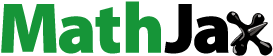
ABSTRACT
Gynura procumbens is a traditional herb and food extensively cultivated in China and Southeast Asian countries. This study was designed to determine the content of the main chemical components and elucidate the antioxidant and anti-inflammatory capacity of G. procumbens flowers extracts (GPFE). GPFE was rich in flavonoids and phenolics and exhibited great reducing power as well as strong scavenging ability of ABTS+ and DPPH radicals. The pro-inflammatory factors including IL-6, IL-1β, NO, TNF-α, PGE2 and their mRNA transcriptions were strongly inhibited with the treatment of GPFE in LPS-stimulated RAW264.7 macrophages. Meanwhile, GPFE could attenuate the inflammation via suppressing the nuclear translocation of NF-κB p65 and downregulating the MAPK signalling pathways. Furthermore, isochlorogenic acid B, isochlorogenic acid A and isochlorogenic acid C isolated from GPFE all had a high content and showed potent anti-inflammatory activities. Our findings suggest that GPFE could be used as a natural anti-inflammatory agent.
1. Introduction
Oxidation is a vital basis of cellular metabolism and an important part of aerobic life, whereas, this process is often accompanied by the formation of free radicals (Cao et al., Citation2022). The production of free radicals can damage mitochondrial DNA and initiate the oxidation chain reactions, causing the development of inflammation (Kumar et al., Citation2017). Inflammation is a normal physiological response that our bodies against the external stimulus, relating to tissue damage and diseases. The inflammation is broadly divided into acute inflammation and chronic inflammation (Seo et al., Citation2015). Specifically, the acute inflammation is self-resolving by nature, while chronic inflammation often occurs at a longer time and does not resolve, easily inducing arthritis, cardiovascular diseases, diabetes, Alzheimer’s disease, cancer and other chronic diseases (Ahmad et al., Citation2018; He et al., Citation2021). In a sense, inflammation is also regarded as “a silent health killer”. Macrophages, a type of immune cell, are an important barrier against most diseases in our body. Generally speaking, macrophages could increase the nitric oxide (NO) release and the production of inflammatory factors consisting of prostaglandin E2 (PGE2), interleukin-1β (IL-1β) and tumour necrosis factor-α (TNF-α) in order to initiate the response of inflammation when stimulated by tissue injury and external microorganism infection. These secretions of pro-inflammatory factors and the expressions of related genes are inextricably linked to the intracellular signalling (Park et al., Citation2020). Mitogen-activated protein kinase (MAPK) is crucial signalling pathways in the inflammatory response and the transcription factors comprising nuclear factor-κB (NF-κB) and activator protein 1 (AP-1) are pivotal components in downstream signalling, functioning at the merging end point of diverse signalling pathways (Endale et al., Citation2017; Shin et al., Citation2017). Hence, any of the components or substances which can downregulate these signalling pathways and the release of inflammatory cytokines are greatly beneficial for inflammatory treatment. Nonsteroidal anti-inflammatory drugs and synthetic antioxidants have been used commonly in the clinical treatment and food industry, whereas these drugs have some side effects including nausea, gastric bleeding, vomiting and liver damage for long-term use (Ahmad et al., Citation2018; Sava et al., Citation2021). Thus, the exploitation of effective natural antioxidant and anti-inflammatory drugs is the focus of current research.
Gynura procumbens (Lour.) Merr. is a popular food extensively cultivated in China and Southeast Asian countries, belonging to the Asteraceae family (Tan et al., Citation2016). Particularly, it is extensively referred to as “Sambung Nyawa” in Malaysia, meaning longevity and “cure-all” (Rosidah et al., Citation2008). Multiple chemical constituents of G. procumbens including steroids, caffeoylquinic acids, fatty acids, flavonoids and terpenoids contribute its rich bioactivities comprising anti-inflammatory, antioxidative, antithrombotic and analgesic (Hwang et al., Citation2014 Kim & Kim, Citation2011;). Thus, G. procumbens has been broadly used in modern medicine in lots of diseases treatment, such as cancer, arthritis, gout and other cardiovascular diseases (Huang et al., Citation2019; Kaewseejan et al., Citation2015). Besides, the leaves of G. procumbens (GPL) were proven to be non-toxic and it was used as a material to make toothpaste, skin cream, candy and tea. National Health Commission of the PRC also approved G. procumbens as a new food resource in 2012 (Hu et al., Citation2019). Taken together, from the perspective of whether edible or medicinal value, G. procumbens shows promising potentials.
To our knowledge, the bioactivities including antioxidant activity and anti-inflammatory activity of GPL have been widely reported (Tan et al., Citation2016), but the research of G. procumbens flowers (GPF) has thus far been absent, neither in terms of bioactivities nor chemical constituents. In addition, GPF are also usually discarded after the GPL are harvested, this not only results in wastage of the G. procumbens resources but also limits the sufficient utilization and further development of G. procumbens. Therefore, in order to fill this gap, we conducted a preliminary study on the chemical components and the bioactivities including the anti-inflammatory and antioxidant activity of GPF. Then the related molecular mechanisms of anti-inflammatory activity and main bioactive components were further elucidated in this work to refine the anti-inflammation theory.
2. Materials and methods
2.1. Materials
Fetal bovine serum (FBS) was obtained from Natocor (Cordoba, Argentina). High glucose Dulbecco’s Modified Eagle’s Medium (DMEM) was purchased from HyClone (Logan, Utah, USA). Penicillin, streptomycin, 3-(4,5-dimethylthiazol-2-yl)-2,5-diphenyltetrazolium bromide (MTT), phosphate buffer saline (PBS), lipopolysaccharide (LPS) and sodium dodecyl sulphate (SDS) were obtained from Sangon Biotech (Shanghai, China). Enhanced BCA Protein Assay Kit, 4,6-diamidino-2phenylindole (DAPI) were obtained from Beyotime (Shanghai, China). Griess B (1%(w/v) sulphanilamide containing 5%(w/v) H3PO4), Griess A (0.1%(w/v) N-(1-naphthyl)-ethylenediamine dihydrochloride), RIPA buffer, dimethyl sulfoxide (DMSO) and sodium nitrite were provided by Solarbio (Beijing, China). ELASA kits of IL-6, TNF-α and IL-1β were obtained from Boster (Wuhan, China). The primary antibodies for iNOS, COX-2, p38, p-ERK, p-p38, ERK, JNK, p-JNK, GAPDH and the secondary antibody goat anti-rabbit (IgG) were provided by AB clonal Biotech (Wuhan, China). Standards were purchased from Shanghai yuanye Bio-Technology Co., Ltd (Shanghai, China). Other analytical grade reagents and chemicals were obtained from Tianjin ZhiYuan Reagent Co., Ltd (Tianjin, China).
