ABSTRACT
This study was to investigate the effects of peptides derived from fermented soybean meal (FSP) on intestinal inflammation and epithelial barrier function of the piglets. Compared to fed soybean meal, piglets fed with fermented soybean meal (FSBM) exhibit lower serum levels of IL-6, IL-1β and D-lactate, and reduced ileum macrophage infiltration. Besides, the FSBM up-regulated (p < 0.05) expression of mucins and tight junction (TJ) proteins in the jejunum and ileum of piglets. The FSP were prepared with alkali extraction and a 3-kDa molecular weight cut-off membrane system. In vitro, FSP reducing the mRNA expression of IL-6, IL-1β, IL-8 and attenuated Escherichia coli K88-induced inflammation in the intestinal porcine epithelial cells-J2 (IPEC-J2). Furthermore, FSP increased (p < 0.05) the expression of TJ proteins and transepithelial electrical resistance in IPEC-J2 cells. These results suggest that FSP could improve small intestinal health by enhancing epithelial barrier function and suppressing inflammation.
1. Introduction
Soybean peptides, the specific protein fragments derived from soybean hydrolysate, are potential components of health-promoting food (Sanjukta & Rai, Citation2016). These bioactive peptides are not only considered to have great nutritive value, but also contribute to various physiological functions through their anti-hypertensive, anti-oxidative, immunomodulatory, anti-diabetic, hypocholesterolemic, anti-cancer, and anti-obesity effects (Korhonen & Pihlanto, Citation2003). Soybean meal (SBM) is a by-product of soybean oil processing, containing significant amounts of soy protein (45.0%–55.0%), 80.0% of which is water-soluble (Wang, Zhang, Sun, & Dai, Citation2013). Fermentation can effectively degrade macromolecule proteins into small peptides; compared to SBM, fermented soybean meal (FSBM) was reported to modulate the expression of genes related to inflammatory responses in piglets (Roh, Carroll, & Kim, Citation2015).
Some fermented feeds were reported to improve intestinal barrier function and immune function in chicken and mouse models (Rokana, Singh, Mallappa, Batish, & Grover, Citation2016; Zhang, Zhu, Rahman, Jin, & Rong, Citation2016). Bioactive peptides derived from food were also demonstrated to increase the proliferation of intestinal epithelial cells and promote intestinal barrier function (Olga, Belén, Cristina, & Fermín, Citation2014). Lunasin, a soy-derived peptide, was proved to have potential anti-cancer and anti-inflammatory properties (Blanca, Chia-Chien, & De Lumen, Citation2009; Dia, Wang, Oh, Lumen, & Mejia, Citation2009). It can inhibit the pro-inflammatory response in lipopolysaccharide (LPS)-induced macrophages by suppressing the NF-κB pathway (Chang et al., Citation2014). Moreover, a 5-kDa peptide from soybean was shown to decrease the expression of most pro-inflammatory cytokines including interleukin-6 (IL-6), cyclooxygenase-2, and inducible nitric oxide, in addition to p50 nuclear translocation (Mejia & Dia, Citation2009). However, little information is available regarding the role of peptides derived from fermented soybean meal in the moderation of intestinal epithelial cells and the immune system.
Intestinal inflammation is a common condition in the swine as it is in human beings. Due to their defective intestinal systems, young piglets are often challenged by nutritional, immunological and psychological disruptions after being weaned (Hu, Xiao, Luan, & Song, Citation2013; Moeser et al., Citation2007). In this stage, piglets will suffer from intestinal mucosal inflammation with increased expression of pro-inflammatory cytokines tumor necrosis factor-α (TNF-α), IL-6, IL-8, IL-1β and activation of NF-κB or MAPK signalling pathways (Wittkopf, Neurath, & Becker, Citation2014; Yi et al., Citation2016). The intestinal barrier is an important line of defense against bacteria or toxin invasion that is culpable for intestinal inflammation (Müller, Autenrieth, & Peschel, Citation2005). Consisting of intestinal epithelial cells and tight junctions, epithelial barrier is the first line of defense. (Magalhaes, Tattoli, & Girardin, Citation2007; Maria, Citation2011). In addition, tight junction (TJ) proteins including zonula occluden (ZO), occludin, claudin etc also play a vital role in intestinal barrier function.
According to Zhu et al. (Citation2017), among the four different dietary treatments (each containing 0%, 5%, 10% and 15% FSBM, respectively), 10% FSBM diet was proved to be most effective in improving immunity and intestinal health in piglets. Roh et al. (Citation2015) also proved that 10% FSBM diet can modulate the expression of genes related to inflammatory responses in pigs. In this study, we set the level of FSBM at 10% in the experiment group to observe the effect of fermented soybean meal on intestinal inflammation and epithelial barrier function in piglets. A model of E. coli K88-infected intestinal porcine epithelial cells-J2 (IPEC-J2) had been applied to further explicate the effect of peptides from FSBM on inflammation and epithelial barrier function.
2. Materials and methods
2.1. Soybean meal and strain
Soybean meal was obtained from Cofine Bio-tech Co. Ltd. (Jiaxing, China). The fermenting strain Bacillus subtilis BS12 (B. subtilis BS12) was isolated from preserved vegetables obtained from a local market (Shaoxing, China).
