Abstract
Adsorption of Hg2+ and methylmercury (MeHg) to multi-walled carbon nanotubes (MWCNTs) modified, respectively, with hydroxyl, amine and carboxyl groups was studied. The effect of various factors like the initial pH, natural organic matter (NOM), Cl- and adsorbent dose on the sorption efficiency were evaluated. It was found that amine-modified MWCNTs showed a strong adsorption capacity to Hg2+ and MeHg, and the removal efficiency could reach up to 92% (0.5 g/L MWCNTs, and 100 μg/L Hg2+ and MeHg) which is independent of pH. NOM had complex effects on the adsorption of Hg2+ and MeHg to MWCNTs. Cl- inhibited the adsorption of Hg2+ and MeHg to MWCNTs. The adsorption of Hg2+ and MeHg was found to be inhomogeneous and homogeneous chemisorption, respectively. Our results suggested that MWCNTs modified with different functional groups can efficiently adsorb both Hg2+ and MeHg in aqueous environment.
1. Introduction
Because of its long distance migration and bioaccumulation, mercury as a global pollutant has been attracting widespread attention [Citation1Citation2]. Mercury in environment and biological body mainly exists in three forms including Hg0, Hg2+ and methylmercury (MeHg). Hg0 and Hg2+ can be converted into MeHg by microorganisms [Citation3Citation4Citation5Citation6]. MeHg is the most toxic organic mercury and is ubiquitous in the aquatic environment [Citation7]. Mercury pollution comes mainly from industrial production, mercury mine and non-ferrous metals processing factory [Citation8]. Various kinds of methods have been used to remove heavy metals from aqueous solutions including ion exchange, chemical precipitation, biological treatment, membrane filtration and adsorption [Citation9]. Recently carbon nanotubes (CNTs), as adsorbents in the field of environment, have attracted more and more attention, because of their unique structure and chemical properties [Citation10Citation11Citation12].
Multi-walled carbon nanotubes (MWCNTs) as one type of CNTs have been used widely to adsorb heavy metals such as Pb2+, Cd2+, Zn2+, Cu2+, Ni2+, Hg2+ from aqueous solutions [Citation9Citation13Citation14Citation15Citation16Citation17]. The MWCNTs have been experimentally proven to possess cylindrical hollow micro-crystals of graphite which have strong adsorption ability, exceptional mechanical properties, unique electrical property, high chemical and thermal stability, a large specific surface area, and high adsorption capacity [Citation13Citation14Citation15]. Researches have shown that the surface functional groups such as amino [Citation18Citation19], carboxyl [Citation20Citation21], hydroxy [Citation20Citation21] and thiol [Citation19Citation22Citation23Citation24] on carbon nanotubes can greatly improve their adsorption capacity for metal ions. Moreover, various aqueous environment relevant factors including pH, ionic strength and natural organic matter (NOM) can affect the adsorption of metal ions onto MWCNTs [Citation12Citation13Citation20Citation25Citation26Citation27]. To assess the efficiency of MWCNTs as absorbents to removal of Hg2+ and MeHg in waters, it is of great importance to understand the effects of NOM and other environmental factors on the sorption of Hg2+ and MeHg to functionalized MWCNTs. Although the adsorption of Hg2+ by MWCNTs has been extensively studied [Citation17Citation28Citation29Citation30], the adsorption of MeHg to functionalized MWCNTs especially in the presence of Hg2+, has not been reported.
In this study, we studied the removal efficiency of Hg2+ and MeHg from aqueous solution by pristine MWCNTs and MWCNTs modified with carboxylic functional group (MWCNTs-COOH), hydroxyl functional group (MWCNTs-OH), and amino functional group (MWCNTs-NH2), respectively. The effects of environmentally relevant factors like pH, NOM, and Cl− on the sorption efficiency were evaluated. In addition, the adsorption isotherms and kinetics were studied by fitting to various models to understand the sorption mechanisms.
