ABSTRACT
The aim of the study was to assess the effect of effective microorganisms on selected parameters of the cellular immune response in the colostrum and milk of sows and in the serum of suckling piglets. A total of 60 female crossbred sows and 60 female piglets were used in the experiment. The material for analysis comprised colostrum and milk collected from sows and blood samples from piglets. Immune response parameters were determined by flow cytometry and ELISA kits. The results showed high expression of CD4-CD25-FoxP3, CD4, and CD25 in the colostrum and milk of sows and of CD4, CD8 in piglet blood. The use of EM Bokashi® as a feed supplement for sows during colostrogenesis stimulates cellular immune mechanisms, expressed by an increase in the Treg cell population in the colostrum and milk, which contributes to effective elimination of microorganisms in the first period of piglets’ life.
Introduction
One of the contemporary challenges of pig farming technology is to increase the productivity and health of pigs while at the same time reducing costs (Tokach et al., Citation2016). The functioning of the reproduction sector is of particular significance for increasing production efficiency (Gaugler et al., Citation1983; Koketsu et al., Citation2017; Tokach et al., Citation2016). At this stage, it is especially important to maintain the health status of the foundation stock of sows and to obtain numerous healthy offspring (Holm et al., Citation2004). While genetic selection has made it possible to achieve high prolificacy in sows, this entails excessive exploitation of these animals (Warda et al., Citation2021). This results in a reduction in the body weight of lactating sows and a decrease in the quantity and quality of the colostrum and milk, which can lead to culling of sows from the herd (Koketsu, Citation2000). Piglets from large litters also have lower average birth weight and ingest less colostrum and milk, which can lower their resistance and thereby reduce their viability and survival rate during the period before weaning (Declerck et al., Citation2016; Douglas et al., Citation2014). Irrespective of these factors, newborn piglets are exposed to numerous pathogenic microbes in their environment that can induce diarrhoea, increasing the risk of death during the pre-weaning period (Choct, Citation2009; Fairbrother et al., Citation2005; Rhouma et al., Citation2017).
An important element of sustainable pig production that helps to limit losses is the proper feeding of sows and piglets during the rearing period (Blavi et al., Citation2021). The administrative ban on the use of antibiotics as feed additives to stimulate growth and reduce the occurrence of infections in pigs has prompted pig producers to seek alternative feeding methods to ensure the animals’ optimal growth and health (Close, Citation2000; Turner et al., Citation2001). Among feed additives that improve the production and health parameters of pigs, probiotics are considered the most important (Ahmed et al., Citation2014; Cho et al., Citation2011). These preparations are most often produced using microbes of the genera Bacillus, Lactobacillus, Enterococcus, Bifidobacterium and Saccharomyces (Chen et al., Citation2005, Citation2006; Choi et al., Citation2011). Numerous studies have shown that the use of probiotics in sows during pregnancy and lactation helps to maintain the microbial balance in the intestines and increase the efficiency of nutrient utilization and absorption from feed (Chaucheyras-Durand & Durand, Citation2010; Stamati et al., Citation2006). The improvement in nutrient digestibility resulting from the use of probiotic additives in sows has a positive effect on production parameters, increasing milk yield, litter size, the number of live-born piglets, and the body weight of suckling piglets at birth and at weaning (Alexopoulos et al., Citation2004; Alonso et al., Citation2016; Liu et al., Citation2017; Stamati et al., Citation2006). Mori et al. (Citation2011) showed that administration of probiotics as feed supplements to pregnant sows causes changes in the composition of the gut microbiota, which significantly decreases the number of pathogenic and conditionally pathogenic bacteria, such as Salmonella, Escherichia coli and Clostridium, in the environment of suckling piglets. A beneficial effect of these changes is a reduction in the frequency of diseases with symptoms of diarrhoea in piglets. It is worth noting that the use of probiotics as feed additives in sows also has a stimulating effect on the development of the immune system in the foetus and suckling piglet (Schultz et al., Citation2004) and on the quantity and qualitative composition of colostrum and milk (Alexopoulos et al., Citation2004; Jang et al., Citation2013; Stamati et al., Citation2006; Wang et al., Citation2011). This is particularly important for building potential immunity to infections in the first period of life. Experimental studies have also confirmed that the use of probiotics in feed for piglets in the period from birth to weaning improves production indicators by increasing body weight at weaning and stimulates the non-specific immune response, expressed as increased concentrations of class G immunoglobulins (IgG) in the serum (Ahmed et al., Citation2014; Scharek et al., Citation2005).
The health-promoting effects of probiotic preparations in the diet of animals depend on a number of factors, primarily the genus, species and stain of microbes used in their production (Sanders & Huis in't Veld, Citation1999). The authors of many experimental studies suggest that probiotics based on different strains of the same species or on strains of a variety of species are the most effective (Rolfe, Citation2000). Supplementation of feed for pigs with probiotics containing strains of different species of microbes has been shown to significantly improve production parameters, including feed conversion and growth (Cai et al., Citation2014; Inatomi et al., Citation2017; Wang et al., Citation2014). One of these, the multi-strain probiotic preparation Bokashi, which combines a variety of species of effective microorganisms (EMs), is currently widely used in multiple areas of production associated with agriculture and livestock farming (Higa & Parr, Citation1994; Mroz, Citation2001; Rashid & West, Citation2007; Szymanski & Patterson, Citation2003). The beneficial effects of EMs on production and health parameters include increased daily weight gains, improved feed digestibility, improved health, and reduced mortality rates (Betancur et al., Citation2021; Gaggia et al., Citation2010; Hanekon et al., Citation2000; Konoplya & Higa, Citation2000; Kumprechtova et al., Citation2000). The use of EMs as feed additives in sows also increases the protective potential of colostrum and milk, helping to reduce infections in piglets (Hasan et al., Citation2018; Higa & Parr, Citation1994; Laskowska et al., Citation2019; Zhang et al., Citation2018).
Our own preliminary research has shown that the use of EMs in the diet of sows causes an increase in the concentrations of pro-inflammatory cytokines TNF-α and IL-6, as well as anti-inflammatory cytokines IL-4, IL-10 and TGF-β and immunoglobulins in the colostrum and milk. These findings clearly indicate that EMs have an immunoregulatory effect on Th2 cells and suggest that they stimulate cellular immune mechanisms protecting the sow and newborn piglets against infection (Laskowska et al., Citation2019).
As yet no extensive research has been conducted on the effect of EMs in the diet of sows during pregnancy and lactation and in suckling piglets or on their effect on cellular immune mechanisms in piglets and the quality of the colostrum and milk. The aim of the study was to assess the effect of EMs on selected parameters of the cellular immune response in the colostrum and milk of sows and in the serum of suckling piglets by determining the percentages of CD3+CD4+, CD3+CD8+, CD25+, CD4+CD8+ (DP) and CD4+CD25+FoxP3+ T lymphocytes, and to assess the qualitative composition of the colostrum and milk of sows receiving a diet supplemented with EMs.
Materials and methods
Experimental animals and use of effective microorganisms
The study was conducted on a pig breeding farm with a closed production cycle. The farm kept 150 sows aged 2–4 years with a body weight of 150–200 kg. From mating to 100 days of gestation the sows were kept in individual pens on litter. Pregnant and lactating sows were fed individual diets depending on the stage of gestation and the number of piglets being reared. The sows were fed PR-C (full-feed diet for the gestation period) twice daily during pregnancy and PR-L (full-feed diet for the lactation period) during lactation, with constant access to water.
All procedures used during the research were approved by the Local Ethics Committee for Animal Testing at the University of Life Sciences in Lublin, Poland (approval number 55/2013, 15 October 2013). The first part of the experiment was conducted on 60 female crossbred sows (Polish Large White x Polish Landrace) in their second parity, randomly selected from the herd, with an initial body weight of 160–180 kg and at the age of 2 years, all born on the same farm (). The sows were assigned to two groups, with 30 sows in each group: control group (A) and experimental group (B). Sows were included in the study on the basis of body condition score (BCS) according to Charette et al. (Citation1996); the BCS of the sows in both groups was 3. The control and experimental groups were housed in separate pig houses, with identical building and environmental conditions, in order to prevent probiotic (EM Bokashi®) cross-contamination. The size, temperature, humidity and hygiene conditions of the pens where the sows were kept were identical for the two groups. Each sow was housed in an individual pen (2200 cm × 2000 cm) during the gestation and lactation period. The lighting program was 16 h light and 8 h darkness. The housing area was kept at 20–21.5°C under humidity of 60–65%, and the ventilation rate was 0.567–0.598 m3/min per sow. All sows were fed the same basal diet during the experiment (). From fertilization to the 90th day of gestation, the sows received feed for pregnant sows (PR-C), and from the 90th day of gestation to the 28th day of lactation, they received feed for lactating sows (PR-L). For the sows in group B, the experimental group, effective microorganisms in the form of the preparation EM Bokashi® were added to the basal feed in the amount of 10 kg/tonne of feed, from mating to weaning.
Table 1. Ingredients and nutritive value of the pigs’ diets (as-fed basis).
The next stage of the experiment was conducted on 30 female piglets (group I) born to the sows in group A and 30 female piglets (group II) born to the sows in group B (). All piglets were born at the same time, with a birth weight of 1.10–1.30 kg. The newborn piglets had constant access to fresh drinking water. From the age of 7 days, they had constant access to complete compound feed for piglets (). The diets were formulated to meet or exceed the nutrient requirement recommendations of the NRC (Citation1998). All piglets were fed the same basal diet during the experiment. For the piglets in group II, the experimental group, a probiotic in the form of the preparation EM Bokashi® was added to the basal feed in the amount of 10 kg/tonne of feed, from the age of 7 days to the end of the experiment.