2.2. Extraction and preparation of sample
GPF were collected from Ganzhou city of China, in June 2020, and were authenticated by Prof. X.H. Li, Jiangxi academy science. A voucher specimen (GPL2020-06) was stored in our laboratory. The GPF samples were dried and immersed in 70% ethanol for a week at room temperature, followed by twice extracting (25 min each) using ultrasonication (35°C, 350 W). Next, it was concentrated under vacuum after filtering. The freeze-drying was further conducted to get crude extract (GPFE).
2.3. Total phenolic content
Total phenolic content (TPC) assay was tested based on the Folin method (Mustafa et al., Citation2019). 0.2 mL GPFE solution was mixed sequentially with 1 mL purified water and 200 μL Folin-phenol reagent, followed by shaking and standing for 3 min. Next, 0.6 mL Na2CO3 (7.5%, w/v) was added and the absorbance of mixed solution was determined at 765 nm by the micro-plate reader (Tecan Infinite 200 Pro, Austria) after 40 min standing. The content was represented by mg gallic acid equivalent (GAE)/g dry extract.
2.4. Total flavonoid content
Total flavonoid content (TFC) assay was tested pursuant to the literature (Mustafa et al., Citation2019). Firstly, 300 μL NaNO2 (5%, w/v) was mixed with 1 mL GPFE solution and left for 6 min in the dark. Afterwards, 300 μL AlNO3 (10%, w/v) and 2 mL NaOH (4%, w/v) were sequentially added in it with a uniform shock before standing for 15 min, followed by measuring at 510 nm to get the absorbance. The content was presented as mg rutin equivalent (RE)/g dry extract.
2.5. HPLC preliminary analysis of caffeoylquinic acids in GPFE
The method of HPLC analysis was built as stated by the earlier study from our laboratory (Cao et al., Citation2021). The SHIMADZU Shim-pack GIST C18 column (4.6 × 250 nm ID, 5 μm) was used in the HPLC system with 35°C column temperature. Flow rate, 1.0 mL/min; UV wavelength, 327 nm; injection volume, 10 μL. The elution procedure was shown in .
Table 1. HPLC gradient elution procedure.
2.6. Antioxidant activity assay
2.6.1. ABTS assay
ABTS method in this work was in keeping with Sun et al. (Citation2020). ABTS working solution consisted of 900 μL K2S2O8 (2.45 mM) and 900 μL ABTS (7.0 mM) and needed to stand in dark for 14 h to turn a dark blue colour. It was necessary to dilute with ethanol to gain the absorbance of 0.7 ± 0.02 at 734 nm before using. Next, 600 μL solution was mixed with different concentrations of 200 μL GPFE evenly with 30 min incubation, followed by detecting at 734 nm (200 μL/well in 96-well plate) to get the absorbance. Ethanol and vitamin C (VC) in this research were used as the control and positive control, respectively. The clearance rate was computed using the following equation:
( 1)
( 1)
2.6.2. DPPH assay
DPPH· clearance rate was detected pursuant to the literature (Sun et al., Citation2020). In short, 350 μL of 0.2 mM DPPH and 350 μL GPFE were placed into the centrifuge tubes. The tubes were shaken homogeneously and left for 30 min reaction. Ultimately, the absorbance of 200 μL solution was tested at 517 nm. The control and the calculation formula (1) were the same as the ABTS assay.
2.6.3. Ferric reducing power assay
The ferric reducing antioxidant power (FRAP) of GPFE was assessed in consonance with the description from Cao et al. (Citation2022). 0.2 mL GPFE was mixed sequentially with 0.5 mL PBS (PH 6.6, 200 μM) and 0.5 mL of 1% K3[Fe(CN6)] (w/v) in 2 mL tubes, followed by 30 min incubation at 55°C. Thereafter, the tubes were cooled promptly and 0.5 mL trichloroacetic acid (10%, w/v) was placed in it for 11 min reaction. Next, 0.5 mL of supernatants in each tube were aspirated and mixed into the solution, which contained 0.5 mL purified water and 0.1 mL FeCl3 (0.1%). The absorbance was finally detected at 700 nm after a 10-min reaction.
2.7. The evaluation of anti-inflammatory activity
2.7.1. Cell culture
RAW 264.7 cells were provided by the Key Laboratory of Pu-er Tea Science and cultured in high glucose DMEM containing 1% penicillin–streptomycin and 10% FBS. Esco CO2 incubator (Shanghai, China) was used to culture the cells with 5% CO2 atmosphere at the constant temperature of 37°C. The cells were subcultured based on the growth.
2.7.2. Cell viability and the determination of NO content
Cytotoxicity of GPFE on RAW264.7 macrophages was measured according to MTT assay (Bayazid et al., Citation2020). RAW264.7 cells were cultured in 96-well plate (5 × 105 cells/well, 200 μL/well) with 24 h incubation. Next, different concentrations of GPFE (0–100 μg/mL) were added in each well (except control group) for 2 h pre-treatment and LPS (1 μg/mL) was added in these wells for extra 24 h incubation. Then 100 μL supernatants of every well were aspired and mixed with an equal volume of Griess reagent (Griess A: Griess B, 1:1, v/v). After 10 min shake and reaction, the absorbance was immediately detected at 540 nm to calculate the NO production based on the NaNO2 standard curve (Baek et al., Citation2015). In addition, the remaining culture solution (100 μL in each well) was entirely discarded and 100 μL serum-free medium containing 1 mg/mL MTT was further added to it for 4 h incubation. 100 μL MTT stop buffer (10% SDS with 0.01 M HCl) was added and treated for another 16–20 h to stop the reaction. At last, the absorbance was detected at 570 nm to evaluate the cytotoxicity of GPFE.
2.7.3. ELISA assay
RAW264.7 macrophages (1 × 106 cells/well) were cultured in six-well plates overnight, and the cells were treated with a range of GPFE concentrations (0–100 μg/mL) for 2 h and then stimulated with LPS (1 µg/mL) for 24 h. The supernatants in six-well plate were collected and the analysis of inflammatory cytokines consisting of TNF-α, PGE2, IL-6 and IL-1β were determined by ELISA kits (Boster, Wuhan, China).
2.7.4. The analysis of mRNA expression by quantitative RT–PCR (RT-qPCR)
RAW264.7 macrophages (1 × 106 cells/well) were cultured in six-well plates with 24 h incubation, GPFE with various concentrations (0–100 μg/mL) were added in each well (except control group) for 2 h pre-treatment and 1 μg/mL LPS was subsequently added for 6 h incubation. Then total RNA was extracted by the TransZol Up RNA extraction kit (Transgen Biotech, Beijing, China). The purities and concentrations of RNA were measured using UV spectrophotometer (Biochrom, UK) with the absorbance 260/280 nm. PrimeScriptTM RT reagent kit with gDNA eraser (Takara, Dalian, China) was used to remove genomic DNA and reverse-transcribe 1 μg total RNA to cDNA. Afterwards, quantitative PCR was performed in 20 μL reaction volumes by qTOWER3G (Analytik Jena, Germany) to quantify the expression levels of mRNA. The cycling procedure was 95°C for 30 s, followed by 40 cycles of 95°C for 5 s and 60°C for 30 s. The expressions of relative genes were analysed according to the 2−ΔΔCt method. The primer sequences were shown in and GAPDH was considered as the internal reference.
