ABSTRACT
Fungal infection and mycotoxins contamination in food and feed products cause significant economic losses to the food and agricultural industry. Although the efficacy of synthetic fungicides is unquestionable, there are serious issues associated with their application in agriculture. Improper and/or prolonged application of these products may cause the emergence of resistant fungal populations, carry-over of chemicals in the human diet and adverse effects on non-target species. By contrast, ease in application and negligible effects on the environment makes microbial volatile organic compounds (VOCs) safe and sustainable substitutes to synthetic fungicides. A considerable research investment has highlighted the efficacy and suitability of VOCs emitted by bacteria, yeast and filamentous fungi for application in food crops and stored products. In this review, focus is made on the potential use of microbial VOCs as inhibitors of toxigenic food mycobiota and their mycotoxins. The mode of action of microbial volatile compounds, possible application in different scenarios, limitations and perspectives are discussed.
1. Introduction
Fungal infections of agricultural crops and their products trigger significant losses to the food and agricultural compartments (Alina, Citation2022; Shabeer et al., Citation2022; Xu et al., Citation2022). The old Food and Agriculture Organization (FAO) notion ‘25% of the food is annually lost due to fungal infection’ is in fact true and even slightly underestimates the actual damage (Almeida et al., Citation2019).
Apart from the mold-associated spoilage, infections of food products by mycotoxin-producing species results in the buildup of toxins (Al Attiya et al., Citation2021; Hassan et al., Citation2019; Citation2018; Ul Hassan et al., Citation2018; Xu et al., Citation2022). An ever-growing list of mycotoxins of human health significance includes mainly those produced by filamenteous fungi. The toxic effects of mycotoxins could be as mild as short-duration gastrointestinal disorders to chronic (immunosuppression, growth retardation, reproductive disorders) and acute (hepatotoxicity, nephrotoxicity, neurological disorders and deaths) in the exposed animal and humans (Alshannaq & Yu, Citation2017; Cimbalo et al., Citation2020; Dey et al., Citation2022). These effects are generally severe in the vulnerable communities, including infants, elderly and immunocompromised patients (Omotayo et al., Citation2019).
Integrated control measures against fungi are proposed at all stages of agricultural production to minimise the damage. Best management practices at pre-harvest stages, including crop rotation, resistant crops, proper sowing and watering time, use of health propagative material and application of fungicides can help dropping the magnitude of the issue (Santiago et al., Citation2015; Shabeer et al., Citation2022; Tilocca et al., Citation2019). At post-harvest stages, there are several approaches (physical, chemical and biological) to preserve food from fungal attack and accumulation of mycotoxin (Ji et al., Citation2016; Sadiq et al., Citation2019). The inappropriate application of synthetic fungicides has led to serious concerns regarding the environment and public health (Tilocca et al., Citation2020). Moreover, the emergence of fungicide-resistant fungal strains is largely linked with the improper use of synthetic antifungal compounds in the field and at the postharvest stage (Fisher et al., Citation2022).
Physical methods, such as binding agents, UV exposure, sorting, cleaning, grading, washing, etc. are also adopted to reduce contamination with mycotoxins in susceptible agricultural commodities, albeit their effectiveness may vary according to the targeted toxin and of the food/feed material (Kolosova & Stroka, Citation2012; Sipos et al., Citation2021).
Several antagonistic bacteria, filamentous fungi and yeast strains have been registered and biocontrol commercial products are being marketed for the protection of food commodities subject to mycotoxin contamination (Freimoser et al., Citation2019; Moore, Citation2022; Moral et al., Citation2020; Tilocca et al., Citation2020). Antifungal formulations utilising non-aflatoxigenic Aspergillus flavus strains, e.g. Alfa-Guard (atoxigenic A. flavus), FUSAclean (non-pathogenic Fusarium oxysporum), AF36® (atoxigenic natural A. flavus) and Aflasafe® (four atoxigenic A. flavus strains) are currently being used with significant reductions in aflatoxins (AFs) in corn, peanuts, cotton seeds and other agricultural crops (Kagot et al., Citation2019). In addition to filamentous fungi, antagonistic yeast (Cryptococcus albidus, Aureobasidium pullulans, Metschnikowia fructicola and Saccharomyces cerevisiae and Candida oleophila,) and bacterial strains (Streptomyces spp., lactic acid bacteria (LAB), Pseudomonas spp., Bacillus spp.,) are registered for their antifungal commercial use in agricultural and food industry (Freimoser et al., Citation2019; Salas et al., Citation2017).
Until recently it was known that most of the biocontrol strains employ competition for space and nutrients as a major mechanism of the antagonism. However, during the last two decades, the focus of attention has shifted from competition to volatile-mediated interaction between the target fungi and biocontrol agent. For instance, non-toxigenic strains of A. flavus used in three commercial formulations (Afla-Guard®, AF36®, Aflasafe®) were thought to compete with the toxigenic A. flavus for the space and nutrients as a mechanism of their antagonism (Bandyopadhyay et al., Citation2019). However, Moore et al. (Citation2021, Citation2022) have recently demonstrated that the volatiles released by non-aflatoxigenic A. flavus are also involved in this interaction.
Volatile organic compounds (VOCs) are characterised by their low solubility in water and high vapour pressure ≥10 Pa (Lammers et al., Citation2022; Tilocca et al., Citation2020) and play diverse physiological roles in the environment (Weisskopf et al., Citation2021). The use of volatiles for human benefits started with their application as perfumes, flavouring agents in food and developing specific aroma and taste of food products (Ebert et al., Citation2017; Verma et al., Citation2022). The volatile profile of microbes depends on several characteristics including the age of the microbes, physical parameters (temperature, pressure, humidity), presence of other organisms in their environment, aeration and the method of collection (Insam & Seewald, Citation2010). Biotechnological application of microbial volatiles was a neglected area of research, particularly due to lack of sophisticated collection and analytical methods (Weisskopf et al., Citation2021). However, during the past decade, application of VOCs in the food, agriculture, pharmaceutical and medicine industry has been largely explored (Lammers et al., Citation2022; Weisskopf et al., Citation2021).
In this review, we will summarise recent research achievements on the role of microbial (bacteria, yeast and filamentous fungi) volatiles in the control of mycotoxigenic fungi and their metabolites in food preservation, with emphasis on pre- and post-harvest damage. Furthermore, the potential utilisation of microbial volatiles in food preservation, their mode of action, limitations and perspective are discussed.