The probiotic EM Bokashi® used in the experiment is manufactured by the company Greenland Technologia EM (Janowiec, Poland). It contains a mixture of microorganisms (). Throughout the experiment, EM Bokashi® was tested once a month in the national reference laboratory of the Department of Hygiene of Animal Feedingstuffs of the National Veterinary Research Institute in Puławy, Poland. In addition, the manufacturer of EM Bokashi® assessed the viability of the probiotic bacterial cells and their content per gram of product in their laboratory, thereby guaranteeing that the product used in the experimental group of sows and piglets was of the same quality.
Table 2. EM Bokashi composition.
Collection and preparation of colostrum and milk samples
Sixty 10 mL samples of colostrum and milk were collected into sterile plastic tubes (Medlab Products, Poland) over a period of 7 days. The samples were transported to the laboratory at a temperature of +4°C to 8°C for no longer than 1 h. Immediately upon delivery to the laboratory, 5 mL of material was designated for cytometric analysis, and the remainder was frozen and stored at –80°C until further analysis. The samples, in 5 mL volumes, were centrifuged at 1300g for 30 min at 4°C. Then the fat layer and supernatant were separated and removed. The precipitate, containing cells of the mammary gland secretion, was washed twice with 2 mL of RPMI-1640 solution (Biomed, Lublin) with the addition of 10% foetal bovine serum, antibiotics (penicillin and streptomycin), and an antifungal agent (amphotericin B). After each wash, the samples were centrifuged at 1300g for 10 min at 4°C. After the final centrifugation, the cells were suspended in 2 mL of sterile PBS.
Blood sample collection
Peripheral blood was collected from the external jugular vein into Vacuette vacuum tubes containing EDTA-K2 as an anticoagulant (Medlab Products, Poland) and into serum separation tubes with a clot activator (Medlab Products, Poland). Blood samples were collected from all piglets in groups I and II at 0, 7, 14, 21, and 28 days after birth. The samples were transported chilled to the laboratory at +48°C for no longer than 1 h. The blood collected into EDTA-K2 and heparin tubes was used for cytometric analysis immediately upon delivery to the laboratory. The blood collected into serum separation tubes with a clot activator was centrifuged at room temperature (20-22°C) for 15 min at 1,000 g, and the serum obtained was apportioned and stored for further study at –80°C.
Determination of selected immune response parameters
Immune response parameters were determined by flow cytometry. Samples of colostrum and milk from sows and blood from piglets were analysed in a flow cytometer (BD FACSVerse™, Becton Dickinson, Franklin Lakes, Brea, New Jersey, USA). The immunophenotype (CD) of cells of the colostrum and milk of sows and blood of piglets was assessed according to Le Jan (Citation1994). Intracellular FoxP3+ staining in cells of the colostrum and milk of sows and blood of piglets was assessed according to Wen et al. (Citation2012) and Käser et al. (Citation2008). FoxP3 expression was determined using the Foxp3/Transcription Factor Staining Buffer Set. Cells from the colostrum and milk of sows and the blood of piglets were first stained with CD4:FITC+/CD25:Alexa Fluor® 647+. After staining of cell surface markers, the samples were permeabilized with FoxP3 Fixation/Permeabilization Concentrate (eBiosciences) at 4°C for 30 min. Then the cells were washed with FoxP3 Permeabilization Buffer and stained with FOXP3:RPE+. Performance quality control (PQC) was carried out using BD FACSuite CS&T research beads as outlined in the BD FACSVerse System User’s Guide.2. Data were acquired using a BD FACSVerse system and BD FACSuite software version 1.0.0.1477. The data was analysed and a data report was automatically generated. From 10,000 to 30,000 events were collected in each measurement. Electronic compensation was used to eliminate residual spectral overlaps between individual fluorochromes.
Monoclonal antibodies
Fluorochrome-labelled monoclonal antibodies for the surface molecules of pig lymphocytes were used for cytometric tests: Mouse anti-Pig CD25:Alexa Fluor® 647, clone K231.3B2; Mouse anti-Pig CD4:FITC, clone MIL17; Mouse anti-Pig CD3:RPE, clone PPT3; Mouse anti-Pig CD3:FITC, clone PPT3; Mouse anti-Pig wCD8:RPE clone 11/295/33 from Bio-Rad Laboratories, Inc. Life Science Research Group (Hercules, California, United States), and FOXP3 Monoclonal Antibody (FJK-16s), PE from eBiosciences (San Diego, California, United States). The following types of antibodies were used in the negative isotopic control: rat IgG2a kappa PE isotype control (eBR2a), mouse IgG1 negative control:Alexa Fluor® 647, mouse IgG1 negative control:RPE, mouse IgG2b negative control:FITC, mouse IgG2a negative control:RPE. Direct cell labelling was used. In each colostrum, milk and blood sample, CD3:RPE+/CD4:FITC+, CD3:FITC+/CD8:RPE+, CD8:RPE+/CD4:FITC+, CD25: Alexa Fluor® 647+, and CD4:FITC+/CD25:Alexa Fluor® 647+/ FOXP3:RPE+ were determined separately.
Analysis of colostrum samples using a fluorescence microscope
Stained cells were visualized under a Leica DMi8 fluorescence microscope (Leica, Germany). Photographs were taken under FLUO-PH contrast with an FITC (cd25) or DA/FI/TX (cd84) cube filter using the inverted Leica DMi8 microsystem (Leica, Germany) with Leica LAS X Imaging and Analysis Software for Wildfield Microscopes (Leica, Germany). Settings were as follows: Camera DMC6200-2000000379, digitalization: 8 bit, composite colour capture mode, objective: HC PL FLUOROTAR L 20x/0.4 DRY, exposure time: 1.711 ms.
Analysis of the composition of sows’ colostrum
The percentage content of solids, fat, protein, lactose, and solids-not-fat (SNF) in sows’ colostrum was determined in the Bentley 150 Infrared Analyser. The somatic cell count in the colostrum was determined using the Bentley 150 Somacount Analyser. The results were expressed as mean and standard deviation (± SEM); values of p < 0.05 were considered significant.
Immunoglobulin analysis in sow colostrum
ELISA kits specific for porcine IgG and IgA (USCN Life Science Inc., Wuhan) were used to determine the immunoglobulin levels in the sow colostrum, following the manufacturer's instructions. Each sample was tested in 3 replicates. The results were expressed as mean and standard deviation (± SEM); values of p < .05 were considered significant.
Statistical analysis
Statistical analysis of the results was performed using Statistica 13.2 PL (StatSoft, Krakow, Poland). A gradient of the data was analysed by the Shapiro–Wilk test, and the results were expressed as arithmetic means and standard deviation.
Due to deviations from the normal distribution, nonparametric tests were used to determine the significance of statistical differences (p < .05) between the control and experimental samples.
The Friedman test and post hoc tests at p < .05 were used to compare the effect of time on the results in the control and experimental (with EMs) groups of piglets and sows. These are nonparametric counterparts of one-way analysis of variance (ANOVA) for repeatable measurements. The grouping variable was time: for piglets, days 0, 7, 14, 21 and 28 and for sows, days 0, 1, 2, 3, 4, 5, 6 and 7. The results were also confirmed using Kendall's coefficient of concordance. This coefficient ranges from 0 to 1; the higher the value, the greater the concordance indicating correlation (absence of randomness) of the results.
The Mann–Whitney U test was used to determine the statistical significance of differences between the control and experimental groups for piglets and sows. The grouping variable was the time of measurement.
The results were presented in graphical form ( and ); the same letters indicate the absence of statistically significant differences. Capital letters designate statistically significant results of comparisons between groups (U test), while lower case letters indicate differences arising from the Friedman and post-hoc tests.
Figure 2. Results of Freedman test, post hoc tests (lowercase letters) and the Mann–Whitney U test (capital letters) for sows: I – average percentage of CD3+CD4+ cells, II – average percentage of CD3+CD8+ cells, III – average percentage of CD25+ cells, IV – average percentage of CD4+CD25+FoxP3+ cells, V – average percentage of CD4+CD8+ (DP – double-positive) cells. Solid line – control group A, dotted line – experimental group B, SD – standard deviation.
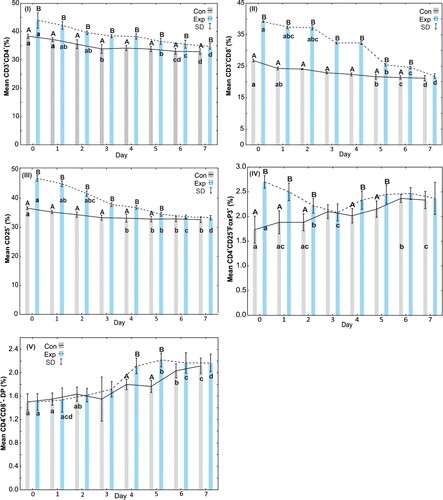
Figure 3. Results of Freedman test, post hoc tests (lowercase letters) and the Mann–Whitney U test (capital letters) for piglets: I – average percentage of CD3+CD4+ cells, II – average percentage of CD3+CD8+ cells, III – average percentage of CD25+ cells, IV – average percentage of CD4+CD25+FoxP3+ cells, V – average percentage of CD4+CD8+ (DP – double-positive) cells. Solid line – control group I, dotted line – experimental group II, SD – standard deviation.