2.2. Preparation of soybean peptides
Soybean meal (SBM) was fermented with B. subtilis BS12 according to the former research (Zhang et al., Citation2017). Briefly, SBM was sterilized at 105°C for 20 min and inoculated with 5% (w v−1) strain culture at 37°C for 24 h. After fermentation, SBM and FSBM were dried by a freeze-dryer and homogenized to pass through a 60-mesh screen. The peptides prepared according to Rayaprolu S J (Rayaprolu, Hettiarachchy, Chen, Kannan, & Mauromostakos, Citation2013). The material was then dissolved in distilled water and protein isolates were prepared by alkali extraction method (pH 9.5 at room temperature for 3 h) with 3N NaOH solution. The supernatants were separated by centrifugation at 5000 g for 10 min and large molecular weight protein were removed with isoelectric precipitation at pH 4.5. The final pH of supernatants was adjusted to 7.0 and freezed dried to obtain crude peptides. Then the crude peptides were dissolved with DI water (1:10 w/v) and filtered through a 0.22-μm membrane filter to remove impurities. The supernatant was fractionated through an ultrafiltration centrifuge tube (Millipore, USA) using a membrane with a 3-kDamolecular weight cut-off and the fractions were collected as soybean meal peptides (SP) and fermented soybean meal peptides (FSP).
2.3. Chemical analysis
The chemical analysis was conducted according to the former study (Zhang et al., Citation2017). Briefly, the dry matter, crude protein, crude fat, ash of SBM, FSBM was according to AOAC (2005). The content analysis of β-conglycinin and glycinin in SBM and FSBM was using an ELISA kit (Longzhoufangke Bio Co., Beijing, China) according to manufacturer’s protocol. The concentration of peptides was quantified using a BCA assay (KeyGEN BioTECH, China) according to the manufacturer’s instructions. The amino acid composition was determined using an Automatic Amino Acid Analyzer (Kowa, Japan).
2.4. Animal and sample collection
All animal experiments were approved by the Animal Care Committee of Zhejiang University and were conducted in accordance with the Guidelines for the Care and Use of Agricultural Animals for Research and Teaching at Zhejiang University. A total of 192 piglets (Duroc × Landrace × Yorkshire) weaned at a body weight of 9.35 ± 0.65 kg were selected. Experimental animals were randomly assigned to two dietary treatments, and each treatment was replicated using six pens, each containing 16 pigs. The two treatments were as follows: (1) commercial feed with 20% SBM (control group); (2) 10% replacement of SBM with FSBM in commercial feed (FSBM group). Both diets were formulated to have similar nutrient content and met or exceeded the nutrient requirements estimated by NRC (Citation2012). All piglets were allowed access to feed and water ad libitum. After 24 days of feeding, blood samples were collected from the anterior vena cava into coagulation accelerator tubes and serum was obtained after centrifugation at 3000 × g for 10 min at 4°C. Jejunum and ileum samples (0.5 × 0.5 cm) were taken and stored immediately at −80°C.
2.5. Cell culture
IPEC-J2 cells were cultured in DMEM-F12 medium (Yuanpei, China) supplemented with 10% fetal bovine serum (Gibco) and antibiotics (100 U mL−1 penicillin and 100 μg mL−1 streptomycin sulfate) at 37°C with 5% CO2 in a humidified incubator before treatment. Cells were incubated with medium only, E. coli K88 (multiplicity of infection (MOI) = 10:1 for 2 h), or FSP + E. coli K88 (pretreated with 50 μg mL−1 fermented soybean peptides and then E. coli K88 at MOI = 10:1 for 2 h). Cells were collected for real-time PCR and western blotting.
IPEC-J2 cells were cultured in transwell dishes for at least 21 days until transepithelial electrical resistance (TER) was stable. IPEC-J2 monolayers were incubated with medium only, E. coli K88 (MOI = 10:1 for 2 h), FSP + E. coli K88 (pretreated with 50 μg mL−1 fermented soybean peptides and then E. coli K88 at MOI = 10:1 for 2 h) in the upper layer. TER was measured using a Millicell ERS-2 epithelial volt-ohm meter (Millipore MERS00002, USA).
2.6. MTT assays
MTT assays were performed according to the manufacturer’s instructions. Briefly, IPEC-J2 cells were cultured in 96-well cell culture plates and incubated with various concentrations of FSP (1, 2, 5, 10, 20, 50, 100, 150, 200 μg mL−1) for 12 h. Then, each well was incubated with MTT for 4 h, which was dissolved with DMSO. The OD was measured at 450 nm and cell proliferation was calculated.
2.7. Enzyme-linked immunosorbent assay (ELISA)
The levels of IL-6 and IL-1β in serum were determined using ELISA kits (Jiancheng, China). In addition, the level of D-Lactate and diamine oxidase (DAO, Enzyme Commission number 1.4.3.6) in serum were determined using ELISA kits (R&D, USA). Samples were measured according to the manufacturer’s instructions.
2.8. Real-time PCR
Total RNA was extracted using Trizol reagent (Invitrogen, USA), and 2 μg of RNA was reversed-transcribed using the Trainscript Reagent (Thermo Fisher Scientific, MA, USA). Real-time PCR was performed using a StepOne PlusTM system (Applied Biosystems, CA, USA). The sequences of primers for real-time PCR are listed in . 18S RNA was used as an internal control. Relative mRNA expression was calculated using the 2−ΔΔCt method.
Table 1. Primers used for real-time PCR in this study.
