Abstract
In the present study the characterization and properties of silver nanoparticles from Prosopis glandulosa leaf extract (AgNPs) were investigated using UV–Vis spectroscopic techniques, energy dispersive X-ray spectrometers (EDS), zeta potential and dynamic light scattering. The UV–Vis spectroscopic analysis showed the absorbance peaked at 487 nm, which indicated the synthesis of silver nanoparticles. The experimental results showed silver nanoparticles had Z-average diameter of 421 nm with higher stability (−200 mV). The EDS analysis also exhibited presentation of silver element. Additionally, the different concentrations of AgNPs (25, 50, 75 and 100 mg/mL) showed antibacterial activity against Acinetobacter calcoaceticus and Bacillus cereus. Finally, AgNPs from leaf extracts of P. glandulosa may be used as an agent of biocontrol of microorganism of importance medical. However, further studies will be needed to fully understand the antimicrobial activity of silver nanoparticles obtain from P. glandulosa.
1. Introduction
Nanoparticles (NPs) are a group of materials with distinctive features and extensive applications in different fields of science and medicine.[Citation1] Metal nanoparticles have been extensively studied and different approaches have been engaged for the preparation of these compounds. Among these metal nanoparticles, silver nanomaterials exhibit broad spectrum biocidal activity toward bacteria, fungi, and viruses.[Citation2] This has motivated its use in a large number of biomedical applications.[Citation3] In this sense, the nanoparticles may penetrate inside the cell causing damage by interacting with phosphorus and sulfur containing compounds such as DNA and protein. Another possible contribution to the bactericidal properties of silver nanoparticles is the release of silver ions from the particles.[Citation4] At the present, various chemical and physical methods are known for preparation of silver and other metal nanoparticles. However, these methods are very costly and toxic to the environment.[Citation5] On the other hand, the biological method provides a feasible alternative for the synthesis of nanoparticles. In this sense, the use of plants as the production assembly of silver nanoparticles has drawn attention, because of its rapid, eco-friendly, economical protocol and providing a single step technique for the biosynthetic processes.[Citation6] Several plant extracts have been used to produce nanoparticles, such as Cissus quadrangularis or Ficus benghalensis.[Citation7,8] In recent years, plant-mediated biological syntheses of nanoparticles have been gaining importance due to its simplicity and great potential with natural reductants.[Citation9] The genus Prosopis L., is characteristic of arid and semiarid zones, and its widespread distribution includes ecosystems in Asia, Africa, and the Americas.[Citation10] In Mexico, the majority of the populations of the genus Prosopis are found in the north and center of the country, where they have formed forest extensions that are adapted to desert climate.
Species such as Prosopis glandulosa Torr., dominate the vegetation mosaic in Baja California Mexico, primarily in an ecosystem known as mezquitales, which is characterized by a hyperarid climate with almost no rainfall (0.6 mm year−1).[Citation11] These populations of the genus Prosopis have been the focus of scientific interest mainly because of their physiological and ecological adaptations to their hyperarid environment. However, studies about the use of P. glandulosa for the biosynthesis of AgNPs as a green chemistry method are scarce. In the present study, we report the easy synthesis of silver nanoparticles by an environmental friendly procedure involving the in situ reduction of Ag by P. glandulosa extracts and the evaluation of their antimicrobial activity against Acinetobacter calcoaceticus and Bacillus cereus.
2. Material and methods
2.1. Biosynthesis of silver nanoparticles (AgNP) from P. glandulosa
To prepare the AgNP from P. glandulosa, healthy leaves of this plant were collected in Baja California, Mexico. The collected leaves were surface sterilized with 0.5% NaOCl (Clorox) for 1 min each, followed by an extensive rinse with deionized sterile water. For sample preparation: P. glandulosa fresh leaves were cut into small pieces and dried using oven an electric oven for 12 h at 50 °C. Then 30 g of dried leaf of P. glandulosa was mixed with 300 mL of deionized water and heated at 70 °C for 30 min. About 50 mL of aqueous P. glandulosa leaf extract was then filtered thrice through Whatman No. 1 filter paper and centrifuged at 4000 rpm for 10 min to remove particulate matter and to get clear solutions which were then refrigerated (4 °C) for further use. For AgNPs synthesis 0.4 mL of aqueous P. glandulosa leaf extract were added into 0.16 mL of aqueous solution of 10 mM silver nitrate and heated to 60 °C for 30 min.
