ABSTRACT
Hyperuricemia is a metabolic disease caused by excessive production of uric acid (UA), which can cause gout, hypertension, hyperlipidemia and other diseases. This study aimed to explore the effect of genistein on hyperuricemia and renal protection in hyperuricemic mice induced by potassium oxonate. Furthermore, genistein significantly downregulated the xanthine oxidase (XOD), adenosine deaminase levels, renal gene expressions of glucose transporter type 9 (mGLUT9) and uric acid transporter 1 (mURAT1) and upregulated organic anion transporters (mOAT1 and mOAT3) and organic cation transporters (mOCT1 and mOCT2). Additionally, genistein improved renal function, renal histopathological features and antioxidant activities in hyperuricemic mice. Genistein also attenuated renal fibrosis by suppressing the JAK2/STAT3 and Wnt/β-catenin signalling pathways in hyperuricemic mice. Overall, this study suggested that both doses of genistein have strong potential against hyperuricemia and associated disorders and may be used as natural supplements for the treatment of UA-related disorders.
1. Introduction
Hyperuricemia is a disease caused by excessive secretion or reduced excretion of uric acid (UA), which can lead to gout, hypertension, hyperlipidemia and other diseases (Guo et al., Citation2020). Abnormal high-serum UA levels can cause the accumulation of UA and acute inflammatory reaction in the kidneys and joints, leading to joint deformity, UA nephropathy (Pan et al., Citation2019) and acute gouty arthritis (Robinson, Citation2018). Reduced excretion of UA is the most dominant cause of hyperuricemia, with approximately 90% of patients presenting with these symptoms (Chen et al., Citation2019). Changes in lifestyle such as consumption of a high-purine diet are also leading to hyperuricemia (Wang et al., Citation2010). In China, the occurrence of hyperuricemia has reached 13.3% (men 19.4% and women 7.9%), and worldwide, the incidence of hyperuricemia ranges from 2.6% to 36% in different populations (Liu et al., Citation2015).
Metabolic cleavage of purine nucleotides results in the production of UA. Xanthine oxidase (XOD) and adenosine deaminase (ADA) catalyzes the oxidation of xanthine or hypoxanthine to UA. The product UA is excreted mainly through the kidneys and intestines. The kidney plays an important role in UA excretion. Excretion of UA in the kidney includes secretion and reabsorption of proximal tubular cells and uric acid transport systems such as glomerular filtration. There are many urate transporters that play vital roles in UA reabsorption and excretion, such as GLUT9, URAT1, ABCG2, OAT1, OAT2, OCT1 and OCT2 (Dinour et al., Citation2010; Ichida et al., Citation2004; Zeng et al., Citation2012).
Excessive UA levels result in the over production of reactive oxygen species (ROS), which further promotes the activation of inflammasomes (Cui et al., Citation2020). Inflammasomes and cytokines such as cysteine-containing aspartate-specific protease-1 (caspase-1), inflammatory cytokine interleukin 1β (IL-1β), TNF-alpha and inflammasomes including NOD-like receptor family pyrin domain containing 3 (NLRP3) are released extracellular, accelerating the process of kidney disease (Tan et al., Citation2019). Furthermore, higher UA is also the main cause of hyperuricemia nephropathy (Li et al., Citation2021).
Nowadays, hyperuricemia and other UA-related disorders are being treated with hypouricemic drugs, including renal urate reabsorption and XOD inhibitors. However, long-term use of these drugs, including benzbromarone, allopurinol and febuxostat, has adverse effects, such as severe hepatotoxicity (Li et al., Citation2021), gastrointestinal and renal toxicity and severe hypersensitivity (Zhou et al., Citation2017). Therefore, there is a need to develop effective, safe and improved therapies to treat UA-related disorders, and natural products could lead to an innovative source of such treatments. Presently, natural products are used to treat and prevent hyperuricemia due to their advantages of high compliance, remarkable efficacy and few adverse reactions (Guo et al., Citation2020; Chen et al., Citation2019; Cui et al., Citation2020; Mehmood et al., Citation2020a, Citation2020b, Citation2020c, Citation2020d, Citation2020e, Citation2019a, Citation2019b; Ishaq et al., Citation2020; Chen et al., Citation2013; Müller et al., Citation2019; Pan et al., Citation2021).
Genistein is an isoflavone compound naturally found in soybeans and soybean products. Previously, it was documented that genistein can be used for the prevention of various metabolic diseases linked with cardiovascular disease (CVD), diabetes, cancer and obesity (Pavese et al., Citation2010; Rehman et al., Citation2019). Moreover, it may also protect against acute kidney injury (AKI)/chronic kidney injury (CKI), such as cisplatin, unilateral ureteral obstruction (UUO)-induced renal fibrosis, radiation, ischemia/reperfusion (I/R)-induced renal injury, morphine and diabetic nephrotoxicity (Stephenson et al., Citation2005; Sung et al., Citation2008; Canyilmaz et al., Citation2016; Ning et al., Citation2020; Jalili et al., Citation2020; Javani et al., Citation2019; Jia et al., Citation2019).
In this study, we analysed the antihyperuricemic activities of genistein in an animal model of hyperuricemia induced by potassium oxonate (PO), and underlying mechanism was further explored.
2. Materials and methods
2.1. Chemicals
Genistein, allopurinol, benzbromarone, PO and carboxymethyl cellulose (CMC) were purchased from Sigma-Aldrich Reagent (Shanghai, China). The total protein (A045-2-2), ADA (A048-2-1), XOD (A002-1-1), UA (C02-2-1), creatinine (C011-2-1), blood urea nitrogen (BUN), malondialdehyde (MDA) (A003-1-2), superoxide dismutase (SOD) (A001-3-2), catalase (CAT) (A007-1-1), glutathione (GSH) (A006-2-1), total antioxidant capacity (T-AOC) (A015-2-1), tumor necrosis factor alpha (TNF-α) (H052-1), interleukin-1β (IL-1β) (H002), monocyte chemoattractant protein-1 (MCP-1) (H115), Caspase-1 (H074), prostaglandin E2 (PGE2) (H099-1) and interleukin-6 (IL-6) (H007-1-1) were purchased from Nanjing Jiancheng Bioengineering Institute (Nanjing, China). All other chemicals used in this study were of analytical grade.
2.2. Animals
Animal experiments were conducted in accordance with the Institutional Guidelines for the Care of Laboratory Animals of the Chinese Academy of Medical Science, and approved by the Institutional Ethics Committee for Research on Laboratory Animal Use. 50 male Kunming mice (SPF) were procured from Beijing Vital River Laboratory Animal Technology Co., Ltd. (approval number SCXK (BJ) 2012-0001). The animals were housed in a control room maintaining the room relative humidity 60%, temperature at 25 ± 5°C on 12-h light/dark cycle along with free access to water and food for 1 week.
2.3. Induction of hyperuricemia
After one week of environmental adjustment, mice were divided into five groups named as G1: normal control group, G2: model group, G3: positive group, G4: low dose genistein group and G5: high dose genistein group (detail presented in ). The hyperuricemia model was established by the oral gavage of PO (0.25% CMC solution) at the dosage of 250 mg/kg bw for 15 days (Ishaq et al., Citation2020). CMC solution (0.25%) was also given to mice in the normal control group for 15 days. The allopurinol was used as a positive control and 10 mg/kg bw was administrated to mice. G4 and G5 groups received genistein at the dosage of 10 and 20 mg/kg bw for 15 days (suspension was made in 0.25% CMC solution).
Table 1. Animal study plan.
2.4 . Collection of urine, blood and tissues
On the last day, mice were transferred to metabolic cages for urine collection. The collected urine samples were centrifuged (2000g for 10 min) and stored at −80°C for further analysis. The blood samples from each mouse were collected after the administration of the final dosage via cardiopuncture. The serum was obtained after centrifugation at 10,000g for 5 min and stored at −80°C. The tissues such as kidneys, liver and heart were quickly and carefully dissected and rinsed with ice-cold PBS and some parts were fixed for H&E staining and others stored at −80°C for various biochemical and PCR assay.
