Abstract
Dyes exposure in aquatic environment creates risks to human health and biota due to their intrinsic toxic mutagenic and carcinogenic characteristics. In this work, a metal-organic frameworks materials, zeolitic imidazolate framework-8 (ZIF-8), was synthesized through hydrothermal reaction for the adsorptive removal of harmful Congo red (CR) from aqueous solution. Results showed that the maximum adsorption capacity of CR onto ZIF-8 was ultrahigh as 1250 mg g−1. Adsorption behaviors can be successfully fitted by the pseudo-second order kinetic model and the Langmuir isotherm equation. Solution conditions (pH condition and the co-exist anions) may influent the adsorption behaviors. The adsorption performance at various temperatures indicated the process was a spontaneous and endothermic adsorption reaction. The enhanced adsorption capacity was determined due to large surface area of ZIF-8 and the strong interactions between surface groups of ZIF-8 and CR molecules including the electrostatic interaction between external active sites Zn−OH on ZIF-8 -and −SO3 or –N=N– sites in CR molecule, and the π–π interaction.
1. Introduction
Nowadays, dyes and pigments are widely used in chemical industries, which may enter ecosystems (e.g. aquatic, soils) during dyes production and subsequent application processes.[Citation1] The occurrence of dyes may lead to severe water pollution problems.[Citation2] Dyes exposure in aquatic environment creates risks to human health and biota due to their intrinsic toxic, mutagenic and carcinogenic characteristics.[Citation2] Among various dye species, Congo red (CR) is a typical benzidine-based azo dye and mainly exists in the effluent of textile, paper, printing industries, etc.[Citation3] After entering natural environment, CR can be metabolized to benzidine, a well-known human carcinogen, which may be a cause for human allergic diseases. Due to its high chemical stability and low biodegradability, conventional biological process was found to be ineffective to decolorize and degrade CR wastewater treatment. Some physical-chemical techniques have been investigated for CR removal from aquatic systems, for example, sonophotocatalytisis, photolysis, coagulation/flocculation and adsorption.[Citation4–7] In all previous studied techniques, adsorption is regarded as a competitive one due to its simplicity, low cost and effectiveness and until now, various adsorbent materials have been examined for CR removal, including activated carbon, clay materials, modified biosorbents and synthetic materials.[Citation8–12]
Metal-organic frameworks (MOFs) represent a class of porous crystalline frameworks, and consist of metal ions (or clusters) and organic ligands.[Citation13] Due to its admirable properties (e.g. ultrahigh surface area, tunable pore structure and surface charge), MOFs have attracted worldwide concerns and been applied in various fields, for example, gas separation/recycle, catalysis, adsorbents and energy storage.[Citation14–17] Nowadays, adsorption and removing of various hazardous compounds from aquatic solution using MOFs are receiving increasing attention with the target pollutant to be both inorganic and organic. Adsorption mechanisms between pollutants and MOFs involve hydrophobic interactions, electrostatic enhancement and π–π interactions.[Citation18] Previous studies have reported that MOFs are promising in some dyes capture in aquatic system due to their high porosities, relatively heterogeneous surface and strong electrostatic interactions.[Citation19,20] However, there are only two studies investigating the adsorptive removal of CR from aquatic solution using different MOFs. Moradi et al. synthesized MIL-100(Fe) (an iron based MOF) which successfully achieved the adsorption capacity of 714 mg g−1 for CR.[Citation11] Khanjani and Morsali synthesized MOF-5 coating on silk fiber which could totally adsorb and remove 50 mg L−1 CR by adding 2 mg adsorbent into 50 mL dye solution.[Citation21]
Zeolitic imidazolate frameworks (ZIFs) are a set of MOF materials with excellent chemical and thermal stabilities that are topologically isomorphic with zeolites and contain functionalized organic links and a high density of transition metal ions.[Citation22,23] Among different ZIFs reported, zeolitic imidazolate framework-8 (ZIF-8) targeting to a series of materials has a sodalite-related zeolite type structure containing narrow six membered-ring pore windows and contains explored Zn2+–N− linkers and Zn and N− extremities on the surface.[Citation22] Different synthesizing methods can be used to produce ZIF-8 materials using solvothermal, microwave-assisted, sonochemical, mechanochemical, dry-gel, and microfluidic methods.[Citation24] The gained ZIF-8 may possess varied physical-chemical properties resulted from different synthesized method.[Citation25] As reported, it is a potential candidate for adsorption/separation applications. Earlier investigations have demonstrated that ZIF-8 has a high performance in gas storage and can effectively separate organic molecules in aquatic solution.[Citation16,26] In contrast, to date, only Fan et al. [Citation16] reported the dye molecule adsorption onto ZIF-8, who found that the uptake capacity of ZIF-8 sythesized from surfactant mediated method for rhodamine B (a cationic dye) was very low and the external adsorption between ZIF-8 and rhodamine B molecules was negligible. The low adsorption capacity is attributed to the larger molecule size of rhodamine B than the pore aperture size of ZIF-8 (3.4 Å), and to the weak specific interaction between functional groups of ZIF-8 and rhodamine B molecule.[Citation27,28]
Current study aims to examine the ZIF-8 nanoparticles synthesized through hydrothermal reaction for adsorption of anionic CR molecules from aquatic solution. The adsorption was studied by conducting batch kinetic and equilibrium experiments. Adsorption kinetics, adsorption isotherms and thermodynamics of CR onto synthesized ZIF-8 were thus systematically investigated. Furthermore, the effects of pH and competition anions were analyzed and the adsorption mechanism was characterized.