2. Materials and methods
2.1. Materials
Four kinds of multi-walled carbon nanotubes (MWCNTs > 95% in purity and special surface area, SSA > 140 m2/g), including unmodified MWCNTs, and MWCNTs-COOH (–COOH group content 2.56%, m/m and SSA > 130 m2/g), MWCNTs-OH (–OH group content 5.58%, m/m and SSA > 233 m2/g), and MWCNTs-NH2 (–NH2 group content 0.45%, m/m and SSA > 233 m2/g) were purchased from Chengdu Organic Chemicals Co. Ltd. (Chengdu, China). They have the same outer diameter (8–15 nm), length (~50 μm) and numbers of walls (6–12). Stock solutions of 1000 mg/L Hg2+ (as Hg) in 5% HNO3 and 60 mg/L MeHg (as Hg) in methanol were purchased from National Institute of Metrology (Beijing, China). All stock solutions were stored in darkness at 4 °C. Mili-Q deionized water was used for all experiments. Serial dilutions of the stock solutions were obtained with deionized water. The working standard solutions were prepared daily. L-cysteine was obtained from Sigma Aldrich Co. (St. Louis, MO, USA). Suwannee River natural organic matter (SRNOM) (Lot No. 2R101N) was obtained from the International Humic Substance Society (St. Paul, MN, USA). One g/L stock solutions of SRNOM were prepared in deionized water and shaken for 12 h at room temperature under the darkness. Then the solution was filtered through 0.22 μm cellulose nitrate membrane. A HPLC system (Agilent 1200 series, Agilent Technologies, Palo Alto, CA) was coupled to the ICP-MS instrument (Agilent 7700cs) by directly connecting the column outlet to the cross-flow nebulizer of ICP-MS through a commercial polytetrafluoroethylene (PTFE) connector. The mobile phase for the HPLC-ICP-MS system consisting of 1 g/L L-cysteine and 0.06 mol/L ammonium acetate was prepared daily.
2.2. Characterization of MWCNTs
The morphology and size of MWCNTs were characterized by transmission electron microscope (TEM, Hitachi, Japan). Ten mg pristine and functionalized MWCNTs were added into 20 mL deionized water to form aqueous solution respectively. Then 20 μL sample of pristine and functionalized MWCNTs were droped on carbon membrane copper net, drying in vacuum oven at room temperature for 12 h.
The surface functional groups of pristine and functionalized MWCNTs were detected by Fourier transform infrared spectroscopy (FT/IR-6100, JASCO, Japan). A transparent thin sheet was pressed and scanned from 400 to 4000 cm−1, which the MWCNTs to KBr quality ratio was 1–100.
2.3. Adsorption experiments
The experiments were performed in 40 mL glass bottles with caps. The total volume of the solution was 20 mL, and Hg2+ and MeHg working standard solutions were spiked into glass bottles to reach a final concentration of 100.0 μg/L Hg, respectively. After adding 10 mg MWCNTs, the solutions were placed on the oscillator to shake at 245 rmp and 20 °C for 90 min to reach adsorption equilibrium.
After achieving the equilibrium, 2 mL solution was taken out from each bottle and filtered through 0.22 μm PTFE membrane. The HPLC-ICP-MS hyphenated system was used to determine Hg2+ and MeHg in the filtered solutions, and the detailed instrumentation and procedure could refer to our previous study [Citation31].
To evaluate the influence of initial pH on adsorption, the pH of the solution was adjusted to 4.0, 5.0, 6.0, 7.0, 8.0, and 9.0, respectively, by using 10 mmol/L phosphate buffer. The effects of other factors, including Cl− (0–800 mmol/L), NOM (0–20 mg/L, DOC) and adsorbent dose (0–3 g/L), on the adsorption were tested at pH 7.0. For each experiment, three parallel samples were prepared.
The removal efficiency of Hg2+ or MeHg was calculated according to the equation [Citation32]: (1)
where R is the removal efficiency of Hg2+ or MeHg, C0 is the initial concentration of Hg2+ or MeHg (mg/L), and Ce is the equilibrium concentration of Hg2+ or MeHg (mg/L).
The adsorption capacity of adsorbent at equilibrium was calculated by the following equation [Citation21]:
(2)
where q is the adsorption capacity of adsorbent (mg/g), C0 is the initial concentration of Hg2+ or MeHg (mg/L), and Ce is the equilibrium concentration of Hg2+ or MeHg (mg/L). m is the adsorbent weight (g), V is the volume of solution (L).
2.4. Adsorption isotherm models
To reveal the adsorption process and evaluate adsorption capacity, adsorption isotherms were studied. The Hg2+ and MeHg adsorption isotherms for MWCNTs, MWCNTs-OH, MWCNTs-NH2, MWCNTs-COOH were modeled by various isotherms.
The Langmuir model is used to describe homogeneous monolayer adsorption on the surface generally. The linear form of the model is given as [Citation33]:
(3)
where qe is the equilibrium adsorption capacity of adsorbent (mg/g), Ce is the equilibrium concentration of Hg2+ or MeHg (mg/L). Qm and b are Langmuir constants indicating the capacity and energy of adsorption, respectively, and can be calculated from the intercept and slope value of the linear plot, 1/qe vs. 1/Ce.