2.5. Determination of serum and urine UA levels and renal function tests
The UA levels in the serum and urine samples were measured using the Nanjing Institute of Bioengineering Co. Ltd (Nanjing, China) kit. The renal function tests such as serum Cr and serum BUN levels were also determined using Nanjing Institute of Bioengineering Co. Ltd assays kits and kits’ catalogue numbers are presented in section 2.1.
2.6. Measurement of XOD and ADA activity
The XOD and ADA activities in the serum and liver tissues were also determined. The liver tissue (0.1 g) added into ice-cold PBS was prepared by centrifuging at 8000g at 4°C for 10 min and the supernatant was used for the analysis of XOD and ADA activities as per the manufacturers’ instruction provided by the Nanjing Institute of Bioengineering Co. Ltd (Nanjing, China).
2.7. Determination of oxidative stress indicators
The Oxidative stress (OS) indicators such as malondialdehyde (MDA) (A003-1-2), SOD (A001-3-2), catalase (CAT) (A007-1-1), glutathione (GSH) (A006-2-1) and T-AOC (A015-2-1) levels in the serum and renal tissues (homogenize in PBS) were determined using kits. The kits were purchased from the Nanjing Jiancheng Bioengineering Institute, and assays were performed according to the method provided by the manufacturer.
2.8. Determination of inflammatory indicators
The inflammatory indicators such as TNF-α, IL-6, MCP-1, Caspase-1 and PGE2 were determined in serum and renal tissues using the enzyme-linked immunosorbent assay (ELISA) kits as per the instructions provided by the manufacturer.
2.9. Histopathological examination
The fixed renal tissues (paraformaldehyde, 4%) were embedded in paraffin, cut, sectioned (5 μm) and stained with hematoxylin and eosin (H&E). Later, images were obtained using microscope BX53 (Olympus, Tokyo, Japan).
2.10. Effects of genistein on mRNA expressions
The renal urate transporters such as UART1, GLUT9, OAT1, OAT3, OCT1, OCT2, and inflammatory indicators NF-κβ, JAK2/STAT3 and Wnt/β-Catenin downstream genes expressions were determined using RT- qPCR analysis. In detail, total RNA from renal cortex samples (1 mg) were isolated using Takara, Japan. After that, 500 mg of RNA was reverse transcribed into cDNA using PrimeScript™ RT reagent kit (TaKaRa, Japan) and subjected to PCR amplification using SYBR Premix Plus and specific primers (Table S1). RT-PCR was performed in a CFX96 Real-Time PCR Detection system (Bio-Rad, USA).
2.11. In silico study
2.11.1. Homology modeling and evaluation of URAT1
The amino acid sequence of URAT1 was obtained from the UNIPROTKB database with accession numbers Q96S37. Then, the 3D structure of URAT1 was built using SWISS-MODEL server (https://swissmodel.expasy.org/). Finally, the quality of 3D structures of URAT1 was evaluated using ERRAT and PROCHECK method, and the results are shown in Figure S1. The ERRAT value was 96.084, and the PROCHECK results showed 93.3% of the amino acid residues in favored regions, 6.1% in allowed regions, 0.6% in generous regions and 0.0% in disallowed regions, indicating the reliability of URAT1 model prediction. Moreover, the quality of URAT1 model established in this study was better than that of the study by Yong et al. (Citation2016).
2.11.2. Molecular docking study of URAT1 and genistein
The 3D structure of genistein was obtained from PubChem (https://pubchem.ncbi.nlm.nih.gov/) with CID numbers 5280961, and structure optimization was performed using the steepest descent method with MMFF94 in the Avogadro program (Hanwell et al., Citation2012). AutoDock Vina package was used for genistein and URAT1 docking (Trott and Olson). AutoDock Tools 1.5.6 software was performed to edit the 3D structure of genistein and URAT1, Gasteiger charges were added. The active pocket positions of URAT1 were set as x = −11.6, y = −13.3, z = 8.0, the grid box was 40 × 40 × 40. After docking, the optimal model was selected according to the binding free energy and binding mode for interaction analysis. Open-Source PyMOL and Discovery Studio 2016 Client were used to visualize the analysis.
2.12. Statistical analysis
The obtained data were analysed using one-way ANOVA followed by multiple comparisons test using GraphPad Prism software. The significance was set at a P < .05, and the results are expressed as mean ± standard error.
3. Results
3.1. Genistein attenuated hyperuricemia via inhibiting key enzymes, reducing UA level and improving renal function
As depicted in (E), PO administration significantly elevated the serum UA levels and decreased urine UA levels in the model group (G2) compared to the normal control group (G1), which indicated that hyperuricemic animal model was successfully developed. Allopurinol (G3) and genistein (G4 and G5) treatment markedly (p < .05) decreased the serum UA and increased urine uric acid (UUA) in hyperuricemia mice compared to model control group (G2). Both genistein doses (10 and 20 mg/kg bw) were effective in reducing the UA level. As presented in (A–E), serum and hepatic XOD (p < .05) and ADA (p < .05) activities were significantly (p < .05) elevated in model group (G2) compared to the normal control group (G1), which indicated the increase in UA level. Allopurinol (G3) and genistein (G4 and G5) at the dosage of 10 and 20 mg/kg simultaneously inhibited XOD (p < .05) and ADA (p < .05) enzymes compared to the PO group. Serum BUN and Cr ((A,B)) levels in the model group were significantly (p < .05) elevated compared to the normal control group. Higher UA level could result in the damage of renal tissues and inflammation. The genistein treatments significantly (p < .05) restored the serum BUN and Cr levels, suggesting that genistein alleviated UA-mediated renal damage.
Figure 1. Effect of genistein on enzymatic activities and UA levels in mice, (A) SXOD, (B) LXOD, (C) SADA, (D) LADA, (E) SUA, (F) UUA, (G) UA synthesis pathway and effect of genistein on targeted enzyme SXOD: Serum xanthine oxidase, LXOD: liver xanthine oxidase, SADA: Serum adenosine deaminase, LADA: Liver adenosine deaminase, SUA: Serum uric acid, UUA: Urine uric acid,#G1: normal group, G2: Model control group, G3: positive control group, G4: low dose genistein treated group, G5: High dose genistein treated group, *p < .05, **p < .01, ***p < .001 compare with G1, #p < .05, ##p < .01, ###p < .001 compare with G2.
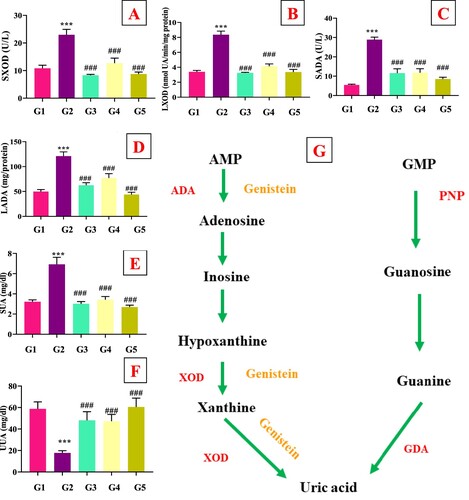
Figure 2. Effect of genistein on renal function tests (A) Serum urea nitrogen, and (B) Serum creatinine,#G1: normal group, G2: Model control group, G3: positive control group, G4: low dose genistein treated group, G5: High dose genistein treated group, *p < .05, **p < .01, ***p < .001 compare with G1, #p < .05, ##p < .01, ###p < .001 compare with G2.