2. Biosynthesis of microbial volatiles
Like other living organisms, microbes also produce complex arrays of volatiles and secrete them during their routine metabolic processes. These molecules are regarded as products of their metabolism (Schmidt et al., Citation2015; Weisskopf et al., Citation2021) and are broadly classified as inorganic and organic compounds (Lammers et al., Citation2022; Schulz-Bohm et al., Citation2017). The major classes of organic volatiles of microbial origin include aromatic compounds, alkanes, alkenes, fatty acids, ester, alcohols, methyl ketones, aldehydes, ketones and short-chain fatty acids (Schmidt et al., Citation2015; Tilocca et al., Citation2020; Veselova et al., Citation2019; Weisskopf et al., Citation2021). The synthesis of these volatiles involves catabolic processes () such as glycolysis, lipolysis and proteolysis (Freimoser et al., Citation2019; Veselova et al., Citation2019).
Figure 1. Pathways involved in the synthesis of microbial volatiles implicated in the antagonism of toxigenic fungi and mycotoxins. Dotted lines indicate the steps involved in the synthesis of known antifungal volatiles, while continuous line indicate non-volatiles intermediate or final products. This figure presents the modification of pathways previously illustrated by Schmidt et al. (Citation2015) and Veselova et al. (Citation2019).
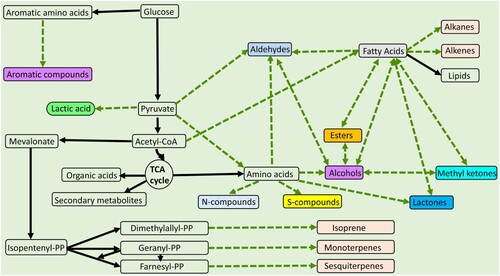
In general, microbial volatiles molecules across Kingdom and within Kingdom are common in their nature (Mari et al., Citation2016; Salas et al., Citation2017; Tilocca et al., Citation2020). However, some molecules are peculiar to a microbial group or even a single strain, making them unique in their physicochemical role (Schmidt et al., Citation2015). Below, we provide a brief summary on overall biosynthesis of volatiles produced by bacteria, yeast and filamentous fungi.
During the aerobic cell environment, pyruvate is converted to acetyl-CoA and enters into fatty acid metabolism or tricarboxylic acid (TCA) cycle. Fatty acid metabolism generates alkanes, alkenes, ketones and aliphatic alcohols (Schmidt et al., Citation2015). Even numbered fatty acids are produced with the β-oxidation of acetyl-CoA. Fatty acids, aldehydes and ketones are the typical volatile end products of fatty acid pathways (Lammers et al., Citation2022). Aromatic compounds, such as 2-phenylethanol (2-PE) are produced mainly through the shikimate pathway and are particularly important in the antifungal activities. For example, several studies highlighted the production of 2-phenylethanol (2-PE) by bacteria (Weisskopf et al., Citation2021), yeast (Farbo et al., Citation2018), and filamentous fungi (Medina-Romero et al., Citation2017), and its inhibitory role against toxigenic fungi and their mycotoxins. Sulphur compounds are important constituents of microbial volatilome and are synthesised from the methanethiol and hydrogen sulphide. Dimethyl disulphide is a dimer of methanethiol which is commonly detected in gas chromatography (GC) volatile profiles of many bacteria (Brock et al., Citation2014). Other sources of sulphur compounds are acids, such as methyl-butyric acid. Terpene hydrocarbons are diverse groups of volatiles produced by fungi and bacteria and have been implicated in their antifungal mode of action (Corcuff et al., Citation2011; Medina-Romero et al., Citation2017; Tenorio-Salgado et al., Citation2013). Terpenes are synthesised by three pathways; (a) the mevalonate pathway, which was long being considered the only source of isopentenyl diphosphate and dimethylallyl diphosphate. This pathway starts with glycolysis leading to acetyl-CoA and isopentenyl diphosphate, which subsequently results in the production of terpenes (Freimoser et al., Citation2019). The other two pathways are leucine and the 2-methylerythritol-4-phosphate (Veselova et al., Citation2019) which also results in the synthesis of terpenes (isoprene, monoterpenes and sesquiterpenes). Along with organic compounds, microbes also produce inorganic molecules such as carbon monoxide, carbon dioxide, hydrogen cyanide, oxygen, hydrogen, hydrogen sulphide, ammonia, nitrogen dioxide, sulphur dioxide, etc. (Tilocca et al., Citation2020; Veselova et al., Citation2019).
3. Bacteria
3.1. Antifungal bacterial volatilome
Organic compounds of bacterial origin are characterised by acids, alcohols, terpenes, benzenoids, acids, ketones, esters, pyrazines and alkenes (Veselova et al., Citation2019; Weisskopf et al., Citation2021). These molecules alone or their consortia play significant antifungal activity against toxigenic fungi and mycotoxins during pre- and post-harvest stages of food systems (Chalivendra & Ham, Citation2019; Lammers et al., Citation2022; Veselova et al., Citation2019). Likewise, bacteria also produce inorganic volatiles such as carbon monoxide (CO), carbon dioxide (CO2), hydrogen cyanide (HCN), oxygen (O2), hydrogen (H2), hydrogen sulphide (H2S), ammonia (NH3), nitrogen dioxide (NO2), sulphur dioxide (SO2), etc., play diverse roles including quorum sensing, electron donor or receptor and as defence molecules (Tilocca et al., Citation2020). Below is a detailed account of microbial profiles of different bacterial genera, with emphasis on their antagonistic activities against mycotoxigenic fungi.