Table 2. Primers list.
2.7.5. Western blot assay
RAW264.7 cells (1 × 106 cells/dish) were cultured in 60 mm dishes for 20 h, CE was added in each dish (except control group) for 2 h pre-treatment and 1 μg/mL LPS was then added for incubation with indicated times. RIPA lysis and PBS were used to prepare the protein lysates. The proteins were quantified using BCA kits and 35 μg proteins were further subjected to SDS-PAGE gels electrophoresis and transferred onto PVDF membranes. Then they were blocked using 5% skim milk at room temperature for 1 h. The Super ECL Plus (US EVERBRIGHT INC., Suzhou, China) was used to detect the protein bands by ChemiScope 3300 system (Clinx, Shanghai, China) after the incubation of primary antibodies for 12–18 h at 4°C and the incubation of secondary antibodies at 24°C for 50 min.
2.7.6. Immunofluorescence assay
The immunofluorescence assay for activity determination of NF-κB p65 in this study was carried out by the instructions of NF-κB activation-nuclear transfer kit (Beyotime, Shanghai, China). In short, RAW264.7 cells were inoculated into 60 mm dishes with 1 × 106 cells/dish overnight. Then, RAW264.7 cells were cleaned and fixed with fixing solution for 15 min after the treatment of CE and LPS, followed by blocking with blocking solution for 1 h. NF-κB p65 primary antibody was added for 16 h incubation at 4°C and the Cy3-conjugated secondary antibody was incubated for 50 min at 24°C. In the end, the cells were observed using Eclipse Ts2 microscope (Nikon, Japan) after staining by DAPI.
2.7.7. Anti-inflammatory activity evaluation of chemical constituents in GPFE
The five caffeoylquinic acids were isolated from GPFE according to our previous literature (Cao et al., Citation2021) and subsequent experiments. The evaluation of anti-inflammatory activity was essentially the same as the MTT methods and NO determination described in “2.7.2” of this work.
2.8. Statistical analysis
The data were processed by SPSS 25.0 (SPSS Inc., Chicago, IL, USA) and shown as mean ± SD values. The significance test was determined by one-way ANOVA followed by the Tukey’s multiple comparison tests and p-values <0.05 were considered as a significant difference.
3. Results and discussion
3.1. Caffeoylquinic acids, flavonoid and phenolic contents of GPFE
The total phenolic content (TPC) and total flavonoid content (TFC) of GPFE were tested using colorimetric methods while the contents of the caffeoylquinic acids including chlorogenic acid (CA), isochlorogenic acid B (ICAB), isochlorogenic acid A (ICAA), isochlorogenic acid C (ICAC) and neochlorogenic acid (NCA) were quantified by HPLC. As shown in , the TPC and TFC of GPFE were 45.563 mg GAE/g dry extract (DW) and 119.245 mg RE/g dry extract (DW), respectively. From and , ICAA was the highest caffeoylquinic acid with the content of 8.386 mg/g while NCA had the lowest content with 1.181 mg/g. Besides, the ICAB, CA and ICAC all had a high content, with 5.716, 5.046 and 5.899 mg/g, respectively.
Figure 1. HPLC chromatograms of samples. (A) The chromatogram of mixed reference standard of caffeoylquinic acids. (B) The chromatogram of GPFE samples. (1: neochlorogenic acid, 2: chlorogenic acid, 3: isochlorogenic acid B, 4: isochlorogenic acid A, 5: isochlorogenic acid C).
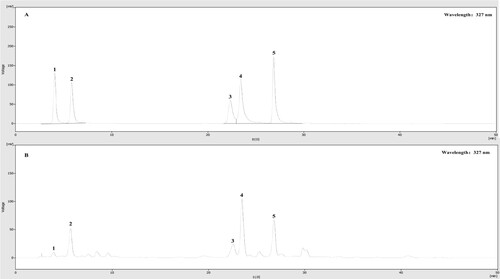
Table 3. Caffeoylquinic acids, flavonoid and phenolic contents of GPFE.
Caffeoylquinic acids extensively exist in multiple herbs and plants, it was also the primary chemical constituents in G. procumbens (Ashraf, Citation2019). Previous literature indicated that the contents of ICAB, CA, ICAC and ICAA in G. procumbens leaves extract (GPLE) were 3.20, 7.18, 9.72 and 28.31 mg/g, much higher than that in G. procumbens stems extract (GPSE) with the contents of 0.40, 2.79, 1.15 and 2.50 mg/g (Li et al., Citation2015; Murugaiyah et al., Citation2018). In this study, the contents of caffeoylquinic acids in GPFE were studied for the first time. After comparison with the GPLE and GPSE, caffeoylquinic acids in different parts of G. procumbens were as follows: GPLE > GPFE > GPSE. This could be because the caffeoylquinic acids are more synthesized in leaves than in flowers or stems, support for this idea was also discovered in the research of Murugesu et al. (Citation2021). Of course, these compounds’ contents in G. procumbens also largely depended on other external factors, such as harvest period, origin area and growing condition. Our study suggested that GPFE had a potential research value because of the rich chemical constituents. Among them, we speculated that the diversity and content of these caffeoylquinic acids could be the main basis in the biological activities of GPFE.
3.2. Antioxidant activity of GPFE
Antioxidants can act through different mechanisms, consisting of hydrogen atoms transfer and electrons transfer. ABTS+· and DPPH· are stable free radicals, it can readily accept electrons or hydrogen atoms from antioxidants to get a stable status (Bai et al., Citation2018). Hence, the DPPH and ABTS assay were typically used to assess the antioxidant capacity by detecting the rate of radical scavenging. As shown in (A), GPFE exhibited strong radical scavenging activity across the ABTS and DPPH assay. The DPPH·and ABTS+· scavenging rates were heightened in a dose-dependent manner with the GPFE concentrations increased and the scavenging activities of 1 mg/mL GPFE were comparable to the VC at the same concentration. In addition, here, FRAP assay was also used to evaluate the GPFE potential antioxidant ability. The reduction of Fe3+ is a quintessential electron transfer reaction (Xiao et al., Citation2015; Yang et al., Citation2019). The antioxidant is a good electron donor which can reduce the Fe3+ to the Fe2+, reflecting the antioxidant ability (Ko et al., Citation2020). The reducing power of GPFE was shown in (B) and indicated by the absorbance at 700 nm. Overall, the higher absorbance stands for the stronger reducing power (Lu et al., Citation2016). The absorbance was continuously increased with the concentration of GPFE. It suggested that GPFE had some antioxidant ability but was still weaker than VC.
Figure 2. Determination of the antioxidant activity of GPFE; (A) ABTS and DPPH assay. (B) Reducing power assay.