2. Materials and methods
2.1. Experimental material preparation
The synthesis of ZIF-8 was performed using ZnCl2 as metal source, C4H6N2 as organic linker, methanol as solvent and CHNaO2 as inducing agent. Firstly, the ZnCl2 (548 mg), CHNaO2 (283 mg) and C4H6N2 (667 mg) were dissolved in methanol (50 mL) together and dispersed using ultrasonic. The synthesis solution was filled in Teflon lined stainless steel autoclave and heated at 393 K for 5 h. The mixture was cooled to room temperature. Then, the precipitated white powder of ZIF-8 was collected after centrifugation (4000 rpm, 20 min) and washed with methanol for three times. The ZIF-8 sample was oven dried at 80 °C prior to using. Commonly used adsorbent zeolite (diameter <0.42 mm) and granular activated carbon (diameter <1 mm) were selected as references in CR removal experiments, which were purchased from Sinopharm Chemical Reagent Co. (Shanghai, China).
The CR was purchased from Aladdin Industrial Co. (Shanghai, China). The molecular structure and selective physical properties of CR were shown in Supplementary materials (Figure S1 and Table S1).The CR stock solution was prepared with ultrapure water, and the desired concentrations of the experimental solutions were obtained through successive dilutions. The concentration of CR was determined by using a UV–visible spectrophotometer at maximum absorbance of 496.5 nm (Shimadzu UV spectrophotometer, UV-1800).
2.2. Adsorption procedure
For conducting the sorption experiment, 5 mg ZIF-8 was added into 20 mL CR solutions with different initial concentrations (25 mL glass vials). Vials were shaken (230 rpm, 30 °C) for certain time intervals. After that, the suspension was centrifuged (12000 rpm, 5 min) and filtered with 0.45 μm membrane prior to determination of the CR concentration.
For studying the batch adsorption equilibrium and the time effect on the adsorption of CR onto ZIF-8, every 5 mg of adsorbent was added into a series of 20 mL CR solution (80–250 mg L−1). Samples were collected at certain time intervals. Relatively long adsorption duration (24 h) was set in preliminary experiments.
The percentage removal (%) and uptake amount, q (mg g−1), were calculated using Equations (1) and (2), respectively:
(1)
(2)
where Ci is initial concentration of CR (mg L−1); Ce is equilibrium concentration of CR (mg L−1); V is solution volume (mL); and m is the weight of ZIF-8 (mg).
2.3. Adsorption kinetics of CR adsorption
To determine the adsorption kinetic, 5 mg ZIF-8 was added into a series of CR solutions (20 mL) with the same initial concentrations. The CR concentrations left in different vials were detected after shaking in predetermined time intervals. The kinetic characteristics of adsorption process were described by pseudo first-order rate equation (Equation (3)) and binuclear pseudo-second order equation described below (Equation (4)).[Citation29,30](3)
(4)
where t is the contact time (min); k1 is the first order adsorption rate constant (min−1); k2 is the second order adsorption rate constant (g mg−1 min−1); qe and qt is the uptake amount at equilibrium and time t, respectively (mg g−1).
2.4. Adsorption isotherm of CR adsorption
To conduct the adsorption isotherm experiment, 5 mg ZIF-8 was added into CR solutions (20 mL) with different initial concentrations (120–1046 mg L−1, pH 7.8, 30 °C, 700 min). Langmuir isotherm (Equation (5)) and Freundlich isotherm (Equation (7)) were selected for analyzing adsorption isotherm.[Citation31,32] Langmuir isotherm is valid for monolayer adsorption on a surface owning to finite identical sites and Freundlich isotherm assumes that the adsorption of dye molecule occurs on a heterogeneous surface by multilayer adsorption.[Citation33](5)
where qe is the uptake amount of CR at equilibrium (mg g−1); Ce is the equilibrium concentration of CR (mg L−1); Qm and b are the Langmuir constants related to maximum adsorption capacity and rate of adsorption, respectively. The essential characteristics of the Langmuir isotherm can be expressed in terms of a dimensionless separation factor (RL):(6)
where C0 is the highest CR concentration (mg L−1). The value of RL indicates the type of the isotherm to be either unfavorable (RL > 1), linear (RL = 1), favorable (0 < RL < 1) or irreversible (RL = 0). The Frendlich isotherm was described as (7)
where KF and 1/n are Frendlich equilibrium constants indicative of adsorption capacity and adsorption intensity respectively. Value of n > 1 represents a favorable adsorption condition. 1/n and KF were obtained by plotting ln qe against ln Ce.
2.5. Thermodynamic of CR adsorption
Five mg ZIF-8 was added into CR solutions (20 mL) with an initial concentration of 640 mg L−1 at different temperatures (293, 303 and 313 K). Thermodynamic parameters of standard Gibbs free energy (ΔG°), standard enthalpy (ΔH°) and standard entropy (ΔS°) were determined. The amounts of ΔH° and ΔS° could be calculated from the slope and intercept of the straight line obtained by plotting ln Kd against 1/T using Equations (8)–(10).[Citation34](8)
where R is the gas constant (8.314 J mol−1 K−1) and T is the absolute temperature of the aqueous solution (K). Kd is the distribution coefficient, which is mass-weighted partition coefficient between solid phase and liquid supernatant phase (mL g−1) reflecting the selectivity for objective pollutant, calculated according to the formula:(9)
where C0 and Ct are the concentrations of CR at contact time of 0 (initial concentration) and t, respectively (mg L−1). V is the volume of solution (mL) and M is the mass of adsorbent used (g).