The character of the Langmuir isotherm can also be expressed by adopting a dimensionless equilibrium parameter, RL, which is defined as [Citation33]:
(4)
where b is the Langmuir constant (L/mg) and C0 is the initial concentration of Hg2+ or MeHg (mg/L). The RL value indicates the shape of isotherm. RL values between 0 and 1 indicate favorable adsorption, while RL > 1, RL = 1, and RL = 0 indicate unfavorable, linear, and irreversible adsorption isotherms, respectively.
The Freundlich model describes the heterogeneity adsorption system and can be expressed as the following equation [Citation28Citation33Citation34]:
(5)
where qe is the equilibrium adsorption capacity of adsorbent (mg/g), Ce is the equilibrium concentration of Hg2+ or MeHg (mg/L). KF (mg1 − n Ln/g) and n are Freundlich constants indicating the relative adsorption capacity and adsorption intensity. 1/n and lnKF are the slope and intercept value of the linear Freundlich equation, respectively.
The Dubinin-Redushkevich (D-R) model is related to adsorption energy and can be expressed as the following equation [Citation33Citation35]:
(6)
(7)
where qe is the equilibrium adsorption capacity of adsorbent (mg/g), qm is the maximum adsorption capacity, Ce is the equilibrium concentration of Hg2+ or MeHg (mol/L), β is related to the mean adsorption energy, ε is the Polanyi potential, and R (J/mol/K) and T (K) are gas constant and the temperature, respectively. E (kJ/mol) is the mean adsorption energy of adsorption per molecule of adsorbent, when it is transferred from infinity in the solution to the solid surface [Citation33]:
(8)
The Temkin isotherm model is based on a hypothesis that the adsorption energy decreases linearly with the surface coverage. The Temkin isotherm can be expressed as the following equation [Citation33]:
(9)
where RT/bT = BT, qe is the equilibrium adsorption capacity of adsorbent (mg/g), qm is the maximum adsorption capacity, Ce is the equilibrium concentration of Hg2+ or MeHg (mg/L), AT and BT are the constants of the linear plot qe vs. lnCe.
2.5. Kinetic models
The adsorption kinetic of Hg2+ and MeHg was studied according to pseudo-first-order and pseudo-second-order adsorption equations [Citation35Citation36Citation37]. The pseudo-first-order equation is given as:
(10)
where qt is the amount of adsorption at time t (mg/g), qe is the equilibrium adsorption capacity (mg/g), and k1 (min−1) is the pseudo-first-order rate constant.
The pseudo-second-order equation is shown as:
(11)
where k2 (g mg−1 min−1) is the pseudo-second-order rate constant.
3. Results and discussion
3.1. Physical characterization
3.1.1. Characterization of MWCNTs with TEM
Figure shows the TEM images of four kinds of MWCNTs, in which no distinct changes in surface morphology was observed for the functionalized MWCNTs in comparison to the pristine MWCNTs.
3.1.2. FTIR spectroscopic characterization of MWCNTs
The FTIR spectrum were used to characterize the surface functional groups of MWCNTs, and the results were shown in Figure . While no major functional group was identified for the pristine MWCNTs, symmetric and asymmetric methylene stretching bands appeared at ~2962 and ~2884 cm−1 were detected in functionalized MWCNTs. Generally these functional groups located on the surface defect of the carbon nanotubes [Citation38]. In the MWCNTs-COOH spectrum the characteristic peaks appeared at the ~3451 and ~1617 cm−1, which are assigned to stretching vibrations of v(–OH) and v(C=O) of –COOH [Citation34Citation39]. The peaks at ~3450 cm−1 correponded to -NH2 stretching vibration in the MWCNTs-NH2 spectrum. In addition, the presence of peaks at ~1415 and ~1262 cm−1, origined from N-H in-plane and C-N bond stretching, respectively [Citation39]. The peaks at ~3439 and ~1265 cm−1 were attributed to -OH stretching vibration in the MWCNTs-OH spectrum [Citation20]. Functional groups on the surface of the carbon nanotubes offered a lot of adsorption sites, which may be useful to increase the adsorption ability of carbon nanotubes.
3.2. Influence of initial pH on adsorption
The pH of solution had a great effect on the adsorption of metal ions [Citation9Citation10Citation40]. To evaluate the effect of initial pH on the Hg2+ and MeHg adsorption, a series of sample solutions with pHs ranging from 4.0 to 9.0 by phosphate buffers were adopted. The results shown in Figure indicate that the pH had no significant effect on the removal of Hg2+ and MeHg on MWCNTs-NH2. This is because the adsorption of Hg2+ and MeHg onto MWCNTs-NH2 is very strong that is independent of the initial pH. It is very important that the stability constant of the compounds of amino group and mercury can reach 1018 [Citation41]. It was found that the maximum removal efficiency is up to more than 92%, which is consist with the results in Ref. [Citation41].