2.9. Western blotting
Total protein was extracted with RAPI buffer and then quantified using the BCA Protein Assay kit (KeyGEN BioTECH, China). Proteins of different molecular weights were separated by 10% sodium dodecyl sulfate-polyacrylamide gel electrophoresis, followed by western blotting. After incubation in 5% skimmed milk at room temperature for 1 h, the membranes were incubated with primary antibodies overnight at 4°C, followed by incubation for 1 h with HRP-conjugated anti-rabbit or anti-mouse IgG. Primary antibodies specific for β-actin (Abcam, USA), phosphorylated NF-κB p65 (Santa Cruz, USA), phosphorylated IκB-α (Epitomics, USA), phosphorylated MAPK p38 (Boston, USA), ZO-1 (Abcam, USA), occludin (Abcam, USA), and claudin-1 (Abcam, USA) were purchased. Anti-rabbit-IgG and anti-mouse-IgG were used as secondary antibodies. The Western blots were observed using ECL (Amersham Pharmacia Biotech, France) and quantified by Image J software.
2.10. Immunohistochemistry
Sections of jejunum and ileum were deparaffinized and rehydrated. The sections were submitted to antigen retrieval using EDTA buffer (pH = 9.0) and a microwave, and then incubated with 3% hydrogen dioxide in the dark for 30 min. Next, the sections were incubated with a primary antibody (1:200 dilution) specific for Mac2 (Google Biotechnology Inc., China) to detect macrophage infiltration. Sections were then incubated with secondary antibody (1:200 dilution) and treated with DAB substrate. Nuclei were stained with Harris hematoxylin. Images were obtained using a DM3000 microscope.
2.11. Statistical analysis
Statistical analysis was performed using a one-way ANOVA or Student’s t-test with SPSS 19.0 software (SPSS, Inc., Chicago, IL, USA). Data were presented as mean ± SEM. Differences were considered significant at p values < 0.05.
3. Results
3.1. FSBM attenuates inflammation in piglets
Compared to the control group, adding 10% FSBM to the diet significantly reduced serum levels of the pro-inflammatory cytokine IL-1β, whereas there was no difference in the serum level IL-6 between the two groups ((A)). As revealed by real-time PCR, FSBM significantly increased the mRNA expression of antibacterial proteins MUC-1 and MUC-2 in the jejunum and ileum of piglets ((B) and (C)). Moreover, the inflammatory cell infiltration in the intestine is correlated with intestinal inflammation, and the level of Mac2 directly reflects the number of macrophages (Bruun, Helge, Richelsen, & Stallknecht, Citation2006; Wright, Moots, Bucknall, & Edwards, Citation2010). The immunohistochemistry results demonstrated that FSBM effectively decreased macrophage infiltration in the jejunum and ileum of piglets compared to that in the control group ((D) and (E)). These results indicated soybean meal fermented with Bacillus subtilis BS12 relieved intestinal inflammation in young piglets by reducing pro-inflammatory cytokine level, moderate mRNA expression and decrease macrophage infiltration.
Figure 1. FSBM attenuates inflammation in young piglets. (A) FSBM significantly decreased serum levels of IL-1β, but not IL-6, as assessed by ELISA. (B and C) Real-time PCR indicated that FSBM increased the expression of MUC-2 in the jejunum (B) and MUC-1 and MUC-2 in the ileum (C). Immunohistochemistry analysis of Mac2 expression was used to assess macrophage infiltration (D) FSBM attenuated macrophage infiltration into jejunum compared to that in controls. (E) FSBM attenuated macrophage infiltration into ileum compared to that in controls. FSBM, fermented soybean meal. Bars represent mean ± S.D. (n = 6); *: p < 0.05 vs control group.
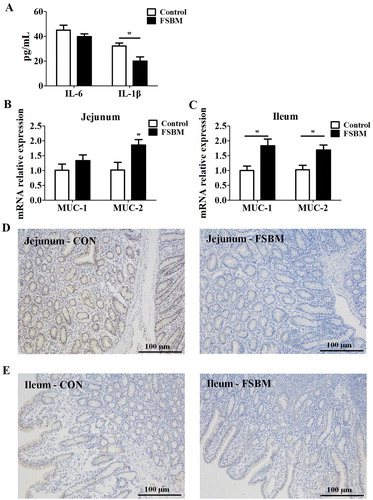
3.2. FSBM improves intestinal epithelial barrier functions in piglets
Intestinal barrier function is an important line of defense against bacteria or toxin invasion, both of which can result in intestinal inflammation (Müller et al., Citation2005). The levels of D-lactate and DAO were used as an index to directly reflect intestinal mucosal injury in weaned piglets (Jiao et al., Citation2015). Compared to that in the control group, diets containing 10% FSBM significantly reduced serum levels of D-lactate in piglets, whereas the level of DAO was not different between the two groups ((A) and (B)). In addition, we performed western blot analysis to detect expression of ZO-1, occludin, and claudin-1. Compared to SBM in the control group, FSBM significantly increased ZO-1, occludin, and claudin-1 expression in the jejunum ((B)), and increased ZO-1 and claudin-1 expression in the ileum ((C)).
Figure 2. FSBM improves intestinal epithelial barrier functions in piglets. (A) FSBM significantly reduced serum levels of D-lactate in serum, as assessed by ELISA. (B) Based on ELISA, there was no difference in DAO levels between the two groups. (C) FSBM effectively increased the expression of TJ proteins ZO-1, occludin, and claudin-1 in the jejunum based on western blot analysis. (D) Quantitative analysis of TJ proteins in the jejunum. (E) FSBM increased the expression of TJ proteins ZO-1 and claudin-1 in the ileum as assessed by western blotting. (F) Quantitative analysis of TJ proteins in the ileum. FSBM, fermented soybean meal. Bars represent mean ± S.D. (n = 6); *: p < 0.05, vs control group.