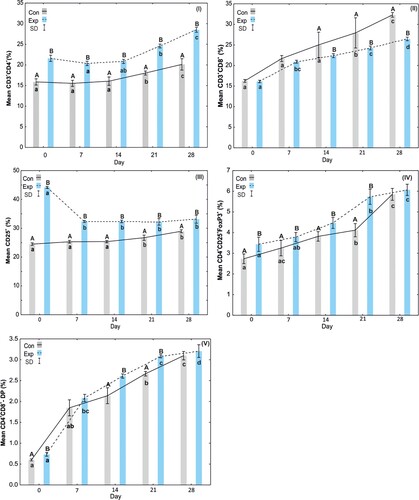
Results
Immune response parameters in the colostrum and milk of sows
The results indicate that the percentage of CD3+CD4+ cells in the colostrum and milk of the sows, both in the control group and in the group receiving EM Bokashi®, was highest on day 0 of the study and gradually decreased up to the last (seventh) day of the experiment. The percentage of CD3+CD4+ cells in the supplemented group was statistically significantly higher on all days of the study than on the corresponding days in the control group. In the supplemented group, statistically significant differences were observed in the CD3+CD4+ percentage on days 3, 6 and 7 in relation to the remaining days ((I)).
The analysis of the CD3+CD8+ percentage showed a gradual decrease from day 0 to day 7 of the study in both groups. The percentage of CD3+CD8+ in the group of supplemented sows was statistically significantly higher than in the control group from days 0 to 6 of the study. On the seventh day, both groups had similar CD3+CD8+ values. In the control group, statistically significant differences in this parameter appeared on days 5, 6 and 7 of the study compared to the previous days. Similar differences were observed in the supplemented group ((II)).
The analysis of the percentage of CD25+ cells in the control and supplemented groups showed a gradual decrease from day 0 to the last day of the study. Statistically significant differences in the percentage of CD25+ cells in the two groups were observed from day 0 to 5 of the experiment. On the 6th and 7th day of the study, the percentage of CD25+ cells was at the same level in both groups. In the group receiving EM Bokashi®, statistically significant differences in the percentage of CD25+ cells were observed on days 5, 6 and 7 ((III) and ).
Figure 4. Microscopic analysis of a fluorochrome-labelled colostrum sample using a Leica DMi8 fluorescence microscope (Leica, Germany). Measurement parameters: Camera DMC6200-2000000379, digitalization: 8 bit, composite colour capture mode, objective: HC PL FLUOROTAR L 20x / 0.4 DRY, exposure time: 1.711 ms. ↑ (red arrow – 1) – CD25+ colostrum cells, ↑ (yellow arrow – 2) – epithelial cell.
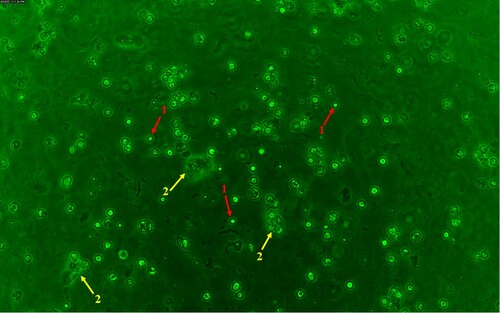
Analysis of the percentage of CD4+CD25+FOXP3+ cells showed statistically significant differences between the control and EM Bokashi®-supplemented groups on days 0, 1 and 2. The percentage of CD4+CD25+FOXP3+ cells was high in the group receiving the supplement and decreased on subsequent days of the study. On the third day of the study, the percentages of CD4+CD25+FOXP3+ cells in both groups were similar. In the group receiving EM Bokashi®, statistically significant differences in the percentage of CD4+CD25+FOXP3+ cells were observed on days 2 and 3 compared to the remaining days ((IV)).
The analysis of the percentage of CD4+CD8+ (DP) cells showed an increase on the fourth and fifth day of the study in the supplemented group, and the values were statistically significantly higher than in the control group. In the control group, an increase in this parameter was observed on the last two days of the experiment, and it differed significantly from the remaining days of the study. In the supplemented group, statistically significant differences in the percentage of CD4+CD8+ (DP) cells were observed on days 5, 6, and 7 compared to the remaining days of the study ((V) and ).
Figure 5. Microscopic analysis of a fluorochrome-labelled colostrum sample using a Leica DMi8 fluorescence microscope (Leica, Germany). Measurement parameters: Camera DMC6200-2000000379, digitalization: 8 bit, composite colour capture mode, objective: HC PL FLUOROTAR L 20x / 0.4 DRY, exposure time: 1.711 ms. ↑ (red arrow) – CD4+CD8+ (DP – double-positive) colostrum cells.
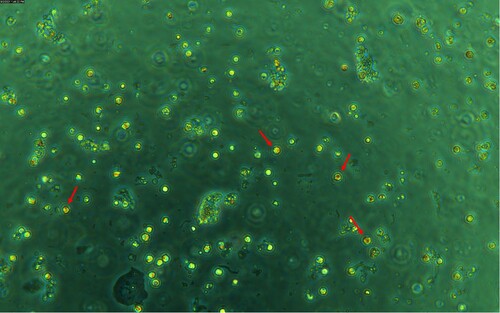
Immune response parameters in piglet sera
The analysis of the percentage CD3+CD4+ cells in the blood of piglets in the control group showed an increase from days 0 to 28 of the study. The percentage of CD3+CD4+ cells in piglets from sows receiving EM Bokashi® was statistically significantly (p < .05) higher on all days of the study than on the corresponding days in the control group. An increase in this parameter was also observed in the period from 0 to 28 days of the study in the blood of piglets from sows receiving the supplement. The Friedman test and post hoc tests showed statistically significant (p < .05) differences in the percentage of CD3+CD4+ cells in the blood of piglets in the experimental group on days 21 and 28 of the study ((I)).
The analysis of the percentage of CD3+CD8+ cells in the blood of piglets showed no statistically significant differences on days 0–14 of the study, but there were statistically significant (p < .05) differences between days 21 and 28. The percentage of CD3+CD8+ cells in the blood of piglets from sows receiving EM Bokashi® was statistically significantly (p < .05) lower on days 21 and 28 than on the corresponding days in the blood of the control piglets. An increase in this parameter was observed in the period from 0 to 28 days of the study in the blood of piglets from sows receiving the supplement ((II)).
The percentage of CD25+ cells in the blood of piglets from sows receiving EM Bokashi® was statistically significantly (p < .05) higher on all days of the study than on the corresponding days in the control group. The highest percentage of CD25+ cells in the blood of piglets from sows receiving the supplement was observed on day 0 of the study. The Friedman test and post hoc tests showed statistically significant (p < .05) differences in the percentage of CD25+ cells in the blood of piglets in the experimental group between day 0 and other days of the study ((III)).
The percentage of CD4+CD25+FOXP3+ in the blood of piglets in the control group increased in the period from 0 to 28 days of the study. The percentage of CD4+CD25+FOXP3+ cells in the blood of piglets from sows receiving EM Bokashi® was statistically significantly (p < .05) higher in the period from day 0 to 21 of the study than on the corresponding days in the blood of piglets in the control group. The percentage of CD4+CD25+FOXP3+ cells in the blood of piglets from sows receiving the supplement was highest on day 28 of the study ((IV)).
The percentage of CD4+CD8+ (DP) cells in the blood of piglets in the control group increased in the period from 0 to 28 days of the study. An almost fourfold increase in this parameter was observed on day 7 compared to day 0. In the group of piglets from sows receiving the supplement, a similar increase in the percentage of CD4+CD8+ (DP) cells was observed between 0 and 28 days of the study. Statistically significant differences in the percentage of CD4+CD8+ (DP) cells compared to day 0 of the study were observed on days 7, 21 and 28. Comparative analysis of the control and experimental groups showed statistically significant differences in the percentage of CD4+CD8+ (DP) cells on days 0, 14 and 21 ((V)).
Composition of sows’ colostrum
The analysis of colostrum composition showed that the percentages of solids, fat, protein, lactose and solids-not-fat in the group supplemented with EM Bokashi® were statistically significantly higher than in the control group. The somatic cell count in the colostrum from both groups was similar. The content of IgG and IgA (mg/mL) was statistically significantly higher in the colostrum of the sows from the group receiving EM Bokashi® compared to the control group. The composition of the sows’ colostrum is presented in .
Table 3. Comparison of composition and immunoglobulin content of colostrum (mean ± SD) in groups of sows receiving a diet without EM (control group – A) and with EMs (experimental group – B).
Discussion
The high immune potential of colostrum determines the resistance of newborn piglets to infection during the pre- and post-weaning period, which significantly influences their condition and productivity in subsequent periods of rearing (Theil et al., Citation2014; Williams, Citation1993; Zhang et al., Citation2018). Modern feeding strategies involving administration of feed supplements with immunomodulatory effects to pregnant sows, in order to enhance the immune potential of the colostrum and milk and increase the effectiveness of passive protection of newborn piglets, are becoming increasingly popular in pig farming (Scharek et al., Citation2005; Stamati et al., Citation2006). One of the feed additives exerting a positive effect on the health and performance parameters of pigs is the multi-strain preparation EM Bokashi®, used in the experiment (Laskowska et al., Citation2019). The literature lacks information on the effect of this preparation on the immune potential of the colostrum and milk of sows and on immunity in piglets. The results of our study clearly demonstrate the effectiveness of EM Bokashi® in improving the immune parameters of colostrum and milk, which translates to better passive protection of piglets against infection.