Figure 3. Effect of pH on removal of Hg2+ (a) and MeHg (b) by MWCNTs.
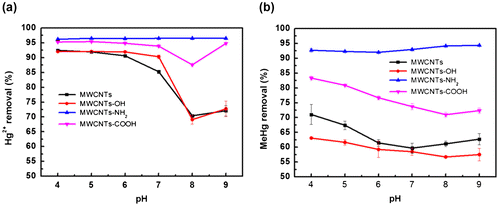
Figure also shows that for pristine MWCNTs, MWCNTs-OH and MWCNTs-COOH, the removal efficiency of Hg2+ and MeHg decreased gradually with the increase of pH. This is because with the increase of solution pH, the Hg(OH)+ or MeHgOH compounds could be generated, which is stable in the water and reduced the Hg2+ and MeHg adsorption [Citation17Citation20]. It should be noted that a slight increase of removal efficiency of Hg2+ and MeHg were observed for pH > 8.0. This might be attributed to the fact that when the solution pH is larger than the critial value pHpzc, mostly in the range of 4–6 [Citation9Citation42], the negative charge surface, which can provide electrostatic interactions, enhances the absorption of Hg2+ and MeHg [Citation40].
3.3. Effect of NOM on adsorption
The impact of NOM on the adsorption of Hg2+ and MeHg to MWCNTs seemed very complicated. There are at least four adsorbing processes in the solution: (i) NOM could combine with Hg2+ and MeHg [Citation25Citation43]; (ii) MWCNTs could adsorb Hg2+ and MeHg [Citation17Citation21Citation29Citation37Citation44Citation45Citation46]; (iii) MWCNTs could adsorb NOM and form MWCNTs-NOM complexes [Citation47]; and (iv) NOM could stabilize the dispersion of MWCNTs [Citation26Citation27]. Depending on the characteristics of MWCNTs and NOM, as well as the aqueous chemistry parameters, these four processes showed different strength and therefore resulted in varied removal efficiency of Hg2+ and MeHg.
The effect of NOM concentration on the adsorption of Hg2+ and MeHg was studied at pH 7.0. As shown in Figure (a), the removal efficiency of Hg2+ decreased gradually when the NOM concentration increased from 0 to 20 mg/L DOC. The influence of NOM on the adsorption of Hg2+ can be understood from the following three aspects. Firstly, MWCNTs could adsorb NOM and Hg2+ at the same time, which generated the competitive adsorption. Secondly, NOM occupied the binding sites on the MWCNTs, reducing the adsorption of Hg2+. Lastly, free NOM in the solution could increase the distribution of Hg2+ in water, which could lead to reduced adsorption of Hg2+.
Figure 4. Effect of NOM on the removal of Hg2+ (a) and MeHg (b) by MWCNTs.
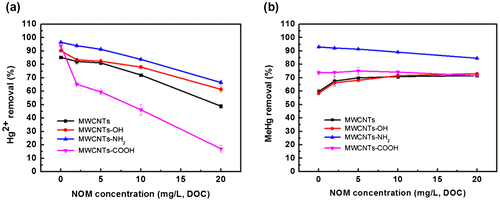
Figure (b) shows that when the NOM concentration increased from 0 to 20 mg/L DOC, the removal efficiency of MeHg varied depending on the functional groups modified on MWCNTs. For MWCNTs and MWCNTs-OH, due to their relatively weak binding to MeHg, when low concentration of NOM was present, the NOM adsorbed on MWCNTs enhanced the adsorption and therefore removal of MeHg. For MWCNTs-NH2 and MWCNTs-COOH that showed relatively strong combination with MeHg, when NOM was present, the NOM adsorbed on MWCNTs could not increase the adsorption of MeHg on MWCNTs; in contrast, the competitive binding of NOM to MeHg in the solution slightly reduced the removal efficiency of MeHg by MWCNTs.
3.4. Effect of Cl− on adsorption
The effect of Cl− on adsorption of Hg2+ and MeHg at pH 7.0 was shown in Figure , in which Cl− exhibited a strong inhibitory effect on the adsorption of Hg2+ and MeHg. With the increase of Cl− concentration from 0 to 800 mmol/L, the removal efficiency of Hg2+ and MeHg decreased sharply except MWCNTs-NH2, in which case the removal efficiency decreased gradually due to the strong adsorption of Hg2+ and MeHg on MWCNTs-NH2.