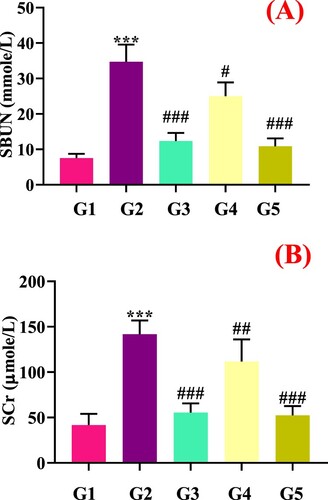
3.2. Genistein restore UA-mediated urate transporters in PO-induced hyperuricemic mice
The docking analysis results of genistein and URAT1 are shown in . As seen in (A–F), genistein binded with the surrounding amino acids (Arg, Phe, Pro and Ala) of URAT1 via hydrogen bond and pi−pi interaction. In addition, in the animal experiment, PO supplementation increased the URAT1 gene expression in model group compared to the normal control group ((A)). The genistein (G4 and G5) markedly (p < .05) downregulated the renal gene expression of mURAT1 with comparison to the model group (G2). Similarly, gene expression of mGLUT9 was also upregulated by PO in model group (G2) compared with the normal group, which was further restored by allopurinol (G3) and genistein (G3 and G4) treatments ((B)).
Figure 3. Molecular docking results of genistein and URAT1. (A) Conformation of complexes URAT1-genistein. (B) Profile map of complexes URAT1-genistein. (C) Genistein could bind to the hydrophobic region of URAT1. (D) Vertical view of complexes URAT1-genistein. (E) and (F) represent 3D and 2D interactions between genistein and URAT1, respectively. Open-Source PyMOL and Discovery Studio 2016 Client software were used to create the image.
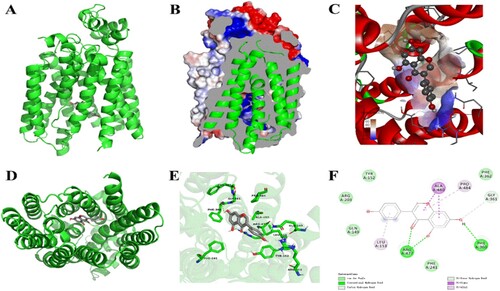
Figure 4. Effect of genistein on renal genes expression of urate transporters and underlying mechanism. (A) URAT1, (B) GLUT9, (C) OCT1, (D) OAT1, (E) OAT3, (F) OCT2, (G) potential mechanism of uric acid lowering via genistein,#G1: normal group, G2: Model control group, G3: positive control group, G4: low dose genistein treated group, G5: High dose genistein treated group, *p < .05, **p < .01, ***p < .001 compare with G1, #p < .05, ##p < .01, ###p < .001 compare with G2.
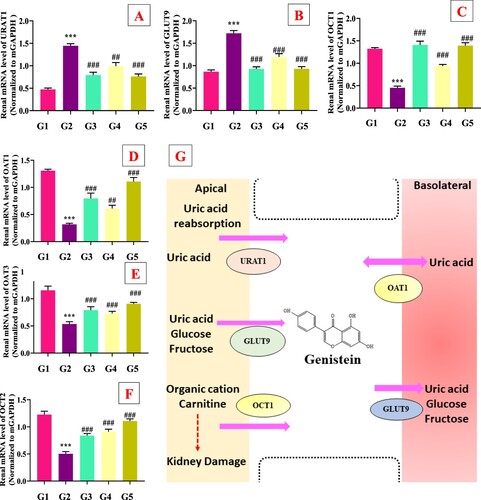
The effects of genistein on organic anion and cation transporters are presented in (C–F). The PO administration significantly downregulated the gene expression of mOAT1, mOAT3, mOCT1 and mOCT2 compared to G1. The allopurinol (G3) and genistein (G3 and G4) treatments markedly (p < .05) upregulated these organic anions and cations transporters compared to the G2 group. These results suggested that genistein has dual function like inhibiting key enzymes and aiding UA secretion via interacting with urate transporters () and )).
3.3. Genistein restored UA-mediated OS in PO-induced hyperuricemic mice
The results of serum and renal OS indicators, such as MDA, SOD, T-AOC, CAT and GSH levels are shown in . Our results showed that compared with the NC group, SOD, T-AOC, CAT, GSH and other OS indexes in serum and kidney tissues of G2 group were downregulated, while MDA content was upregulated (). Interestingly, allopurinol (G3) and genistein (G3 and G4) markedly (p < .05) restored antioxidant status in hyperuricemic mice via increasing SOD, T-AOC, CAT, GSH levels and decreasing MDA content in serum and renal tissues (). These results confirmed that genistein has a strong potential to improve antioxidant activities in hyperuricemic mice.
Table 2. Effect of genistein on serum and renal antioxidant activities in PO-induced hyperuricemic mice (n = 10, mean ± SD).
3.4. Genistein protect renal fibrosis via inhibiting Wnt/β-catenin signalling pathway in hyperuricemic mice
To further confirm the protective effect of genistein on hyperuricemia-associated renal fibrosis, we determined the expression of downstream renal genes involved in Wnt/β-catenin signalling pathway. We noticed that compared with the normal control group (G1), PO significantly downregulated the mAxin (p < .05), mGSK (p < .05) and mAPC (p < .05), and upregulated mβ-catenin (p < .05), mWnt3 (p < .05), mWnt4 (p < .05), mLRP5 (p < .05), mLRP6 (p < .05) and mFirizzled4 (p < .05) gene expression. The allopurinol (G3) and genistein (G4 and G5) administration restored these gene expressions compared with the G2 group ((A–I)).
3.5. Genistein protected renal fibrosis via inhibiting JAK2/STAT3 signalling pathway in hyperuricemic mice
As shown in (A–C), compared with the normal group, PO (G1) markedly elevated the gene expressions of mJAK2 (p < .05), mSTAT3 (p < .05) and mSOCS3 (p < .05). The genistein treated groups (G4 and G5) and allopurinol (G3) treatments significantly (p < .05) downregulated the renal mJAK2 (p < .05), mSTAT3 (p < .05) and mSOCS3 (p < .05) gene expression compared with the model group (PO).
3.6. Genistein protected renal morphological and inflammation in hyperuricemic mice
As presented in , results of H&E staining clearly showed severe tubular injury, swelling, atrophy and dilation in the renal tissues of the G2 group with high UA. Furthermore, glomerular cells proliferated, and inflammatory cells infiltrated into the interstitium in the hyperuric acid model group. The genistein treatment (G3 and G4) markedly (p < .05) restored the UA-mediated renal injury compared to the model group. The G2 also showed protection in a similar way. These morphological features of the kidney also provided supporting factors for biochemical analysis.
Figure 5. Effect of genistein on renal#histopathology features (magnification at 300×). G1: normal group, G2: Model control group, G3: positive control group, G4: low dose genistein treated group, G5: High dose genistein treated group.
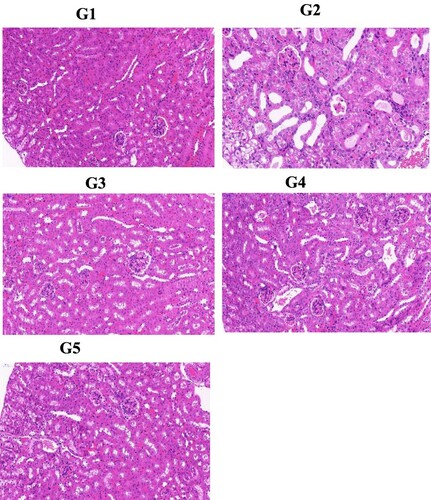
The protective effect of genistein on hyperuricemia-associated renal inflammation was also investigated by analyzing the levels of inflammatory cytokines such as (TNF-α, PGE2, IL-1β, COX-2, MCP-1 and IL-6) in serum and renal tissues. As shown in (A–G), PO significantly (p < .05) elevated the serum (TNF-α, IL-1β, and IL-6) and renal (PGE2, COX-2, and MCP-1) levels in model group (G2) compared with the normal control group (G1). The supplementation of genistein (G4 and G5) and allopurinol (G3) significantly (p < .05) decreased the serum and renal TNF-α, PGE2, IL-1β, COX-2 and IL-6 levels compared with the model group G2. Furthermore, renal gene expression of mNF-κβ was also upregulated in the G2 group compared with the G1 group, which was restored by the allopurinol (G3) and genistein (G3 and G4) treatments ().