The presence in colostrum and milk of CD3+/CD4+ T cells (helper lymphocytes) and CD3+/CD8+ T cells (cytotoxic/suppressor lymphocytes) is considered an indicator of the physiological and immune status of the sow and determines the activation of an early immune response in suckling piglets in the case of infection (Binns, Citation1994; Boersma et al., Citation2001; Le Jan, Citation1996; Pomorska-Mol et al., Citation2010). The colostrum and milk of sows whose feed was supplemented with EM Bokashi® had a high percentage of CD3+CD4+ and CD3+CD8+ T cells, which demonstrates the strong effect of the probiotic on the proliferation and differentiation of lymphocytes and its stimulatory effect on cellular immune mechanisms. The results suggest that EM Bokashi®, which contains numerous bacterial and fungal antigens, activates the body’s immune reaction in response to stimulation by these antigens, and the T cells present in the colostrum are a population of functional cells involved in the immune response. Analysis of the results of the present study also indicates that an increase in CD4+ expression on T cells in sows receiving feed supplemented with EM Bokashi® indirectly contributes to activation of cytotoxic CD8+ T cells, which enhances the immune potential of colostrum and milk and determines effective elimination of antigens colonizing the mammary gland. It should be stressed that the presence of T cells in the colostrum of sows is mainly linked to selective colonization of the mammary gland by these cells in response to antigens of intestinal microbes (Field, Citation2005). Administration of the multi-strain preparation EM Bokashi® to sows during colostrogenesis therefore alters the structure of the intestinal microbiome and, by stimulating immune processes in the intestines, modulates the systemic cellular response, which in turn affects the local cellular response of the mammary gland. The increased percentage of CD4 and CD8 lymphocytes in the colostrum of sows receiving EM Bokashi® is indicative of the specific antigenic activity of the microbes contained in the probiotic, owing to which these cells are able to overcome the barrier of the intestinal epithelium of newborn piglets and migrate with the blood to peripheral tissues, inhibiting the development of the inflammatory response to antigens (Nechvatalova et al., Citation2011; Williams, Citation1993). This hypothesis is supported by the high concentration of CD4 cells and low concentration of CD8 cells in the blood of piglets born to sows whose feed was supplemented with EMs, in which antigen presentation processes are dominant, while no inflammatory response involving cytotoxic CD8 cells is observed. Similar observations were made by Michałkiewicz et al. (Citation2003) in a study in humans, in which the use of a multi-strain probiotic based on lactic acid bacteria caused an increase in CD4 expression on lymphocytes, with no effect on CD8 expression. These dependencies indicate that the body’s reaction to a probiotic is limited to a response to antigens of acidophilic bacteria. Similar results were obtained by Scharek-Tedin et al. (Citation2009) and Wang et al. (Citation2009) in a study in pigs in which administration of Enterococcus faecium and Lactobacillus fermentum with the diet resulted only in an increase in the percentage of CD4+CD8− lymphocytes. This effect of probiotic bacteria is clearly linked to the process of differentiation of T cells, which plays an important role in the regulation of immune functions. This effect should also be considered to be linked to a known trait of probiotic bacteria, which partially inhibit mitogen-induced proliferation of polymorphonuclear cells, but underlying this effect is the inhibitory effect of as yet unidentified proteins contained in the microbiome of probiotic preparations.
In a recently published study, Forner et al. (Citation2021) reported that the dominant subset in the population of immunocompetent cells of the colostrum of sows is CD3+CD8+ T cells, which produce cytokines taking part in inflammatory processes (Hlavova et al., Citation2014). In our study as well, CD3+CD8+ T cells were the dominant lymphocyte population in the colostrum and milk of the sows receiving EMs. Our previously published research (Laskowska et al., Citation2019) showed that the colostrum and milk of sows receiving EMs had high concentrations of IFN-γ and TNF-α promoting cellular immune mechanisms and ensuring a functional response. These cytokines play an important role during lactation, taking part in immune defence of the mammary gland by activating phagocytosis in macrophages or stimulating cytotoxic Tc lymphocytes. Therefore the high percentage of CD3+CD8+ cells, on the one hand, may be a response to the production of these cytokines, and on the other hand may be the result of stimulation of general immune mechanisms, primarily phagocytosis by monocytes and macrophages, as an effect of intake of the bacterial antigens contained in the multi-strain preparation EM Bokashi. It can therefore be assumed that one of the immunological effects of the multi-strain preparation EM Bokashi® in pigs is induction of pro-inflammatory signals activating the cytotoxicity of cells taking part in protection against infection, accompanied by modulation of the immune response towards maintenance of the Th1/Th2 balance, which was also indicated by the increased percentage of regulatory cells in the colostrum.
The results of the present study pertaining to the changes in the percentage of T cells with a CD25+ phenotype in the group of pigs receiving feed supplemented with EMs demonstrate activation of a specific immune response to antigens of the microbes contained in the probiotic, expressed as stimulation of Th1 lymphocytes responsible for antigen recognition and presentation. The CD25 molecule is one of the markers of T cell activation (Piriou et al., Citation2003), in which it plays a key role (Inobe & Schwartz, Citation2004). Increased CD25 expression on the surface of T cells in the group of pigs receiving a diet supplemented with EM Bokashi® suggests that the probiotic stimulates the activity of these cells and has a potential immunomodulatory role. In correlation with the high percentage of CD4+CD25+FoxP3+ cells in the piglets from the experimental group, these results indicate that some of these cells perform an immunoregulatory and immunosuppressive function, and that as regulatory cells they can inhibit the inflammatory response caused by excessive intake of the antigens contained in the multi-strain preparation EM Bokashi®.
EM-activated T cells present in the colostrum and milk of sows should effectively prevent mammary gland infections, and ingested with the colostrum by piglets should protect against infections in the first few days of life. This hypothesis is supported by the increased percentage of cells with co-expression of CD4+ and FoxP3+ receptors in the colostrum of sows from the experimental group, which constitute not only a pool of regulatory cells, but probably also memory cells, ultimately influencing the animals’ immune potential and health status. The high percentage of Treg cells may also be due to excessive synthesis of TGF-β (Laskowska et al., Citation2019), which promotes differentiation of activated B and T cells in the population of T regulatory cells.
One of the most important mechanisms of the protective effect of probiotics is regulation of the Th1/Th2 balance and stimulation of the Th1 response (Scharek-Tedin et al., Citation2009; Walsh et al., Citation2008). Regulatory processes of the Th1/Th2 response mainly involve induction of T regulator (Treg) Foxp3+ cells (Kwon et al., Citation2013; Lavasani et al., Citation2010; Ogawa et al., Citation2016; Tankou et al., Citation2018). Multi-strain probiotics have mainly been shown to induce the production of CD4+Foxp3+ Treg cells and increase the suppressor activity of naturally occurring CD4+CD25+ Treg cells, mediated by regulatory dendritic cells (rDC)25 (Kwon et al., Citation2013; Zhao et al., Citation2013; Zhou et al., Citation2015). The significantly higher percentage of CD4+CD25+FoxP3+ T cells and increased CD25+ expression in the experimental group of piglets, in conjunction with the values of other immune parameters, indicate that the EMs used in the diet stimulate a pool of Treg cells activating Th lymphocytes, mainly Th1. The low percentage of these cells in the piglets from the control group suggests a lack of antigen activation or suppressive effect of this subpopulation on helper lymphocytes. Similar findings in humans were reported by Ashraf et al. (Citation2014), who showed that an increase or decrease in the percentage of CD4+CD25+ cells is dependent on the strength of the immune response to foreign antigens ingested with the probiotic. This type of effect of probiotics has been exploited in clinical practice to control the course of certain diseases, e.g. autoimmune diseases (Tankou et al., Citation2018). In the Experimental Autoimmune Encephalomyelitis (EAE) model in animals and humans, oral administration of probiotics resulted in the production of immunoregulatory and anti-inflammatory cytokines, such as TGF-β and IL-10, and an increase in the percentage of Treg cells (Lavasani et al., Citation2010; Tankou et al., Citation2018), which was synonymous with an increase in Foxp3 expression. This effect of probiotics is also confirmed by the results of previous research by Laskowska et al. (Citation2019), which showed that the concentrations of anti-inflammatory IL-10 and pro-inflammatory TNF-α and IL-6 in pigs whose feed was supplemented with EM Bokashi increased with age. The results of the present study pertaining to CD4+CD25+FoxP3+, CD8 and CD4+ expression confirm that oral administration of probiotics in pigs reduces proliferation of cytotoxic T lymphocytes and directs cytokine synthesis to an anti-inflammatory Th2 and regulatory Treg profile. These phenomena are responsible for inhibiting an inflammatory reaction that can lead to enterocyte damage due to the ingestion of the numerous antigens contained in a multi-strain probiotic preparation (Ghosh et al., Citation2004). This type of modulating effect of bacterial antigens at the lymphocyte level helps to maintain the state of tolerance of the Th1/Th2 immune response for the diverse intestinal bacteria additionally enriched with EM Bokashi®, which prevents the development of allergic reactions involving APCs (Sydora et al., Citation2003). The high percentage of CD4+CD25+FoxP3+ Treg cells in the group of pigs receiving EM Bokashi® is undoubtedly indicative of maintenance of immune tolerance at the level of the entire body, which directly translates to the animals’ health. EMs used in pigs as a feed additive are also likely to stimulate dendritic cells (DC) of the intestinal epithelium, thereby enhancing the reactivity of T cells towards transformation into Treg cells. Cells produced in this manner migrate to the mammary gland via the blood vessels. The large pool of these cells present in the colostrum and milk of sows exerts a beneficial effect on piglets (Ogawa et al., Citation2016) by maintaining a balance between the activity of regulatory cells and effector cells, which determines the optimum strength of the body’s specific immune response to potential infection.