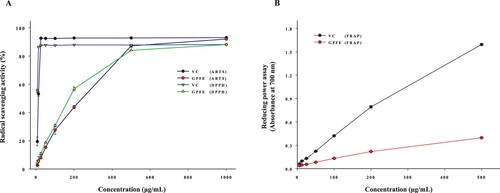
So far, the antioxidant activities of GPLE have been investigated copiously. It was reported that GPLE possessed strong radical scavenging activities in DPPH assay (IC50 = 473.70 μg/mL) and ABTS assay (IC50 = 223.18 μg/mL) (Kaewseejan et al., Citation2015). Nevertheless, the antioxidant activities of GPFE were first studied in this work and the IC50 values of radical scavenging rates in GPFE were 157.95 μg/mL (DPPH assay) and 180.23 μg/mL (ABTS assay). As we all know, IC50 is a key indicator that can reflect the antioxidant capacity. Lower IC50 represents stronger antioxidant ability (Yang et al., Citation2019). Apparently, from the data of IC50, the antioxidant activities of GPFE were stronger than GPLE. These results were probably associated with the active ingredients of GPLE and GPFE. Phenolics and flavonoids contribute immensely to various bioactivities, especially in antioxidation (Yin et al., Citation2014). They are considered an excellent source of antioxidants. Studies by Kaewseejan et al. (Citation2015) suggested that the TPC and TFC in GPLE were 16.08 mg GAE/g DW and 10.33 mg RE/g DW. Compared with these results, our study found that the TPC and TFC in GPLE were much less than that in GPFE. Thus, we speculated that the stronger antioxidant capacity of GPFE may originate from the high contents of flavonoids and phenolics to a great extent. Whereas, specific studies are required to confirm this speculation.
3.3. Anti-inflammatory activity of GPFE
3.3.1. Effect of GPFE on cell viability and NO production
NO is a key inflammatory mediator closely associated with the inflammatory response. Low level of NO (<300 nM) exerts anti-inflammatory effects while excessive NO release can intensify the process of inflammation (Sava et al., Citation2021). Therefore, the inhibition of NO release becomes a crucial indicator, reflecting the anti-inflammatory activity. Hence, firstly, the effects of GPFE with different concentrations on NO release in RAW264.7 cells was studied in this work. From (A), the release of NO in LPS group (34.58 ± 0.33 μM) was significantly higher than control group (6.18 ± 0.59 μM), and indicated that LPS can successfully stimulate the inflammation of RAW264.7 cells. GPFE (12.5–100 μg/mL) prominently reduced the NO production and the NO production was decreased in a dose-dependent manner when the RAW264.7 cells were treated with GPFE. In addition, GPFE in various concentrations (0–100 μg/mL) all had high cell viability (over 90%) and no significant cytotoxicity ((B)). It suggested that GPFE inhibited NO without causing cell death and these concentrations were also safe for subsequent experiments.
Figure 3. Effects of GPFE on NO release and cell viability in RAW264.7 cells induced with LPS. (A) The NO production was tested using the Griess reagent. (B) The cell viability was determined by MTT method. Data were presented as means ± SD (n = 3). *p < 0.05 vs. LPS group, **p < 0.01 vs. LPS group, ##p < 0.01 vs. control group.
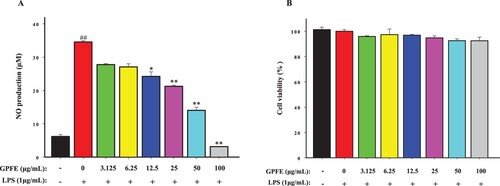
3.3.2. Effect of GPFE on pro-inflammatory factors productions
Besides NO, RAW264.7 cells can also increase the secretion of other inflammatory factors, such as PGE2, IL-6, IL-1β and TNF-α when stimulated with LPS. The secretion of these inflammatory factors was also studied in this work by ELASA kits. From , it was clear that LPS treatment prominently increased the concentrations of four inflammatory factors. However, the secretion of IL-6, TNF-α, PGE2 and IL-1β all reduced significantly when 100 μg/mL GPFE was added. It suggested that GPFE exerts anti-inflammatory activity according to the inhibition of inflammatory factors.
Figure 4. Effects of GPFE on pro-inflammatory factors production in LPS-induced RAW264.7 cells. The production of pro-inflammatory factors was determined by ELASA kits. (A) TNF-α production. (B) IL-1β production. (C) IL-6 production. (D) PGE2 production. Data were normalized to GAPDH and presented as means ± SD (n = 3). ##p < 0.01 vs. control group, *p < 0.05 vs. LPS group, **p < 0.01 vs. LPS group.
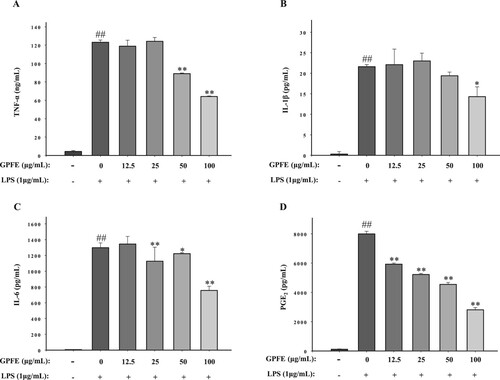
3.3.3. Effect of GPFE on pro-inflammatory factors mRNA expressions
RT-qPCR was used to measure the mRNA expression levels quantitatively. As displayed in , IL-1β, COX-2, IL-6, iNOS and TNF-α mRNA expression levels in control group were extremely low while the presence of LPS significantly increased these expression levels. The mRNA expressions including IL-1β, IL-6 and TNF-α were strongly reduced with the pre-treatment of GPFE on the test concentrations (12.5–100 μg/mL). GPFE also significantly suppressed the COX-2 mRNA expressions at 100 μg/mL. In addition, GPFE (12.5–100 μg/mL) all markedly inhibited iNOS mRNA expressions in a dose-dependent manner.
Figure 5. Effects of GPFE on mRNA expressions of pro-inflammatory factors in LPS-induced RAW264.7 cells. The mRNA expressions were determined by RT-qPCR. (A) IL-1β mRNA level. (B) IL-6 mRNA level. (C) TNF-α mRNA level. (D) COX-2 mRNA level. (E) iNOS mRNA level. Data were normalized to GAPDH and presented as means ± SD (n = 3). ##p < 0.01 vs. control group, *p < 0.05 vs. LPS group, **p < 0.01 vs. LPS group.
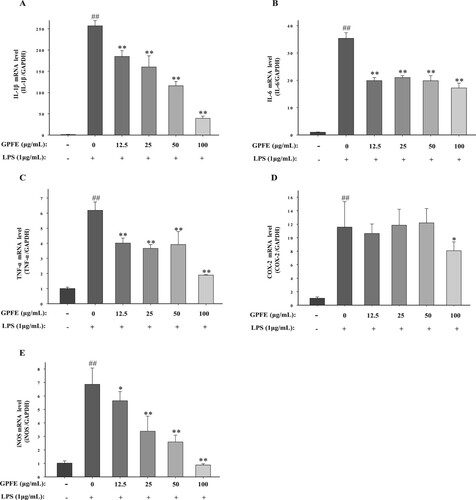
3.3.4. Effect of GPFE on COX-2 and iNOS protein expressions
The effect of GPFE in different concentrations on COX-2 and iNOS protein expressions in RAW264.7 cells were studied using western blot. COX-2 and iNOS expressions were prominently upregulated after the treatment of LPS, whereas 100 μg/mL GPFE all strongly downregulated their expressions (). Furthermore, GPFE at 100 μg/mL nearly entirely suppressed iNOS protein expression and the expression was almost downregulated to the basal level compared with control group.