4. Discussion
The overproduction of UA results to hyperuricemia, and per long time persist might trigger renal inflammation and fibrosis which positively contribute to hyperuricemia nephropathy (Li et al., Citation2021). That is why a new safe and effective UA-lowering strategy is urgently needed to cope hyperuricemia and associated renal nephropathy. Hyperuricemia in the animal can be induced by several ways such as administration of high fructose, yeast extract, potassium oxonate (PO) and adenine. Among all, the model of hyperuricemia induced by PO is most commonly used. PO selectively inhibits uricase of UA metabolism, which causes hyperuricemia in rodents and blocks the activity of hepatic uricase (Tang et al., Citation2017). Effectiveness of therapeutic agents and pathophysiological mechanism of hyperuricemia can be assessed using PO-induced hyperuricemia disease model in animals (Lee et al., Citation2018). In this study, we induced hyperuricemia by oral gavage of PO (0.25% CMC solution) at the dosage of 250 mg/kg bw for 15 days. The PO administration significantly alter various biochemical parameters such UA, XOD, ADA, BUN and SCr levels.
XOD is an important enzyme responsible for UA production via catalyzing xanthine to hypoxanthine to UA (Fukunari et al., Citation2004). Furthermore, ADA is also an important enzyme involved in the UA synthesis. ADA is a catalytic enzyme responsible for the production of hypoxanthine nucleoside from adenosine (Pang et al., Citation2017). The PO administration increases the serum and hepatic XOD and ADA levels in hyperuricemic mice, which were further restored by genistein treatment (). Many authors reported that polyphenolic compounds potently inhibited XOD and ADA both in vitro and in vivo experiments. Authors proposed that polyphenolic compounds bind closely to enzymes (XOD and ADA) via hydrogen bonds or pi−pi interactions with the surrounding amino acid, thus occupying the active site of enzymes and slowing down the catalysis of UA ((G)) (Guo et al., Citation2020; Chen et al., Citation2019; Cui et al., Citation2020; Mehmood et al., Citation2020a, Citation2020b, Citation2020c, Citation2020d, Citation2020e, Citation2019a, Citation2019b; Ishaq et al., Citation2020; Chen et al., Citation2013; Müller et al., Citation2019; Pan et al., Citation2021; Li et al., Citation2021).
The BUN and creatinine (Cr) are the key indicators of renal function. The higher these values, the greater the kidney damage. The PO administration (G2) significantly increases the serum BUN and creatinine levels which were further restored by genistein (G4 and G5) (). Mehmood et al., Citation2020c also observed that the polyphenolic compounds enrich stevia residue extract downregulated serum Cr and BUN in hyperuricemic mice. Ishaq et al. (Citation2020) also reported that sinapic acid could improve renal function via decreasing the serum Cr and BUN in PO-induced hyperuricemic mice. Recently, sunflower head, apigenin, and other bioactive compounds have also been reported to improve renal function in hyperuricemic mice (Tan et al., Citation2019; Mehmood et al., Citation2018, Citation2020b, Citation2020c, Citation2020e, Citation2020d; Tang et al., Citation2017; Lee et al., Citation2018; Pang et al., Citation2017).
It has been reported that approximately 90% of hyperuricemia patients cannot adequately excrete UA (Ichida Citation2009). More than 70−75% UA excreted via the renal pathway, whereas 20−25% UA excreted by intestinal tract. Notably, UA is a polar molecule and cannot easily pass through the membrane. Thus, it depended on ion channels to finish secretion and reabsorption in the renal proximal convoluted tubules. Therefore, renal tubular secretion and reabsorption processes are the key mechanisms of UA excretion. There are many urate transporters (URAT1, GLUT9, OCT1, and OCT2, OAT1, and OAT3) involved in excreting UA levels (Mandal & Mount, Citation2015). URAT1 is mainly involved in the reabsorption of UA in the renal proximal convoluted tubules and is encoded by the SLC22A12 gene. It is a key target of reducing UA drugs such as benzbromarone, losartan and fenofibrate (Ichida et al., Citation2004). GLUT9 (encoded by the SLC2A9 gene) is also responsible for the reabsorption of UA (Nigam et al., Citation2007). Organic anions transporters (OAT1 and OAT3) and organic cations transporters (OCT1 and OCT2) were involved in the renal secretion of urate. Reduced urate secretion was observed in OAT1-knockout mice, which confirmed the importance of organic anions transporters in the pathogenesis of hyperuricemia (Nigam et al., Citation2007). The results of our study concluded that genistein (G4 and G5) treatment markedly rebalance urate transporters (URAT1, GLUT9, OCT1, OCT2, OAT1 and OAT3) ( and ). Natural products and isolated compounds have been reported to regulate urate transporters such as sunflower head, stevia residue extract, grapefruit juice, Cordyceps militaris, Camellia japonica bee pollen, curcumin, phloretin and mangiferin (Wang et al., Citation2010; Chen et al., Citation2019; Xu et al., Citation2021; Pan et al., Citation2019; Yang et al., Citation2018; Mehmood et al., Citation2018, Citation2020b, Citation2020c, Citation2020d; Cui et al., Citation2020).
OS is defined as the higher production of ROS. The oxidant producing enzyme (XO) is released from the liver and linked to glycosaminoglycans and endothelial cells. . During the production of UA, XO generates ROS (oxygen radicals or hydrogen peroxide) around endothelial cells, further leading to the production of OH or ONOO− (Aslan et al., Citation2001; Osarogiagbon et al., Citation2000; Schmidt et al., Citation2018). It is well-documented that the OS of asymptomatic young patients with primary hyperuricemia is significantly higher than that of healthy subjects (Zhou et al., Citation2018). Moreover, it also reported that people with hyperuricemia and gout are at higher risk of developing cardiovascular diseases (Hayyan et al., Citation2016; Cristóbal-García et al., Citation2015). Abnormal UA levels lead to OS, decrease endogenous antioxidant defenses, increase ROS production, lipid peroxidation and initiate a cascade of reactions. MDA, SOD, CAT, T-AOC and GSH play an important role in UA-mediated OS (Lan et al., Citation2012). Therefore, this study investigated the protective effect of genistein on UA-mediated OS in hyperuricemia mice. We observed that genistein restored UA-mediated OS via modulating serum and renal MDA, SOD, CAT, GSH and T-AOC levels (). Genistein was reported to possess strong antioxidant activities. For example, genistein can markedly decrease the renal MDA content in diabetic mice (Kim & Lim, Citation2013). Similar results have been reported by many authors (Kim & Lim, Citation2013; Mehmood et al., Citation2020b, Citation2020c, Citation2020d). In an hyperuricemic model study, Mehmood et al. (Citation2020c, Citation2020d) documented that polyphenolic compounds-rich stevia residue extract ameliorate UA-mediated OS via restoring the activity of MDA, SOD, T-AOC, CAT, GSH-Px and NO levels in serum and renal tissues. In another study, the same research group observed that grapefruit juice markedly improved renal antioxidant activity (CAT, MDA, GSH, and SOD) in PO-induced hyperuricemic mice (Mehmood et al., Citation2020b). Many other bioactive compounds such as curcumin, phloretin and mangiferin were also reported to restore UA-mediated OS in animal experiments (Chen et al., Citation2019; Cui et al., Citation2020;Yang et al., Citation2018).