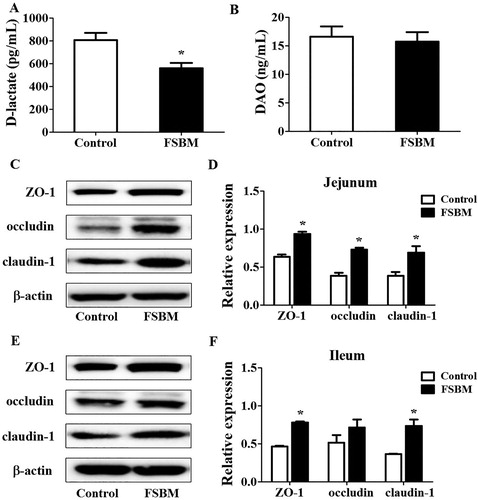
3.3. Chemical composition of FSBM
An analysis of the chemical composition in SBM and FSBM demonstrated that in FSBM, crude protein was increased by 12.57% and peptides was increased by over six folds, whereas Ether extract content was decreased by 27.14% when compared against SBM (). Moreover, fermentation reduced the level of the antigenic protein Glycinin and β-conglycinin by 86.79% and 80.18%, respectively.
Table 2. Nutrient composition of SBM and FSBM.
3.4. Amino acids composition of peptides in FSBM
Amino acid analysis showed that the amino acids compositions in SP and FSP were similar. Asparagine and glutamic acid were major amino acids in both SP and FSP (Dilshat, Parida, Nurmuhammat, Kimono, & Nobuo, Citation2012; Liu, Lv, Xu, & Guo, Citation2016). In this study, the contents of isoleucine, leucine, methionine, and valine in FSP were increased by 34.74%, 47.31%, 109.80%, and 30.4%, respectively, compared to SP (). Isoleucine, leucine and valine were essential branched-chain amino acids. The increase of branched-chain amino acids would facilitate the absorption of peptides by intestinal peptide transporters (Matthews, Citation1990). Methionine is an essential amino acid playing crucial roles in mammalian metabolism such as protein synthesis, methylation of DNA and polyamine synthesis (Cavuoto & Fenech, Citation2012). The synthetic peptides containing an amino terminal formyl-methionine residue could elicit immune reactivity directed towards M. tuberculosis (Dow et al., Citation2000). Methionine enkephalin could suppress TLR7-MyD88-TRAF6-NF-κB p65 signalling pathways and treat influenza A virus infection in mice (Tian et al., Citation2017). With the increasing of branched-chain amino acids and methionine in FSP, we speculated that FSP could exhibit immune regulation effect in host intestinal immune system.
Table 3. The amino acid composition of peptides (%).
3.5. Determination of effective FSP concentration in E. coli K88-infected IPEC-J2 cells
Regarding the effective dose for FSP in IPEC-J2 cells, we determined that concentrations less than 100 μg mL−1 had no significant effect on cell viability based on MTT assays ((A)). Furthermore, FSP displayed an inhibition effect on IL-6 production depending on its concentration in E. coli K88-infected IPEC-J2 cells. We found that 50 μg mL−1 FSP could effectively suppress IL-6 levels, and recommend this concentration to be used for further experiments ((B)).
Figure 3. Determination of effective fermented soybean peptide concentrations in IPEC-J2 cells. (A) FSP less than 100 μg mL−1 had no effect on cell viability, as assessed by MTT assays. (B) Based on ELISA, FSP inhibited IL-6 levels in a concentration-dependent manner in E. coli K88-infected IPEC-J2 cells and 50 μg mL−1 was determined to be an effective dose. FSP, small peptides in fermented soybean meal. Bars represent mean ± S.D. (n = 3); *: p < 0.05 vs control group; *: p < 0.05 vs E. coli K88.
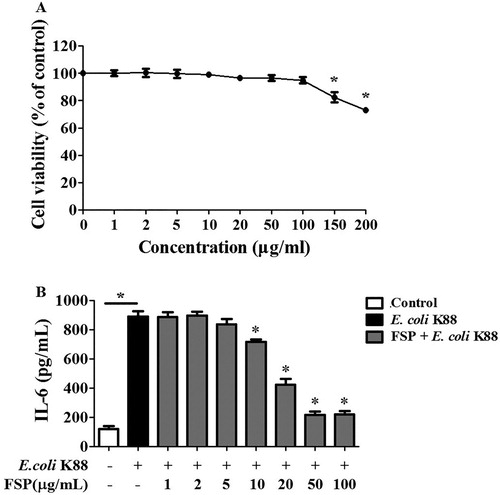
3.6. Effects of FSP on E. coli K88-induced inflammation in IPEC-J2 cells
To verify the effects of FSP on immunomodulation, we measured the levels of pro-inflammatory IL-1β, IL-8, IL-22, and tumor necrosis factor α (TNF-α) with real-time PCR. We found that FSP significantly reduced the mRNA expression of IL-1β, IL-8, and TNF-α in E. coli K88-infected IPEC-J2 cells ((A)). In addition, phosphorylation of NF-κB, IκB-α, and p38 MAPK was effectively inhibited with FSP pre-treatment, compared to that in control E. coli K88-treated IPEC-J2 cells ((B)).
Figure 4. Effects of fermented soybean peptides on E. coli K88-induced inflammation in IPEC-J2 cells. (A) FSP significantly decreased the expression of IL-1β, IL-8, and TNF-α in E. coli K88-infected cells, as determined by real-time PCR. (B) FSP significantly suppressed the phosphorylation of NF-κB, IκB-α, and p38 in E. coli K88-infected cells, as determined by western blotting. (C) Quantitative analysis of key proteins, NF-κB, IκB-α, and p38, in IPEC-J2 cells. FSP, small peptides in fermented soybean meal. Bars represent mean ± SEM (n = 3); *: p < 0.05 vs control group; *: p < 0.05, vs E. coli K88 group.