After obtaining ΔH° and ΔS° values of the adsorption, ΔG° of each temperature was calculated as follows:(10)
2.6. Conditional adsorption experiments
To analyze the effect of solution pH on the adsorption, the pH of CR solution was adjusted with 0.1 M HCl and NaOH to get the final pH of 4, 6, 8 and 12 (initial CR concentration, 625 mg L−1, 30 °C). The effects of competition ions on the adsorption were evaluated by addition of 0.02 M NaCl, Na2SO4, NaHCO3 and Na2C2O4 to the aqueous solution (initial CR concentration, 583 mg L−1, 30 °C). For studying the effect of CR adsorption on the zeta potential variation of ZIF-8, every 5 mg adsorbent was put into 20 mL CR solution at various initial concentrations of 0, 40, 80 and 100 mg L−1 (pH 7.8, 30 °C). After 700 min, the total amount of CR in solution was transferred onto ZIF-8 with adsorption amount of 0, 160, 320, 400 mg g−1, respectively. The final zeta potentials of ZIF-8 loaded with different amount of CR were measured with Zeta Potential Analyzer (Malvern Zetasizer Nanao ZS). Furthermore, the UV–vis spectra of CR solution after adsorption for different time intervals (initial CR concentration, 120 mg L−1, 30 °C) were also determined by UV spectrophotometer (Shimadzu, UV-1800). In order to investigate the regeneration of the adsorbent, CR-loaded ZIF-8 was washed with 0.05 mol L−1 NaOH after the adsorption (pH 4, C0 = 400 mg L−1, 6 h, 30 °C) and was shaken with another 20 mL 0.05 mol L−1 NaOH for 4 h. After that, the desorbed ZIF-8 was washed with deionized water and was dried at 60 °C for the next adsorption test. The adsorption-desorption cycle was repeated for three times.
2.7. Characteristics analysis of adsorbents
The morphology of the samples (before and after adsorption) were measured with a scanning electron microscopic (SU8010, Hitachi, Japan). A thin Pt film was loaded to the samples with ion sputter coating equipment (MC1000, Hitachi) prior to the SEM detection. The composition and phase of the as-prepared products before and after adsorption of CR were evaluated by powder X-ray diffractometer (XRD, X’Pert PRO, PANalytical Co., Netherlands) employing Cu-Kα radiation at a scan rate of 2° min−1. The generator voltage was 40 kV and the tube current was 40 mA. The Nitrogen adsorption isotherms were obtained using a surface area and porosity analyzer (Micromeritics, Tristar II 3020) at 77 K. Prior to measurements, the samples were heated at 150 °C for 12 h. The Fourier transform infra-red (FTIR) spectra of the ZIF-8 samples (before and after adsorption) and CR were obtained using a Jasco FTIR-4100 spectrometer from 400 to 4000 cm−1. The textural properties of the synthesized ZIF-8 were similar to those of previous reports.[Citation25,35]
3. Results and discussion
3.1. Adsorption kinetics of CR onto ZIF-8
The adsorption performance of the ZIF-8 for removing CR in water was evaluated. Figure showed the time profiles of the CR removal with ZIF-8 at various initial CR concentrations. It was clear that the adsorption rate was initially fast and then gradually decreased to reach equilibrium.
Two kinetic models, pseudo-first order model and pseudo-second order model were selected to study the adsorption kinetic of CR onto ZIF-8. Obviously, Pseudo-second order model (Figure (b)) could fit the experimental results better than pseudo-first order model (Figure (a)). Table showed the kinetic parameters for different initial concentrations of CR obtained utilizing two adsorption kinetic models. The pseudo-second order model generated a high degree of fitting (R2 > 0.9918). The rate constants, k2 for pseudo-second order decreased with the increase of initial concentration of CR (80–200 mg L−1). This phenomenon is probably due to the lower competition for the adsorption sites at lower initial concentration of CR.[Citation8] Similarly, the competition for the surface active sites will be stronger at higher initial concentration and the adsorption rates were consequently decreased.[Citation36] The pseudo-second order model is based on the assumption that the rate-limiting factor in the adsorption was determined by chemic-sorption. Thus, the CR adsorption process onto synthesized ZIF-8 was a chemisorption rather than physical sorption.
Figure 2. Kinetic modeling of the adsorption: (a) pseudo first order, (b) pseudo second order diffusion models.
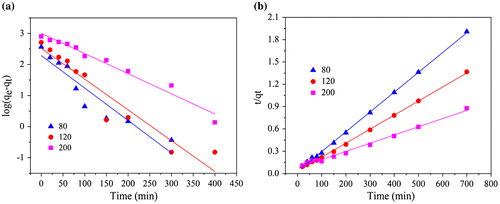
Table 1. Kinetic parameters for adsorption of CR dye using ZIF 8.