Figure 6. Effects of GPFE on COX-2 and iNOS protein expressions in LPS-induced RAW264.7 cells. (A) Western blots of COX-2, iNOS and GAPDH protein expression. (B) Quantitative graph of COX-2 and iNOS. Data were normalized to GAPDH and presented as means ± SD. #p < 0.05 vs. control group, *p < 0.05 vs. LPS group.
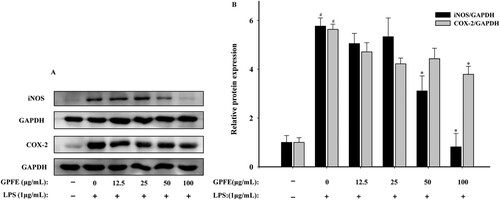
3.3.5. Effect of GPFE on the regulation of transcription factor
p65 is a key subunit of NF-κB and it can enter to the nucleus to regulate the expressions of inflammatory genes and protein (Yang et al., Citation2018). In this work, the confocal microscope was used to observe the effect of GPFE with various concentrations on p65 nuclear translocation in order to confirm whether the GPFE involved the regulation of NF-κB activation. As shown in , p65 in nucleus was dramatically increased in LPS group, activating the NF-κB. With the treatment of 100 μg/mL GPFE, p65 entry from the cytoplasm into the nucleus was markedly inhibited. Collectively, GPFE alleviated inflammation by suppressing NF-κB activation.
3.3.6. Effect of GPFE on regulation of MAPK signalling pathways
Generally, NF-κB expression regulation is related to the MAPK signalling pathways (Shin et al., Citation2017). Hence, to verify whether the MAPK signalling pathways were involved, RAW264.7 cells were treated with LPS and GPFE for different time points and the expression levels of p-p38, p-ERK and p-JNK were measured. As seen in , the phosphorylation levels of ERK, JNK and p38 protein were markedly promoted and at 30 min after the LPS was added while 100 μg/mL GPFE produced an obvious inhibition in their phosphorylation level at the same time. Taken together, it suggested that GPFE attenuated inflammation via MAPK signalling pathways downregulation.
3.3.7. Preliminary evaluation in anti-inflammatory activity of chemical constituents from GPFE
As shown in , the anti-inflammatory activities of five caffeoylquinic acids in GPFE were evaluated in this work. The MTT assay showed that all five caffeoylquinic acids at three different concentrations (10, 20 and 40 μM) exhibited no cytotoxicity. The results of NO production indicated that 20 μM ICAB significantly inhibited the NO release of LPS-induced RAW264.7 cells. In addition, ICAA, ICAB and ICAC all markedly reduced the NO production at 40 μM while the remaining two compounds did not show a significant effect on NO production at the same concentration.
Figure 9. Effects of caffeoylquinic acids of GPFE on NO release and cell viability in RAW264.7 cells induced with LPS. (A) The NO production was tested using the Griess reagent. (B) The cell viability was determined by MTT method. Data were presented as means ± SD (n = 3). *p < 0.05 vs. LPS group, ##p < 0.01 vs. control group. (CA, chlorogenic acid; NCA, neochlorogenic acid; ICAA, isochlorogenic acid A; ICAB, isochlorogenic acid B; ICAC, isochlorogenic acid C).
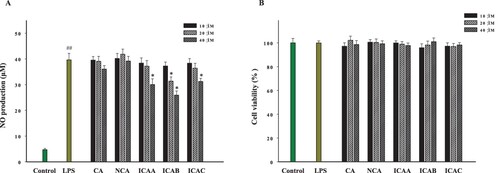
Inflammation, a complex pathophysiology response triggered by foreign microbial pathogens, is often accompanied with various diseases. LPS is the main component of the Gram-negative bacteria cell wall, which belongs to these foreign pathogens, potently activating RAW264.7 cells and triggering inflammation (Cheng et al., Citation2014; Gong et al., Citation2020). Hence, the LPS-stimulated RAW264.7 macrophages are a classical vitro cell model for the assessment and selection of natural products derived from medicinal plants in anti-inflammatory activity.
The inflammatory cytokines produced by immune cells are a significant marker in inflammatory response. Bilberry (Vaccinium myrtillus L.) could suppress NO generation and reverse inflammatory factors such as iNOS, TNF-α, COX-2 and IL-6 in LPS-induced RAW264.7 cells. It was also considered to be a natural powerful anti-inflammatory fruit (Bayazid et al., Citation2021). In this study, GPFE markedly inhibited NO secretion without causing cell death and it also significantly reduced the secretion of inflammatory factors consisting of IL-6, PGE2, TNF-α and IL-1β. PGE2 and NO, as the pivotal regulatory factors in inflammation, majorly derive from arachidonic acid and l-arginine of COX-2 and iNOS proteins, respectively (Alam et al., Citation2020). IL-6 is a multidirectional interleukin which mainly secreted by macrophages and monocytes. It can stimulate target cells and recruit STAT to activate the MAPK signalling pathways (Klimontov et al., Citation2020). IL-1β is a multi-functional inflammatory factor, closely associated with pain and local or systemic inflammation. Furthermore, TNF-α can stimulate the secretion of other cytokines including IL-6 and IL-1β by the regulation of cytokine cascade, which in turn, enhance the leukocytes recruitment to inflammation sites (Cao et al., Citation2019). The secretion of these inflammatory factors derives from the related genes transcription and proteins translation, which are activated by a complex signalling cascade. In this work, we discovered that GPFE could prominently suppress the release of these inflammatory factors via inhibiting the expression of related genes and proteins, as confirmed by the result of RT-qPCR and Western Blotting ( and ). A study reported by Ning et al., which pointed out that 250 μg/mL GPLE was capable of reducing NO production by obviously downregulating iNOS protein expression (Citation2019). In addition, the essential oils of G. procumbens markedly inhibited the COX-2 overexpression to alleviate the inflammation and deswelling in mice (Huang et al., Citation2019). All of these findings proved the anti-inflammatory activity of G. procumbens. Notwithstanding that these works just confined to the level of protein or gene, the studies of related signalling pathways were still poorly involved. Hence, further research is needed.