Wnt/β-catenin is an evolutionary conserved signalling pathway that controls embryogenesis, injury repair and pathogenesis of different diseases. Wnt/β-catenin is activated mainly in response to renal injury. Frequent activation of Wnt/β-catenin leads to the development of AKI to chronic kidney disease (CKD) (Xiao et al., Citation2016; Nusse & Clevers, Citation2017; Zuo & Liu, Citation2018). The genistein administration restored these gene expressions of Wnt/β-catenin signalling pathway compared with the G2 group (). Our results were closely related to the previous authors’ findings. Many authors have reported that natural products/compounds ameliorate renal fibrosis via suppressing downstream genes involved in the Wnt/β-catenin signalling pathway (Li et al., Citation2021; Wang et al., Citation2018a; Wang, et al., Citation2018b). In detail, Zhang and Chen (Citation2011) reported that genistein inhibited the human colon cancer cell line via Wnt signaling pathway. In another study, poricoic acid ZG and poricoic acid ZH were reported to attenuate renal fibrosis via inhibiting the activation of Wnt/β-Catenin signalling pathway (Wang et al., Citation2018a; Wang, et al., Citation2018b). Mehmood et al. (Citation2020d) reported that polyphenolic-enrich stevia residue extract attenuates renal fibrosis via inhibiting the activation of Wnt/β-Catenin signalling pathway in mice. In addition, recently Li et al. (Citation2021) recently documented that apigenin alleviated renal fibrosis in hyperuricemic mice via the Wnt/β-catenin pathway.
Figure 6. Genistein attenuates renal fibrosis inhibits the Wnt/β-catenin and its downstream target genes expression in PO-induced hyperuricemic mice., G1: normal group, G2: Model control group, G3: positive control group, G4: low dose genistein treated group, G5: High dose genistein treated group, *p < .05, **p < .01, ***p < .001 compare with G1, #p < .05, ##p < .01, ###p < .001 compare with G2.
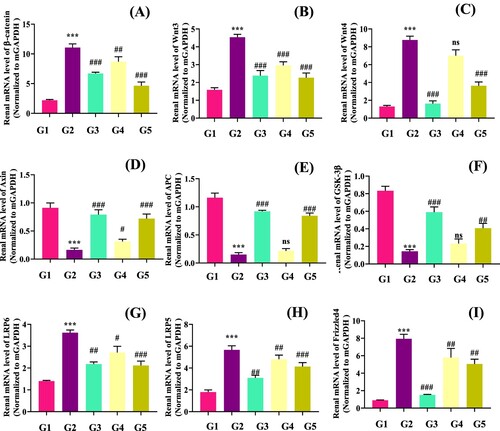
Renal fibrosis is an important pathologic process of hyperuricemia nephropathy, which is mainly caused by deposition of extracellular matrix (ECM). Many mechanisms of UA-mediated renal fibrosis have been reported. Among them, JAK2/STAT3 is an important pathway of renal fibrosis. Previously, Pan et al. (Citation2021) documented that renal interstitial fibrosis may be due to the activation of Src/STAT3 pathway in hyperuricemic renal nephropathy. We also determined renal gene expressions involved in the JAK2/STAT3 signalling pathway. The genistein treatment significantly (p < .05) downregulated the renal mJAK2 (p < .05), mSTAT3 (p < .05) and mSOCS3 (p < .05) gene expression compared with the model group (G2) (). Many polyphenolic compounds (genistein, fisetin, caffeic acid, astilbin, etc.) were reported to suppress the JAK2-STAT3 signalling cascade (Cheng et al., Citation2020; Hu et al., Citation2021). Ethanol extract of Liriodendron chinense (Hemsl.) Sarg barks were also reported to attenuate hyperuricemic nephropathy via inhibiting JAK2/STAT3 signalling pathway in mice (Pan et al., Citation2021).
Figure 7. Genistein attenuates renal fibrosis via inhibiting JAK2/STAT3 pathway and its downstream target genes expression in PO-induced hyperuricemic mice, G1: normal group, G2: Model control group, G3: positive control group, G4: low dose genistein treated group, G5: High dose genistein treated group *p < .05, **p < .01, ***p < .001 compare with G1, #p < .05, ##p < .01, ###p < .001 compare with G2.
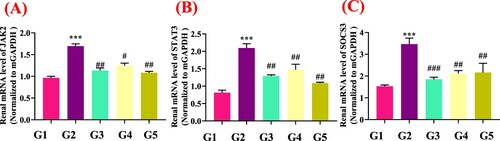
It has been documented that UA-mediated ROS activated the mitogen-activated protein kinase (MAPK) signal transduction pathway which further affected various transcription factors, including nuclear factor kappa-light-chain-enhancer of activated B cells (NF-κβ). ROS enhances the NF-kβ translocation from cytosol into the nucleus during OS, which leads to the generation of nitric oxide (iNOS) and COX-2 inflammatory enzymes. Moreover, NF-κβ altered the gene expression encoding mediators and cytokines such as IL-1β and TNF-α (Ho & Bray, Citation1999; Lee & Burckart, Citation1998). Hence, NF-κβ caspase-1 activation, cytokines play a pivotal role in the inflammatory response during UA-mediated ROS (Qian et al., Citation2019;Yin et al., Citation2015). The genistein (G4 and G5) treatment significantly rebalances the UA-associated renal inflammation in mice (). The polyphenolic compounds were reported to restore the UA-mediated renal inflammation via downregulating inflammatory cytokines levels and inhibiting NRLP3 pathway (Pan et al., Citation2019; Chen et al., Citation2019; Mehmood et al., Citation2020c, Citation2020d; Chen et al., Citation2013; Müller et al., Citation2019; Qian et al., Citation2019).
Figure 8. Effect of genistein on serum inflammatory cytokines levels in PO-induced hyperuricemic mice. (A) Renal PGE2, (B) Renal COX-2, (C) Serum TNF-α, (D) Renal genes expression of NF-κβ, (E) Serum IL-6, (F) Serum IL-1β, (G) Renal MCP-1, G1: normal group, G2: Model control group, G3: positive control group, G4: low dose genistein treated group, G5: High dose genistein treated group, *p < .05, **p < .01, ***p < .001 compare with G1, #p < .05, ##p < .01, ###p < .001 compare with G2.
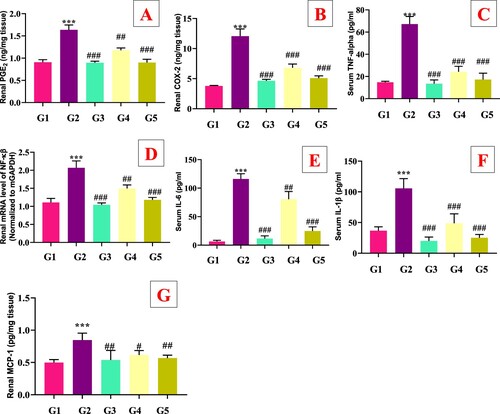
5. Conclusions
In this study, we investigated the protective effect of genistein on PO-induced hyperuricemia in mice. Administration of genistein at the dosage of 10 and 20 mg/kg bw decreased the serum UA level and renal function biomarkers. Genistein decreased XOD and ADA activities in serum and liver. Moreover, genistein treatments (G4 and G5) also downregulated the renal mRNA expression of mURAT1 and mGLUT9 and upregulated mOAT1, mOAT3, mOCT1 and mOCT2 expression in hyperuricemic mice. Genistein also improved antioxidant activities and decreased renal inflammation and fibrosis in PO-induced hyperuricemia mice via JAK2/STAT3 and Wnt/β-catenin pathway. In summary, our results provide an effective treatment for hyperuricemia.
Institutional review board statement
The animal experiment was according to the protocols and Ethical number; A356-60 that approved by Ethical Committee for Scientific the Beijing Key Laboratory of Functional Food from Plant Resources.
