for 1 h at 37°C. After the washing step, pre-incubated 10 µg/mL of 50 µL of F(ab’)2 fragments and 50 µL gradient volumes (0, 5, 10, 20, 30, 40, 50 µL) of phage supernatants (107 cfu/mL) were added to each well, followed by incubation for 1 h at 37°C. Then, the plates were washed, and 100 µL/well HRP-conjugated goat anti-rabbit IgG(H+L) monoclonal antibody (1:4000 diluted by PBS) was added and incubated for 1 h at 37°C. In conjunction with the washing step, 100 µL/well of the tetramethylbenzidine solution (100 µL of 10 mg/mL TMB-DMSO and 25 µL of 0.65% (V/V) H2O2 diluted with 10 mL of citrate-sodium citrate buffer (CPBS, 100 mM, pH 5.5)) were added and incubated for 15 min. The reaction was stopped with 50 µL/well of 2 M H2SO4, and absorbance was read by a microplate reader at 450 nm.
Dose–response curves were generated by OriginLab 2017 software. The inhibition ratio (Y) of mean values (n = 3) was plotted against the volumes of individual clones with phage form (X). The inhibition rate was calculated as follows.where A is the free phage, and B is the specimen to be tested.
2.5. ELISA binding analysis with brush border membrane vesicles
The brush border membrane vesicles (BBMV) were prepared from the midgut tissue of the fifth instar of O.furnacalis (Guenée) larvae referring to Tan et al. (Citation2013). Binding activity of the anti-idiotype phage nanobody of Cry1F toxin with BBMV was tested by non-competitive ELISA. The 96-well plates were coated with 10 µg/mL BBMV. Phages that had 107 titers with ten times echelon dilution (precipitated by PEG6000/NaCl and resolved in PBS) were added to the wells. The remaining steps were the same as in 2.4. MPBS used as a negative control.
2.6. Expression of the anti-idiotype nanobody (VHH)
The nucleotide sequence of the anti-idiotype nanobody (VHH) was measured by TSINGKE (Nanjing, China). In order to amplify the anti-idiotype nanobody gene, forward primer (Camel Nco I F: 5′ CATGCCATGGATCAGGTGCAGCTCGTG GAATCC 3′) with an Nco I site (underlined), and reverse primer (Camel Not I R: 5′ ATAGTTTAGCGGCCGCTGAGGAGACGGTGACCTG 3′) with a Not I site (underlined) were designed and synthesized by TSINGKE. The phagemid vectors with anti-idiotype nanobody gene amplification were used as the templates. The polymerase chain reaction (PCR) reaction mixture (50 μL) contained 25 μL of 2×High-Fidelity (Pfu) Master Mix, 1 μL of templates, 2 μL of primers and 20 μL of ddH2O. PCR was performed at 98°C for 2 min followed by 35 cycles (98°C for 30 s, 56°C for 30 s, 72°C for 30 s), and then 72°C for 2 min. PCR products were purified and then digested with Nco I and Not I overnight at 37°C. The digested PCR products were inserted into the pET-26b (+) expression vector, which was predigested with the same restriction endonuclease.
A freshly transformed E. coli BL21 (DE3) colony was cultured in 2×TY medium supplemented with 50 μg/mL kanamycin at 37°C overnight. This overnight culture was inoculated into 2 × TY medium at 7°C until the OD600 was 0.6–0.8. The expression of the anti-idiotype nanobody was induced with 1 mM IPTG consecutively cultivated overnight at 25°C and 220 rpm. Cells were analyzed by SDS-PAGE and Western blotting to verify the expression of the anti-idiotype nanobody (VHH). Cells harvested by centrifugation at 10000 g for 15 min at 4°C were suspended by PBS and lysed by sonication for 40 min at 100 W (3/4 s) to disrupt cell membranes and release cellular contents. The cell lysates were clarified by centrifugation at 12,000 g for 30 min. The inclusion body was dissolved and passed through a nickel-nitrilotriacetic acid (Ni-NTA) affinity column that was equilibrated with buffer A (0.5 M NaCl, 20 mM Tris, 8 M urea and 10 mM imidazole). After washing the captured column with five column volumes of buffer A, the specific bound protein was then eluted using a stepwise gradient of 100, 200, 300, 400 and 500 mM imidazole in buffer B (0.5 M NaCl, 20 mM Tris, 8 M urea and 500 mM imidazole). Fractions containing the target protein only were identified by SDS-PAGE and were dialyzed with renaturation solutions (6 M,4 M,2 M urea in PBS). After renaturation, the target protein was dialyzed with PBS to remove residual urea. The concentration of the anti-idiotype nanobody purified protein was determined using the Bradford method.