3.2. Adsorption isotherms of CR onto ZIF-8
The adsorption isotherm of CR adsorption onto ZIF-8 was showed in Figure (a). Langmuir and Freundlich isotherms were widely used in describing adsorption organic pollutants onto porous materials. In current study, Freundlich isotherm was failed in fitting adsorption data during the whole experimental period. According to experiences of previous studies on adsorption of pollutants onto ZIF-8, the Langmuir isotherm model was selected to explain how adsorption takes place for CR onto ZIF-8.[Citation27,37] In Langmuir fitting (Figure (b)), results of high degree of linear fitting between Ce/qe vs. Ce were obtained (R2 = 0.9998). The fitting of the model was highly significant as it suggests the monolayer coverage of the CR on the surface of the ZIF-8. The Qm at the experimental condition for CR onto ZIF-8 (pH 7.8) is found to be 1250 mg g−1.
Figure 3. (a) Adsorption isotherm of CR onto ZIF-8; (b) adsorption isotherm of CR onto ZIF-8 fitted by Langmuir isotherm equation.
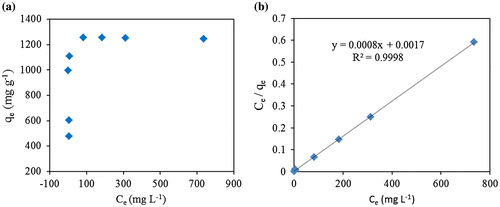
The separation factor of RL was calculated using Equation (6) (Figure S2). All RL values were in the range of 0–1, which pointed to the favorable adsorption of CR over ZIF-8. It has been observed that the RL values decrease with the initial concentration increase from 156 to 1046 mg L−1, indicating the adsorption was more favorable at higher initial concentrations.
The maximum CR removal capacity of the ZIF-8 (1250 mg g−1) showed a high adsorption capacity among the previous reported values. Maximum adsorption capacities among various types of adsorbent for CR were compared in Table and the ZIF-8 synthesized in present study performed a relatively attractive adsorption capacity.
3.3. Thermodynamic of CR adsorption onto ZIF-8
Thermodynamic parameters for the adsorption of CR on ZIF-8 at different temperatures were shown in Table . The distribution coefficient of Kd increased remarkably with the increase of temperature (293–313 K), indicating that high temperature is favorable for the selective adsorption of CR. The same phenomenon was also observed by Reddy et al., who employed jujube seeds to adsorb CR from aqueous solution.[Citation40] The positive value of ΔS0 indicated an increase in disorder of solid-liquid interface of material during adsorption of dye.[Citation9] The negative values of ΔG0 and the positive values of ΔH0 revealed that the adsorption process of CR onto the ZIF-8 surface was a spontaneous and endothermic adsorption reaction.
Table 2. Thermodynamic parameters for the adsorption of CR on ZIF-8 at different temperatures.
3.4. Characteristics of ZIF-8 before and after adsorption
The XRD analysis was performed for ZIF-8 samples before and after adsorption (Figure (a)). All characteristics peaks are highly consistent with the simulated pattern of ZIF-8 and published patterns for synthesized ZIF-8 in previous studies.[Citation37,41] The crystallized structure of ZIF-8 was stable after the adsorption by comparing XRD patterns for original and CR loaded ZIF-8. The FTIR spectra proved the boundary surface functional groups on ZIF-8.[Citation35] Different from the XRD patterns, the FTIR spectra of pristine and CR loaded ZIF-8 illustrated in Figure (b) showed some variations. The peak at 420 cm−1 assign to Zn–N mode in ZIF-8 which could generate Zn–OH and N–H groups after dissociative adsorption of water.[Citation42] Therefore, the broad peak at 3440 in spectra of CR loaded ZIF-8 is ascribed to the vibration of N–H and OH groups.[Citation38]
Figure 4. (a) XRD pattern of pristine ZIF-8 and CR loaded ZIF-8; (b) FTIR spectra of pristine ZIF-8 and CR loaded ZIF-8; (c) SEM micrographs of pristine ZIF-8; (d) SEM micrograph of CR loaded ZIF-8.