The color change was observed which stands as a preliminary identification of the formation of AgNPs.[Citation12] Additionally, the AgNPs were purified by centrifugation at 10,000 rpm for 15 min to remove excess silver ions. The centrifugation process was repeated three times to remove all silver colloids with deionized water. After the sediment (AgNPs) was transferred to freeze dryer and the powder was used in antibacterial Assays.
2.2. Characterization of AgNPs from P. glandulosa
To determine the time point of maximum production of silver nanoparticles, the absorption spectra of the samples previously prepared with P. glandulosa leaf extract and silver nitrate were scanned in UV–Vis (vis) spectra, between wavelengths of 400–500 nm in a spectrophotometer (Thermo Scientific BioMate 3 Spectrophotometer, USA), having a resolution of 1 nm and using water as the blank.
2.3. Scanning electron microscopy and energy dispersive X-ray
A scanning electron microscope (SEM) (JEOL 6010; JEOL, Tokio, Japan) was employed to characterize the size and morphology of the AgNPs at an accelerating voltage of 10 kV according to the following procedure: a drop of AgNPs sample was transferred on to carbon coated copper grids. Then the film on the SEM grid was allowed to dry by putting it under a mercury lamp for 3 min and analyzed for size determination.[Citation13] For EDS analysis, the AgNPs were dried and drop coated on to carbon film. EDS analysis was then performed using the oxford instrument Thermo EDS.
2.4. Zeta potential and dynamic light scattering
In order to find out the stability and size distribution of AgNPs obtained from P. glandulosa. Zeta potential was measured by using a Nanotrac Wave (Microtrac) according to.[Citation14] Two thousand micro liter of sample was transferred in the clear disposable zeta cell for the measurement of zeta potential. Measurements were made by means of Dynamic Light Scattering (DLS) in the range of 0.1–1000 μm at 25 °C, using laser wavelength of 780 nm and a scattering angle of 90°. The DLS data were analyzed by the Microtrac FLEX operating software.[Citation15]
2.5. Antibacterial assays
The antibacterial activity of the synthesized AgNPs was determined using the disk diffusion method. Two types of bacteria, including Gram-negative bacteria (Acinetobacter calcoaceticus) and Gram-positive bacteria (Bacillus cereus) were tested. Nutrient agar medium plates were prepared, sterilized and solidified. After solidification bacterial cultures were swabbed on these plates (each strain was swabbed uniformly onto individual agar plates). Sterile paper disks were placed on the agar plates. The plates were divided into three groups and each group included two types of bacteria (A. calcoaceticus and B. cereus); in the first group, 20 μL of sterile distilled water were applied to the disks as control. In the second group, 20 μL of different concentration of AgNPs, previously diluted with deionized water (25, 50, 75 and 100 mg/ mL) was added to each disk. In the third group, 20 μL of leaf extract of P. glandulosa were applied to the disks (control). All the plates were incubated at 32 °C for 24 h. After incubation was completed, the zone of inhibition, which appeared as a clear area around the disks, was measured.
3. Results
3.1. Biosynthesis of AgNPs-P. glandulosa
The green synthesis of AgNPs through P. glandulosa extracts were carried out and confirmed by visual observation. After the addition of the plant extract to the aqueous AgNO3 solution, the color of the solution reaction changed from yellowish to a reddish brown color within 30 min of incubation at 60 °C (Figure ), indicating the formation of Ag-NPs. On the other hand, the UV–Vis spectroscopy is an important preliminary technique to ascertain the formation and stability of metal nanoparticles in aqueous suspension. Thus formation of AgNPs by reduction of aqueous Ag+ during exposure to the aqueous extract of P. glandulosa were followed and characterized by UV–Vis spectroscopy. As shown in Figure , the surface plasmon resonance of the AgNPs was centered at approximately 487 nm.