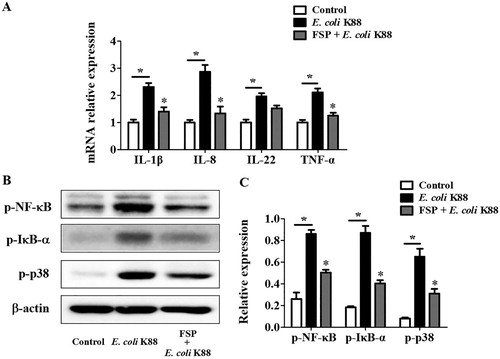
3.7. Effects of FSP on epithelial barrier function in E. coli K88-infected IPEC-J2 cells
Transepithelial electrical resistance (TER) value is an indispensable index for determining whether the epithelial barrier is damaged or not. Transwell assays were used to evaluate the permeability of IPEC-J2 cells. E. coli K88 infection severely disrupted TER in IPEC-J2 cells within 120 min; however, we found that FSP significantly ameliorated E. coli K88-induced decreases in TER in these cells ((A)). Moreover, pre-treatment with FSP effectively increased the expression of TJ proteins including ZO-1, occludin, and claudin-1 in E. coli K88-infected IPEC-J2 cells ((B)).
Figure 5. Effects of fermented soybean peptides on epithelial barrier function in E. coli K88-infected IPEC-J2 cells. (A) FSP effectively ameliorated E. coli K88-induced decreases in trans-epithelial resistance (TER) in IPEC-J2 cells. (B) FSP up-regulated the expression of tight junction (TJ) proteins ZO-1, occludin, and claudin-1 in E. coli K88-infected IPEC-J2 cells, as determined by western blotting. (C) Quantitative analysis of TJ proteins in E. coli K88-infected IPEC-J2 cells. Bars represent mean ± SEM (n = 3); *: p < 0.05 vs control group, *: p < 0.05, vs E. coli K88.
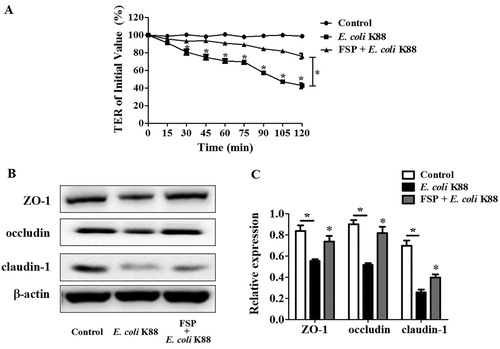
4. Discussion
Intestinal inflammation is a common phenomenon in young piglets during their growth. The experiments and analysis in our study showed that replacing 10% of SBM with FSBM significantly improved the intestinal health of piglets. The results indicated that FSBM significantly decreases IL-6, IL-1β, and D-lactate levels in piglet serum. One of the major reasons is that allergenic proteins like beta-conglycinin and glycinin subunits were degraded during fermentation (). Thus, FSBM would cause much less inflammation than SBM. Another important reason is the production of bioactive compounds like peptides, oligosaccharides and isoflavone with fermentation. The peptides from soybeans were found to be anti-inflammatory, by virtue of decreasing expression of inflammatory markers like TNF-α, IL-1β, and IL-6 in LPS treated RAW 264.7 macrophages (Min et al., Citation2017). The content of peptides in the soybean meal was increased by 7.78-fold, from 12.5 mg g−1–97.2 mg g−1 after fermentation (). The additional peptides derived from fermentation could inhibit the inflammation in intestine.
Previous studies observed that the decrease in TJ protein expression in weaned piglets was usually followed by damage to the physical barrier function (Hu et al., Citation2013). Fermented products like milk beverage, soy germ, and a barley-soybean mixture were reported to be protective of intestinal epithelial cells (Chen, Hsu, Hung, & Chen, Citation2016; Lara et al., Citation2012; Woo et al., Citation2016). Some food-derived bioactive peptides exert health-promoting actions like maintenance, reinforcement or repairment of the intestinal barrier function (Olga et al., Citation2014). Most researches on soybean peptides focused on their antioxidant, antimicrobial, anticancer, and anti-inflammatory effects (Sanjukta & Rai, Citation2016). Recently, bioactive peptides were suggested to promote intestinal epithelial cell proliferation and up-regulate TJ protein. Compare to SBM, FSBM contains more bioactive peptides. We believe that the peptides in fermented soybean meal might participate in intestinal epithelial barrier recovering.