3.1.1. Bacillus spp.
Bacillus spp. as a biocontrol agent have several advantages over the other microbial genera because of their convenience in application, environmental safety (with few exceptions), resilience to harsh conditions, genetic diversity, broad range of antifungal activity and minimal nutritional requirements (Chalivendra & Ham, Citation2019). These benefits associated with Bacilli place them on top of the list of commercialised biocontrol agents (Effmert et al., Citation2012). In the last few decades, there are many novel reports on the role of Bacillus spp. against toxigenic fungi and mycotoxin biosynthesis in food commodities (). The main species of Bacillus implicated in biocontrol of toxigenic yeast are B. amyloliquefaciens (Arrebola et al., Citation2010; Chaves-López et al., Citation2015; Shi et al., Citation2014; Yuan et al., Citation2012), B. subtilis (Arrebola et al., Citation2010; Chaves-López et al., Citation2015), B. velezensis G341 (Calvo et al., Citation2020; Lim et al., Citation2017), B. cereus (Chaves-López et al., Citation2015), B. methylotrophicus (He et al., Citation2020), B. thuringiensis (He et al., Citation2020), B. licheniformis (Ul Hassan et al., Citation2019), B. megaterium (Mannaa & Kim, Citation2018; Saleh et al., Citation2021), B. simplex (Al Attiya et al., Citation2021) and B. pumilus (Morita et al., Citation2019). The prominent antifungal volatile molecules synthesised by Bacillus spp., include (but not limited to) 2,3-butanediol, 3-hydroxy-2-butanone (Ryu et al., Citation2003), tetradecane, pentadecane (Yuan et al., Citation2012), 3-hydroxy-2-butanone, dimethylsulfoxide, 1-butanol, (Lim et al., Citation2017), 3-methyl-1-butanol (Ul Hassan et al., Citation2019), diacetyl, 2-undecanone, 2-nonanone, 1-butanol, 2-heptanone, benzaldehyde, acetoin (Calvo et al., Citation2020), quinoline, benzenemethanamine 1-octadecene (Al Attiya et al., Citation2021), tetracosane, palmitic acid (Saleh et al., Citation2021), 1-butanol, propanone, acetic acid, 2-methylpropanoic acid, ethyl acetate, carbon disulphide (Chaves-López et al., Citation2015), 3-hydroxy-2-butanone (acetoin) (Arrebola et al., Citation2010), ethanol, S-()-2-methylbutylamine, methyl isobutyl ketone (Morita et al., Citation2019), 2-butyl 1-octanol, 4-trifluoroacetyl hexadecane, 5-methyl-2-phenyl-1H-indole and dimethyl disulphide (Mannaa & Kim, Citation2018).
Table 1. Summary of antagonistic bacterial species/strains, their target toxigenic fungi, bioactive volatile molecule(s) and experimental application.
In a study by Shi et al. (Citation2014), B. amyloliquefaciens isolated from the peanut shell inhibited F. graminearum by 41.4–54.5% in co-culture assay and by 92.7–100% in tip-culture assay. Additionally, upon in-vivo application on wheat kernels, this strain inhibited deoxynivalenol (DON) synthesis by 16.69–90.30%. Ul Hassan et al. (Citation2019) demonstrated that volatiles (mainly 3-methyl-1-butanol) emitted by B. licheniformis inhibited all tested toxigenic Aspergillus and Penicillium species. A. westerdijkiae showed high sensitivity to Bacillus licheniformis strain 350-2 (BL350-2) volatiles with an inhibition ratio of 62%, while other representative strains including A. carbonarius, A. ochraceus, A. niger, A. parasiticus, A. flavus and P. verrrucosum were all inhibited at 44–60% compared to unexposed controls. In in vivo testing on maize ears, volatile of BL350-2 showed 88% inhibition of A. flavus and complete inhibition of AFs biosynthesis. In a study by Saleh et al. (Citation2021), we found that a B. megaterium strain (BM344-1) producing tetracosane and palmitic acid significantly inhibited toxigenic P. verrucosum, A. flavus, and F. verticillioides by 67, 29% and 18%, respectively. Upon exposure to BM344-1 volatiles, there was 100% inhibition of AFs, ochratoxin A (OTA) and fumonisins (FUMs) synthesis by the three tested toxigenic fungi. In vivo tests performed on maize ears showed that the inhibition of A. flavus (51%) by BM344-1 was higher, whereas the effect on mycotoxin biosynthesis was lower compared to artificial media. These differences strongly suggest that antifungal activities of bacterial strains are associated with other factors, such as the effect of substrate and the experimental setup.
The volatiles of B. simplex BS350-3 showed 1-octadecene, benzenemethanamine and quinoline as the most bioactive molecules against toxigenic A. carbonarius and A. flavus on coffee beans (Al Attiya et al., Citation2021). The level of AFs contamination in artificially infected coffee beans was significantly lower (26.65 ± 3.33 μg/kg) in the existence of BS350-3 volatiles, compared to the unexposed control coffee beans (63.60 ± 8.52 μg/kg). Likewise, BS350-3 volatiles significantly inhibited OTA contamination at 1.88 ± 0.33 μg/kg by A. carbonarius as compared to unexposed infected coffee beans, where the level was 4.13 ± 0.81 μg/kg (Al Attiya et al., Citation2021).
3.1.2. Lactic acid bacteria (LAB)
LAB constitute acid-tolerant, heterogenous bacteria producing acid as an end product of carbohydrate fermentation (Salas et al., Citation2017), and are found in diverse ecological niches including soil, human body, animals and plants (Broberg et al., Citation2007; Crowley et al., Citation2013). LAB contribute significantly to agriculture industry in production and preservation of food, particularly in the control of toxigenic fungi (Chalivendra & Ham, Citation2019; Dopazo et al., Citation2022; Gallo et al., Citation2021, Citation2022; Sadiq et al., Citation2019). In accordance with their GRAS (Generally Considered as Safe) status, and some probiotic properties (Crowley et al., Citation2013), LAB are regarded as green preservatives (Nasrollahzadeh et al., Citation2022). The mode of action of LAB are diverse and involves the secretion of lytic enzymes, production of antifungal volatiles, competition for nutrients and space, degradation of produced mycotoxins, etc. (Nešić et al., Citation2021; Tilocca et al., Citation2020). LAB volatilome comprises a long list of antifungal molecules including 6-octadecenoic acid methyl ester, pentadecane, 7-hexadecenal, phenol, 2-methyldecane, hexadecanoic acid methyl ester, dotriacontane (Shehata et al., Citation2019), acetoin, diacetyl (Diaz et al., Citation2021), butyl phenol (Varsha et al., Citation2015), cyclo (L-Phe-trans-4-OH-L-Pro), 3-phenyllactic acid (Ström et al., Citation2002), polyporic acid (Valerio et al., Citation2016), 3,6-bis(2-methylpropyl)-2,5-piperazinedion (Yang & Chang, Citation2010), 3-phenyllactic acid, 3-hydroxydecanoic acid (Broberg et al., Citation2007), monohydroxy octadecenoic acid, coriolic acid (Black et al., Citation2013) and acetic acid, propionic acid, 1,2 propanediol (Gallo et al., Citation2021, Citation2022).