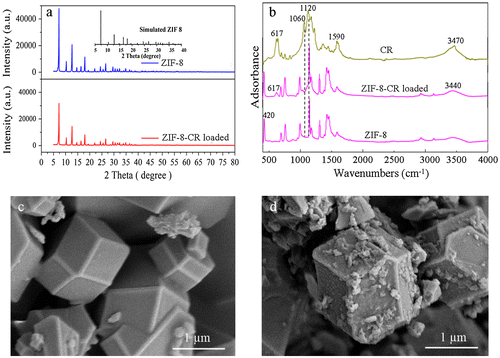
The intense peak at 619 nm in spectra of CR and ZIF-8 after adsorption is assigned to –SO3.[Citation43] Furthermore, the variation peak at 1060 also assigned to S=S stretching vibrations in CR could still be observed in spectra of CR-loaded ZIF-8 but it shifted to some higher wave numbers (1060–1120 cm−1).[Citation44] Variations above indicated the successful interaction of –SO3 in CR onto Zn–OH sites of ZIF-8 by ion exchange. The bond at 1590 cm−1 attributed to C=C in benzene in CR structure diminishes in ZIF-8 after adsorption could be related to the π–π interaction between benzene rings of CR and the aromatic imidazole rings of ZIF-8.[Citation12]
The SEM images visually displayed a crystalline structure of the ZIF-8 and the alterations between original and CR-loaded ZIF-8 (Figure (c) and (d)), showing that the ZIF-8 was coated with dye molecules after the adsorption process. The dye molecules seem to have formed a film covering the ZIF-8 particles and porosity in aggregates. Fan et al. [Citation16] found that it was unable for Rhodamine B to enter the pores in ZIF-8 and just surface sorption occurred since the molecule size of Rhodamine B at 13.4 Å was larger than the aperture size of ZIF-8 at 3.4 Å. However, they further found that the adsorption capacity was very low although the SSA of the synthesized ZIF-8 was as high as 1256 m2 g−1. In this study, the molecular size of CR is 26.6 Å (Table S1, Supplementary materials) which is much larger than the Rhodamine B molecule, and thus the CR could not enter the pores in ZIF-8 structure.[Citation45] The adsorption of large CR molecule depends on the external surface area of the ZIF-8 and the average primary particle size of ZIF-8 was around 0.93 μm (Figure S3).[Citation46] The large adsorption capacity may be attributed to the strong adsorption of CR on the surface of ZIF-8, where surface chemical interactions (e.g. through functional groups) between ZIF-8 and CR could not be negligible for the adsorption process. Our result was also consistent with a previous study from Tanaka et al. who found the adsorption capacities of Rhodamine B onto monocrystal and polycrystal ZIF-8 could reach 9.0 and 8.8 μmol g−1, respectively mainly through the adsorption occurring on the external surfaces and grains boundaries of ZIF-8 particles.[Citation46]
3.5. Conditional adsorption properties
ZIF-8 contains different functional groups which possess different charges. The zeta potential of ZIF-8 remained positive at pH below around 10 and varied to negative at higher pH values was found in previous studies.[Citation26,27] The changes of electricity properties of ZIF-8 were detected before and after adsorption by analyzing its zeta potential. It should be noted that the adsorbed CR anions brought negative zeta potential onto ZIF-8 (Figure (a)). The linear fitting between adsorbed amount of CR and the variation in zeta potential of ZIF-8 (Zetafinal − Zetaoriginal) was satisfied (R2 = 0.9556). This proves that the adsorption occurs between the negatively charged sites in dye anions and positively charged sites of ZIF-8. Besides, the π–π interactions between benzene or naphthalene rings of CR with the aromatic imidazole rings of ZIF-8 should also be considered for the adsorption of CR onto ZIF-8.[Citation27,42]
Figure 5. (a) The effect of the amount of adsorbed CR on the changes in zeta potential of ZIF-8 (pH 7.8); (b) UV–vis detection for CR removal efficiency at different time (initial concentration, 120 mg L−1); (c) Effect of competition anions on the adsorption capacities; (d) Effect of initial pH on the adsorption.
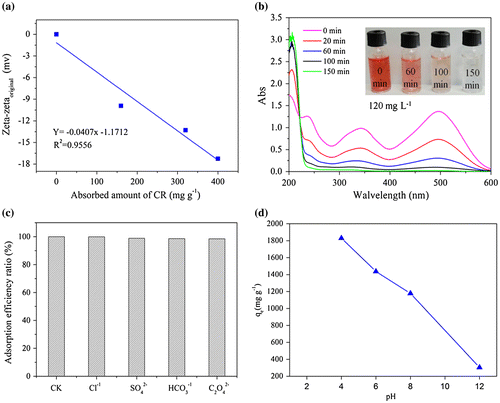
In the UV–vis spectra of CR solutions (Figure (b)), a main peak at around 496 nm is corresponding to chromophore structure next to the azo agent. Peaks located at 347 and 235 nm were assigned to the naphthalene and benzoic rings, respectively. There was no obvious horizontal shift for these peaks during the whole adsorption period. The spectra also prove high solvent stability of ZIF-8 structure after dispersed in water during the adsorption process, which agrees with the results of XRD patterns. Figure (b) also reveals that the removal rate of CR using ZIF-8 is extremely high because the color of 120 mg L−1 CR solution is almost colorless after adsorption for 150 min. Various anions coexist with CR in wastewater, and some common anions (Cl−, SO42−, HCO3−, and C2O42−) were selected to analyze their effects on the removal efficiency (Figure (c)), showing that coexisting mono or di-valent anions have no significant effects on the adsorption capacities.
As an acidic dye, CR contains negatively charged sulfonated group, and pH may play an important role in the active sites on ZIF-8 and in the dye speciation during the adsorption process. The adsorption efficiency of CR on ZIF-8 decreased with the increase of pH (Figure (d)). It can be seem that, the adsorption effect still occurred when the ZIF-8 was negatively charged at pH 12 (point zero charge at pH 10). Negatively charge on the adsorbent increased following the pH increased, which does not favor the adsorption of the anionic dye due to the electrostatic repulsion.[Citation11] This is similar to the previous finding with other adsorbents.[Citation38]
The regeneration ability of ZIF-8 after adsorption was shown in Figure S4. It was apparent that the adsorption capacity was decreased to 44% in the second run and was further decreased to 18% in the third run. The loss of the adsorption capacity may be resulted from several reasons. Firstly, some inactive sites on ZIF-8 were occupied by some undesorbed CR molecules due to the incompletely desorption. Secondly, some minor collapse of the crystalline structure of ZIF-8 may be happened during the continued adsorption-desorption cycles.[Citation27]
3.6. Comparison of adsorption capacities of ZIF-8 with other adsorbents
The adsorption efficiency of ZIF-8 for CR was firstly compared with activated carbon and zeolite under the same adsorption conditions (5 mg adsorbent into 20 mL CR solution, initial CR concentration of 80 mg L−1, pH 7.8, 230 rpm, 30 °C). As showed in Figure , the adsorption efficiency of ZIF-8 was much higher than the other two adsorbents.