Enteric bacterial pathogen invasion can affect the physiological functions of the intestinal epithelium, such as activation of the inflammatory cascade and alteration to the tight junction barrier functions (Berkes, Viswanathan, Savkovic, & Hecht, Citation2003; Clarke, Francella, Huegel, & Weiser, Citation2011). Intestinal pathogens are capable of inciting inflammation in the gastrointestinal mucosa (Berkes et al., Citation2003; Ruchaud-Sparagano, Maresca, & Kenny, Citation2010), with NF-κB and MAPK being two pivotal signalling pathways regulating this inflammation (Czerucka, Dahan, Mograbi, Rossi, & Rampal, Citation2001; Eckmann & Neish, Citation2011). Pathogen-associated molecular patterns such as LPS bind toll-like receptors to elicit a response. Enteric pathogens induce the inflammatory response through the activation of NF-κB, which is liberated from the inhibitory IκB protein (Andy, Bonnet, & Manolis, Citation2011; Yu Fu et al., Citation2011). NF-κB controls the expression of essential pro-inflammatory cytokines, chemokines, and immune receptors including IL-1β, TNF-α, IL-6, IL-8, iNOS, T cell receptor-α, and MHC class II molecules. Similarly, the p38 in MAPK pathway can be activated by MAPK kinase kinase (MAPKKK) and affects cytokine production (Czerucka et al., Citation2001; Thalhamer, McGrath, & Harnett, Citation2008). In this study, 50 μg mL−1 FSP effectively attenuated inflammation in E. coli K88-infected epithelial cells via NF-κB and p38 pathways and down-regulated the pro-inflammatory cytokines IL-6, IL-1β, IL-8, and TNF-α. These results suggested that FSP can dampen inflammation in E. coli K88-infected IPEC-J2 cells.
During an intestine infection, the enteropathogenic Escherichia coli (EPEC) trigger TJ barrier disruption (Guttman & Finlay, Citation2009; Roxas et al., Citation2010; Spitz et al., Citation1995), leading to a drop in TER and an increase in paracellular permeability in intestinal epithelial cells. Pretreating IPEC-J2 cells with 50 μg mL−1 FSP effectively protected the piglets against intestinal barrier dysfunction by increasing the expression of TJ proteins (ZO-1, occludin, and claudin-1) and elevating TER in E. coli K88-infected IPEC-J2 cells. In previous research, a fermented barley and soybean mixture could maintain the TJ protein expression in colitis mice (Woo et al., Citation2016) and it was suggested that isoflavones in fermented soy germ played a protective role on intestinal TJ barrier (Lara et al., Citation2012). Our study corroborated and enriched the findings from previous studies by demonstrating that FSP protect the epithelial barrier from E. coli K88-induced dysfunction by moderating TJ proteins.
5. Conclusion
This study reported the function of FSBM and its constitutive peptides in intestinal protection. FSP, after purification by ultrafiltration, exert strong immunomodulatory and epithelial barrier function promoting effects by inhibiting NF-κB and MAPK signalling, decreasing pro-inflammatory cytokine levels, increasing the expression of TJ proteins, and enhancing TER in epithelial cells. Therefore, in addition to the nutritive benefits, fermentation of SBM can suppress intestinal inflammation, an effect that is likely attributed to the presence of FSP. Our study provides theoretical support for the contention that small peptides from fermented SBM can improve intestinal health.
Supplemental Material
Download MS Excel (9.7 KB)Acknowledgement
This research was supported by the Modern Agro-Industry Technology Research System of China (CARS-35), Key Agriculture Program of Zhejiang Major Science and Technology Projects (2015C02022), and China and Zhejiang province Postdoctoral Science Foundation (518000-X91604 and 518000-X81601). We would like to thank Editage [www.editage.cn] for English language editing.
Disclosure statement
No potential conflict of interest was reported by the authors.
Additional information
Funding
References
- Andy, W., Bonnet, M. C., & Manolis, P. (2011). NF-κB in the regulation of epithelial homeostasis and inflammation. Cell Research, 21, 146–158. doi: 10.1038/cr.2010.175
- Berkes, J., Viswanathan, V. K., Savkovic, S. D., & Hecht, G. (2003). Intestinal epithelial responses to enteric pathogens: Effects on the tight junction barrier, ion transport, and inflammation. Gut, 52, 439–451. doi: 10.1136/gut.52.3.439
- Blanca, H. L., Chia-Chien, H., & De Lumen, B. O. (2009). Antioxidant and anti-inflammatory properties of cancer preventive peptide lunasin in RAW 264.7 macrophages. Biochemical and Biophysical Research Communications, 390, 803–808. doi: 10.1016/j.bbrc.2009.10.053
- Bruun, J. M., Helge, J. W., Richelsen, B., & Stallknecht, B. (2006). Diet and exercise reduce low-grade inflammation and macrophage infiltration in adipose tissue but not in skeletal muscle in severely obese subjects. American Journal of Physiology-Endocrinology and Metabolism, 290, E961–E967. doi: 10.1152/ajpendo.00506.2005
- Cavuoto, P., & Fenech, M. F. (2012). A review of methionine dependency and the role of methionine restriction in cancer growth control and life-span extension. Cancer Treatment Reviews, 38, 726–736. doi: 10.1016/j.ctrv.2012.01.004