The transcription of inflammatory genes in cells is usually regulated by NF-κB. Specifically, the activation of NF-κB can promote the release of inflammatory cytokines, which in turn, makes a positive feedback loop to increase the NF-κB (Tantowi et al., Citation2020). Moreover, the activation of NF-κB is regulated by intricate signaling pathways comprising MAPK. MAPK family contains three major signalling cascades: JNK, ERK and p38 MAPK, relating to inflammatory reaction and cell growth, differentiation and apoptosis (Jin et al., Citation2008). As one of the pivotal upstream components of NF-κB, MAPK signalling pathways can regulate the activation and nuclear translocation of NF-κB (Yang et al., Citation2020). Under normal conditions, IκBα binds to NF-κB composed of p50 and p65. When stimulated with LPS, the related signals are transmitted and the IκBα is activated and phosphorylated, followed by ubiquitination and degradation, then the NF-κB is released and the subunit p65 quickly enters the nucleus to initiate or enhance the target gene transcription (Gong, Wen, et al., Citation2019; Yang et al., Citation2018). This process is considered a positive inflammatory reaction. In our study, we discovered that GPFE could mitigate inflammation via inhibiting the nuclear translocation of NF-κB and downregulating the MAPK signalling pathways (). Flavonoids, a class of phenolic compounds, occur widely in plants and are important plant metabolites. Peng et al. (Citation2019) suggested that flavonoids of Exocarpium Citri Grandis could regulate the activation of MAPK and NF-κB signalling pathways to exert anti-inflammatory effects. It proved a significant role of flavonoids in anti-inflammatory activity. Besides, caffeoylquinic acid is also a major class of phenolic compounds in medicinal plants. As a common caffeoylquinic acid, CA can also prominently reduce the release of NO, TNF-α and IL-1β by downregulating the expression of iNOS, Ninj1 and COX-2 and suppressing the nuclear translocation of NF-κB (Hwang et al., Citation2014). In our previous work, five caffeoylquinic acids including CA, NCA, ICAB, ICAA and ICAC were separated from GPFE for the first time. Then the content and the anti-inflammatory activity of these five compounds were determined in this study. We found that these five caffeoylquinic acids were the primary phenolic compounds in GPFE (), furthermore, ICAB, ICAA and ICAC all exhibited a strong anti-inflammatory activity. However, it was interesting that CA did not show significant inhibition of NO production in this work, probably owing to the lower test concentration. Numerous studies have indicated that di-caffeoylquinic acids possessed better pharmacological effects than mono-caffeoylquinic acids (Izuta et al., Citation2009; Murugesu et al., Citation2021), our study also echoes this statement in anti-inflammatory activity. Caffeoyl structures containing catechol groups are more effective structures in caffeoylquinic acids and the increase of caffeoyl groups in caffeoylquinic acids can increase their bioactivity (Taira et al., Citation2014). Studies conducted by Gong, Chu, et al. (Citation2019) pointed out that di-caffeoylquinic acids had more effective abilities in reducing phosphorylation of p38/MAPK cascades and IL-8 secretion, compared to mono-caffeoylquinic acid. This conclusion also provides support for our findings. Therefore, ICAB, ICAA and ICAC could play a dominant role in anti-inflammatory activity of GPFE.
4. Conclusion
In this work, the chemical compositions and bioactivities of GPFE were studied and the results suggested that GPFE was rich in flavonoids and phenolics and exhibited strong antioxidant activity as well as anti-inflammatory ability. Caffeoylquinic acids were the major phenolic constituents in GPFE, among them, the di-caffeoylquinic acids including ICAB, ICAA and ICAC could contribute largely to the anti-inflammatory activity of GPFE. In conclusion, GPFE has the potential to be an antioxidant and anti-inflammatory agent in future treatment with a promising application prospect. Further in vivo experiments are necessary to refine the evaluation of anti-inflammatory of GPFE.
Disclosure statement
No potential conflict of interest was reported by the author(s).
Additional information
Funding
References
- Ahmad, M. H., Fatima, M., Hossain, M., & Mondal, A. C. (2018). Evaluation of naproxen-induced oxidative stress, hepatotoxicity and in-vivo genotoxicity in male Wistar rats. Journal of Pharmaceutical Analysis, 8(6), 400–406. https://doi.org/10.1016/j.jpha.2018.04.002
- Alam, M. B., Chowdhury, N. S., Sohrab, M. H., Rana, M. S., Hasan, C. M., & Lee, S.-H. (2020). Cerevisterol alleviates inflammation via suppression of MAPK/NF-κB/AP-1 and activation of the Nrf2/HO-1 signaling cascade. Biomolecules, 10(2), 199. https://doi.org/10.3390/biom10020199
- Ashraf, K. (2019). An updated phytochemical and pharmacological review on Gynura procumbens. Asian Journal of Pharmaceutical and Clinical Research, 12, 9–14. https://doi.org/10.22159/ajpcr.2019.v12i4.28996
- Baek, K.-S., Hong, Y. D., Kim, Y., Sung, N. Y., Yang, S., Lee, K. M., Park, J. Y., Park, J. S., Rho, H. S., & Shin, S. S. (2015). Anti-inflammatory activity of AP-SF, a ginsenoside-enriched fraction, from Korean ginseng. Journal of Ginseng Research, 39(2), 155–161. https://doi.org/10.1016/j.jgr.2014.10.004
- Bai, M., Han, W., Zhao, X., Wang, Q., Gao, Y., & Deng, S. (2018). Glycosaminoglycans from a sea snake (Lapemis curtus): extraction, structural characterization and antioxidant activity. Marine Drugs, 16(5), 170. https://doi.org/10.3390/md16050170
- Bayazid, A. B., Chun, E. M., Al Mijan, M., Park, S. H., Moon, S.-K., Lim, B. O. J. F., & Immunology, A. (2021). Anthocyanins profiling of bilberry (Vaccinium myrtillus L.) extract that elucidates antioxidant and anti-inflammatory effects. Food and Agricultural Immunology, 32(1), 713–726. https://doi.org/10.1080/09540105.2021.1986471