2.7. Bioassay for the anti-idiotype nanobody
The Bt-susceptible O. furnacalis (Guenée) purchased from Keyun Biotechnology Co. Ltd. (Henan, China) was bred using standard rearing techniques over 50 generations. The LC50 was 0.40 ± 0.012 µg/mL (P > 0.05) for Cry1F toxin detected during the second instar bioassay in our laboratory. Screened monoclonal phages with higher binding activity that had a titer of 1.067×107 cfu/mL (precipitated by PEG/NaCl 6000 and resolved in 3 mL PBS) and 400 µg/mL purified protein (the highest concentration after purification) were tested by mixing poison into the feed for bioassay. Two µg/mL of the Cry1F toxin and PBS were used as positive and blank controls, respectively.
3. Results
3.1. Production and purification of F(ab’)2 fragments
After five rounds of immunization, the first rabbit produced antisera against the Cry1F toxin with a titer of 8×106. The concentration of the purified pAbs was approximately 4 mg/mL. F(ab’)2 fragments of anti-Cry1F toxin IgG were obtained with a digestion process using immobilized pepsin and detected by SDS-PAGE and ELISA. The resulting F(ab’)2 in non-reducing and non-boiled SDS-PAGE derived from the rabbit migrated with a lower apparent molecular weight of 88 kDa; in reducing SDS-PAGE, F(ab’)2 fragments migrated near 26 kDa, and Fc fragments migrated at 28∼30 kDa (). Missing Fc fragments and F(ab’)2 fragments cannot specifically bind to Goat anti-Rabbit IgG Fc, but could specifically bind to Goat anti-Rabbit IgG(H+L). Goat-anti-Rabbit IgG Fc and Goat anti-Rabbit IgG(H+L) were used as secondary antibodies separately in indirect non-competitive ELISA to further confirm the activity and purity of the F(ab’)2 fragments (). The results proved that Fc fragments barely existed. Furthermore, the affinity constant (Kaff) of F(ab’)2 fragments was measured by non-competitive ELISA (), and was found to be 4.94×108 L/mol. This result proved that F(ab’)2 fragments have high binding affinity for the Cry1F toxin.
3.2. Panning of the VHH phage display library
After three rounds of the panning process, output phages did not increase significantly, and the absorbance of the outcome from the first round was higher than that of the original library and the two other rounds with polyclonal phage ELISA. As a result, a total of 4×96 phage clones randomly selected from the first round were analyzed by monoclonal phage ELISA, and six were identified to specifically bind to the F(ab’)2 fragments competing with the blank control MPBS (A). The phage clone 5B exhibited the highest sensitivity and was therefore selected for further research. The six individual clones were verified by PCR using specific primers, and the bands were all around 400 bp (B). The DNA sequencing results of the six phage clones are shown in C.
3.3. Competitive inhibition of phage ELISA
The results showed that the ratios of competitive inhibition increased with the addition of the 5B phage, but other samples had smaller changes. The 5B phage had a certain inhibitory effect between F(ab’)2 fragments and the Cry1F toxin with a rate of 47.00% (). This illustrated that anti-idiotype nanobody 5B had some characteristics of a simulated antigen.