Figure 5. Effect of Cl− on the removal of Hg2+ (a) and MeHg (b) by MWCNTs.
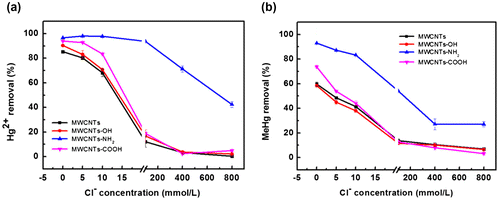
It is well known that Hg2+ and MeHg inclined to bind to Cl− to form complexes, which could reduce their sorption to MWCNTs. While HgCl2 was the main existing form for Cl− concentration in the range of 5−115 mmol/L, HgCl42− dominated when the concentration increased from 115 to 800 mmol/L (Figure S1). High concentration of Cl− in solution could also promote the formation of very stable MeHgCl complex which showed lower sorption than MeHg to MWCNTs. These results agreed with those of previous studies [Citation20Citation48]. de Diego et al. [Citation48] found that a high concentration NaCl could led to declined adsorption rates of mercury species. Chen et al. [Citation20] revealed that the adsorption of Hg2+ dropped drastically from 94.3% to 1.5% as the increase of Cl− concentration from 0 to 1.0 mol/L and as the pH increasing from 4.3 to 10.5.
3.5. Effect of adsorbent dose
Figure shows the effect of adsorbent dose on the adsorption of Hg2+ and MeHg at pH 7.0. The strong sorption of Hg2+ to MWCNTs make it requires low dose of MWCNTs to remove Hg2+, and high (>90%) and almost equal removal efficiencies were obtained in the studied range of MWCNTs concentration (0.5−2.0 g/L) for all the four MWCNTs. Due to the relatively weak sorption of MeHg to MWCNTs, the removal efficiency of MeHg increased with the MWCNTs dose. The maximum value of removal efficiency was observed at 1.0 g/L MWCNTs-NH2 for the strong complex between the -NH2 and MeHg, and at about 2.0 g/L of the other three MWCNTs.
3.6. Adsorption isotherms
Adsorption isotherm was conducted by varying the initial concentration of Hg2+ and MeHg from 10–500 μg/L (Figures S2-S5), and obtained isotherm parameters were shown in Tables and . The R2 values in Tables and indicate that the Freundlich model and Dubinin-Radushkevich model can describe the Hg2+ adsorption very well, while the Langmuir and Dubinin-Radushkevich models showed the best fit to the MeHg adsorption.
Table 1. Parameters of isotherms for Hg2+ adsorption on MWCNTs (mean ± SD, n = 3).
Table 2. Parameters of isotherms for MeHg adsorption on MWCNTs (mean ± SD, n = 3).
From the Langmuir model, the maximum adsorption capacities Qm of Hg2+ and MeHg are in the order of MWCNTs-NH2 > MWCNTs-COOH > MWCNTs-OH > MWCNTs. For the initial concentration of 100 μg/L Hg2+ and MeHg, the values of RL were between 0 and 1, indicating the favorable adsorption onto the four kinds of MWCNTs. The R2 of MeHg was closer to one than that of Hg2+, which indicated that the adsorption of MeHg agreed more closely with Langmuir isotherms. The Qm of MWCNT-COOH for Hg2+ was 133.5 mg/g. The result was higher than that in the literature [Citation20].
The coefficient KF in the Freundlich model, representing the adsorption capacity, shows the same order as the Langmuir coefficient Qm. The n values ranged between 0 and 10 for all the absorbents, revealing the favorable adsorption process. The Freundlich isotherm also indicated that the adsorption presented in the surface of absorbents for Hg2+ was heterogeneity with the regression coefficient 0.862 to 0.960.
In the Dubinin-Radushkevich model, the mean free energy value EDR is used to identify adsorption mechanism. The EDR value is between 8 kJ/mol and 16 kJ/mol for the chemical adsorption process, and below 8 kJ/mol for physical adsorption [Citation37]. As the EDR values ranged from 8.15 to 12.37 kJ/mol, the adsorption of Hg2+ and MeHg to the four MWCNTs is the chemical adsorption. From the Temkin model, the adsorption energy BT shows the biggest value in the adsorption of Hg2+ and MeHg on MWCNTs-NH2, suggesting that Hg2+ and MeHg could adsorb to MWCNTs-NH2.