Antifungal volatiles of LAB () were shown to inhibit toxigenic Aspergillus (Broberg et al., Citation2007; Gallo et al., Citation2022; Shehata et al., Citation2019), Penicillium (Aunsbjerg et al., Citation2015; Yang & Chang, Citation2010) and Fusarium (Diaz et al., Citation2021; Ström et al., Citation2002; Varsha et al., Citation2015), as well as their ability to produce mycotoxins. Acetoin and diacetyl were identified as bioactive volatile molecules of Enterococcus faecium and Enterococcus casseliflavus which resulted in 33% and 10% growth inhibition of F. verticillioides, respectively. In this study, Enterococcus spp. also inhibited FB1 synthesis by 89% (Diaz et al., Citation2021). In another study, VOCs of Lactobacillus sp. RM1 showed antagonistic activities against toxigenic fungi and AFs and OTA synthesis in vitro. On wheat grains, A. parasiticus was completely inhibited by bacterial volatiles for at least 2 weeks of co-incubation. These antagonistic effects were mainly due to 6-octadecenoic acid methyl ester, 2-methyldecane, pentadecane, 7-hexadecenal, phenol, hexadecanoic acid methyl ester, dotriacontane (Shehata et al., Citation2019). Lactobacillus paracasei strain DGCC 2132 inhibited P. solitum and Penicillium sp. by producing a volatile (diacetyl) at pH 6.5 and 4.5, respectively (Aunsbjerg et al., Citation2015). A defined volume (200 μg/mL) of commercial diacetyl successfully inhibited both fungi for 14 and 20 days, respectively. A summarised account of antifungal activities of different LAB is presented in .
3.1.3. Pseudomonas spp.
There are several studies on the inhibitory role of volatiles synthesised and released by Pseudomonas spp., against toxigenic fungi (Cordero et al., Citation2014; Lal et al., Citation2022; Mannaa et al., Citation2017; Mannaa & Kim, Citation2018; Nazik et al., Citation2020; Ossowicki et al., Citation2017; Wang et al., Citation2021). The VOCs mix of some hydrocarbons emitted by Pseudomonas fluorescens MGR12 significantly inhibited F. proliferatum both on rich and minimal media (Cordero et al., Citation2014). Nazik et al. (Citation2020) performed series of experiments to explore the role of Pseudomonas aeruginosa volatiles against different representative strains of A. flavus and A. fumigatus. Only in the volatile mix collected from the antagonistic strains a number of bioactive molecules were identified, including methyl thiobutyrate, 2-undecanone, methyl thiocyanate, methyl thio isovalerate, heptane, 2-heptanone, decanone, mercaptoacetone, 2-undecanol, 1-undecanol, 2-tridecanone and 2-dodecanone. Among these, methyl thiobutyrate, 2-undecanone and 2-heptanone, when tested alone, showed strong antifungal activity against the tested Aspergilli. In contrast to the reported results of Scott et al. (Citation2019), Nazik et al. (Citation2020) concluded that VOCs released by P. aeruginosa never promote A. fumigatus growth ().
In another investigation, Ossowicki et al. (Citation2017) reported the emission of volatiles active against F. culmorum by a soil isolate of Pseudomonas donghuensis P482. The list of molecules showing in vitro inhibitory effects includes S-methyl thioacetate, dimethyl sulphide, dimethyl trisulphide, 1-undecan, methyl thiocyanate and HCN.
3.1.4. Streptomyces spp.
Streptomyces was believed to be the largest antimicrobial producing genus in the twentieth century (Watve et al., Citation2001), and hence there exist several registered commercial products based on Streptomyces strains (Bubici, Citation2018). Biocontrol activities of Streptomyces spp., volatiles against toxigenic Aspergillus, Penicillium, Fusarium and Alternaria are documented in several studies (Cho et al., Citation2017; Corral et al., Citation2020; Li et al., Citation2010; Lyu et al., Citation2020; Wang et al., Citation2013). In an in vitro assay, the volatiles emitted by Streptomyces alboflavus significantly inhibited A. flavus, F. moniliforme, A. niger, P. citrinum and A. ochraceus. A major component of S. alboflavus TD-1 volatilome was identified as 2-methylisoborneol by GCMS (Wang et al., Citation2013). Similarly, Corral et al. (Citation2020) tested the volatiles of four maize and apple isolates of Streptomyces (S. kanamyceticus, S. misionensis, S. cangkringensis and S. kanamyceticus) on Alternaria alternata and F. solani. Bacterial volatiles (trans-2-hexenal as a major molecule) inhibited the fungal growth up to 96.7%. Lyu et al. (Citation2020) exposed toxigenic A. parasiticus and A. flavus to the VOCs emitted by Streptomyces yanglinensis strain 3–10, both in vitro and on peanuts kernels. Bacterial volatiles repressed the expression of AFs biosynthesis genes along with fungal sporulation, vegetative growth and conidial germination. Likewise, on peanut kernels, bacterial volatiles showed significant inhibition of both fungal infection and AFs biosynthesis. GCMS-based analysis showed high concentrations of 2-methyl-2-bornene and 2-methylisoborneol in bacterial volatiles among 19 other compounds. Commercial methyl 2-methylbutyrate showed the highest in vitro activity, suggesting it as a major responsible for inhibition. S. alboflavus TD-1, an isolate from wheat bran produced two antifungal volatiles (dimethyl trisulphide and benzenamine), which strongly inhibited A. flavus by suppressing growth of fungi and its AFs biosynthetic potential, as evidenced by downregulation of key AFs synthesis genes (Yang et al., Citation2019).
3.1.5. Burkholderia spp.
The volatiles of a few species in the genus Burkholderia including, Burkholderia ambifaria (Groenhagen et al., Citation2013), Burkholderia tropica (Tenorio-Salgado et al., Citation2013), Burkholderia gladioli (Elshafie et al., Citation2012) and Burkholderia cenocepacia (Chen et al., Citation2020) have shown to produce inhibitory effects on toxigenic fungi and their mycotoxin synthesis (). Elshafie et al. (Citation2012) reported the production of 1-methyl-4-(1-methylethenyl)-cyclohexene, an antifungal volatile by B. gladioli active against a range of toxigenic fungi such as A. flavus, A. niger, and P. expansum. Likewise, Chen et al. (Citation2020) reported the antagonistic activity of B. cenocepacia against F. solani and A. niger by producing volatile inhibitory molecules including indole, dimethyl trisulphide, methyl salicylate, methyl benzoate, methyl anthranilate, benzyl acetate, allyl benzyl ether and nonanoic acid. Like other bacteria, the volatiles of Burkholderia showed inhibitory activities against toxigenic Aspergillus, Fusarium, Alternaria and Penicillium species (Chen et al., Citation2020; Elshafie et al., Citation2012; Groenhagen et al., Citation2013; Tenorio-Salgado et al., Citation2013).