Sample availability
Samples of the compounds available from the authors.
Acknowledgment
Bi Wei-Yun contributes to software, validation, formal analysis, writing—original draft preparation and Zhu Cailin contribute interview and editing, visualization, supervision, project administration, funding acquisition
Data availability statement
All data are presented in the figure. However, additional information can be obtained from corresponding author on request.
Disclosure statement
No potential conflict of interest was reported by the author(s).
References
- Aslan, M., Ryan, T. M., Adler, B., Townes, T. M., Parks, D. A., Thompson, J. A., Tousson, A., Gladwin, M. T., Patel, R. P., Tarpey, M. M., & Batinic-Haberle, I. (2001). Oxygen radical inhibition of nitric oxide-dependent vascular function In sickle cell disease. Proceedings of the National Academy of Sciences of the United States of America, 98(26), 15215–15220. https://doi.org/10.1073/pnas.221292098
- Canyilmaz, E., Uslu, G. H., Bahat, Z., Kandaz, M., Mungan, S., Haciislamoglu, E., Mentese, A., & Yoney, A. (2016). Comparison of the effects of melatonin and genistein on radiation–induced nephrotoxicity: Results of an experimental study. Biomedical Reports, 4(1), 45–50. https://doi.org/10.3892/br.2015.547
- Chen, L., Lan, Z., Lin, Q., Mi, X., He, Y., Wei, L., Lin, Y., Zhang, Y., & Deng, X. (2013). Polydatin ameliorates renal injury by attenuating oxidative stress-related inflammatory responses in fructose-induced urate nephropathic mice. Food and Chemical Toxicology, 52, 28–35. https://doi.org/10.1016/j.fct.2012.10.037
- Chen, Y., Li, C., Duan, S., Yuan, X., Liang, J., & Hou, S. (2019). Curcumin attenuates potassium oxonate-induced hyperuricemia and kidney inflammation In mice. Biomedicine & Pharmacotherapy, 118, 109195. https://doi.org/10.1016/j.biopha.2019.109195
- Cheng, W. X., Huang, H., Chen, J. H., Zhang, T. T., Zhu, G. Y., Zheng, Z. T., Lin, J. T., Hu, Y. P., Zhang, Y., Bai, X. L., & Wang, Y. (2020). Genistein inhibits angiogenesis developed during rheumatoid arthritis through the IL-6/JAK2/STAT3/VEGF signalling pathway. The Journal of Orthopaedic Translation, 22(2020), 92–100. https://doi.org/10.1016/j.jot.2019.07.007
- Cristóbal-García, M., García-Arroyo, F. E., Tapia, E., Osorio, H., Arellano-Buendía, A. S., Madero, M., Rodríguez-Iturbe, B., Pedraza-Chaverrí, J., Correa, F., Zazueta, C., & Johnson, R. J. (2015). Renal oxidative stress induced by long-term hyperuricemia alters mitochondrial function and maintains systemic hypertension. Oxidative Medicine and Cellular Longevity, 2015, Article ID 535686. https://doi.org/10.1155/2015/535686
- Cui, D., Liu, S., Tang, M., Lu, Y., Zhao, M., Mao, R., … Liu, J. (2020). Phloretin ameliorates hyperuricemia-induced chronic renal dysfunction through inhibiting NLRP3 inflammasome and uric acid reabsorption. Phytomedicine, 66, 153111. https://doi.org/10.1016/j.phymed.2019.153111
- Dinour, D., Gray, N. K., Campbell, S., Shu, X., Sawyer, L., Richardson, W., … Holtzman, E. J. (2010). Homozygous SLC2A9 mutations cause severe renal hypouricemia. The Journal of the American Society of Nephrology, 21(1), 64–72. https://doi.org/10.1681/ASN.2009040406
- Fukunari, A., Okamoto, K., Nishino, T., Eger, B. T., Pai, E. F., Kamezawa, M., Yamada, I., & Kato, N. (2004). Y-700 [1-[3-cyano-4-(2, 2-dimethylpropoxy) phenyl]-1H-pyrazole-4-carboxylic acid]: a potent xanthine oxidoreductase inhibitor with hepatic excretion. The Journal of Pharmacology and Experimental Therapeutics, 311(2), 519–528. https://doi.org/10.1124/jpet.104.070433
- Guo, L. F., Chen, X., Lei, S. S., Li, B., Zhang, N. Y., Ge, H. Z., Yang, K., Lv, G. Y., & Chen, S. H. (2020). Effects and mechanisms of dendrobium officinalis six nostrum for treatment of hyperuricemia with hyperlipidemia. Evidence-Based Complementary and Alternative Medicine, 2020, 1–12. https://doi.org/10.1155/2020/2914019
- Hanwell, M. D., Curtis, D. E., Lonie, D. C., Vandermeersch, T., Zurek, E., & Hutchison, G. R. (2012). Avogadro: An advanced semantic chemical editor, visualization, and analysis platform. J. Cheminformatics, 4(1), 1–17. https://doi.org/10.1186/1758-2946-4-17
- Hayyan, M., Hashim, M. A., & AlNashef, I. M. (2016). Superoxide ion: Generation and chemical implications. Chemical Reviews, 116(5), 3029–3085. https://doi.org/10.1021/acs.chemrev.5b00407
- Ho, E., & Bray, T. M. (1999). Antioxidants, NF-jB activation, and diabetogenesis. Proceedings of the Society for Experimental Biology and Medicine, 222(3), 205–213. https://doi.org/10.1046/j.1525-1373.1999.d01-137.x
- Hu, Q. P., Huang, X. Y., Feng, W., Chen, F. F., Yan, H. X., Zhang, X., & Zhou, Y. W. (2021). Genistein Protects Epilepsy-Induced Brain Injury Through Regulating The JAK2/STAT3 and Keap1/Nrf2 Signaling Pathways in The Developing Rats.