3.7. Adsorption kinetics
The pseudo-first-order and pseudo-second-order kinetics models were adopted, to describe the adsorption kinetics of Hg2+ and MeHg to the MWCNTs (Figures S6 and S7), and the regression coefficients and the parameters of the kinetic models are shown in Tables and . Since the R2 values of the pseudo-second-order kinetic model are closer to one than that of the pseudo-first-order kinetic model, the pseudo-second-order kinetic model is more suitable to describe the adsorption processes of the Hg2+ and MeHg.
Table 3. Kinetic parameters for adsorption of Hg2+ onto MWCNTs.
Table 4. Kinetic parameters for adsorption of MeHg onto MWCNTs.
4. Conclusions
This work demonstrated that MWCNTs-NH2, MWCNTs-COOH, MWCNTs-OH and MWCNTs are very efficient adsorbents for removal of Hg2+ and MeHg from aqueous solution, with the order of adsorption capacity as MWCNTs-NH2 > MWCNTs-COOH > MWCNTs-OH > MWCNTs. For all the four MWCNTs, the adsorption of Hg2+ and MeHg is pH dependent, and NOM and Cl− concentration also plays an important role. Increase of NOM concentration caused a significant reduction of the removal efficiency of Hg2+, while Cl− produced a strong inhibiting effect on the adsorption of Hg2+ and MeHg. The adsorption isotherm model of Hg2+ agreed with the Freundlich and Dubinin-Radushkevich isotherms very well, suggesting it is the heterogeneity and chemical sorption. The MeHg adsorption followed the Langmuir and Dubinin-Radushkevich isotherms, which belongs to the homogeneity and chemical adsorption.
Supplemental data
Supplemental data for this article can be accessed https://doi.org/10.1080/09542299.2017.1378596.
Disclosure statement
No potential conflict of interest was reported by the authors.
Funding
This work was supported by the National Key R&D Program of China [grant number 2016YFA0203102], Strategic Priority Research Program of the Chinese Academy of Sciences [grant number XDB14020101], and the National Natural Science Foundation of China [grant number 21621064].
TCSB_1378596_Supplementary_material.doc
Download MS Word (1 MB)References
- Tang W, Cheng J, Zhao W, et al. Mercury levels and estimated total daily intakes for children and adults from an electronic waste recycling area in Taizhou, China: key role of rice and fish consumption. J Environ Sci. 2015;34:107–115.10.1016/j.jes.2015.01.029
- Yan H, Rustadbakken A, Yao H, et al. Total mercury in wild fish in Guizhou reservoirs, China. J Environ Sci. 2010;22(8):1129–1136.10.1016/S1001-0742(09)60228-X
- Fleming EJ, Mack EE, Green PG, et al. Mercury methylation from unexpected sources: molybdate-inhibited freshwater sediments and an iron-reducing bacterium. Appl Environ Microbiol. 2006;72(1):457–464.10.1128/AEM.72.1.457-464.2006
- Gilmour CC, Henry EA, Mitchell R. Sulfate stimulation of mercury methylation in freshwater sediments. Environ Sci Technol. 1992;26(11):2281–2287.10.1021/es00035a029
- Wu H, Ding Z, Liu Y, et al. Methylmercury and sulfate-reducing bacteria in mangrove sediments from Jiulong River Estuary, China. J Environ Sci. 2011;23(1):14–21.10.1016/S1001-0742(10)60368-3
- Amde M, Yin YG, Zhang D, et al. Methods and recent advances in speciation analysis of mercury chemical species in environmental samples: a review. Chem Speciation Bioavail. 2016;28(1–4):51–65.
- Mergler D, Anderson HA, Chan LHM, et al. Methylmercury exposure and health effects in humans: a worldwide concern. Ambio. 2007;36(1):3–11.