Figure 6. Comparison of CR adsorption behavior among zeolite, activated carbon and ZIF-8.
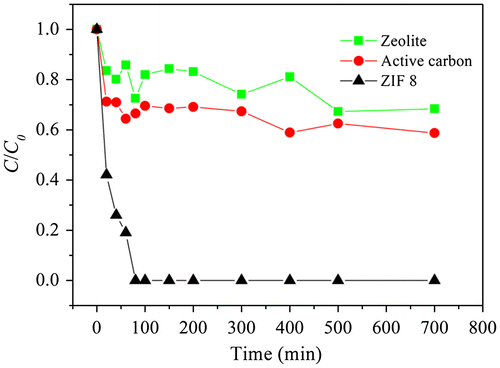
The CR concentration almost decreased to zero after 60 min following ZIF-8 addition, while the final CR concentrations were 55.7 and 47.0 mg L−1 when adding zeolite and activated carbon as the sorbents, respectively. The SSA of ZIF-8 at 1256 m2 g−1 was much higher than zeolite and activated carbon (57 and 372 m2 g−1, respectively, Table S2). And also, the pore volume for ZIF-8 at 0.6 cm3 g−1 was larger than those for zeolite and activated carbon (0.14 and 0.37 cm3 g−1, respectively). Generally, a larger SSA and pore volume are favorable for the adsorption process between adsorbent and CR in solution.[Citation9] For the three adsorbents, the adsorption processes increased rapidly at the initial stages. This trend could be attributed to the large vacant surface sites for interaction between different adsorbents and CR molecule.[Citation36]
Typically, the ZIF-8 used in current study possessed better adsorption performance than other previous studied MOFs, MIL-100 (714 mg g−1) and silk fibers coating MOF-5 (calculated adsorption capacity was aroud 1250 mg g−1), even though the specific surface area (2800 m2 g−1) for MIL-100 was larger than twice for ZIF-8.[Citation11,21] It can also be revealed from Table that there was no certain relationship between listed SSA of various adsorbents and the corresponding adsorption capacities. Therefore, the large adsorption capacity of CR onto the ZIF-8 was dominated both of the large SSA of the adsorbent and the chemical interactions between the surface functional groups on ZIF-8 and CR molecules including the strong electrostatic interaction between external active sites Zn–OH on ZIF-8 and –SO3 or –N=N– sites in CR molecule, and the π–π interaction.
Table 3. Comparison of maximum adsorption capacities of various adsorbents for CR.
4. Concluding remarks
ZIF-8 can be used for efficient adsorption of CR from aqueous solution. The fast adsorption kinetics, high adsorption capacity and solvent stability of ZIF-8 were observed. Although the molecular size of CR is much larger than pore aperture in ZIF-8, the ultra-high adsorption efficiency is attributed to the hydration process of ZIF-8 to form a surface hydrogen group by dissociative adsorption of water which benefits the adhesion of CR anionic species tightly to the surface of ZIF-8 as well as the π–π interaction between CR and ZIF-8. Furthermore, ZIF-8 exhibits a wide applicable pH range (4–12) and the adsorption efficiency decreases with the increase of pH. The coexisting mono or di-valent anions have no evident effects on adsorption capacities. Therefore, ZIF-8 could be an efficient adsorbent for the removal of CR from aquatic system and is a new choice for CR removal in practical wastewater treatment.
Supplemental data
Supplemental data for this article can be accessed here.
Funding
This work was supported by the National Natural Science Foundation of China under [grant number 41501516]; the Natural Science Foundation of Jiangsu Province, China under [grant number BK20150682]; and the Research Foundation of Jiangsu Environmental Protection Department, China under [grant number TH2014210].
supplementary_material-revised.doc
Download MS Word (339.5 KB)Acknowledgments
We would particularly like to thank Dr. C. Kong and Mr. N. Gu from Ningbo institute of Materials Technology and Engineering, Chinese Academy of Sciences for their skillful help in synthesizing ZIF-8 particles.
References
- Bhattacharyya R, Ray SK. Removal of Congo red and methyl violet from water using nano clay filled composite hydrogels of poly acrylic acid and polyethylene glycol. Chem. Eng. J. 2015;260:269–283.10.1016/j.cej.2014.08.030
- Vimonses V, Jin B, Chow CWK. Insight into removal kinetic and mechanisms of anionic dye by calcined clay materials and lime. J. Hazard. Mater. 2010;177:420–427.10.1016/j.jhazmat.2009.12.049
- Han R, Ding D, Xu Y, et al. Use of rice husk for the adsorption of Congo red from aqueous solution in column mode. Bioresour. Technol. 2008;99:2938–2946.10.1016/j.biortech.2007.06.027
- Bejarano-Pérez NJ, Suárez-Herrera MF. Sonophotocatalytic degradation of Congo red and methyl orange in the presence of TiO2 as a catalyst. Ultrason. Sonochem. 2007;14:589–595.10.1016/j.ultsonch.2006.09.011
- Zhu H, Jiang R, Xiao L, et al. Photocatalytic decolorization and degradation of Congo red on innovative crosslinked chitosan/nano-CdS composite catalyst under visible light irradiation. J. Hazard. Mater. 2009;169:933–940.10.1016/j.jhazmat.2009.04.037
- Vijayaraghavan G, Shanthakumar S. Performance study on algal alginate as natural coagulant for the removal of Congo red dye. Desalin. Water Treat. 2016;57:6384–6392.