3.2. Antagonistic mechanism of bacterial volatiles
The precise mechanism of interaction of bacterial volatiles with toxigenic fungi resulting in the suppression of fungal growth and mycotoxin biosynthesis is not well outlined. However, diverse effects are reported on the fungal strains as well as the host commodity/crop to produce biological effects (Schmidt et al., Citation2016, Citation2015; Tilocca et al., Citation2020). In laboratory settings, inhibition of fungal growth and/or mycotoxin production are primary criteria to declare/screen the biological agents (Al Attiya et al., Citation2021; Saleh et al., Citation2021; Ul Hassan et al., Citation2019). In reality, the composition of volatiles produced by any antagonistic strain is regulated by several physicochemical parameters including the nature of microbial strains, substrate composition, existence of other microbes, environmental conditions (temperature, vapour pressure, water activity) and the age of bacterial cells (Corcuff et al., Citation2011; Effmert et al., Citation2012; Ezra & Strobel, Citation2003; Lammers et al., Citation2022). To achieve optimal antifungal activity, it’s critical to validate specific physicochemical conditions and environmental attributes. For instance, at 0.98 aw, 20 out of 59 Bacillus isolates inhibited both A. flavus and A. parasiticus. However, on lower 0.95 aw, only 3 isolates showed their effectiveness (Bluma & Etcheverry, Citation2006). Similarly, on maize kernel extract agar, Bacillus isolates from maize field displayed a higher inhibition of F. verticillioides and A. flavus compared to the same strains being cultured on PDA, suggesting specific nutritional requirements for optimal metabolite biosynthesis (Palumbo et al., Citation2007). The lack of inhibitory activity by candidate/known biocontrol agents on popular growth media are likely due to unavailability of desired nutrients (Chalivendra et al., Citation2018), leading to poor growth and toxic metabolite synthesis. Under such stress situations, antagonistic bacterial strains may also produce growth-promoting substances and increase disease severity as well as mycotoxin production (Cray et al., Citation2016). Additionally, microbes needing nutrient-rich media for the production of inhibitory molecules might not be an economically viable choice for field application (Chalivendra & Ham, Citation2019).
The reduction of mycelium growth may not necessarily be associated with the inhibition of mycotoxin biosynthesis. There are several reports on enhanced mycotoxicity by Fusarium and Aspergillus when mycelial growth was inhibited (Santiago et al., Citation2015). Chalivendra et al. (Citation2018) tested 29 Bacillus isolates for their antifungal and anti-aflatoxigenic potential on A. flavus. In total, 3 isolates inhibited A. flavus growth, while 26 were able to suppress AFs synthesis. These findings suggest that screening for biocontrol activity should include both the effect on fungal growth as well as on mycotoxin biosynthesis, to reduce the chances of losing effective strains. A mycotoxin biocontrol cocktail could include microbial strains with different inhibitory potential over a range of species. Another possibility is to include microbial strains with strong mycotoxin inhibiting (but no antifungal) activity with fungicides for a robust toxin control measure (Ons et al., Citation2020).
HCN, a common inorganic volatile, leads to production of cyanide ions, which are known inhibitors of metallic enzymes such as cytochrome c oxidases. The inhibition of key fungal enzymes consequently leads to a cascade of events passing over the impaired function and finally death (Effmert et al., Citation2012). Antifungal effects include the suspension of hyphal growth inducing cell injury, thereby affecting mycotoxins synthesis and stability (Chalivendra & Ham, Citation2019). In many fungi-bacteria interactions, it has been reported that exposed fungi recover once removed from the bacterial volatiles environment, suggesting a clear fungistatic effect, while in others the effects of bacterial compounds are irreversible (Chalivendra & Ham, Citation2019; DeFilippi et al., Citation2018).
Bacterial volatiles interact with cell wall of target fungi by releasing enzymes as reported by Tamreihao et al. (Citation2016). In a study, on exposure to caryolan-1-ol (a major volatile) of Streptomyces spp, Cho et al. (Citation2017) reported inhibition of endomembrane systems of target fungi.
4. Yeast
4.1. Antifungal yeast volatilome
Application of yeast volatile molecules in the protection of food crops and their products have several advantages over synthetic fungicides (Freimoser et al., Citation2019; Tilocca et al., Citation2020). Yeasts in general are easy to cultivate in cheaper growth media without costly equipment, are safer to handle in BSL1 facilities and are Generally Regarded As Safe (GRAS) organisms (Breuer & Harms, Citation2006; Ebert et al., Citation2017; Papp et al., Citation2021; Spadaro & Droby, Citation2016). summarises recent studies (after the year 2000) on the yeast volatiles, their spectrum of antifungal activities, and mode of action to minimise the impact of toxigenic fungi and mycotoxins in food.
Table 2. Summary of antagonistic yeast species/strains, their target toxigenic fungi, bioactive volatile molecule(s) and experimental application.
4.1.1. Hanseniaspora spp.
Two species of genus Hanseniaspora, namely H. uvarum and H. opuntiae are known for postharvest protection of food by releasing VOCs effective against toxigenic Aspergillus (Apaliya et al., Citation2018; Galván et al., Citation2022; Gómez-Albarrán et al., Citation2021; Masoud & Kaltoft, Citation2006; Masoud et al., Citation2005; Tejero et al., Citation2021), Penicillium (de Paiva et al., Citation2017) and Fusarium (Hameed et al., Citation2019). The antagonistic volatiles of Hanseniaspora spp., are mainly acids including acetic acid, isobutyric acid, 2-methylbutanoic acid, octanoic acid (Galván et al., Citation2022; Tejero et al., Citation2021) or 2-phenethyl acetate, furfuryl acetate (Galván et al., Citation2022; Masoud et al., Citation2005), isoamyl alcohol, ethyl propionate, isobutyl acetate and ethyl acetate (Masoud & Kaltoft, Citation2006).