One of the distinguishing characteristics of the immune system of pigs is the presence of double-positive (DP) lymphocytes with simultaneous expression of CD4+ and CD8+ molecules (Löving & Magnusson, Citation2002; Lunney & Pescovitz, Citation1987). The currently prevailing opinion is that these cells are associated with immune memory, as indicated by the gradual increase in their quantity, dependent on the age of the animal, phenotypic expression of memory cells, and the body’s capacity for a repeated response to antigens (Zuckermann et al., Citation1998; Zuckermann & Husmann, Citation1996). Research by many authors indicates that DP cells originate in response to antigen stimulation of CD4+CD8− lymphocytes, and that their proliferation results from the provoked response to the antigen (Zuckermann et al., Citation1998; Zuckermann & Husmann, Citation1996). In the present study, changes in the percentage of DP lymphocytes in the colostrum and milk of sows were similar in the experimental group and the control group, which indicates stabilization of the immune response in the mammary gland. These results also suggest that there was no ongoing disease process in the mammary gland, in which these cells function only as immune memory cells. The temporary increase in the percentage of DP cells in the milk of sows receiving EMs on days 4 and 5 of lactation may have been due to strong antigen stimulation of DP cells by environmental antigens.
The percentage of CD4+CD8+ lymphocytes in the peripheral blood of pigs depends on age, ranging from 1% in month-old piglets to about 60% at the age of three years (Forner et al., Citation2021; Zuckermann & Husmann, Citation1996). This is confirmed by the results of our study, in which the percentage of DP cells ranged from 0.6% to 0.73% in newborn piglets and increased during the pigs’ development, reaching 3.09-3.20% of circulating T cells at 28 days of age. These changes are believed to be associated with progressive antigen stimulation of the lymphoid organs, particularly in the case of the use of a multi-strain EM preparation as a feed additive (Scharek et al., Citation2007). It should be stressed that throughout the experiment the percentage of DP cells was higher in the experimental group than in the control group, although statistically significant differences between the groups were shown only between 14 and 21 days of age. These results clearly confirm the stimulatory effect of EMs on the percentage of DP cells in the experimental group and indicate active acquisition of immune memory.
One of the most important factors determining the survival of newborn piglets is the provision of high-quality colostrum immediately after birth, which ensures normal physiological development and protection against infection in the first few days of life (Choct, Citation2009; Le Dividich et al., Citation2005; Zhang et al., Citation2018). The quality of colostrum and milk is largely influenced by the diet of sows during pregnancy and lactation (Farmer & Quesnel, Citation2009). The available literature shows that better nutrient utilization by the sow during these periods is reflected in the high quality of the colostrum and milk, which indirectly affects the growth rate and health of piglets (Nechvatalova et al., Citation2011; Zhang et al., Citation2018). Ahasan et al. (Citation2015) showed that supplementation of the diets of sows with probiotics based on Bacillus, Lactobacillus and Streptococcus strains improves colostrum quality and the quality and quantity of milk and results in larger, more viable litters and higher piglet birth weight. The results of our research indicate that feeding sows a diet supplemented with EM Bokashi® improves the quality and composition of milk, mainly by increasing the concentrations of fat, protein, and sugars, including lactose. Similar results were obtained by Alexopoulos et al. (Citation2004), who reported demonstrated significantly higher content of fat and protein in the milk of sows receiving Bacillus species-based probiotics in comparison to controls. It should be added that the fat contained in colostrum and milk can to some extent be used by piglets immediately after birth as an additional energy pool, and may also increase metabolism, helping piglets to maintain a constant body temperature after birth (Craig et al., Citation2019; Schollenberger et al., Citation1986). In addition, some fatty acids, including polyunsaturated fatty acids (PUFA), play an important role in brain development and function (Dullemeijer et al., Citation2008). High fat concentrations in the colostrum of sows receiving a probiotic supplement may therefore affect the survival rate of piglets in the post-natal period. Contrasting results were reported by Betancur et al. (Citation2021), who found that Lactobacillus plantarum CAM6 administered to sows as a feed additive reduced the fat concentration in their milk. The most likely cause of this effect was the presence in L. plantarum Lp9 of genes encoding bile salt hydrolases (BSH), which reduce cholesterol concentrations through enzymatic deconjugation of bile acid salts and reduce absorption of cholesterol and saturated fatty acids (Begley et al., Citation2006). The use of EM Bokashi®, containing a variety of microbes, in the diet of sows, should activate different mechanisms of action, increasing the body’s metabolic activity, which determines higher concentrations of compounds such as proteins and fats in the colostrum and milk. For newborn piglets, born with physiologically low reserves of energy essential for maintaining appropriate body temperature, vital activity, and development, it is also important to supply energy in the form of sugars (Declerck et al., Citation2015). The higher lactose concentrations noted in the group of sows receiving feed supplemented with EMs in comparison with controls is evidence of the sows’ better utilization of energy from feed and capacity to store it. This can also be explained by the symbiotic relationship between the microbes contained in the probiotic and those comprising the animal’s own intestinal microbiome. This interaction promotes microbial homeostasis in the intestines, which indirectly improves feed digestibility and increases absorption of nutrients, such as polysaccharides, proteins, and lipids, enhancing glycogenesis in sows (Dowarah et al., Citation2017). Stabilization of the intestinal microbiome by probiotics not only influences metabolic processes, but also stimulates digestion and intestinal absorption. This leads to an increase in the concentration of certain nutrients in milk (Betancur et al., Citation2021; Scharek-Tedin et al., Citation2015), including minerals and solids-non-fat (SNF), which was shown in the group of sows receiving EMs.
Apart from its nutritional value, colostrum is an important source of immunoglobulins providing passive protection of suckling piglets. Class IgG and IgA antibodies present in colostrum take part in systemic and local defence responses in piglets’ first few days of life until activation of the GALT (Bland et al., Citation2003; Hurley & Theil, Citation2011; Porter & Allen, Citation1972; Quesnel, Citation2011). The results of the experiment indicate that the use of EMs in the diet of sows enhances the immune potential of colostrum, which is manifested by an increase in the concentration of class IgG and IgA antibodies. Similar observations are reported by Chattha et al. (Citation2013), Inatomi et al. (Citation2017), and Laskowska et al. (Citation2019), who also showed increases in the IgG and/or IgA concentration in the milk of cows receiving feed supplemented with probiotics. The authors cited are in agreement that the increased concentration of antibodies in the milk was mainly the effect of the probiotic strains on local synthesis of these immunoglobulins. Similar results were described in humans by Prescott et al. (Citation2008), who showed that the use of Lactobacillus rhamnosus and Bifidobacterium lactis as a diet supplement for four weeks before parturition resulted in a significant increase in TGF-β and IgA concentrations in the milk of women. It is likely that the increase in the immune potential of the colostrum and milk observed in our study is linked to the activating influence of EM Bokashi® on the subpopulation of B cells and promotion of the Th2 immune profile, which also determines the penetration of immunoglobulin into body fluids, including colostrum and milk. The high concentrations of IgG and IgA in the colostrum and milk of sows in the experimental group is indicative of the protective role of mammary gland secretions for piglets in the first few days of life (Scharek et al., Citation2005). Proper ingestion of colostrum with high immune potential provides piglets with more effective passive immunity through agglutination of antigens, neutralization of viruses and bacterial toxins, and prevention of bacterial adhesion to epithelial cells (Bandrick et al., Citation2008; Hlavova et al., Citation2014). Irrespective of protection of piglets, active substances present in the mammary gland secretions of sows also protect the mammary gland itself against infection by binding antigens and taking part in immune processes (Zhang et al., Citation2018).
Conclusions
To conclude, the use of the multi-strain preparation EM Bokashi® as a feed additive for sows during the colostrogenesis period has a beneficial effect on colostrum quality by increasing its immune potential. The high expression of CD4 and CD8 in the cells of the colostrum and milk of sows and in the blood of piglets indicate the promotion of cellular immune mechanisms, expressed as an increase in the population of antigen-presenting Th1 lymphocytes in the colostrum and milk and in the blood, which contributes to more effective elimination of microbes in the first period of the piglets’ life. The simultaneous high percentage of CD4+CD25+FoxP3+ Treg cells is evidence of the immunoregulatory effect of the EM Bokashi® on Th1 cells and determines the maintenance of the Th1/Th2 balance. A full explanation of the effect of the multi-strain probiotic preparation EM Bokashi® on mechanisms regulating the immune response at the cellular level in the mammary gland of sows requires further study.
Disclosure statement
No potential conflict of interest was reported by the author(s).