3.2. SEM and EDS analysis
SEM has been employed to characterize the size, shape and morphology of synthesized silver nanoparticles. SEM provided further insight into the morphology and size details of the silver nanoparticles. SEM image (Figure ) recorded at high magnifications of the Ag nanoparticles synthesized by treating AgNO3 solution with P. glandulosa leaf extract. In general, the SEM image shows that the majority of nanoparticles were in spherical shape with varying size as visually seen from the adjacent photographs. It can be seen that some of the particles are well dispersed and many of them have formed aggregates. On the other hand, the EDS analysis gives qualitative as well as quantitative status of elements that may be involved in formation of nanoparticles. The elemental profile of synthesized nanoparticles using extract of P. glandulosa shows higher counts at 3 keV due to silver, confirms the formation of silver nanoparticles (Figure ).
3.3. Zeta potential and DLS
Particle size, size distribution and zeta potential were important characterizations of the silver nanoparticles because they govern the other characterizations, such as saturation solubility and dissolution velocity, physical stability, or even biological performances.[Citation16] As shown in Table , zeta potential was found to be −200.0 mV for synthesized AgNPs. On the other hand, the size distribution of the synthesized AgNPs is depicted in Figure . It is observed that the particles obtained are polydisperse mixtures in the range of 32–600 nm with the average mean size (diameter) of 421 nm.
Table 1. Zeta-potential analysis of silver nanoparticles synthesized from P. glandulosa.
3.4. Antimicrobial activity of synthesized silver nanoparticles
In the present study, the antibacterial activity of the synthesized AgNPs from P. glandulosa against A. calcoaceticus and B. cereus was investigated. Our results showed that all concentrations of AgNPs from P. glandulosa present a clear zone of inhibition against tested bacteria with respect to leaf extract of this plant used as control and the zone of inhibition increased with respect to the concentration of biosynthesized AgNPs used in the medium culture (Figure and Table ). In contrast, the leaf extract of P. glandulosa did not exhibit antimicrobial effect against tested bacteria (Figure ).
Figure 6. Antibacterial activity of P. glandulosa extract and AgNPs from P.glandulosa (a) Bacillus cereus and (b) Acinetobacter calcoaceticus.
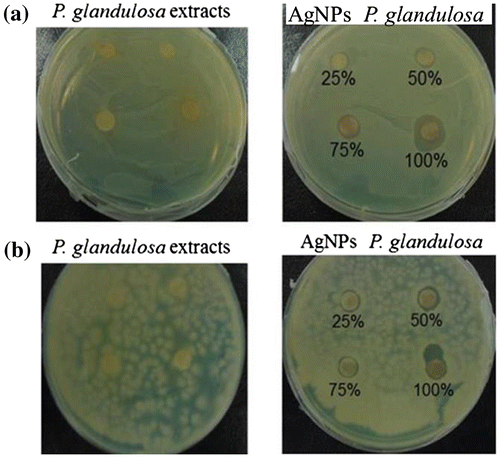
Table 2. Inhibition of microorganisms by AgNPs from P. glandulosa.
4. Discussion
In the present study, the silver nanoparticles exhibit reddish brown color in aqueous solution due to excitation of surface plasmon vibrations in silver nanoparticles.[Citation17] Initially the reduction of Ag+ ions leads to the formation of silver atoms (Ag) which may follow by agglomeration into oligomer cluster. These clusters eventually lead to the formation of colloidal Ag particles.[Citation18] Similar findings were reported by [Citation19] who used aqueous extract of fresh leaves of P. juliflora with 0.01 M AgNO3 aqueous solution for the synthesis of AgNPs and they revealed that metabolites such as terpenoids or flavonoids could be responsible for the reduction and capping of AgNPs.