- Wang S, Zhang L, Wang L, et al. A review of atmospheric mercury emissions, pollution and control in China. Front Environ Sci Eng. 2014;8(5):631–649.10.1007/s11783-014-0673-x
- Stafiej A, Pyrzynska K. Adsorption of heavy metal ions with carbon nanotubes. Sep Purif Technol. 2007;58(1):49–52.10.1016/j.seppur.2007.07.008
- Rao GP, Lu C, Su F. Sorption of divalent metal ions from aqueous solution by carbon nanotubes: a review. Sep Purif Technol. 2007;58(1):224–231.10.1016/j.seppur.2006.12.006
- Upadhyayula VKK, Deng S, Mitchell MC, et al. Application of carbon nanotube technology for removal of contaminants in drinking water: a review. Sci Total Environ. 2009;408(1):1–13.10.1016/j.scitotenv.2009.09.027
- Ihsanullah, Abbas A, Al-Amer AM, et al. Heavy metal removal from aqueous solution by advanced carbon nanotubes: critical review of adsorption applications. Sep Purif Technol. 2016;157:141–161.10.1016/j.seppur.2015.11.039
- Li YH, Ding J, Luan Z, et al. Competitive adsorption of Pb2+, Cu2+ and Cd2+ ions from aqueous solutions by multiwalled carbon nanotubes. Carbon. 2003;41(14):2787–2792.10.1016/S0008-6223(03)00392-0
- Li YH, Wang S, Luan Z, et al. Adsorption of cadmium(II) from aqueous solution by surface oxidized carbon nanotubes. Carbon. 2003;41(5):1057–1062.10.1016/S0008-6223(02)00440-2
- Li YH, Wang S, Wei J, et al. Lead adsorption on carbon nanotubes. Chem Phys Lett. 2002;357(3–4):263–266.10.1016/S0009-2614(02)00502-X
- Lu C, Chiu H, Liu C. Removal of zinc(II) from aqueous solution by purified carbon nanotubes: kinetics and equilibrium studies. Ind Eng Chem Res. 2006;45(8):2850–2855.10.1021/ie051206h
- Shadbad MJ, Mohebbi A, Soltani A. Mercury(II) removal from aqueous solutions by adsorption on multi-walled carbon nanotubes. Korean J Chem Eng. 2011;28(4):1029–1034.10.1007/s11814-010-0463-5
- Vuković GD, Marinković AD, Škapin SD, et al. Removal of lead from water by amino modified multi-walled carbon nanotubes. Chem Eng J. 2011;173(3):855–865.10.1016/j.cej.2011.08.036
- Jiang L, Li S, Yu H, et al. Amino and thiol modified magnetic multi-walled carbon nanotubes for the simultaneous removal of lead, zinc, and phenol from aqueous solutions. Appl Surf Sci. 2016;369:398–413.10.1016/j.apsusc.2016.02.067
- Chen PH, Hsu CF, Tsai DDW, et al. Adsorption of mercury from water by modified multi-walled carbon nanotubes: adsorption behaviour and interference resistance by coexisting anions. Environ Technol. 2014;35(15):1935–1944.10.1080/09593330.2014.886627
- Yaghmaeian K, Khosravi Mashizi R, Nasseri S, et al. Removal of inorganic mercury from aquatic environments by multi-walled carbon nanotubes. J Environ Health Sci Eng. 2015;13(1):1–9.
- Lim JK, Yun WS, Yoon Mh, et al. Selective thiolation of single-walled carbon nanotubes. Synth Met. 2003;139(2):521–527.10.1016/S0379-6779(03)00337-0
- Hu J, Shi J, Li S, et al. Efficient method to functionalize carbon nanotubes with thiol groups and fabricate gold nanocomposites. Chem Phys Lett. 2005;401(4–6):352–356.10.1016/j.cplett.2004.11.075
- Kim YT, Mitani T. Surface thiolation of carbon nanotubes as supports: a promising route for the high dispersion of Pt nanoparticles for electrocatalysts. J Catal. 2006;238(2):394–401.10.1016/j.jcat.2005.12.020
- Feyte S, Tessier A, Gobeil C, et al. In situ adsorption of mercury, methylmercury and other elements by iron oxyhydroxides and organic matter in lake sediments. Appl Geochem. 2010;25(7):984–995.10.1016/j.apgeochem.2010.04.005
- Hyung H, Kim JH. Natural organic matter (NOM) adsorption to multi-walled carbon nanotubes: effect of NOM characteristics and water quality parameters. Environ Sci Technol. 2008;42(12):4416–4421.10.1021/es702916h
- Hyung H, Fortner JD, Hughes JB, et al. Natural organic matter stabilizes carbon nanotubes in the aqueous phase. Environ Sci Technol. 2007;41(1):179–184.10.1021/es061817g
- Bandaru NM, Reta N, Dalal H, et al. Enhanced adsorption of mercury ions on thiol derivatized single wall carbon nanotubes. J Hazard Mater. 2013;261:534–541.10.1016/j.jhazmat.2013.07.076
- Tawabini B, Al-Khaldi S, Atieh M, et al. Removal of mercury from water by multi-walled carbon nanotubes. Water Sci Technol. 2010;61(3):591–598.10.2166/wst.2010.897