References
- Ahasan, A. S. M. L., Agazzi, A., Invernizzi, G., Bontempo, V., & Savoini, G. (2015). The beneficial role of probiotics in monogastric animal nutrition and health. Journal of Dairy, Veterinary & Animal Research, 2(4), 116–132. https://doi.org/10.15406/jdvar.2015.02.00041
- Ahmed, S. T., Hoon, J., Mun, H. S., & Yang, C. J. (2014). Evaluation of Lactobacillus and Bacillus-based probiotics as alternatives to antibiotics in enteric microbial challenged weaned piglets. African Journal of Microbiology Research, 8(1), 96–104. https://doi.org/10.5897/AJMR2013.6355
- Alexopoulos, C., Georgoulakis, I. E., Tzivara, A., Kritas, S. K., Siochu, A., & Kyriakis, S. C. (2004). Field evaluation of the efficacy of a probiotic containing Bacillus licheniformis and Bacillus subtilis spores, on the health status and performance of sows and their litters. Journal of Animal Physiology and Animal Nutrition, 88(11-12), 381–392. https://doi.org/10.1111/j.1439-0396.2004.00492.x
- Alonso, L., Fontecha, J., & Cuesta, P. (2016). Combined effect of Lactobacillus acidophilus and β-cyclodextrin on serum cholesterol in pigs. British Journal of Nutrition, 115(1), 1–5. https://doi.org/10.1017/S0007114515003736
- Ashraf, R., Vasiljevic, T., Smith, S. C., & Donkor, O. N. (2014). Effect of cell-surface components and metabolites of lactic acid bacteria and probiotic organisms on cytokine production and induction of CD25 expression in human peripheral mononuclear cells. Journal of Dairy Science, 97(5), 2542–2558. https://doi.org/10.3168/jds.2013-7459
- Bandrick, M., Pieters, M., Pijoan, C., & Molitor, T. W. (2008). Passive transfer of maternal Mycoplasma hyopneumoniae-specific cellular immunity to piglets. Clinical and Vaccine Immunology, 15(3), 540–543. https://doi.org/10.1128/CVI.00466-07
- Begley, M., Hill, C., & Gahan, C. G. (2006). Bile salt hydrolase activity in probiotics. Applied and Environmental Microbiology, 72(3), 1729–1738. https://doi.org/10.1128/AEM.72.3.1729-1738.2006
- Betancur, C., Martínez, Y., Tellez-Isaias, G., Castillo, R., & Ding, X. (2021). Effect of oral administration with Lactobacillus plantarum CAM6 strain on sows during gestation-lactation and the derived impact on their progeny performance. Mediators of Inflammation, 6615960, 1–8. https://doi.org/10.1155/2021/6615960
- Binns, R. M. (1994). The null/gamma delta TCR+ T cell family in the pig. Veterinary Immunology and Immunopathology, 43(1-3), 69–77. https://doi.org/10.1016/0165-2427(94)90122-8
- Bland, I. M., Rooke, J. A., Bland, V. C., Sinclair, A. G., & Edwards, S. A. (2003). Appearance of immunoglobulin G in the plasma of piglets following intake of colostrum, with or without a delay in sucking. Animal Science Journal, 77(2), 277–286. https://doi.org/10.1017/S1357729800059014
- Blavi, L., Solà-Oriol, D., Llonch, P., López-Vergé, S., Martín-Orúe, S. M., & Pérez, J. F. (2021). Management and feeding strategies in early life to increase piglet performance and welfare around weaning: A review. Animals, 11(2), 302. https://doi.org/10.3390/ani11020302
- Boersma, W. J., Zwart, R. J., Sinkora, J., Rehakova, Z., Haverson, K., & Bianchi, A. T. (2001). Summary of workshop findings for porcine B-cell markers. Veterinary Immunology and Immunopathology, 80(1-2), 63–78. https://doi.org/10.1016/S0165-2427(01)00279-3
- Cai, C. J., Cai, P. P., Hou, C. L., Zeng, X. F., & Qiao, S. Y. (2014). Administration of Lactobacillus fermentum I5007 to young piglets improved their health and growth. Journal of Animal and Feed Sciences, 23(3), 222–227. https://doi.org/10.22358/jafs/65684/2014
- Charette, R., Bigras-Poulin, M., & Martineau, G.-P. (1996). Body condition evaluation in sows. Livstock Production Science, 46(2), 107–115. https://doi.org/10.1016/0301-6226(96)00022-X
- Chattha, K. S., Vlasova, A. N., Kandasamy, S., Esseili, M. A., Siegismund, C., Rajashekara, G., & Saif, L. J. (2013). Probiotics and colostrum/milk differentially affect neonatal humoral immune responses to oral rotavirus vaccine. Vaccine, 31(15), 1916–1923. https://doi.org/10.1016/j.vaccine.2013.02.020
- Chaucheyras-Durand, F., & Durand, H. (2010). Probiotics in animal nutrition and health. Beneficial Microbes, 1(1), 3–9. https://doi.org/10.3920/BM2008.1002
- Chen, Y. J., Min, B. J., Cho, J. H., Kwon, O. S., Son, K. S., Kim, H. J., & Kim, I. H. (2006). Effects of dietary Bacillus-based probiotic on growth performance, nutrients digestibility, blood characteristics and fecal noxious gas content in finishing pigs. Asian-Australasian Journal of Animal Sciences, 19(4), 587–592. https://doi.org/10.5713/ajas.2006.587
- Chen, Y. J., Son, K. S., Min, B. J., Cho, J. H., Kwon, O. S., & Kim, I. H. (2005). Effects of dietary probiotic on growth performance, nutrients digestibility, blood characteristics and fecal noxious gas content in growing pigs. Asian-Australasian Journal of Animal Sciences, 18(10), 1464–1468. https://doi.org/10.5713/ajas.2005.1464
- Cho, J. H., Zhao, P. Y., & Kim, I. H. (2011). Probiotics as a dietary additive for pigs: A review. Journal of Animal and Veterinary Advances, 10(16), 2127–2134. https://doi.org/10.3923/javaa.2011.2127.2134
- Choct, M. (2009). Managing gut health through nutrition. British Poultry Science, 50(1), 9–15. https://doi.org/10.1080/00071660802538632
- Choi, J. Y., Shinde, P. L., Ingale, S. L., Kim, J. S., Kim, Y. W., Kim, K. H., Kwon, I. K., & Chae, B. J. (2011). Evaluation of multimicrobe probiotics prepared by submerged liquid or solid substrate fermentation and antibiotics in weaning pigs. Livestock Science, 138(1-3), 144–151. https://doi.org/10.1016/j.livsci.2010.12.015
- Close, W. H. (2000). Producing pigs without antibiotic growth promoters. Advances in Pork Production, 11, 47–56.
- Craig, J. R., Dunshea, F. R., Cottrell, J. J., Wijesiriwardana, U. A., & Pluske, J. R. (2019). Primiparous and multiparous sows have largely similar colostrum and milk composition profiles throughout lactation. Animals (Basel), 9(2), 35. https://doi.org/10.3390/ani9020035
- Declerck, I., Dewulf, J., Piepers, S., Decaluwé, R., & Maes, D. (2015). Sow and litter factors influencing colostrum yield and nutritional composition. Journal of Animal Science, 93(3), 1309–1317. https://doi.org/10.2527/jas.2014-8282
- Declerck, I., Dewulf, J., Sarrazin, S., & Maes, D. (2016). Long-term effects of colostrum intake in piglet mortality and performance. Journal of Animal Science, 94(4), 1633–1643. https://doi.org/10.2527/jas.2015-9564
- Douglas, S. L., Edwards, S. A., & Kyriazakis, I. (2014). Management strategies to improve the performance of low birth weight pigs to weaning and their long-term consequences. Journal of Animal Science, 92(5), 2280–2288. https://doi.org/10.2527/jas.2013-7388
- Dowarah, R., Verma, A. K., & Agarwal, N. (2017). The use of Lactobacillus as an alternative of antibiotic growth promoters in pigs: A review. Animal Nutrition, 3(1), 1–6. https://doi.org/10.1016/j.aninu.2016.11.002
- Dullemeijer, C., Zock, P., Coronel, R., Den Ruijter, H., Katan, M., Brummer, R., & Brouwer, I. (2008). Differences in fatty acid composition between cerebral brain lobes in juvenile pigs after fish oil feeding. British Journal of Nutrition, 100(4), 794–800. https://doi.org/10.1017/S0007114508943737
- Fairbrother, J. M., Nadeau, E., & Gyles, C. L. (2005). Escherichia coli in postweaning diarrhea in pigs: An update on bacterial types, pathogenesis, and prevention strategies. Animal Health Research Reviews, 6(1), 17–39. https://doi.org/10.1079/AHR2005105
- Farmer, C., & Quesnel, H. (2009). Nutritional, hormonal, and environmental effects on colostrum in sows. Journal of Animal Science, 87(suppl_13), 56–64. https://doi.org/10.2527/jas.2008-1203
- Field, C. J. (2005). The immunological components of human milk and their effect on immune development in infants. The Journal of Nutrition, 135(1), 1–4. https://doi.org/10.1093/jn/135.1.1
- Forner, R., Bombassaro, G., Bellaver, F. V., Maciag, S., Fonseca, F. N., Gava, D., Lopes, L., Marques, M. G., & Bastos, A. P. (2021). Distribution difference of colostrum-derived B and T cells subsets in gilts and sows. PloS one, 16(5), e0249366. https://doi.org/10.1371/journal.pone.0249366
- Gaggia, F., Mattarelli, P., & Biavati, B. (2010). Probiotics and prebiotics in animal feeding for safe food production. The International Journal of Food Microbiology, 141, S15–S28. https://doi.org/10.1016/j.ijfoodmicro.2010.02.031
- Gaugler, H. R., Buchanan, D. S., Hintz, R. L., & Johnson, R. K. (1983). Sow productivity comparisons for four breeds of swine: Purebred and crossbred litters. Journal of Animal Science, 59(4), 941–947. https://doi.org/10.2527/jas1984.594941x
- Ghosh, S., van Heel, D., & Playford, R. J. (2004). Probiotics in inflammatory bowel disease: Is it all gut flora modulation? Gut, 53(5), 620–622. https://doi.org/10.1136/gut.2003.034249
- Hanekon, D., Prinsloo, J. F., & Schoonbee, H. J. (2000). A comparison of the effect of anolyte and Effective Microorganisms (Kyusei EMTM) on the faecal bacterial loads in the water and on fish produced in pig-cum-fish integrated production units. Water SA. http://www.emturkey.com.tr/eskisite/TR/dosya/1-126/h/02-a-comparison-of-the-effect-ofanolyte-and-effective-.pdf
- Hasan, S., Junnikkala, S., Peltoniemi, O., Paulin, L., Lyyski, A., Vuorenmaa, J., & Oliviero, C. (2018). Dietary supplementation with yeast hydrolysate in pregnancy influences colostrum yield and gut microbiota of sows and piglets after birth. PloS One, 13(5), e0197586. https://doi.org/10.1371/journal.pone.0197586
- Higa, T., & Parr, J. (1994). Beneficial and effective microorganisms for a sustainable agriculture and environment. International Nature Farming Center, Atami, Japan (p. 16). Asia-Pacific Natural Agriculture Network-APNAN (1995). EM application manual. (first eds.) http://www.agriton.nl/apnanman.html.