- Ichida, K. (2009). What lies behind serum urate concentration? Insights from genetic and genomic studies. Genome Medicine, 1(12), 1–10. https://doi.org/10.1186/gm118
- Ichida, K., Hosoyamada, M., Hisatome, I., Enomoto, A., Hikita, M., Endou, H., & Hosoya, T. (2004). Clinical and molecular analysis of patients with renal hypouricemia in Japan-influence of URAT1 gene on urinary urate excretion. The Journal of the American Society of Nephrology, 15(1), 164–173. https://doi.org/10.1097/01.ASN.0000105320.04395.D0
- Ishaq, M., Mehmood, A., Ur Rehman, A., Dounya Zad, O., Li, J., Zhao, L., … Lian, Y. (2020). Antihyperuricemic effect of dietary polyphenol sinapic acid commonly present in various edible food plants. J. Food Biochem, 44(2), e13111. https://doi.org/10.1111/jfbc.13111
- Jalili, C., Rashidi, I., Roshankhah, S., Jalili, F., & Salahshoor, M. R. (2020). Protective effect of genistein on the morphine-induced kidney disorders in male mice. Electronic Journal of General Medicine, 17(3), 1–7. https://doi.org/10.29333/ejgm/7874
- Javani, G., Alihemmati, A., Habibi, P., Yousefi, H., Karimi, P., Ebraheimi, V., & Ahmadiasl, N. (2019). The effects of genistein on renal oxidative stress and inflammation of ovariectomized rats. Jundishapur Journal of Natural Pharmaceutical Products, 14(4). https://doi.org/10.5812/jjnpp.57149
- Jia, Q., Yang, R., Liu, X. F., Ma, S. F., & Wang, L. (2019). Genistein attenuates renal fibrosis in streptozotocin–induced diabetic rats. Molecular Medicine Reports, 19(1), 423–431. https://doi.org/10.3892/mmr.2018.9635
- Kim, M. J., & Lim, Y. (2013). Protective effect of short-term genistein supplementation on the early stage in diabetes-induced renal damage. Mediators of Inflammation, 2013, 1–25. https://doi.org/10.1155/2013/510212
- Lan, Z., Liu, J., Chen, L., Fu, Q., Luo, J., Qu, R., Kong, L., & Ma, S. (2012). Danggui-Shaoyao-San ameliorates cognition deficits and attenuates oxidative stress-related neuronal apoptosis in d-galactose-induced senescent mice. The Journal of Ethnopharmacology, 141(1), 386–395. https://doi.org/10.1016/j.jep.2012.02.050
- Lee, J. I., & Burckart, G. J. (1998). Nuclear factor kappa B: Important transcription factor and therapeutic target. The Journal of Clinical Pharmacology, 38(11), 981–993. https://doi.org/10.1177/009127009803801101
- Lee, Y. S., Kim, S. H., Yuk, H. J., & Kim, D. S. (2018). DKB114, a mixture of Chrysanthemum indicum linne flower and Cinnamomum cassia (L.) J. presl bark extracts, improves hyperuricemia through inhibition of xanthine oxidase activity and increasing urine excretion. Nutrients, 10(10), 1381. https://doi.org/10.3390/nu10101381
- Li, Y., Zhao, Z., Luo, J., Jiang, Y., Li, L., Chen, Y., Zhang, L., Huang, Q., Cao, Y., Zhou, P., & Pang, J. (2021). Apigenin ameliorates hyperuricemic nephropathy by inhibiting URAT1 and GLUT9 and relieving renal fibrosis via the Wnt/β-catenin pathway. Phytomedicine, 87, 153585. https://doi.org/10.1016/j.phymed.2021.153585
- Liu, R., Han, C., Wu, D., Xia, X., Gu, J., Guan, H., … Teng, W. (2015). Prevalence of hyperuricemia and gout In mainland China from 2000 to 2014: A systematic review and meta-analysis. BioMed Research International, 2015, 1–17. https://doi.org/10.1155/2015/762820
- Mandal, A. K., & Mount, D. B. (2015). The molecular physiology of uric acid homeostasis. The Annual Review of Physiology, 77(1), 323–345. https://doi.org/10.1146/annurev-physiol-021113-170343
- Mehmood, A., Ishaq, M., Zhao, L., Safdar, B., Rehman, A. U., Munir, M., Raza, A., Nadeem, M., Iqbal, W., & Wang, C. (2019a). Natural compounds with xanthine oxidase inhibitory activity: A review. Chemical Biology & Drug Design, 93(4), 387–418. https://doi.org/10.1111/cbdd.13437
- Mehmood, A., Rehman, A. U., Ishaq, M., Zhao, L., Li, J., Usman, M., Zhao, L., Rehman, A., Zad, O. D., & Wang, C. (2020a). In vitro and in silico xanthine oxidase inhibitory activity of selected phytochemicals widely present in various edible plants. Combinatorial Chemistry & High Throughput Screening, 23(9), 917–930. https://doi.org/10.2174/1386207323666200428075224
- Mehmood, A., Zhao, L., Ishaq, M., Safdar, B., Wang, C., & Nadeem, M. (2018). Optimization of total phenolic contents, antioxidant, and invitro xanthine oxidase inhibitory activity of sunflower head. Journal of Food, 16(1), 957–964. https://doi.org/10.1080/19476337.2018.1504121
- Mehmood, A., Zhao, L., Ishaq, M., Usman, M., Zad, O. D., Hossain, I., Raka, R. N., Naveed, M., Zhao, L., Wang, C., & Nadeem, M. (2020b). Uricostatic and uricosuric effect of grapefruit juice in potassium oxonate-induced hyperuricemic mice. The Journal of Food Biochemistry, 23(9), 917–930. https://doi.org/10.2174/1386207323666200428075224
- Mehmood, A., Zhao, L., Ishaq, M., Xin, W., Zhao, L., Wang, C., Hossen, I., Zhang, H., Lian, Y., & Xu, M. (2020c). Anti-hyperuricemic potential of stevia (Stevia rebaudiana bertoni) residue extract in hyperuricemic mice. Food & Function, 11(7), 6387–6406. https://doi.org/10.1039/C9FO02246E
- Mehmood, A., Zhao, L., Ishaq, M., Zad, O. D., Zhao, L., Wang, C., Usman, M., Lian, Y., & Xu, M. (2020d). Renoprotective effect of stevia residue extract on adenine-induced chronic kidney disease in mice. Journal of Functional Foods, 72, 103983. https://doi.org/10.1016/j.jff.2020.103983
- Mehmood, A., Zhao, L., Wang, C., Hossen, I., & Nadeem, M. (2020e). Stevia residue extract alone and combination with allopurinol attenuate hyperuricemia in fructose–PO-induced hyperuricemic mice. J. Food Biochem, 44(1), e13087. https://doi.org/10.1111/jfbc.13087
- Mehmood, A., Zhao, L., Wang, C., Nadeem, M., Raza, A., Ali, N., & Shah, A. A. (2019b). Management of hyperuricemia through dietary polyphenols as a natural medicament: A comprehensive review. Critical Reviews in Food Science and Nutrition, 59(9), 1433–1455. https://doi.org/10.1080/10408398.2017.1412939
- Müller, d. S., Coelho, C. M., Araújo, G. B., Saúde-Guimarães, M. C. D. P. M., & A, D. (2019). Lychnophora pinaster ethanolic extract and its chemical constituents ameliorate hyperuricemia and related inflammation. The Journal of Ethnopharmacology, 242, 112040. https://doi.org/10.1016/j.jep.2019.112040
- Nigam, S. K., Bush, K. T., & Bhatnagar, V. (2007). Drug and toxicant handling by the OAT organic anion transporters In the kidney and other tissues. Nature Reviews Nephrology, 3(8), 443–448. https://doi.org/10.1038/ncpneph0558
- Ning, Y., Chen, J., Shi, Y., Song, N., Yu, X., Fang, Y., & Ding, X. (2020). Genistein ameliorates renal fibrosis through regulation snail via m6A RNA demethylase ALKBH5. Frontiers in Pharmacology, 11. https://doi.org/10.3389/fphar.2020.579265
- Nusse, R., & Clevers, H. (2017). Wnt/β-catenin signaling, disease, and emerging therapeutic modalities. Cell, 169(6), 985–999. https://doi.org/10.1016/j.cell.2017.05.016
- Osarogiagbon, U. R., Choong, S., Belcher, J. D., Vercellotti, G. M., Paller, M. S., & Hebbel, R. P. (2000). Reperfusion injury pathophysiology in sickle transgenic mice. Blood, 96(1), 314–320. https://doi.org/10.1182/blood.V96.1.314