- Sheela T, Nayaka YA, Viswanatha R, et al. Kinetics and thermodynamics studies on the adsorption of Zn(II), Cd(II) and Hg(II) from aqueous solution using zinc oxide nanoparticles. Powder Technol. 2012;217:163–170.10.1016/j.powtec.2011.10.023
- Wang ZH, Yin YG, He B, et al. L-cysteine-induced degradation of organic mercury as a novel interface in the HPLC-CV-AFS hyphenated system for speciation of mercury. J Anal At Spectr. 2010;25(6):810–814.10.1039/b924291k
- Zhao XH, Jiao FP, Yu JG, et al. Removal of Cu(II) from aqueous solutions by tartaric acid modified multi-walled carbon nanotubes. Colloid Surf A-Physicochem Eng. 2015;476:35–41.10.1016/j.colsurfa.2015.03.016
- Gupta A, Vidyarthi SR, Sankararamakrishnan N. Enhanced sorption of mercury from compact fluorescent bulbs and contaminated water streams using functionalized multiwalled carbon nanotubes. J Hazard Mater. 2014;274:132–144.10.1016/j.jhazmat.2014.03.020
- Hadavifar M, Bahramifar N, Younesi H, et al. Adsorption of mercury ions from synthetic and real wastewater aqueous solution by functionalized multi-walled carbon nanotube with both amino and thiolated groups. Chem Eng J. 2014;237:217–228.10.1016/j.cej.2013.10.014
- Xu D, Tan X, Chen C, et al. Removal of Pb(II) from aqueous solution by oxidized multiwalled carbon nanotubes. J Hazard Mater. 2008;154(1–3):407–416.10.1016/j.jhazmat.2007.10.059
- Zabihi M, Ahmadpour A, Asl AH. Removal of mercury from water by carbonaceous sorbents derived from walnut shell. J Hazard Mater. 2009;167(1–3):230–236.10.1016/j.jhazmat.2008.12.108
- Moghaddam HK, Pakizeh M. Experimental study on mercury ions removal from aqueous solution by MnO2/CNTs nanocomposite adsorbent. J Ind Eng Chem. 2015;21:221–229.10.1016/j.jiec.2014.02.028
- Scheibe B, Borowiak-Palen E, Kalenczuk RJ. Oxidation and reduction of multiwalled carbon nanotubes – preparation and characterization. Mater Charact. 2010;61(2):185–191.10.1016/j.matchar.2009.11.008
- Vuković G, Marinković A, Obradović M, et al. Synthesis, characterization and cytotoxicity of surface amino-functionalized water-dispersible multi-walled carbon nanotubes. Appl Surf Sci. 2009;255(18):8067–8075.10.1016/j.apsusc.2009.05.016
- Ren X, Chen C, Nagatsu M, et al. Carbon nanotubes as adsorbents in environmental pollution management: A review. Chem Eng J. 2011;170(2–3):395–410.10.1016/j.cej.2010.08.045
- Ma Y, La P, Lei W, et al. Adsorption of Hg(II) from aqueous solution using amino-functionalized graphite nanosheets decorated with Fe3O4 nanoparticles. Desalin Water Treat. 2016;57(11):5004–5012.10.1080/19443994.2014.998292
- Pillay K, Cukrowska EM, Coville NJ. Multi-walled carbon nanotubes as adsorbents for the removal of parts per billion levels of hexavalent chromium from aqueous solution. J Hazard Mater. 2009;166(2–3):1067–1075.10.1016/j.jhazmat.2008.12.011
- Hintelmann H, Welbourn PM, Evans RD. Measurement of complexation of methylmercury(II) compounds by freshwater humic substances using equilibrium dialysis. Environ Sci Technol. 1997;31(2):489–495.10.1021/es960318k
- Kushwaha S, Sreedhar B, Padmaja P. Sorption of phenyl mercury, methyl mercury, and inorganic mercury onto chitosan and barbital immobilized chitosan: spectroscopic, potentiometric, kinetic, equilibrium, and selective desorption studies. J Chem Eng Data. 2010;55(11):4691–4698.10.1021/je100317t
- Kushwaha S. Sudhakar PP. Adsorption of mercury(II), methyl mercury(II) and phenyl mercury(II) on chitosan cross-linked with a barbital derivative. 2011;86(2):1055–1062.
- Jeong HY, Klaue B, Blum JD, et al. Sorption of mercuric ion by synthetic nanocrystalline mackinawite (FeS). Environ Sci Technol. 2007;41(22):7699–7705.10.1021/es070289l
- Lu C, Su F. Adsorption of natural organic matter by carbon nanotubes. Sep Purif Technol. 2007;58(1):113–121.10.1016/j.seppur.2007.07.036
- de Diego A, Tseng CM, Dimov N, et al. Adsorption of aqueous inorganic mercury and methylmercury on suspended kaolin: influence of sodium chloride, fulvic acid and particle content. Appl Organomet Chem. 2001;15(6):490–498.10.1002/(ISSN)1099-0739