- Chang, H. C., Lewis, D., Tung, C. Y., Han, L., Henriquez, S. M. P., Voiles, L., … Pollok, K. E. (2014). Soypeptide lunasin in cytokine immunotherapy for lymphoma. Cancer Immunology, Immunotherapy, 63, 283–295. doi:10.1016/j.ctrv.2012.01.004 doi: 10.1007/s00262-013-1513-8
- Chen, Y. P., Hsu, C. A., Hung, W. T., & Chen, M. J. (2016). Effects of Lactobacillus paracasei 01 fermented milk beverage on protection of intestinal epithelial cell in vitro. Journal of the Science of Food and Agriculture, 96, 2154–2160. doi: 10.1002/jsfa.7331
- Clarke, T., Francella, N., Huegel, A., & Weiser, J. J. (2011). Invasive bacterial pathogens exploit TLR-mediated downregulation of tight junction components to facilitate translocation across the epithelium. Cell Host & Microbe, 9, 404–414. doi:10.1016/j.chom.2011.04.012. doi: 10.1016/j.chom.2011.04.012
- Czerucka, D., Dahan, S., Mograbi, B., Rossi, B., & Rampal, P. (2001). Implication of mitogen-activated protein kinases in T84 cell responses to enteropathogenic Escherichia coli infection. Infection and Immunity, 69, 1298–1305. doi: 10.1128/IAI.69.3.1298-1305.2001
- Dia, V. P., Wang, W., Oh, V. L., Lumen, B. O. D., & Mejia, E. G. D. (2009). Isolation, purification and characterisation of lunasin from defatted soybean flour and in vitro evaluation of its anti-inflammatory activity. Food Chemistry, 114, 108–115. doi: 10.1016/j.foodchem.2008.09.023
- Dilshat, Y., Parida, H., Nurmuhammat, A., Kimono, U., & Nobuo, Y. (2012). Effects of soybean peptide on immune function, brain function, and neurochemistry in healthy volunteers. Nutrition, 28, 154–159. doi: 10.1016/j.nut.2011.05.008
- Dow, S. W., Roberts, A., Vyas, J., Rodgers, J., Rich, R. R., Orme, I., & Potter, T. A. (2000). Immunization with f-Met peptides induces immune reactivity against Mycobacterium tuberculosis. Tubercle and Lung Disease, 80, 5–13. doi: 10.1054/tuld.1999.0226
- Eckmann, L., & Neish, A. S. (2011). NF-κB and mucosal homeostasis. Current Topics in Microbiology Immunology, 349, 145–158. doi: 10.1007/82_2010_103
- Guttman, J. A., & Finlay, B. B. (2009). Tight junctions as targets of infectious agents. Biochimica et Biophysica Acta (BBA) - Biomembranes, 1788, 832–841. doi: 10.1016/j.bbamem.2008.10.028
- Hu, C. H., Xiao, K., Luan, Z. S., & Song, J. (2013). Early weaning increases intestinal permeability, alters expression of cytokine and tight junction proteins, and activates mitogen-activated protein kinases in pigs. Journal of Animal Science, 91, 1094–1101. doi: 10.2527/jas.2012-5796
- Jiao, L. F., Ke, Y. L., Xiao, K., Song, Z. H., Hu, C. H., & Shi, B. (2015). Effects of cello-oligosaccharide on intestinal microbiota and epithelial barrier function of weanling pigs. Journal of Animal Science, 93, 1157–1164. doi: 10.2527/jas.2014-8248
- Korhonen, H., & Pihlanto, A. (2003). Food-derived bioactive peptides–opportunities for designing future foods. Current Pharmaceutical Design, 9, 1297–1308. doi: 10.2174/1381612033454892
- Lara, M., Valérie, B., Christel, S. C., Valérie, B., Corinne, L., Mathilde, L., … Eric, H. (2012). A low dose of fermented soy germ alleviates gut barrier injury, hyperalgesia and faecal protease activity in a rat model of inflammatory bowel disease. Plos One, 7, e49547. doi: 10.1371/journal.pone.0049547
- Liu, H., Lv, Y., Xu, J., & Guo, S. (2016). Soybean peptide aggregates improved calcium binding capacity. LWT – Food Science Technology, 67, 174–180. doi: 10.1016/j.lwt.2015.11.046
- Magalhaes, J. G., Tattoli, I., & Girardin, S. E. (2007). The intestinal epithelial barrier: How to distinguish between the microbial flora and pathogens. Seminars in Immunology, 19, 106–115. doi: 10.1016/j.smim.2006.12.006
- Maria, R. (2011). The intestinal epithelial barrier in the control of homeostasis and immunity. Trends in Immunology, 32, 256–264. doi: 10.1016/j.it.2011.04.003
- Matthews, D. M. (1990). Protein absorption: Development and present state of the subject.
- Mejia, E. G. D., & Dia, V. P. (2009). Lunasin and lunasin-like peptides inhibit inflammation through suppression of NF-κB pathway in the macrophage. Peptides, 30, 2388–2398. doi: 10.1016/j.peptides.2009.08.005
- Min, Y. K., Lee, Y. J., Li, M., Baek, S. Y., Kang, T. S., & Jeong, H. S. (2017). Characteristics and in vitro anti-inflammatory activities of protein extracts from pre-germinated black soybean [Glycine max (L.)] treated with high hydrostatic pressure. Innovative Food Science Emerging Technologies, 43, S146685641730423X. doi: 10.1016/j.ifset.2017.07.027
- Moeser, A. J., Carin Vander, K., Ryan, K. A., Wooten, J. G., Dianne, L., Cook, V. L., & Blikslager, A. T. (2007). Stress signaling pathways activated by weaning mediate intestinal dysfunction in the pig. American Journal of Physiology-Gastrointestinal and Liver Physiology, 292, G173–G181. doi: 10.1152/ajpgi.00197.2006
- Müller, C. A., Autenrieth, I. B., & Peschel, A. (2005). Intestinal epithelial barrier and mucosal immunity. Cellular and Molecular Life Sciences, 62, 1297–1307. doi: 10.1007/s00018-005-5034-2
- National Research Council. (2012). Nutrient requirements of swine (11th rev ed.). Washington, DC: The National Academies Press.