- Pan, J., Shi, M., Li, L., Liu, J., Guo, F., Feng, Y., Ma, L., & Fu, P. (2019). Pterostilbene, a bioactive component of blueberries, alleviates renal fibrosis in a severe mouse model of hyperuricemic nephropathy. Biomedicine & Pharmacotherapy, 109, 1802–1808. https://doi.org/10.1016/j.biopha.2018.11.022
- Pan, J., Zhang, C., Shi, M., Guo, F., Liu, J., Li, L., Ren, Q., Tao, S., Tang, M., Ye, H., & Fu, P. (2021). Ethanol extract of Liriodendron chinense (Hemsl.) Sarg barks attenuates hyperuricemic nephropathy by inhibiting renal fibrosis and inflammation in mice. The Journal of Ethnopharmacology, 264, 113278. https://doi.org/10.1016/j.jep.2020.113278
- Pang, M., Fang, Y., Chen, S., Zhu, X., Shan, C., Su, J., Yu, J., Li, B., Yang, Y., Chen, B., & Lv, G. (2017). Gypenosides inhibits xanthine oxidoreductase and ameliorates urate excretion in hyperuricemic rats induced by high cholesterol and high fat food (lipid emulsion). Medical Science Monitor: International Medical Journal of Experimental and Clinical Research, 23(2017), 1129–1140. https://doi.org/10.12659/msm.903217
- Pavese, J. M., Farmer, R. L., & Bergan, R. C. (2010). Inhibition of cancer cell invasion and metastasis by genistein. Cancer and Metastasis Reviews, 29(3), 465–482. https://doi.org/10.1007/s10555-010-9238-z
- Qian, X., Wang, X., Luo, J., Liu, Y., Pang, J., Zhang, H., Xu, Z., Xie, J., Jiang, X., & Ling, W. (2019). Hypouricemic and nephroprotective roles of anthocyanins in hyperuricemic mice. Food & Function, 10(2), 867–878. https://doi.org/10.1039/C8FO02124D
- Rehman, K., Ali, M. B., & Akash, M. S. H. (2019). Genistein enhances the secretion of glucagon-like peptide-1 (GLP-1) via downregulation of inflammatory responses. Biomedicine & Pharmacotherapy, 112, 108670. https://doi.org/10.1016/j.biopha.2019.108670
- Robinson, P. C. (2018). Gout–An update of aetiology, genetics, co-morbidities and management. Maturitas, 118, 67–73. https://doi.org/10.1016/j.maturitas.2018.10.012
- Schmidt, H. M., Kelley, E. E., & Straub, A. C. (2018). The impact of xanthine oxidase (XO) on hemolytic diseases. Redox Biology, 21(2019), 101072. https://doi.org/10.1016/j.redox.2018.101072
- Stephenson, T. J., Setchell, K. D. R., Kendall, C. W. C., Jenkins, D. J. A., Anderson, J. W., & Fanti, P. (2005). Effect of soy protein-rich diet on renal function in young adults with insulin-dependent diabetes mellitus. Journal of Clinical Nephrology, 64(1), 1–11. https://doi.org/10.5414/cnp64001
- Sung, M. J., Kim, D. H., Jung, Y. J., Kang, K. P., Lee, A. S., Lee, S., … Park, S. K. (2008). Genistein protects the kidney from cisplatin-induced injury. Kidney International, 74(12), 1538–1547. https://doi.org/10.1038/ki.2008.409
- Tan, J., Wan, L., Chen, X., Li, X., Hao, X., Li, X., … Ding, H. (2019). Conjugated linoleic acid ameliorates high fructose-induced hyperuricemia and renal inflammation in rats via NLRP3 inflammasome and TLR4 signaling pathway. Molecular Nutrition & Food Research, 63(12), 1801402. https://doi.org/10.1002/mnfr.201801402
- Tang, D. H., Ye, Y. S., Wang, C. Y., Li, Z. L., Zheng, H., & Ma, K. L. (2017). Potassium oxonate induces acute hyperuricemia In the tree shrew (tupaia belangeri chinensis). Experimental Animals, 66(3), 209–216. https://doi.org/10.1538/expanim.16-0096
- Wang, M., Chen, D. Q., Chen, L., Cao, G., Zhao, H., Liu, D., Vaziri, N. D., Guo, Y., & Zhao, Y. Y. (2018a). Novel inhibitors of the cellular RAS components, poricoic acids, target Smad3 phosphorylation and Wnt/β-catenin pathway against renal fibrosis. The British Journal of Pharmacology, 175(13), 1384–1395. https://doi.org/10.1111/bph.14333
- Wang, M., Chen, D. Q., Chen, L., Liu, D., Zhao, H., Zhang, Z. H., Vaziri, N. D., Guo, Y., Zhao, Y. Y., & Cao, G. (2018b). Novel RAS inhibitors poricoic acid ZG and poricoic acid ZH attenuate renal fibrosis via a Wnt/β-catenin pathway and targeted phosphorylation of smad3 signaling. Journal of Agricultural and Food Chemistry, 66(8), 1828–1842. https://doi.org/10.1021/acs.jafc.8b00099
- Wang, X., Wang, C. P., Hu, Q. H., Lv, Y. Z., Zhang, X., OuYang, Z., & Kong, L. D. (2010). The dual actions of sanmiao wan as a hypouricemic agent: Down-regulation of hepatic XOD and renal mURAT1 in hyperuricemic mice. The Journal of Ethnopharmacology, 128(1), 107–115. https://doi.org/10.1016/j.jep.2009.12.035
- Xiao, L., Zhou, D., Tan, R. J., Fu, H., Zhou, L., Hou, F. F., & Liu, Y. (2016). Sustained activation of Wnt/β-catenin signaling drives AKI to CKD progression. The Journal of the American Society of Nephrology, 27(6), 1727–1740. https://doi.org/10.1681/ASN.2015040449
- Xu, Y., Cao, X., Zhao, H., Yang, E., Wang, Y., Cheng, N., & Cao, W. (2021). Impact of Camellia japonica bee pollen polyphenols on hyperuricemia and gut microbiota in potassium oxonate-induced mice. Nutrients, 13(8), 2665. https://doi.org/10.3390/nu13082665
- Yang, H., Bai, W., Gao, L., Jiang, J., Tang, Y., Niu, Y., Lin, H., & Li, L. (2018). Mangiferin alleviates hypertension induced by hyperuricemia via increasing nitric oxide releases. Journal of Pharmacological Sciences, 137(2), 154–161. https://doi.org/10.1016/j.jphs.2018.05.008
- Yin, Y., Li, X., Sha, X., Xi, H., Li, Y. F., Shao, Y., Mai, J., Virtue, A., Lopez-Pastrana, J., Meng, S., & Tilley, D. G. (2015). Early hyperlipidemia promotes endothelial activation via a caspase-1-sirtuin 1 pathway. Arteriosclerosis, Thrombosis, and Vascular Biology, 35(4), 804–816. https://doi.org/10.1161/ATVBAHA.115.305282
- Yong, T., Zhang, M., Chen, D., Shuai, O., Chen, S., Su, J., Yong, T., Zhang, M., Chen, D., Shuai, O., Chen, S., Su, J., Jiao, C., Feng, D., & Xie, Y. (2016). Actions of water extract from Cordyceps militaris in hyperuricemic mice induced by potassium oxonate combined with hypoxanthine. Journal of Ethnopharmacology, 194, 403–411. https://doi.org/10.1016/j.jep.2016.10.001
- Zeng, Y., Zhang, R., Wu, J., Liu, M., Peng, W., Yu, X., & Yang, X. (2012). Organic anion transporter 1 (OAT1) involved in renal cell transport of aristolochic acid. Human & Experimental Toxicology, 31(8), 759–770. https://doi.org/10.1177/0960327111424302
- Zhang, Y., & Chen, H. (2011). Genistein attenuates WNT signaling by up-regulating sFRP2 In a human colon cancer cell line. Experimental Biology and Medicine, 236(6), 714–722. https://doi.org/10.1258/ebm.2011.010347
- Zhou, Q., Jiang, S., Zhou, T., Tian, J., & Chen, J. (2017). A study comparing the safety and efficacy of febuxostat, allopurinol, and benzbromarone in Chinese gout patients: A retrospective cohort study. International Journal of Clinical Pharmacology and Therapeutics, 55(2), 163. https://doi.org/10.5414/CP202629
- Zhou, Y., Zhao, M., Pu, Z., Xu, G., & Li, X. (2018). Relationship between oxidative stress and inflammation in hyperuricemia: Analysis based on asymptomatic young patients with primary hyperuricemia. Medicine, 97(49), e13108. https://doi.org/10.1097/MD.0000000000013108
- Zuo, Y., & Liu, Y. (2018). New insights into the role and mechanism of Wnt/β-catenin signalling in kidney fibrosis. Nephrology, 23, 38–43. https://doi.org/10.1111/nep.13472