4.1.2. Debaryomyces spp.
A Debaryomyces hansenii isolate from marine environment showed several biotechnological benefits (Breuer & Harms, Citation2006; Medina-Córdova et al., Citation2018), including the reduction of toxigenic fungal growth and mycotoxins in different food matrices (Huang et al., Citation2021; Medina-Córdova et al., Citation2016; Núñez et al., Citation2015; Gil-Serna et al., Citation2011; Gil-Serna et al., Citation2009). This yeast is considered as safe (BIOHAZ, Citation2012) by European Food Safety Authority (EFSA) and interacts with the toxigenic fungi using different mechanisms of action including downregulation of the expression of mycotoxin synthesis genes (Gil-Serna et al., Citation2011) and synthesis of antifungal volatiles (Núñez et al., Citation2015). In a study by Medina-Córdova et al. (Citation2016), volatiles of D. hansenii antagonise the growth of Aspergillus sp., F. subglutinans and F. proliferatum at 97–98%. In in vivo testing, the volatiles of this yeast were able to entirely suppress growth on maize grains, showing a partial inhibition of theses fungi and disease appearance. Additionally, there was 60% reduction in FUMs synthesis by F. subglutinans upon exposure to yeast VOCs. In another study, D. hansenii volatiles suppressed toxigenic Penicillium spp. at >60% in co-culture experiments (Núñez et al., Citation2015). The main antifungal volatile molecules released by D. hansenii include 2-methyl-butanol, 2-methyl-propanol, 3-methyl-butanol, (Núñez et al., Citation2015), 2-pentanone, acetic acid, acetone, 2-phenylethanol and 3-methylbutanoic acid, (Huang et al., Citation2021). In the , antifungal activities of Debaryomyces spp., are summarised.
4.1.3. Saccharomyces spp.
Saccharomyces spp. play an important role in food safety particularly in controlling fungi and mycotoxins in food products (Papp et al., Citation2021). Saccharomyces cerevisiae volatiles exert inhibitory effects on growth and on mycotoxin biosynthesis (Nally et al., Citation2015; Oro et al., Citation2018; Ul Hassan et al., Citation2021). The VOCs-mediated antagonistic activity of Saccharomyces cerevisiae is mainly due to ethyl acetate (Oro et al., Citation2018), as reported against A. flavus (Ul Hassan et al., Citation2021), A. caelatus, A. versicolor, A. terreus, A. carbonarius (Nally et al., Citation2015) and Penicillium spp. (Oro et al., Citation2018).
4.1.4. Candida spp.
Candida spp. demonstrated significant inhibition of fungi including A. flavus (Jaibangyang et al., Citation2020; Ul Hassan et al., Citation2021), A. carbonarius (Farbo et al., Citation2018; Tilocca et al., Citation2019; Tilocca et al., Citation2020), A. ochraceus (Farbo et al., Citation2018), A. niger (Ando et al., Citation2012), Penicillium (Arrarte et al., Citation2017; Gomomo et al., Citation2022) and Alternaria spp., (Arrarte et al., Citation2017) as well as their mycotoxin synthesis. The volatiles of Candida pyralidae (Gomomo et al., Citation2022), Candida intermedia (Tilocca et al., Citation2019; Ul Hassan et al., Citation2021), Candida nivariensis (Jaibangyang et al., Citation2020), Candida sake (Arrarte et al., Citation2017), Candida friedrichii (Farbo et al., Citation2018; Fiori et al., Citation2014) and Candida maltosa (Ando et al., Citation2012) inhibited toxigenic fungi. The bioactive volatile molecules produced by different Candida species include isoamyl alcohol, phenethyl alcohol, isoamyl acetate (Ando et al., Citation2012), 2-phenylethanol (Farbo et al., Citation2018) and 1-pentanol (Jaibangyang et al., Citation2020). Candida volatiles inhibit fungal sporulation, mycelial growth and mycotoxin synthesis (Farbo et al., Citation2018; Jaibangyang et al., Citation2020; Ul Hassan et al., Citation2021) by direct cytolytic effects (Fiori et al., Citation2014), downregulating the expression of relevant pathway genes (Farbo et al., Citation2018) or mitochondrial metabolic activity (Tilocca et al., Citation2019).
4.1.5. Pichia/Wickerhamomyces spp.
The biocontrol efficacy of Pichia spp. by releasing inhibitory volatiles play an important role against pre- and post-harvest spoilage fungi (Gomomo et al., Citation2022; Masoud et al., Citation2005). In this regard, Pichia kluyveri (Gomomo et al., Citation2022; Masoud et al., Citation2005), Pichia kudriavzevii, Pichia occidentalis (Choińska et al., Citation2020), Pichia pastoris (Liu et al., Citation2014), P. membranifaciens (Lutz et al., Citation2013), P. anomala (Chang et al., Citation2015; Contarino et al., Citation2019; Hua et al., Citation2014; Masoud et al., Citation2005; Oro et al., Citation2018) are well known for their inhibitory role. In a study on P. anomala strain WRL-076, Hua et al. (Citation2014) reported production of antifungal volatile 2-phenylethanol which resulted in depressed spore germination, suppression of vegetative growth and of AFs synthesis in A. flavus. The inhibition of AFs synthesis was associated with the 10,000-fold downregulation of AFs biosynthetic genes, as well as altered chromatin modifying genes.