The elemental analysis of the silver nanoparticles revealed highest proportion of silver followed by C, Cl and O. This result is consistent with the results reported by [Citation18] who found that higher counts of AgNPs synthesized using banana peel extract at 3 keV. On the other hand, our results showed that zeta potential was found to be −200.0 mV for synthesized AgNPs from P. glandulosa. Similar result were observed by [Citation20,21] that mentioned that a zeta potential higher than 30 mV or lesser than −30 mV is indicative of a stable system. In this context, colloidal suspension of Ag nanoparticles synthesized using Ceriops tagal leaves extract and Malus domestica fruit extract were highly stable with a zeta potential of −34.18 mV.[Citation22] The values of zeta potential for the AgNPs obtained in the present study indicate a long term stability of the colloids, which could be attributed to the presence of bioactive components present in the aqueous extract that cover nanoparticles stabilizing them. On the other hand, in the present study the average size of the particles obtains from P. glandulosa was of 421 nm. These findings are in agreement with previous studies realized by Palanisamy et al. [Citation23], who reported that the particle size of the synthesized silver nanoparticles using Emblica officinalis leaf extract was in the range 139–595 nm with average size 367 nm. The mechanism behind the activity of AgNPs from P. glandulosa against A. calcoaceticus and B. cereus is not yet fully explored and there are some common mechanisms behind up to date. However, there are various theories suggested about the action of AgNPs on microbes to cause the antimicrobial effect. One possibility of growth-restriction may be a chance of the generation of free radicals by AgNPs positioned at surface which may have been thrashed lipid membrane followed by destruction of microorganisms.[Citation24,25] Another fact is that the DNA has sulfur and phosphorus as its major components; the nanoparticles can act on these soft bases and destroy the DNA which would definitely lead to cell death.[Citation25]
However, further studies are needed to confirm their potential of AgNPs from P. glandulosa in the biocontrol of the microorganisms evaluated.
5. Conclusions
The biosynthesis of silver nanoparticles using Prosopis glandulosa is a simple, environmentally friendly, low-cost and non-toxic approach. The fabricated nanoparticles showed antimicrobial activity against B. cereus and A. calcoaceticus at different concentration. Our findings indicate that AgNPs from P. glandulosa may have potential benefits as biocontrol agents for human pathogens. However, further studies are needed to confirm their potential, determine their effect on human pathogens and identify the bioactive compounds in P. glandulosa.
Disclosure statement
No potential conflict of interest was reported by the authors.
Funding
This work was supported by CONACYT CIENCIA BASICA 2013 (proyecto 21975) and the Universidad Autonoma de Baja California (18a Convocatoria interna de Proyectos).
Acknowledgements
The authors are particularly gratefully to Conacyt Ciencia Basica 2013 (219750) and Universidad Autonoma de Baja California 18a Convocatoria Interna de Proyectos.
References
- Reddy NJ, Nagoor V, Rani D., et al. Evaluation of antioxidant, antibacterial and cytotoxic effects of green synthesized silver nanoparticals by piper longum fruit. Mater Sci Eng C Mater Biol Appl. 2014; 34:115–12210.1016/j.msec.2013.08.039
- Alt V, Bechert T, Streinrucke P, et al. An in vitro assessment of the antibacterial properties and cytotoxicity of nanoparticulate silver bone cement. Biomaterials. 2004;25:4383–4391.10.1016/j.biomaterials.2003.10.078
- Sanpui P, Chattopadhyay A, Ghosh S. Induction of apoptosis in cancer cells at low silver nanoparticle concentrations using chitosan nanocarrier. ACS Appl Mater Interfaces. 2011;3:218–228.10.1021/am100840c
- Sadhasivam S, Shanmugam P, Yun K. Biosynthesis of silver nano-particles by Streptomyces hygroscopicus and antimicrobial activity against medically important pathogenic microorganisms. Colloids Surf B. 2010;81:358–362.10.1016/j.colsurfb.2010.07.036
- Nanda A, Saravanan M. Biosynthesis of silver nanoparticles from Staphylococcus aureus and its antimicrobial activity against MRSA and MRSE. Nanomedicine. 2009;5:452–456.
- Jeong SH, Yeo SY, Yi SC, et al. The effect of filler particle size on the antibacterial properties of compounded polymer/ silver fibers. J Mater Sci. 2005;40:5407–5411.10.1007/s10853-005-4339-8
- Valli JS, Vaseeharan B. Biosynthesis of silver nanoparticles by Cissus quadrangularis extracts. Mater Lett. 2012;82:171–173.10.1016/j.matlet.2012.05.040
- Saxena A, Tripathi RM, Zafar F, et al. Green synthesis of silver nanoparticles using aqueous solution of Ficus benghalensis leaf extract and characterization of their antibacterial activity. Mater Lett. 2012;6:791–794.