3.4. Binding analysis of anti-idiotypic nanobody phage to BBMV of O. furnacalis (Guenée)
O. furnacalis (Guenée) is a target insect of the Cry1F toxin. To verify whether these anti-idiotype nanobodies bind to BBMV of O. furnacalis (Guenée) that could be used to map the toxin-binding site instead of the Cry1F toxin. In , the results showed that the absorbance values of 3G and 5B were positively correlated with the titer, but 5B was the strongest binder to BBMV of O. furnacalis (Guenée) larvae based on phage ELISA. This might have occurred due to the fact that 3G and 5B mimicked the toxin epitopes, which have different distances from or affinity to the binding site ().
3.5. Expression of the anti-idiotype nanobody (VHH)
It is difficult to quantify the phage display forms of anti-idiotypic nanobodies; based on the above results, the anti-idiotype nanobody 5B was selected for prokaryotic expression. 5Brecombinated and expressed with an N-terminal pelB signal sequence and a C-terminal His-tag. The strain containing the recombinant plasmid without IPTG was used as a negative control. The SDS-PAGE result showed that the induced E. coli BL21 (DE3) harbouring the 5B fragment had a specific protein band at about 20 KDa. As the anti-idiotype nanobody 5B was fused with His-tag, anti-His antibodies could be used to identify the 5B protein. After Western blotting, the test group presented only one band at around 20 KDa (), which was consistent with the SDS-PAGE result. The 5B protein was purified using an Ni-NTA column with a 300 mM imidazole section (). After renaturation, the target protein was yielded on quantity of 0.4 mg per litre of culture.
Figure 7. Western blotting results of the 5B protein that was confirmed by anti-His antibodies. Note. Lane M: Protein ladder; Lane 1: Negative control without IPTG; Lane 2: Inclusion body; Lane 3: Purified protein with 100 mM imidazole buffer; Lane 4: Purified protein with 200 mM imidazole buffer; Lane 5: Purified protein with 300 mM imidazole buffer.
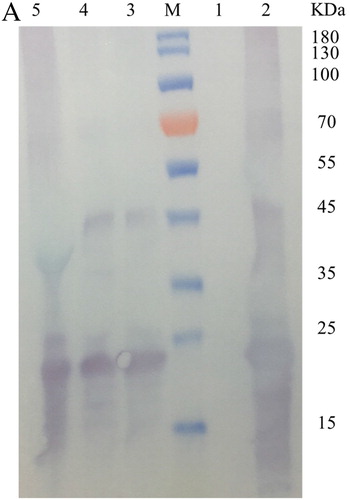
Figure 8. SDS-PAGE (12% Tris-MOPS) results of the 5B purified protein. Lane M: Protein ladder; Lane 1: Negative control without IPTG; Lane 2: Culture supernatants from centrifugation and sonication; Lane 3: Inclusion body; Lane 4: eluate solution passed through a nickel-nitrilotriacetic acid (Ni-NTA) affinity column; Lane 5: Purified protein with 300 mM imidazole buffer.
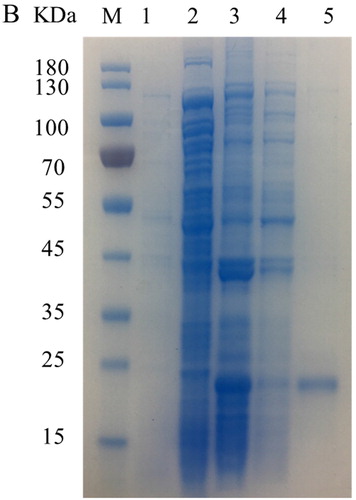
3.6. Bioassay for the anti-idiotype nanobody
The results of the bioassay revealed that the anti-idiotype nanobody 5B had certain insecticidal activity, with mortality rates of 15.7% (phage, P < 0.05) and 23.3% (protein, P < 0.05) in comparison the respective blank and positive controls ().
4. Conclusion
The current work obtained an anti-idiotypic nanobody with some potential to replace Cry1F toxin. The competitive inhibition rate of 5B phage against the Cry1F toxin reached 47.00%, and it had high binding activity with BBMV of O. furnacalis (Guenée). In addition, anti-idiotypic nanobody 5B expressed through prokaryotic system had the same activity as that in phage form, and mortality compared to controls could reach 23.3%.