4.1.6. Lachancea and Cyberlindnera spp.
There are a couple of studies describing the role of volatiles emitted by Lachancea and Cyberlindnera spp., in the biocontrol of Aspergillus, Penicillium and a few Fusarium fungi. The first ever work was performed by Fiori et al. (Citation2014) on Lachancea thermotolerans 751 and Cyberlindnera jadinii 273 against OTA-producer strains of A. carbonarius. The yeast volatiles significantly inhibited growth on detached grape berries and on synthetic media. Later on, toxigenic species of Aspergillus (Alkuwari et al., Citation2022; Farbo et al., Citation2018; Tilocca et al., Citation2019; Ul Hassan et al., Citation2021; Zeidan et al., Citation2018), Penicillium and Fusarium (Alkuwari et al., Citation2022; Zeidan et al., Citation2018) were exposed to the same yeast volatiles to investigate the mechanism of antagonism (). Zeidan et al. (Citation2018) exposed mycotoxin-producing strains of F. graminearum, P. verrucosum and A. parasiticus to the volatiles emitted by L. thermotolerans and observed up to 48% reduced mycelium growth and much higher (96%) depression in their mycotoxin biosynthesis. In another study, Farbo et al. (Citation2018) reported the precise nature of bioactive compound in the volatile blend of L. thermotolerans and C. jadinii as 2-phenylethanol (2-PE): this compound is involved in the inhibition of vegetative growth, sporulation and downregulation of the key genes (pks, laeA, veA and others) involved in growth and mycotoxin synthesis. Taking forward the findings of Farbo et al. (Citation2018), a step ahead proteomic investigation was carried out by Tilocca et al. (Citation2019) to explore the metabolic profile of A. carbonarius exposed to 2-PE. An array of metabolic alterations was observed in the VOCs – exposed A. carbonarius, including the reduction in protein synthesis, inhibition of fungal growth and defence system. Among the biocontrol benefits, more recently it was demonstrated that fermentation processes involving L. thermotolerans improve the sensory profiles of wines (Hranilovic et al., Citation2022; Citation2021).
4.1.7. Metschnikowia spp.
There is plenty of information about the antifungal efficacy of Metschnikowia spp., cell-free extracts against phytopathogenic and mycotoxin-producing fungi (Settier-Ramírez et al., Citation2021), however only one species, i.e. Metschnikowia pulcherrima is known for its antifungal volatiles (de Paiva et al., Citation2017; Oro et al., Citation2018). In in vitro assays, VOCs released by M. pulcherrima, the major component being ethyl acetate, were able to suppress the growth of A. alternata and A. carbonarius. Likewise, ethyl acetate fumigation completely protected apple fruit from spoilage and fungal infection. In another study, M. pulcherrima L672 volatiles significantly delayed the infection by P. expansum (a patulin producer) on sweet cherries (de Paiva et al., Citation2017).
4.1.8. Cryptoccocus spp.
Cryptoccocus victoriae and Cryptococcus albidus are two important species of the genus Cryptoccocus known for their antifungal volatiles. Apart from fungal spore germination inhibition, Lutz et al. (Citation2013) demonstrated 100 and 62% inhibition of P. expansum growth upon exposure to C. albidus and C. victoriae volatiles, respectively. The precise nature of antifungal volatiles was not studied.
Among yeast species being registered or commercialised as plant protecting agents against different pathogens, S. cerevisiae, M. fructicola, A. pullulans, C. albidus and C. oleophila are preminent in the list of approved yeasts (Freimoser et al., Citation2019). However, biocontrol products based solely on the antifungal volatiles and their contactless application for the preservation of food is still awaited.
4.2. Antagonistic mechanism of yeast volatiles
Pre-harvest application of yeast’s VOC for the control of toxigenic fungi in agricultural settings has received marginal attention. This is because of low molecular weight of VOCs, leading to less chances to concentrate enough in the open environment to interact with fungal communities (Freimoser et al., Citation2019; Papp et al., Citation2021; Tilocca et al., Citation2020). However, their use in post-harvest storage is applicable, where there are enough chances particularly in a closed environment to interact with their target fungal strains and inhibit their growth and/or mycotoxin synthesis (Tilocca et al., Citation2020). There are several mechanisms by which yeast VOCs antagonise the growth and mycotoxins accumulation activities of toxigenic fungi. Among these, down regulation of mycotoxins pathways genes in VOCs exposed fungi is widely accepted (Farbo et al., Citation2018). However, this is not a general mechanism of antagonism involved in all type of fungal communities in any situation. Farbo et al. (Citation2018), in their same study noted no effect in gene expression of A. ochraceus, whereas A. carbonarius was strongly inhibited. Exposure of A. flavus to 2-phenylethanol (a major bioactive molecule in yeast volatilomes) resulted in suppressed AFs synthesis by inhibition of expression of all pathway genes (Chang et al., Citation2015), while at high 2-phenylethanol exposure levels fungal protein and amino acid synthesis was strongly altered (Liu et al., Citation2014). A proteomic investigation on a toxigenic A. carbonarius by Tilocca et al. (Citation2019) revealed that yeast VOCs significantly target protein synthesis, detoxification of toxic metabolites, mitochondrial metabolism and their proliferative activity.
Another direct interaction of the yeast VOCs is the permeability changes in the target fungi membranes, thereby leading to abnormal diffusion of molecules and ions and eventually the death of the affected cells (Ando et al., Citation2012; Di Francesco et al., Citation2015; Simoncini et al., Citation2015). This further suggested that less volatile molecules, staying for a longer time on the membrane of fungi, might have stronger inhibitory potential on fungal cells. Pennerman et al. (Citation2019) studied the effect of a volatile compound (1-octen-3-ol) on two non-toxigenic strains of P. expansum and found that exposed fungi were able to synthesise more patulin as compared to unexposed ones. He correlated this increased production with the upregulation of glucose oxidase.
5. Filamentous fungi
5.1. Role of fungal volatiles in the biocontrol of mycotoxin-producing fungi
The role of filamentous fungal volatiles in the biocontrol of mycotoxin-producing fungi has least been studied and largely limited to the post-harvest decay prevention of fruits and vegetables (Mari et al., Citation2016). VOCs of a few filamentous species from genus Aspergillus, Penicillium, Ceratocystis, Muscodor, Galactomyces, Trichoderma have been tested to be potentially inhibitory towards toxigenic fungi. However, Muscodor albus and non-aflatoxigenic A. flavus have been best characterised so far for their biocontrol volatiles (Mari et al., Citation2016; Moore, Citation2022). Below is a brief account of both of these genera and details are reported in .
Table 3. Biocontrol activities of filamentous fungal species/strains against mycotoxigenic fungi, major bioactive volatile molecule(s) and experimental application.