- Hlavova, K., Stepanova, H., & Faldyna, M. (2014). The phenotype and activation status of T and NK cells in porcine colostrum suggest these are central/effector memory cells. Veterinary Journal, 202(3), 477–482. https://doi.org/10.1016/j.tvjl.2014.09.008
- Holm, B., Bakken, M., Klemetsdal, G., & Vangen, O. (2004). Genetic correlation between reproduction and production traits in swine. Journal of Animal Science, 82(12), 3458–3464. https://doi.org/10.2527/2004.82123458x
- Hurley, W. L., & Theil, P. K. (2011). Perspectives on immunoglobulins in colostrum and milk. Nutrients, 3(4), 442–474. https://doi.org/10.3390/nu3040442
- Inatomi, T., Amatatsu, M., Romero-Pérez, G. A., Inoue, R., & Tsukahara, T. (2017). Dietary probiotic compound improves reproductive performance of porcine epidemic diarrhoea virus -infected sows reared in a Japanese commercial swine farm under vaccine control condition. Frontiers in Immunology, 8, 1877. https://doi.org/10.3389/fimmu.2017.01877
- Inobe, M., & Schwartz, R. H. (2004). CTLA-4 engagement acts as a brake on CD41 T cell proliferation and cytokine production but is not required for tuning T cell reactivity in adaptive tolerance. Journal of Immunology, 173(12), 7239–7248. https://doi.org/10.4049/jimmunol.173.12.7239
- Jang, Y. D., Kang, K. W., Piao, L. G., Jeong, T. S., Auclair, E., Jonvel, S., D'Inca, R., & Kim, Y. Y. (2013). Effects of live yeast supplementation to gestation and lactation diets on reproductive performance, immunological parameters and milk composition in sows. Livestock Science, 152(2-3), 167–173. https://doi.org/10.1016/j.livsci.2012.12.022
- Käser, T., Gerner, W., Hammer, S. E., Patzl, M., & Saalmüller, A. (2008). Detection of Foxp3 protein expression in porcine T lymphocytes. Veterinary Immunology and Immunopathology, 125(1-2), 92–101. https://doi.org/10.1016/j.vetimm.2008.05.007
- Koketsu, Y. (2000). Productivity characteristics of high-performing swine farms. Journal of the American Veterinary Medical Association, 215(3), 376–379. https://doi.org/10.2460/javma.2000.216.376
- Koketsu, Y., Tani, S., & Iida, R. (2017). Factors for improving reproductive performance of sows and herd productivity in commercial breeding herds. Porcine Health Management, 3(1), 1. https://doi.org/10.1186/s40813-016-0049-7
- Konoplya, E. F., & Higa, T. (2000). EM application in animal husbandry – Poultry farming and its action mechanisms. Paper presented at the International Conference on EM Technology and Nature Farming, October 2000, Pyongyang, DPR Korea.
- Kumprechtova, D., Zobac, P., & Kumprecht, I. (2000). The effect of Sacchromyces cervisiae SC47 on chicken broiler performance and nitrogen output. Czech Journal of Animal Science, 45(4), 169–177.
- Kwon, H. K., Kim, G. C., Kim, Y., Hwang, W., Jash, A., Sahoo, A., Kim, J. E., Nam, J. H., & Im, S. H. (2013). Amelioration of experimental autoimmune encephalomyelitis by probiotic mixture is mediated by a shift in T helper cell immune response. Clinical Immunology, 146(3), 217–227. https://doi.org/10.1016/j.clim.2013.01.001
- Laskowska, E., Jarosz, Ł, & Grądzki, Z. (2019). Effect of multi-microbial probiotic formulation Bokashi on pro- and anti-inflammatory cytokines profile in the serum, colostrum and milk of sows, and in a culture of polymorphonuclear cells isolated from colostrum. Probiotics and Antimicrobial Protein, 11(1), 220–232. https://doi.org/10.1007/s12602-017-9380-9
- Lavasani, S., Dzhambazov, B., Nouri, M., Fak, F., Buske, S., Molin, G., Thorlacius, H., Alenfall, J., Jeppsson, B., & Westrom, B. (2010). A novel probiotic mixture exerts a therapeutic effect on experimental autoimmune encephalomyelitis mediated by IL-10 producing regulatory T cells. PLoS One, 5(2), e9009. https://doi.org/10.1371/journal.pone.0009009
- Le Dividich, J., Rooke, J. A., & Herpin, P. (2005). Nutritional and immunological importance of colostrum for the new-born pig. Journal of Agricultural Science -Cambridge, 143(6), 469–485. https://doi.org/10.1017/S0021859605005642
- Le Jan, C. (1994). A study by flow cytometry of lymphocytes in sow colostrum. Research in Veterinary Science, 57(3), 300–304. https://doi.org/10.1016/0034-5288(94)90121-X
- Le Jan, C. (1996). Cellular components of mammary secretions and neonatal immunity: A review. Veterinary Research, 27(4-5), 403–417.
- Liu, W. C., Yun, H. M., Pi, S. H., & Kim, I. H. (2017). Supplementing lactation diets with herbal extract mixture during summer improves the performance of sows and nursing piglets. Annals of Animal Science, 17(3), 835–847. https://doi.org/10.1515/aoas-2016-0084
- Löving, M., & Magnusson, U. (2002). Sows intramammarily inoculated with Escherichia coli at parturition. II efects on the densities of MHC class II+, CD4+ and CD8+ cells in the mammary gland. Veterinary Immunology and Immunopathology, 90(1-2), 45–54. https://doi.org/10.1016/S0165-2427(02)00203-9
- Lunney, J. K., & Pescovitz, M. D. (1987). Phenotypic and functional characterization of pig lymphocyte populations. Veterinary Immunology and Immunopathology, 17(1-4), 135–144. https://doi.org/10.1016/0165-2427(87)90134-6
- Michałkiewicz, J., Krotkiewski, M., Gackowska, L., Wyszomirska-Gołda, M., Helmin-Basa, A., Dzierżanowska, D., & Madaliński, K. (2003). Immunomodulatory effects of lactic acid bacteria on human peripheral blood mononuclear cells. Microbial Ecology in Health and Disease, 15(4), 185–192. https://doi.org/10.1080/08910600310022729
- Mori, K., Ito, T., Miyamoto, H., Ozawa, M., Wada, S., Kumagai, Y., Matsumoto, J., Naito, R., Nakamura, S., Kodama, H., & Kurihara, Y. (2011). Oral administration of multispecies microbial supplements to sows influences the composition of gut microbiota and faecal organic acids in their post-weaned piglets. The Journal of Bioscience and Bioengineering, 112(2), 145–150. https://doi.org/10.1016/j.jbiosc.2011.04.009
- Mroz, Z. (2001). Some developments on Dutch nutritional approaches to protect piglets against post-weaning gastrointestinal disorders in the absence of in-feed antibiotics. Journal of Animal and Feed Sciences, 10(Suppl. 1), 153–167. https://doi.org/10.22358/jafs/70018/2001
- Nechvatalova, K., Kudlackova, H., Leva, L., Babickova, K., & Faldyna, M. (2011). Transfer of humoral and cell-mediated immunity via colostrum in pigs. Veterinary Immunology and Immunopathology, 142(1-2), 95–100. https://doi.org/10.1016/j.vetimm.2011.03.022
- NRC. (1998). Nutrient requirements of swine (10th rev. ed.). Natl. Acad. Press.
- Ogawa, S., Okutani, M., Tsukahara, T., Nakanishi, N., Kato, Y., Fukuta, K., Romero-Perez, G. A., Ushida, K., & Inoue, R. (2016). Comparison of gene expression profiles of T cells in porcine colostrum and peripheral blood. American Journal of Veterinary Research, 77(9), 961–968. https://doi.org/10.2460/ajvr.77.9.961
- Piriou, L., Chevallier, S., Hutet, E., Charley, B., Le Potier, M. F., & Albina, E. (2003). Humoral and cell-mediated immune responses of d/d histocompatible pigs against classical swine fever (CSF) virus. Veterinary Research, 34(4), 389–404. https://doi.org/10.1051/vetres:2003013
- Pomorska-Mol, M., Markowska-Daniel, I., & Bednarek, D. (2010). Flow cytometric analysis of leukocytes in porcine mammary secretions. Bulletin Of The Veterinary Institute In Pulawy, 54(2), 188–192.
- Porter, P., & Allen, W. D. (1972). Classes of immunoglobulins related to immunity in the pig. Journal of the American Veterinary Medical Association, 160(4), 511–518.
- Prescott, S. L., Wickens, K., Westcott, L., Jung, W., Currie, H., Black, P. N., Stanley, T. V., Mitchell, E. A., Fitzharris, P., Siebers, R., Wu, L., & Crane, J. (2008). Supplementation with Lactobacillus rhamnosus or Bifidobacterium lactis probiotics in pregnancy increases cord blood interferon-gamma and breast milk transforming growth factor-beta and immunoglobin A detection. Clinical and Experimental Allergy, 38(10), 1606–1614. https://doi.org/10.1111/j.1365-2222.2008.03061.x
- Quesnel, H. (2011). Colostrum production by sows: Variability of colostrum yield and immunoglobulin G concentrations. Animal, 5(10), 1546–1553. https://doi.org/10.1017/S175173111100070X
- Rashid, M. T., & West, J. (2007). Dairy waste water treatment with effective microorganisms and duckweed for pollutants and pathogen control. In M. K. Zaidi (Ed.), Wastewater reuse-risk assessment, decision-making and environmental security (pp. 93–102). Springler Link.