Compared to the Cry1F toxin protein with three domains, anti-idiotypic nanobodies might simulate partial binding sites of one of the domains binding the receptors. There is lower similarity (1.57%) of amino acid sequences between activated-Cry1F toxin and anti-idiotype nanobody 5B (), but the 5B is very similar to the Domain II or Domain III region of Cry1F toxin in spatial structure, that there are two or three pairs of anti-parallel β folds (). Referring to the classic pore formation model of Cry1 toxins, the Domain II and Domain III of Cry1 toxins bind to the receptor proteins on the apical microvilli of epithelial cells lining the gut from larval midguts (Bravo, Gill, & Soberón, Citation2007; Gómez, Flores, Bravo, & Soberón, Citation2015; Muñoz-Garay et al., Citation2006), then the toxin undergoes a conformational change that permits insertion of a helical hairpin of Domain I into the cell membrane. Finally, association with additional toxin molecules through oligomerization leads to the formation of a pore. Ion flux through the pore leads to osmotic cell lysis and eventual death of the susceptible insects (Hua, Masson, Jurat-Fuentes, Schwab, & Adang, Citation2001; Masson, Tabashnik, Liu, Brousseau, & Schwartz, Citation1999). The 5B without perforated structural Domain shows lower insecticidal activity, but it has the better binding activity with BBMV of O.furnacalis (Guenée). If the pore formation domain is equipped, it is possible to improve insecticidal activity of the anti-idiotypic nanobody. So, the results provide a basis for subsequent structural modification in order to improve its biological activity, it also contributes an idea in developing new pesticide products.
Disclosure statement
No potential conflict of interest was reported by the authors.
Additional information
Funding
References
- Beatty, J. D., Beatty, B. G., & Vlahos, W. G. (1987). Measurement of monoclonal antibody affinity by non-competitive enzyme immunoassay. Journal of Immunological Methods, 100, 173–179. doi: 10.1016/0022-1759(87)90187-6
- Bravo, A., Gill, S. S., & Soberón, M. (2007). Mode of action of Bacillus thuringiensis Cry and Cyt toxins and their potential for insect control. Toxicon, 49, 423–435. doi: 10.1016/j.toxicon.2006.11.022
- Carrière, Y., Degain, B. A., Unnithan, G. C., Harpold, V. S., Heuberger, S., Li, X., & Tabashnik, B. E. (2018). Effects of seasonal changes in cotton plants on the evolution of resistance to pyramided cotton producing the Bt toxins Cry1Ac and Cry1F in Helicoverpa zea. Pest Management Science, 74, 627–637. doi: 10.1002/ps.4746
- Davtyan, T. K., Poghosyan, D. A., Sukiasyan, A. G., & Grant, M. D. (2013). The anti-idiotypic antibody 1F7 stimulates monocyte interleukin-10 production and induces endotoxin tolerance. Journal of Inflammation, 10, 14. doi: 10.1186/1476-9255-10-14
- Deyev, S. M., & Lebedenko, E. N. (2009). Modern technologies for creating synthetic antibodies for clinical application. Acta Naturae, 1, 32–50. doi: 10.32607/20758251-2009-1-1-32-50
- Eger, C., Siebert, N., Seidel, D., Zumpe, M., Jüttner, M., Brandt, S., … Lode, H. N. (2016). Generation and characterization of a human/mouse chimeric GD2-mimicking anti-idiotype antibody ganglidiximab for active immunotherapy against neuroblastoma. PLoS One, 11, e0150479. doi: 10.1371/journal.pone.0150479