- Bhaumik M, McCrindle R, Maity A. Efficient removal of Congo red from aqueous solutions by adsorption onto interconnected polypyrrole–polyaniline nanofibres. Chem. Eng. J. 2013;228:506–515.10.1016/j.cej.2013.05.026
- Namasivayam C, Kavitha D. Removal of Congo red from water by adsorption onto activated carbon prepared from coir pith, an agricultural solid waste. Dyes Pigm. 2002;54:47–58.10.1016/S0143-7208(02)00025-6
- Vimonses V, Lei S, Jin B, et al. Kinetic study and equilibrium isotherm analysis of Congo red adsorption by clay materials. Chem. Eng. J. 2009;148:354–364.10.1016/j.cej.2008.09.009
- Zhu HY, Fu YQ, Jiang R, et al. Adsorption removal of Congo red onto magnetic cellulose/Fe3O4/activated carbon composite: equilibrium, kinetic and thermodynamic studies. Chem. Eng. J. 2011;173:494–502.10.1016/j.cej.2011.08.020
- Moradi SE, Dadfarnia S, Haji Shabani AM, et al. Removal of Congo red from aqueous solution by its sorption onto the metal organic framework MIL-100(Fe): equilibrium, kinetic and thermodynamic studies. Desalin. Water Treat. 2014;1–13.
- Wu L, Liu Y, Zhang L, et al. A green-chemical synthetic route to fabricate a lamellar-structured Co/Co(OH)2 nanocomposite exhibiting a high removal ability for organic dye. Dalton Trans. 2014;43:5393–5400.10.1039/c3dt53369g
- Hu H, Liu S, Chen C, et al. Two novel zeolitic imidazolate frameworks (ZIFs) as sorbents for solid-phase extraction (SPE) of polycyclic aromatic hydrocarbons (PAHs) in environmental water samples. The Analyst. 2014;139:5818–5826.10.1039/C4AN01410C
- Yan Q, Lin Y, Kong C, et al. Remarkable CO2/CH4 selectivity and CO2 adsorption capacity exhibited by polyamine-decorated metal-organic framework adsorbents. Chem. Commun. 2013;49:6873–6875.10.1039/c3cc43352h
- Vermoortele F, Ameloot R, Alaerts L, et al. Tuning the catalytic performance of metal-organic frameworks in fine chemistry by active site engineering. J. Math. Chem. 2012;22:10313–10321.10.1039/c2jm16030g
- Fan X, Wang W, Li W, et al. Highly Porous ZIF-8 nanocrystals prepared by a surfactant mediated method in aqueous solution with enhanced adsorption kinetics. ACS Appl. Mater. Interfaces. 2014;6:14994–14999.
- Lin Y, Zhang Q, Zhao C, et al. An exceptionally stable functionalized metal-organic framework for lithium storage. Chem. Commun. 2015;51:697–699.10.1039/C4CC07149B
- Hasan Z, Jhung SH. Removal of hazardous organics from water using metal-organic frameworks (MOFs): plausible mechanisms for selective adsorptions. J. Hazard. Mater. 2015;283:329–339.10.1016/j.jhazmat.2014.09.046
- Li L, Li JC, Rao Z, et al. Metal organic framework [Cu3 (BTC)2 (H2O)3] for the adsorption of methylene blue from aqueous solution. Desalin. Water Treat. 2014;52:7332–7338.10.1080/19443994.2013.821955
- Xiao L, Xiong Y, Tian S, et al. One-dimensional coordination supramolecular polymer [Cu(bipy)(SO4)]n as an adsorbent for adsorption and kinetic separation of anionic dyes. Chem. Eng. J. 2015;265:157–163.10.1016/j.cej.2014.11.134
- Khanjani S, Morsali A. Ultrasound-promoted coating of MOF-5 on silk fiber and study of adsorptive removal and recovery of hazardous anionic dye “congo red”. Ultrason. Sonochem. 2014;21:1424–1429.10.1016/j.ultsonch.2013.12.012
- Park KS, Ni Z, Cote AP, et al. Exceptional chemical and thermal stability of zeolitic imidazolate frameworks. Proc. Nat. Acad. Sci. 2006;103:10186–10191.10.1073/pnas.0602439103
- Wang B, Côté AP, Furukawa H, et al. Colossal cages in zeolitic imidazolate frameworks as selective carbon dioxide reservoirs. Nature. 2008;453:207–211.10.1038/nature06900
- Lee YR, Jang MS, Cho HY, et al. ZIF-8: a comparison of synthesis methods. Chem. Eng. J. 2015;271:276–280.10.1016/j.cej.2015.02.094
- Wu YN, Zhou M, Zhang B, et al. Amino acid assisted templating synthesis of hierarchical zeolitic imidazolate framework-8 for efficient arsenate removal. Nanoscale. 2014;6:1105–1112.10.1039/C3NR04390H
- Khan NA, Jung BK, Hasan Z, et al. Adsorption and removal of phthalic acid and diethyl phthalate from water with zeolitic imidazolate and metal-organic frameworks. J. Hazard. Mater. 2014;282:194–200.