- Bayazid, A. B., Park, S. H., Kim, J. G., & Lim, B. O. (2020). Green chicory leaf extract exerts anti-inflammatory effects through suppressing LPS-induced MAPK/NF-κB activation and hepatoprotective activity in vitro. Food and Agricultural Immunology, 31(1), 513–532. https://doi.org/10.1080/09540105.2020.1742667
- Cao, M.-y., Hu, J.-w., Gu, Z., Xiong, W., Wu, L., Xu, J.-g., & Wu, L.-y. (2021). Purification of four caffeoylquinic acid derivatives from the flowers of Gynura procumbens by HSCCC. Journal of Chromatographic Science, 59(10), 971–977. https://doi.org/10.1093/chromsci/bmab036
- Cao, M.-y., Wu, J., Wu, L., Gu, Z., Xie, C.-q., Wu, L.-y., Hu, J.-w., & Xu, G.-z. (2022). Separation of three flavonoid glycosides from Polygonum multiflorum Thunb. leaves using HSCCC and their antioxidant activities. European Food Research and Technology, 248(1), 129–139. https://doi.org/10.1007/s00217-021-03865-0
- Cao, Y., Li, F., Luo, Y., Zhang, L., Lu, S., Xing, R., Yan, B., Zhang, H., & Hu, W. (2019). 20-Hydroxy-3-oxolupan-28-oic acid attenuates inflammatory responses by regulating PI3K–AKT and MAPKs signaling pathways in LPS-stimulated RAW264. 7 macrophages. Molecules, 24(3), 386. https://doi.org/10.3390/molecules24030386
- Cheng, B. C.-Y., Ma, X.-Q., Kwan, H.-Y., Tse, K.-W., Cao, H.-H., Su, T., Shu, X., Wu, Z.-z., & Yu, Z.-l. (2014). A herbal formula consisting of Rosae Multiflorae Fructus and Lonicerae Japonicae Flos inhibits inflammatory mediators in LPS-stimulated RAW 264.7 macrophages. Journal of Ethnopharmacology, 153(3), 922–927. https://doi.org/10.1016/j.jep.2014.02.029
- Endale, M., Kim, T.-H., Kwak, Y.-S., Kim, N.-M., Kim, S.-H., Cho, J. Y., Yun, B.-S., & Rhee, M.-H. (2017). Torilin inhibits inflammation by limiting TAK1-mediated MAP kinase and NF-κB activation. Mediators of Inflammation, 2017. https://doi.org/10.1155/2017/7250968
- Gong, G., Xie, F., Zheng, Y., Hu, W., Qi, B., He, H., Dong, T. T., & Tsim, K. W. (2020). The effect of methanol extract from Saussurea involucrata in the lipopolysaccharide-stimulated inflammation in cultured RAW 264.7 cells. Journal of Ethnopharmacology, 251, 112532. https://doi.org/10.1016/j.jep.2019.112532
- Gong, J., Chu, B., Gong, L., Fang, Z., Zhang, X., Qiu, S., Wang, J., Xiang, Y., Xiao, G., & Yuan, H. J. A. (2019). Comparison of phenolic compounds and the antioxidant activities of fifteen Chrysanthemum morifolium Ramat cv.‘Hangbaiju’in China. Antioxidants, 8(8), 325. https://doi.org/10.3390/antiox8080325
- Gong, L.-B., Wen, T., Li, Z., Xin, X., Che, X.-F., Wang, J., Liu, Y.-P., & Qu, X.-J. (2019). DYNC1I1 promotes the proliferation and migration of gastric cancer by up-regulating IL-6 expression. Frontiers in Oncology, 9(article 491), 1–18. doi:10.3389/fonc.2019.00491
- He, M. T., Park, H. S., Kim, Y. S., Lee, A. Y., & Cho, E. J. (2021). Protective effect of membrane-free stem cells against lipopolysaccharide and interferon-gamma-stimulated inflammatory responses in RAW 264.7 macrophages. International Journal of Molecular Sciences, 22(13), 6894. https://doi.org/10.3390/ijms22136894
- Hu, J.-W., Wu, J., Zhang, Y., Huang, B.-H., Fu, J.-P., Wu, L., & Xu, G. (2019). Chemical constituents of the stems of Gynura procumbens. Chemistry of Natural Compounds, 55(3), 583–585. https://doi.org/10.1007/s10600-019-02751-2
- Huang, X.-L., Li, X.-J., Qin, Q.-F., Li, Y.-S., Zhang, W. K., & Tang, H.-B. (2019). Anti-inflammatory and antinociceptive effects of active ingredients in the essential oils from Gynura procumbens, a traditional medicine and a new and popular food material. Journal of Ethnopharmacology, 239, 111916. https://doi.org/10.1016/j.jep.2019.111916
- Hwang, S. J., Kim, Y.-W., Park, Y., Lee, H.-J., & Kim, K.-W. (2014). Anti-inflammatory effects of chlorogenic acid in lipopolysaccharide-stimulated RAW 264.7 cells. Inflammation Research, 63(1), 81–90. https://doi.org/10.1007/s00011-013-0674-4
- Izuta, H., Narahara, Y., Shimazawa, M., Mishima, S., Kondo, S.-i., Hara, H. J. B., & Bulletin, P. (2009). 1, 1-diphenyl-2-picrylhydrazyl radical scavenging activity of bee products and their constituents determined by ESR. Biological and Pharmaceutical Bulletin, 32(12), 1947–1951. https://doi.org/10.1248/bpb.32.1947
- Jin, Y., Kim, H. P., Chi, M., Ifedigbo, E., Ryter, S. W., & Choi, A. M. (2008). Deletion of caveolin-1 protects against oxidative lung injury via up-regulation of heme oxygenase-1. American Journal of Respiratory Cell and Molecular Biology, 39(2), 171–179. https://doi.org/10.1165/rcmb.2007-0323OC
- Kaewseejan, N., Sutthikhum, V., & Siriamornpun, S. (2015). Potential of Gynura procumbens leaves as source of flavonoid-enriched fractions with enhanced antioxidant capacity. Journal of Functional Foods, 12, 120–128. https://doi.org/10.1016/j.jff.2014.11.001
- Kim, M.-S., & Kim, S.-H. (2011). Inhibitory effect of astragalin on expression of lipopolysaccharide-induced inflammatory mediators through NF-κB in macrophages. Archives of Pharmacal Research, 34(12), 2101–2107. https://doi.org/10.1007/s12272-011-1213-x
- Klimontov, V. V., Korbut, A. I., Orlov, N. B., Dashkin, M. V., & Konenkov, V. I. (2020). Multiplex bead array assay of a panel of circulating cytokines and growth factors in patients with albuminuric and non-albuminuric diabetic kidney disease. Journal of Clinical Medicine, 9(9), 3006. https://doi.org/10.3390/jcm9093006
- Ko, M.-J., Nam, H.-H., & Chung, M.-S. (2020). Subcritical water extraction of bioactive compounds from Orostachys japonicus A. Berger (Crassulaceae). Scientific Reports, 10(1), 1–10. https://doi.org/10.1038/s41598-019-56847-4
- Kumar, S., Sharma, S., & Vasudeva, N. (2017). Review on antioxidants and evaluation procedures. Chinese Journal of Integrative Medicine, 1–12. doi:10.1007/s11655-017-2414-z
- Li, X.-Y., Zhang, M.-T., Mu, Y.-M., Li, T.-T., Yang, Y.-L., Zeng, Z.-X., Li, Y.-S., & Zhou, H.-B. (2015). Quantitative analysis of multiple-components by a single marker of chlorogenic acid from Gynura procumbens (Lour.) Merr. Chinese Journal of Hospital Pharmacy, 35(22), 5. doi:10.13286/j.cnki.chinhosppharmacyj.2015.22.07