- Farias, J. R., Andow, D. A., Horikoshi, R. J., Sorgatto, R. J., Fresia, P., Dos Santos, A. C., & Omoto, C. (2014). Field-evolved resistance to Cry1F maize by Spodoptera frugiperda (Lepidoptera: Noctuidae) in Brazil. Crop Protection, 64, 150–158. doi: 10.1016/j.cropro.2014.06.019
- Gómez, I., Flores, B., Bravo, A., & Soberón, M. (2015). Bacillus thuringiensis Cry1AbMod toxin counters tolerance associated with low cadherin expression but not that associated with low alkaline phosphatase expression in Manduca sexta. Peptides, 68, 130–133. doi: 10.1016/j.peptides.2014.08.012
- Grant, M. D. (2002). Antibody convergence along a common idiotypic axis in immunodeficiency virus and hepatitis C virus infections. Journal of Medical Virology, 66, 13–21. doi: 10.1002/jmv.2105
- Grimi, D. A., Parody, B., Ramos, M. L., Machado, M., Ocampo, F., Willse, A., … Head, G. (2018). Field-evolved resistance to Bt maize in sugarcane borer (Diatraea saccharalis) in Argentina. Pest Management Science, 74, 905–913. doi: 10.1002/ps.4783
- Hu, L., Liu, A., Chen, W., Yang, H., Wang, X., & Chen, F. (2017). A non-toxic enzyme-linked immunosorbent assay for aflatoxin B1 using anti-idiotypic antibodies as substitutes. Journal of the Science of Food and Agriculture, 97, 1640–1645. doi: 10.1002/jsfa.7914
- Hua, G., Masson, L., Jurat-Fuentes, J. L., Schwab, G., & Adang, M. J. (2001). Binding analyses of Bacillus thuringiensis Cry δ-endotoxins using brush border membrane vesicles of Ostrinia nubilalis. Applied and Environmental Microbiology, 67, 872–879. doi: 10.1128/AEM.67.2.872-879.2001
- Jerne, N. K. (1974). Towards a network theory of the immune system. Annual Immunology, 125C, 373–389.
- Kiyohara, T., Totsuka, A., Yoneyama, T., Ishii, K., Ito, T., & Wakita, T. (2009). Characterization of anti-idiotypic antibodies mimicking antibody- and receptor-binding sites on hepatitis A virus. Archives of Virology, 154, 1263–1269. doi: 10.1007/s00705-009-0433-6
- Koprowski, H., Herlyn, D., Lubeck, M., DeFreitas, E., & Sears, H. F. (1984). Human anti-idiotype antibodies in cancer patients: Is the modulation of the immune response beneficial for the patient? Proceedings of the National Academy of Sciences, 81, 216–219. doi: 10.1073/pnas.81.1.216
- Liu, Y., Lin, M., Wu, J., Hu, X., Zhang, X., Xu, C., … Liu, X. (2018). Generation of panels of anti-idiotypic single-chain variable fragments mimicking Cry2Aa toxin using the chain shuffling technique. Food and Agricultural Immunology, 29, 735–743. doi: 10.1080/09540105.2018.1440535
- Masson, L., Tabashnik, B. E., Liu, Y. B., Brousseau, R., & Schwartz, J. L. (1999). Helix 4 of the Bacillus thuringiensis Cry1Aa toxin lines the lumen of the ion channel. Journal of Biological Chemistry, 274, 31996–32000. doi: 10.1074/jbc.274.45.31996
- Muñoz-Garay, C., Sánchez, J., Darszon, A., de Maagd, R. A., Bakker, P., Soberón, M., & Bravo, A. (2006). Permeability changes of manduca sexta midgut brush border membranes induced by oligomeric structures of different cry toxins. The Journal of Membrane Biology, 212, 61–68. doi: 10.1007/s00232-006-0003-8
- Qiu, Y., Li, P., Dong, S., Zhang, X., Yang, Q., Wang, Y., … Liu, X. (2018). Phage-mediated competitive chemiluminescent immunoassay for detecting Cry1Ab toxin by using an anti-idiotypic camel nanobody. Journal of Agricultural and Food Chemistry, 66, 950–956. doi: 10.1021/acs.jafc.7b04923