- Rhouma, M., Fairbrother, J. M., Beaudry, F., & Letellier, A. (2017). Post weaning diarrhea in pigs: Risk factors and non-colistin-based control strategies. Acta Veterinaria Scandinavica, 59(1), 31. https://doi.org/10.1186/s13028-017-0299-7
- Rolfe, R. D. (2000). The role of probiotic cultures in the control of gastrointestinal health. The Journal of Nutrition, 130(2), 396S–402S. https://doi.org/10.1093/jn/130.2.396S
- Sanders, M. E., & Huis in't Veld, J. (1999). Bringing a probiotic-containing functional food to the market: Microbiological, product, regulatory and labeling issues. Antonie Van Leeuwenhoek, 76(1/4), 293–315. https://doi.org/10.1023/A:1002029204834
- Scharek, L., Altherr, B. J., Tölke, C., & Schmidt, M. F. (2007). Influence of the probiotic Bacillus cereus var. toyoi on the intestinal immunity of piglets. Veterinary Immunology and Immunopathology, 120(3-4), 136–147. https://doi.org/10.1016/j.vetimm.2007.07.015
- Scharek, L., Guth, J., Reiter, K., Weyrauch, K. D., Tara, D., Schwerk, P., Schierack, P., Schmidt, M. F., Wieler, L. H., & Tedin, K. (2005). Influence of a probiotic Enterococcus faecium strain on development of the immune system of sows and piglets. Veterinary Immunology and Immunopathology, 105(1-2), 151–161. https://doi.org/10.1016/j.vetimm.2004.12.022
- Scharek-Tedin, L., Filter, M., Taras, D., Wrede, P., & Schmidt, M. F. G. (2009). Influence of an Enterococcus faecium probiotic on the development of Peyer's patches B cells in piglets. Archives of Animal Nutrition, 63(5), 343–355. https://doi.org/10.1080/17450390903052771
- Scharek-Tedin, L., Kreuzer-Redmer, S., Twardziok, S. O., Siepert, B., Klopfleisch, R., Tedin, K., Zentek, J., & Pieper, R. (2015). Probiotic treatment decreases the number of CD14-expressing cells in porcine milk which correlates with several intestinal immune parameters in the piglets. Frontiers in Immunology, 6, 108. https://doi.org/10.3389/fimmu.2015.00108
- Schollenberger, A., Degorski, A., Frymus, T., & Schollenberger, A. (1986). Cells of sow mammary secretions.1. Morphology and differential counts during lactation. Journal of Veterinary Medicine Series A, 33(1-10), 31–38. https://doi.org/10.1111/j.1439-0442.1986.tb00502.x
- Schultz, M., Göttl, C., Young, R. J., Iwen, P., & Vanderhoof, J. A. (2004). Administration of oral probiotic bacteria to pregnant women causes temporary infantile colonization. The Journal of Pediatric Gastroenterology and Nutrition, 38(3), 293–297. https://doi.org/10.1097/00005176-200403000-00012
- Stamati, S., Alexopoulos, C., Siochu, A., Saoulidis, K., & Kyriakis, S. C. (2006). Probiosis in sows by administration of bacillus toyoi spores during late pregnancy and lactation: Effect on their health status/performance and on litter characteristics. The International Journal of Probiotics & Prebiotics, 1(1), 33–40.
- Sydora, B. C., Tavernini, M. M., Wessler, A., Jewell, L. D., & Fedorak, R. N. (2003). Lack of interleukin-10 leads to intestinal inflammation, independent of the time at which luminal microbial colonization occurs. Inflammatory Bowel Diseases, 9(2), 87–97. https://doi.org/10.1097/00054725-200303000-00002
- Szymanski, N., & Patterson, R. A. (2003). Effective microorganisms (EM) and wastewater systems in future directions for on-site systems: Best management practice. In R. A. Patterson, & M. J. Jones (Eds.), Proceedings of on-site conference (pp. 347–354). University of New England, Lanfax Laboratories Armidale.
- Tankou, S. K., Regev, K., Healy, B. C., Cox, L. M., Tjon, E., Kivisakk, P., Vanande, I. P., Cook, S., Gandhi, R., & Glanz, B. (2018). Investigation of probiotics in multiple sclerosis. Multiple Sclerosis Journal, 24(1), 58–63. https://doi.org/10.1177/1352458517737390
- Theil, P. K., Lauridsen, C., & Quesnel, H. (2014). Neonatal piglet survival: Impact of sow nutrition around parturition on fetal glycogen deposition and production and composition of colostrum and transient milk. Animal, 8(7), 1021–1030. https://doi.org/10.1017/S1751731114000950
- Tokach, M. D., Goodband, B. D., & O'Quinn, T. G. (2016). Performance-enhancing technologies in swine production. Animal Frontiers, 6(4), 15–21. https://doi.org/10.2527/af.2016-0039
- Turner, J. L., Dritz, S. S., & Minton, J. E. (2001). Review: Alternatives to conventional antimicrobials in swine diets. The Professional Animal Scientist, 17(4), 217–226. https://doi.org/10.15232/S1080-7446(15)31633-8
- Walsh, M. C., Gardiner, G. E., Hart, O. M., Lawlor, P. G., Daly, M., Lynch, B., Richert, B. T., Radcliffe, S., Giblin, L., Hill, C., Fitzgerald, G. F., Stanton, C., & Ross, P. (2008). Predominance of a bacteriocin-producing Lactobacillus salivarius component of a five-strain probiotic in the porcine ileum and effects on host immune phenotype. FEMS Microbiology Ecology, 64(2), 317–327. https://doi.org/10.1111/j.1574-6941.2008.00454.x
- Wang, A. N., Yi, X. W., Yu, H. F., Dong, B., & Qiao, S. Y. (2009). Free radical scavenging activity of Lactobacillus fermentum in vitro and its antioxidative effect on growing-finishing pigs. Journal of Applied Microbiology, 107(4), 1140–1148. https://doi.org/10.1111/j.1365-2672.2009.04294.x
- Wang, J., Ji, G. F., Hou, C. L., Wang, S. X., Zhang, D. Y., Liu, H., Shan, D. C., & Wang, Y. M. (2014). Effects of Lactobacillus johnsonii XS4 supplementation on reproductive performance gut environment and blood biochemical and immunological index in lactating sows. Livestock Science, 164, 96–101. https://doi.org/10.1016/j.livsci.2014.03.008
- Wang, S. P., Yin, Y. L., Qian, Y., Li, L. L., Li, F. N., Tan, B. E., Tang, X. S., & Huang, R. L. (2011). Effects of folic acid on the performance of suckling piglets and sows during lactation. Journal of the Science of Food and Agriculture, 91(13), 2371–2377. https://doi.org/10.1002/jsfa.4469
- Warda, A., Rekiel, A., Blicharski, T., Batorska, M., Sońta, M., & Więcek, J. (2021). The effect of the size of the litter in which the sow was born on her lifetime productivity. Animals, 11(6), 1525. https://doi.org/10.3390/ani11061525
- Wen, K., Bui, T., Li, G., Liu, F., Li, Y., Kocher, J., & Yuan, L. (2012). Characterization of immune modulating functions of γδ T cell subsets in a gnotobiotic pig model of human rotavirus infection. Comparative Immunology, Microbiology and Infectious Diseases, 35(4), 289–301. https://doi.org/10.1016/j.cimid.2012.01.010
- Williams, P. P. (1993). Immunomodulating effects of intestinal absorbed maternal colostral leukocytes by neonatal pigs. Canadian Journal of Veterinary Research, 57(1), 1–8.
- Zhang, S., Chen, F., Zhang, Y., Lv, Y., Heng, J., Min, T., Li, L., & Guan, W. (2018). Recent progress of porcine milk components and mammary gland function. Journal of Animal Science and Biotechnology, 9(1), 77. https://doi.org/10.1186/s40104-018-0291-8
- Zhao, H. M., Huang, X. Y., Zuo, Z. Q., Pan, Q. H., Ao, M. Y., Zhou, F., Liu, H. N., Liu, Z. Y., & Liu, D. Y. (2013). Probiotics increase T regulatory cells and reduce severity of experimental colitis in mice. World Journal of Gastroenterology, 19(5), 742–749. https://doi.org/10.3748/wjg.v19.i5.742
- Zhou, D., Zhu, Y.-H., Zhang, W., Wang, M.-L., Fan, W.-Y., Song, D., Yang, G.-Y., Jensen, B. B., & Wang, J.-F. (2015). Oral administration of a select mixture of Bacillus probiotics generates Tr1 cells in weaned F4ab/acR− pigs challenged with an F4+ ETEC/VTEC/EPEC strain. Veterinary Research, 46(1), 95. https://doi.org/10.1186/s13567-015-0223-y
- Zuckermann, F. A., & Husmann, R. J. (1996). Functional and phenotypic analysis of porcine peripheral blood CD4/CD8 double-positive T cells. Immunology, 87(3), 500–512. https://doi.org/10.1046/j.1365-2567.1996.494570.x
- Zuckermann, F. A., Pescovitz, M. D., Aasted, B., Domingeuz, J., Trebichavsky, L., Novikov, B., Valpotic, I., Nielsen, J., Arn, S., Sachs, D. H., Lunney, J. K., Boyd, P., Walker, J., Lee, R., Davis, W. C., Barbosa, I. R., & Saalmüller, A. (1998). Report on the analyses of mAb reactive with porcine CD8 for the second international swine CD workshop. Veterinary Immunology and Immunopathology, 60(3–4), 291–303. https://doi.org/10.1016/S0165-2427(97)00106-2