- Kumar V, Yadav SK. Plant-mediated synthesis of silver and gold nanoparticles and their applications. J Chem Technol Biotechnol. 2009;84:151–157.10.1002/jctb.v84:2
- Pérez-García EA, Meave JA, Cevallos-Ferriz SRS. Flora and vegetation of the seasonally dry tropics in Mexico: origin and biogeographical implications. Acta Bot Mex. 2012;100:149–193.
- Carevic FS. The role of ecophysiological studies in the genus Prosopis: implications for the conservation of drought-prone species. Idesia. 2014;32:77–81.10.4067/S0718-34292014000400010
- Ahmed S, Saifullah M, Babu LS, et al. Green synthesis of silver nanoparticles using Azadirachta indica aqueous leaf extract. J Radiat Res Appl Sci. 2016;9:1–7.10.1016/j.jrras.2015.06.006
- Ramalingam V, Rajaram R, Premkumar C, et al. Biosynthesis of silver nanoparticles from deep sea bacterium Pseudomonas aeruginosa JQ989348 for antimicrobial, antibioflim and cytotoxic activity. J Basic Microbiol. 2013;53:1–9.
- Singh A, Jain D, Upadhyay MK, et al. Green synthesis of silver nanoparticles using Argemone Mexicana leaf esctract and evaluation of their antimicrobial activities. Dig J Nanomater Biostruct. 2010;5:483–489.
- Zhou C, Qi W, Lewis EN, et al. Concomitant Raman spectroscopy and dynamic light scattering for characterization of therapeutic proteins at high concentrations. Anal Biochem. 2015;472:7–20.10.1016/j.ab.2014.11.016
- Visahl RP, Agrawal YK. Nanosuspesion: an approach to enhance solubility of drugs. J Adv Pharm Technol Res. 2011;2:81–87.
- Shankar SS, Rai A, Ahmad A, et al. Rapid synthesis of Au, Ag, and bimetallic Au core-Ag shell nanoparticles using Neem (Azadirachta indica) leaf broth. J. Colloid Interface Sci. 2004;275:496–502.10.1016/j.jcis.2004.03.003
- Ibrahim HMM. Green synthesis and characterization of silver nanoparticles using banana peel extract and their antimicrobial activity against representative microorganisms. J Radiat Res Appl Sci. 2015;8:265–275.10.1016/j.jrras.2015.01.007
- Raja K, Saravanakumar A, Vijayakumar R. Efficient synthesis of silver nanoparticles from Prosopis juliflora leaf extract and its antimicrobial activity using sewage. Spectrochim Acta, Part A. 2012;97:490–494.10.1016/j.saa.2012.06.038
- Rai A, Singh A, Ahmad A, et al. Role of halide ions and temperature on the morphology of biologically synthesized gold nanotriangles. Langmuir. 2006;22:736–741.10.1021/la052055q
- Tripathy A, Raichur AM, Chandrasekaran N, et al. Process variables in biomimetic synthesis of silver nanoparticles by aqueous extract of Azadirachta indica (Neem) leaves. J Nanopart Res. 2010;12:237–246.10.1007/s11051-009-9602-5
- Dhas SP, Mukerjhee A, Chandrasekaran N. Phytosynthesis of silver nanoparticles using Ceriops tagal and its antimicrobial potential against human pathogens. Int J Pharm Pharm Sci. 2013;5:349–352.
- Palanisamy K, Kalaiselvi PA, Gabriel M, et al. Emblica officinalis leaf extract mediated green synthesis of antibacterial silver nanoparticles against human pathogens. World J Pharm Sci. 2014;3:2019–2032.
- Sharma G, Jasuja ND, Rajgovind P. et al. Synthesis, characterization and antimicrobial activity of Abelia grandiflora assisted AgNPs. J Microb Biochem Technol. 2014; 6: 274–278
- Morones JR, Elechiguerra JL, Camacho A, et al. The bactericidal effect of silver nanoparticles. Nanotechnology. 2005;16:2346–2353.10.1088/0957-4484/16/10/059