- Ren, X., Zhang, Q., Wu, W., Yan, T., Tang, X., Zhang, W., … Li, P. (2019). Anti-idiotypic nanobody-phage display-mediated real-time immuno-PCR for sensitive, simultaneous and quantitative detection of total aflatoxins and zearalenone in grains. Food Chemistry, 297, 124912. doi: 10.1016/j.foodchem.2019.05.186
- Ruffini, P. A., Os, A., Dolcetti, R., Tjønnfjord, G. E., Munthe, L. A., & Bogen, B. (2014). Targeted DNA vaccines eliciting crossreactive anti-idiotypic antibody responses against human B cell malignancies in mice. Journal of Translational Medicine, 12, 207. doi: 10.1186/1479-5876-12-207
- Shabbir, M. Z., Quan, Y., Wang, Z., Bravo, A., Soberón, M., & He, K. (2018). Characterization of the Cry1Ah resistance in Asian corn borer and its cross-resistance to other Bacillus thuringiensis toxins. Scientific Reports, 8, 234. doi: 10.1038/s41598-017-18586-2
- Shu, M., Xu, Y., Dong, J., Zhong, C., Hammock, B. D., Wang, W., & Wu, G. (2019). Development of a noncompetitive idiometric nanobodies phage immumoassay for the determination of fumonisin B1. Food and Agricultural Immunology, 30, 510–521. doi: 10.1080/09540105.2019.1604637
- Storer, N. P., Babcock, J. M., Schlenz, M., Meade, T., Thompson, G. D., Bing, J. W., & Huckaba, R. M. (2010). Discovery and characterization of field resistance to Bt maize: Spodoptera frugiperda (Lepidoptera: Noctuidae) in Puerto Rico. Journal of Economic Entomology, 103, 1031–1038. doi: 10.1603/EC10040
- Storer, N. P., Kubiszak, M. E., Ed King, J., Thompson, G. D., & Santos, A. C. (2012). Status of resistance to Bt maize in Spodoptera frugiperda: Lessons from Puerto Rico. Journal of Invertebrate Pathology, 110, 294–300. doi: 10.1016/j.jip.2012.04.007
- Tan, S. Y., Cayabyab, B. F., Alcantara, E. P., Huang, F., He, K., Nickerson, K. W., & Siegfried, B. D. (2013). Comparative binding of Cry1Ab and Cry1F Bacillus thuringiensis toxins to brush border membrane proteins from Ostrinia nubilalis, Ostrinia furnacalis and Diatraea saccharalis (Lepidoptera: Crambidae) midgut tissue. Journal of Invertebrate Pathology, 114, 234–240. doi: 10.1016/j.jip.2013.08.007
- Vélez, A. M., Vellichirammal, N. N., Jurat-Fuentes, J. L., & Siegfried, B. D. (2016). Cry1F resistance among lepidopteran pests: A model for improved resistance management? Current Opinion in Insect Science, 15, 116–124. doi: 10.1016/j.cois.2016.04.010
- Wang, Y., Wang, Y., Wang, Z., Bravo, A., Soberón, M., & He, K. (2016). Genetic basis of Cry1F-resistance in a laboratory selected Asian corn borer strain and its cross-resistance to other Bacillus thuringiensis toxins. PLoS One, 11(8), e0161189. doi: 10.1371/journal.pone.0161189
- Xu, Y., Xiong, L., Li, Y., Xiong, Y., Tu, Z., Fu, J., & Chen, B. (2015). Anti-idiotypic nanobody as citrinin mimotope from a naive alpaca heavy chain single domain antibody library. Analytical and Bioanalytical Chemistry, 407, 5333–5341. doi: 10.1007/s00216-015-8693-3
- Yildirim, T., Başalp, A., Yücel, F., Manav, Aİ, & Sezen, İY. (2004). Generation of anti-idiotypic antibodies that mimic HBsAg and vaccination against hepatitis B virus. Hybridoma and Hybridomics, 23, 192–197. doi: 10.1089/1536859041224307
- Zhang, C., Zhang, Q., Tang, X., Zhang, W., & Li, P. (2019). Development of an anti-idiotypic VHH antibody and toxin-free enzyme immunoassay for ochratoxin A in cereals. Toxins, 11, 280. doi: 10.3390/toxins11050280