- Li J, Wu YN, Li Z, et al. Zeolitic imidazolate framework-8 with high efficiency in trace arsenate adsorption and removal from water. J. Phys. Chem. C. 2014;118:27382–27387.10.1021/jp508381m
- Kwon HT, Jeong HK. Highly propylene-selective supported zeolite-imidazolate framework (ZIF-8) membranes synthesized by rapid microwave-assisted seeding and secondary growth. Chem. Commun. 2013;49:3854–3856.10.1039/c3cc41039k
- Ho YS, McKay G, Wase DAJ, et al. Study of the sorption of divalent metal ions on to peat. Adsorpt. Sci. Technol. 2000;18:639–650.10.1260/0263617001493693
- Wang MX, Zhang QL, Yao SJ. A novel biosorbent formed of marine-derived Penicillium janthinellum mycelial pellets for removing dyes from dye-containing wastewater. Chem. Eng. J. 2015;259:837–844.10.1016/j.cej.2014.08.003
- Qin FX, Jia SY, Liu Y, et al. Adsorptive removal of bisphenol A from aqueous solution using metal-organic frameworks. Desalin. Water Treat. 2015;54:93–102.10.1080/19443994.2014.883331
- Yang K, Zhu L, Xing B. Sorption of phenanthrene by nanosized alumina coated with sequentially extracted humic acids. Environ. Sci. Pollut. Res. 2010;17:410–419.10.1007/s11356-009-0163-z
- Hameed BH, Rahman AA. Removal of phenol from aqueous solutions by adsorption onto activated carbon prepared from biomass material. J. Hazard. Mater. 2008;160:576–581.10.1016/j.jhazmat.2008.03.028
- Ding D, Zhao Y, Yang S, et al. Adsorption of cesium from aqueous solution using agricultural residue – walnut shell: equilibrium, kinetic and thermodynamic modeling studies. Water Res. 2013;47:2563–2571.10.1016/j.watres.2013.02.014
- Jing HP, Wang CC, Zhang YW, et al. Photocatalytic degradation of methylene blue in ZIF-8. RSC Adv. 2014;4:54454–54462.10.1039/C4RA08820D
- Dawood S, Sen TK. Removal of anionic dye Congo red from aqueous solution by raw pine and acid-treated pine cone powder as adsorbent: equilibrium, thermodynamic, kinetics, mechanism and process design. Water Res. 2012;46:1933–1946.10.1016/j.watres.2012.01.009
- Jiang JQ, Yang CX, Yan XP. Zeolitic imidazolate framework-8 for fast adsorption and removal of benzotriazoles from aqueous solution. ACS App. Mater. Interfaces. 2013;5:9837–9842.10.1021/am403079n
- Wang L, Wang A. Adsorption properties of Congo red from aqueous solution onto surfactant-modified montmorillonite. J. Hazard. Mater. 2008;160:173–180.10.1016/j.jhazmat.2008.02.104
- Toor M, Jin B, Dai S, et al. Activating natural bentonite as a cost-effective adsorbent for removal of Congo-red in wastewater. J. Ind. Eng. Chem. 2015;21:653–661.10.1016/j.jiec.2014.03.033
- Reddy MCS, Sivaramakrishna L, Reddy AV. The use of an agricultural waste material, Jujuba seeds for the removal of anionic dye (Congo red) from aqueous medium. J. Hazard. Mater. 2012;203–204:118–127.10.1016/j.jhazmat.2011.11.083
- Wang S, Fan Y, Jia X. Sodium dodecyl sulfate-assisted synthesis of hierarchically porous ZIF-8 particles for removing mercaptan from gasoline Chem. Eng. J. 2014;256:14–22.
- Chizallet C, Lazare S, Bazer-Bachi D, et al. Catalysis of transesterification by a nonfunctionalized metal-organic framework: acido-basicity at the external surface of ZIF-8 probed by FTIR and ab initio calculations. J. Am. Chem. Soc. 2010;132:12365–12377.10.1021/ja103365s
- Rimbu GA, Stamatin I, Jackson CL, et al. The morphology control of polyaniline as conducting polymer in fuel cell technology. J. Optoelectron. Adv. Mater. 2006;8:670–674.
- Telke AA, Joshi SM, Jadhav SU, et al. Decolorization and detoxification of Congo red and textile industry effluent by an isolated bacterium Pseudomonas sp. SU-EBT. Biodegradation. 2010;21:283–296.10.1007/s10532-009-9300-0
- Lorenc-Grabowska E, Gryglewicz G. Adsorption characteristics of Congo red on coal-based mesoporous activated carbon. Dyes Pigm. 2007;74:34–40.10.1016/j.dyepig.2006.01.027
- Tanaka S, Kida K, Nagaoka T, et al. Mechanochemical dry conversion of zinc oxide to zeolitic imidazolate framework. Chem. Commun. 2013;49:7884–7886.10.1039/c3cc43028f