- Lu, Q., Sun, Y., Shu, Y., Tan, S., Yin, L., Guo, Y., & Tang, L. (2016). HSCCC separation of the two iridoid glycosides and three phenolic compounds from Veronica ciliata and their in vitro antioxidant and anti-hepatocarcinoma activities. Molecules, 21(9), 1234. https://doi.org/10.3390/molecules21091234
- Murugaiyah, V., Saeed, M. A. A., Kuong, Y.-M., Murugesu, K., Parasuraman, S., Asmawi, M. Z., & Sadikun, A. (2018). Lipid-lowering effect of hydroalcoholic extracts of Gynura procumbens in chemical-and high-fat diet-induced hyperlipidemic rats. Pharmacognosy Magazine, 14(55), 184. https://doi.org/10.4103/pm.pm_451_17
- Murugesu, K., Saghir, S. A. M., Sadikun, A., Khaw, K.-Y., & Murugaiyah, V. J. A. C. (2021). Exploiting column chemistry for chromatographic separation and quantification of caffeoylquinic acids in Gynura procumbens. Acta Chromatographica, 33(2), 170–178. https://doi.org/10.1556/1326.2020.00690
- Mustafa, I., Chin, N. L., Fakurazi, S., & Palanisamy, A. (2019). Comparison of phytochemicals, antioxidant and anti-inflammatory properties of sun-, oven-and freeze-dried ginger extracts. Foods, 8(10), 456. https://doi.org/10.3390/foods8100456
- Ning, T. J., Yusoff, S. D., Jubri, Z., Buang, F., Song, T. Z., Budiono, A., Jantan, I., Dianita, R., Kumolosasi, E., & Azmi, N. (2019). Inhibitory effects of Gynura procumbens ethanolic extract on nitric oxide production and inducible nitric oxide synthase (iNOS) protein expression in macrophages. Sains Malaysiana, 48(8), 1737–1744. https://doi.org/10.17576/jsm-2019-4808-20
- Park, C.-H., Min, S.-Y., Yu, H.-W., Kim, K., Kim, S., Lee, H.-J., Kim, J.-H., & Park, Y.-J. (2020). Effects of apigenin on RBL-2H3, RAW264. 7, and HaCaT cells: Anti-allergic, anti-inflammatory, and skin-protective activities. International Journal of Molecular Sciences, 21(13), 4620. https://doi.org/10.3390/ijms21134620
- Peng, Y., Hu, M., Lu, Q., Tian, Y., He, W., Chen, L., Wang, K., Pan, S. J. F., & Immunology, A. (2019). Flavonoids derived from Exocarpium Citri Grandis inhibit LPS-induced inflammatory response via suppressing MAPK and NF-κB signalling pathways. Food and Agricultural Immunology, 30(1), 564–580. https://doi.org/10.1080/09540105.2018.1550056
- Rosidah, Y. M., Sadikun, A., & Asmawi, M. (2008). Antioxidant potential of Gynura procumbens. Pharmaceutical Biology, 46(9), 616–625. https://doi.org/10.1080/13880200802179642
- Sava, A., Buron, F., Routier, S., Panainte, A., Bibire, N., Constantin, S. M., Lupașcu, F. G., Focșa, A. V., & Profire, L. (2021). Design, synthesis, in silico and in vitro studies for new nitric oxide-releasing indomethacin derivatives with 1, 3, 4-oxadiazole-2-thiol scaffold. International Journal of Molecular Sciences, 22(13), 7079. https://doi.org/10.3390/ijms22137079
- Seo, Y.-J., Lee, K.-T., Rho, J.-R., & Choi, J.-H. (2015). Phorbaketal A, isolated from the marine sponge Phorbas sp., exerts its anti-inflammatory effects via NF-κB inhibition and heme oxygenase-1 activation in lipopolysaccharide-stimulated macrophages. Marine Drugs, 13(11), 7005–7019. https://doi.org/10.3390/md13117005
- Shin, J. Y., Kang, J. S., Byun, H. W., & Ahn, E. K. (2017). Regulatory effects and molecular mechanism of Trigonostemon reidioides on lipopolysaccharide-induced inflammatory responses in RAW264. 7 cells. Molecular Medicine Reports, 16(4), 5137–5142. https://doi.org/10.3892/mmr.2017.7297
- Sun, L.-P., Shi, F.-F., Zhang, W.-W., Zhang, Z.-H., & Wang, K. (2020). Antioxidant and anti-inflammatory activities of safflower (Carthamus tinctorius L.) honey extract. Foods, 9(8), 1039. https://doi.org/10.3390/foods9081039
- Taira, J., Uehara, M., Tsuchida, E., Ohmine, W. J. J. o. a., & chemistry, f. (2014). Inhibition of the β-catenin/Tcf signaling by caffeoylquinic acids in sweet potato leaf through down regulation of the Tcf-4 transcription. Journal of Agricultural and Food Chemistry, 62(1), 167–172. https://doi.org/10.1021/jf404411r
- Tan, H.-L., Chan, K.-G., Pusparajah, P., Lee, L.-H., & Goh, B.-H. (2016). Gynura procumbens: An overview of the biological activities. Frontiers in Pharmacology, 7(article 52), 1–14. doi:10.3389/fphar.2016.00052
- Tantowi, N. A. C. A., Mohamed, S., Lau, S. F., & Hussin, P. (2020). Comparison of diclofenac with apigenin-glycosides rich Clinacanthus nutans extract for amending inflammation and catabolic protease regulations in osteoporotic-osteoarthritis rat model. DARU Journal of Pharmaceutical Sciences, 28(2), 443–453. https://doi.org/10.1007/s40199-020-00343-y
- Xiao, Y., Zheng, Y., Wu, S., Zhang, E.-H., Chen, Z., Liang, P., Huang, X., Yang, Z.-H., Ng, I.-S., & Chen, B.-Y. (2015). Pyrosequencing reveals a core community of anodic bacterial biofilms in bioelectrochemical systems from China. Frontiers in Microbiology, 6, 1410. https://doi.org/10.3389/fmicb.2015.01410
- Yang, F., Qi, Y., Liu, W., Li, J., Wang, D., Fang, L., & Zhang, Y. (2019). Separation of five flavonoids from aerial parts of Salvia miltiorrhiza bunge using HSCCC and their antioxidant activities. Molecules, 24(19), 3448. https://doi.org/10.3390/molecules24193448
- Yang, Y., Ding, Z., Wang, Y., Zhong, R., Feng, Y., Xia, T., Xie, Y., Yang, B., Sun, X., & Shu, Z. (2020). Systems pharmacology reveals the mechanism of activity of Physalis alkekengi L. var. franchetii against lipopolysaccharide-induced acute lung injury. Journal of Cellular and Molecular Medicine, 24(9), 5039–5056. https://doi.org/10.1111/jcmm.15126
- Yang, Y., Wu, J.-J., Cheng, C.-D., Bao, D.-J., Dong, Y.-F., Li, D.-X., Niu, W.-X., Zhou, C.-X., & Niu, C.-S. (2018). G-protein-coupled receptor kinase-5 promotes glioblastoma progression by targeting the nuclear factor kappa B pathway. American Journal of Translational Research, 10(11), 3370–3384.
- Yin, L., Wei, L., Fu, R., Ding, L., Guo, Y., Tang, L., & Chen, F. (2014). Antioxidant and hepatoprotective activity of Veronica ciliata Fisch. extracts against carbon tetrachloride-induced liver injury in mice. Molecules, 19(6), 7223–7236. https://doi.org/10.3390/molecules19067223