- Olga, M. A., Belén, R. G., Cristina, M., & Fermín, S. D. M. (2014). Food derived bioactive peptides and intestinal barrier function. International Journal of Molecular Sciences, 15, 22857–22873. doi: 10.3390/ijms151222857
- Rayaprolu, S. J., Hettiarachchy, N. S., Chen, P., Kannan, A., & Mauromostakos, A. (2013). Peptides derived from high oleic acid soybean meals inhibit colon, liver and lung cancer cell growth. Food Research International, 50, 282–288. doi: 10.1016/j.foodres.2012.10.021
- Roh, S. G., Carroll, J. A., & Kim, S. W. (2015). Effects of fermented soybean meal on innate immunity-related gene expressions in nursery pigs acutely challenged with lipopolysaccharides. Animal Science Journal, 86, 508–516. doi: 10.1111/asj.12319
- Rokana, N., Singh, R., Mallappa, R. H., Batish, V. K., & Grover, S. (2016). Modulation of intestinal barrier function to ameliorate Salmonella infection in mice by oral administration of fermented milks produced with Lactobacillus plantarum MTCC 5690 – a probiotic strain of Indian gut origin. Journal of Medical Microbiology, 65, 1482–1493. doi: 10.1099/jmm.0.000366
- Roxas, J. L., Athanasia, K., Amy, B., Samuel, T., Sandhya, R., Kanakeshwari, F., … Gail, H. (2010). Enterohemorrhagic E. coli alters murine intestinal epithelial tight junction protein expression and barrier function in a Shiga toxin independent manner. Laboratory Investigation, 90, 1152–1168. doi: 10.1038/labinvest.2010.91
- Ruchaud-Sparagano, M. H., Maresca, M., & Kenny, B. (2010). Enteropathogenic Escherichia coli (EPEC) inactivate innate immune responses prior to compromising epithelial barrier function. Cellular Microbiology, 9, 1909–1921. doi: 10.1111/j.1462-5822.2007.00923.x
- Sanjukta, S., & Rai, A. K. (2016). Production of bioactive peptides during soybean fermentation and their potential health benefits. Trends in Food Science & Technology, 50, 1–10. doi: 10.1016/j.tifs.2016.01.010
- Spitz, J., Yuhan, R., Koutsouris, A., Blatt, C., Alverdy, J., & Hecht, G. (1995). Enteropathogenic Escherichia coli adherence to intestinal epithelial monolayers diminishes barrier function. American Journal of Physiology, 268, 374–379. doi: 10.1152/ajpgi.1995.268.2.G374
- Thalhamer, T., McGrath, M. A., & Harnett, M. M. (2008). MAPKs and their relevance to arthritis and inflammation. Rheumatology, 47, 409–414. doi: 10.1093/rheumatology/kem297
- Tian, J., Jiao, X., Wang, X., Geng, J., Wang, R., Liu, N., … Shan, F. (2017). Novel effect of methionine enkephalin against influenza A virus infection through inhibiting TLR7-MyD88-TRAF6-NF-κB p65 signaling pathway. International Immunopharmacology, 55, 38–48. doi: 10.1016/j.intimp.2017.12.001
- Wang, H., Zhang, S., Sun, Y., & Dai, Y. (2013). ACE-inhibitory peptide isolated from fermented soybean meal as functional food. International Journal of Food Engineering, 9, 1–8. doi: 10.1515/ijfe-2012-0207
- Wittkopf, N., Neurath, M. F., & Becker, C. (2014). Immune-epithelial crosstalk at the intestinal surface. Journal of Gastroenterology, 49, 375–387. doi: 10.1007/s00535-013-0929-4
- Woo, J. K., Choi, S., Kang, J. H., Kim, D. E., Hurh, B. S., Jeon, J. E., … Oh, S. H. (2016). Fermented barley and soybean (BS) mixture enhances intestinal barrier function in dextran sulfate sodium (DSS)-induced colitis mouse model. BMC Complementary and Alternative Medicine, 16, 498. doi: 10.1186/s12906-016-1479-0
- Wright, H. L., Moots, R. J., Bucknall, R. C., & Edwards, S. W. (2010). Neutrophil function in inflammation and inflammatory diseases. Rheumatology, 49, 1618–1631. doi: 10.1093/rheumatology/keq045
- Yi, H., Jiang, D., Zhang, L., Xiong, H., Han, F., & Wang, Y. (2016). Developmental expression of STATs, nuclear factor-κB and inflammatory genes in the jejunum of piglets during weaning. International Immunopharmacology, 36, 199–204. doi: 10.1016/j.intimp.2016.04.032
- Yu Fu, W., Xiang, X., Xia, F., Chun, Z., Qiang, W., Xi, W., … Jing-Long, Y. (2011). A cell-penetrating peptide suppresses inflammation by inhibiting NF-κB signaling. Molecular Therapy the Journal of the American Society of Gene Therapy, 19, 1849–1857. doi: 10.1038/mt.2011.82
- Zhang, Y., Shi, C., Wang, C., Lu, Z., Wang, F., Feng, J., & Wang, Y. (2017). Effect of soybean meal fermented with Bacillus subtilis BS12 on growth performance and small intestinal immune status of piglets. Food and Agricultural Immunology, 1–14. doi: 10.1080/09540105.2017.1360258
- Zhang, J., Zhu, J., Rahman, M. R. T., Jin, S., & Rong, J. (2016). The sterilized fermented feed improves intestinal barrier function and immune function in chicken. Faseb Journal, 30, Supplement lb247.
- Zhu, J., Gao, M., Zhang, R., Sun, Z., Wang, C., Yang, F., … Hao, Z. (2017). Effects of soybean meal fermented by L. plantarum, B. subtilis and S. cerevisieae on growth, immune function and intestinal morphology in weaned piglets. Microbial Cell Factories, 16(1), 191. doi: 10.1186/s12934-017-0809-3