5.1.1. Muscodor spp.
Muscodor albus was discovered in late 1990s as an excellent bio-preservation candidate for the post-harvest fumigation of fruits and vegetables. The bioactive molecules in the volatiles of M. albus were identified by Ezra and Strobel (Citation2003) as propanoic acid and 2-methyl-ester. These volatiles showed strong antifungal activity against toxigenic and phytopathogenic F. solani, A. ochraceus, and P. expansum. In the following year, Mercier and Jiménez (Citation2004) reported isobutyric acid and 2-methyl-1-butanol as volatiles of M. albus being effective against P. expansum. The exploration of bio-preservative potential of M. albus continued on, and Corcuff et al. (Citation2011) reported that fumigation of potatoes with M. albus volatiles can protect tubers from important pathogens including F. sambucinum. In a more extensive work, Braun et al. (Citation2012) found a strong antifungal activity of M. albus volatiles on a range of toxigenic fungi including A. flavus, A. carbonarius, A. ochraceus, A. niger, F. graminearum and P. verrucosum (). In their study, 2-methyl-butanol and isobutyric acid were reported as major antifungal biomolecules. Meanwhile, other M. albus strains were isolated, but their activity against fungal pathogens was not comparable, nor the nature of their produced volatile molecules showed any similarity with good biocontrol strains. However, in spite of its huge biofumigation potential, M. albus was not able to obtain registration as a commercial bio-preservative product by the US Environment Protection Agency, because of toxicity of one of its metabolites (Mari et al., Citation2016).
5.1.2. Aspergillus spp.
Among all mycotoxins, control of AFs and their producing fungi remained a major focus of the scientific communities for decades (Ji et al., Citation2016; Shabeer et al., Citation2022). This is mainly because of the range of their toxic effects, and established regulation by the food regulatory authorities in most of the developed nations as well as in several developing countries (van Egmond et al., Citation2007). Thus, in many of the published reports, the biocontrol efforts are directed mainly towards A. parasiticus and A. flavus, the two major producers of AFs in food (Moore, Citation2022). In these efforts, the use of non-aflatoxigenic fungal strains (A. flavus) for the control of aflatoxigenic strains showed the most prominent role (Moore et al., Citation2021; Moore, Citation2022). It is advisable to employ the non-toxigenic intra-specific strains against the toxigenic strains to achieve the optimal benefits because these strains normally share the same ecological niche. Non-aflatoxigenic strains are presumably more aggressive colonisers than the toxigenic ones, as it’s generally assumed that mycotoxins synthesis in fungi is inversely associated with mycelial growth (Ehrlich et al., Citation2011), and for a good biocontrol agent good colonising property is a prerequisite, so that it can out-compete toxigenic strains (Moore, Citation2022). According to Horn and Dorner (Citation2009) non-toxigenic fungal strains retain the ability to degrade the products of toxigenic strains or convert and then potentially utilise them for their metabolic processes (Maxwell et al., Citation2021). Application of high dose and annual recommended use of non-aflatoxigenic fungal strains not only protect the subjected crops in pre-harvest, but their biocontrol effects diffuse to the neighbouring agricultural plots (Weaver & Abbas, Citation2019) as well as to the post-harvest storage of grains (Alberts et al., Citation2017; Dorner & Cole, Citation2002).
There is overwhelming evidence on the application of intraspecies fungal volatile in the control of counterpart toxigenic species (Ehrlich, Citation2014; Singh & Lee, Citation2018) that can display a significant activity in the inhibition of toxigenic fungi (). Application of fungal volatiles in biocontrol of mycotoxins and toxigenic fungi has been most successful among all other microbial volatiles.
The story of potentially safe biocontrol of A. flavus and AFs started with in discovery of a non-aflatoxigenic strain of A. flavus (AF36) back in 1980s, which was later on (Ehrlich & Cotty, Citation2004) found to be having a mutation in a key cluster gene pksA (aflC). Meantime, another aggressive coloniser, the non-aflatoxigenic A. flavus NRRL21882, was discovered and used in a biocontrol formulation (Alfa-Guard®) for application on the peanuts field (Dorner & Lamb, Citation2006). The successful field trials of AF36 and NRRL21882 led to their US environmental protection agency (EPA)-approval for pre-harvest use only. Nowadays, two commercial formulations AF36, namely Prevail® (used in western part of USA) and Alfa-Gurad® (licenced by Syngenta) are being used Eastern US and other countries like Turkey (Lavkor et al., Citation2019) and Brazil (Reis et al., Citation2020) for the effective control of A. flavus and AFs. Interestingly, Alfa-Guard® apart from AFs control also protects maize crops from F. verticillioides and fumonisin contamination (Reis et al., Citation2020). The success story of biocontrol strains kept on going with the discovery of K49 (Abbas et al., Citation2006) in U.S.A. and A2085 (active ingredient of AF-X1TM in Italy), MyToolBox Af01 used in maize crops in Serbia. All these strains were either having single gene mutation (K49, AF36), absence of complete AFs genes cluster (A2089, NRRL21882) or missing couple of cluster genes (MyToolBox Af01). The technology of A. flavus biocontrol with the use of non-aflatoxigenic strains is still evolving and a composite formulation of four non-aflatoxigenic strains in each Afla-Safe® (being used in Africa) (Bandyopadhyay et al., Citation2016), and FourSureTM in U.S.A. (Moral et al., Citation2020) are being practiced with high success. However, the mechanism of action of these non-aflatoxigenic A. flavus strains is still not completely understood. Competitive exclusion and competition for nutrients and space are certainly major mechanisms, but these are not sufficient to fully explain the efficacy of biocontrol strains. In recent years, the role of antagonistic volatiles is being explored in their biocontrol mechanism (Moore et al., Citation2021, Citation2022). Based on the volatile profiles of non-aflatoxigenic A. flavus (Lucca et al., Citation2010) in an in vitro environment, Moore et al. (Citation2021) applied 3-octanone, trans-2-methyl-2-butenal, 2,3-dihydrofuran and decane against toxigenic A. flavus strains. All the volatile molecules significantly inhibited AFs and later two molecules (2,3-dihydrofuran and decane) along with AFs synthesis inhibition, also suppress cyclopiazonic acid CPA production (Moore et al., Citation2021). In another study, the same team reported significant reduction in AFs and CPA synthesis in toxigenic A. flavus when exposed to three VOCs of non-toxigenic A. flavus (i.e. 2,3-dihydrofuran, 3-octanone and decane), while there was a minimal effect on fungal growth of toxigenic A. flavus strain. To further investigate the antagonistic mechanism of action of non-aflatoxigenic strains, Moore et al. (Citation2019) hypothesised the possible role of extrolites produced by non-aflatoxigenic A. flavus on the inhibition and AFs synthesis of toxigenic A. flavus. The precise chemical nature of extrolites and their mode of action remain unexplored.
It has been more than a decade (Bandyopadhyay et al., Citation2019) on the successful commercial application of non-aflatoxigenic fungi for the control of toxigenic A. flavus and mycotoxins. However, Moore (Citation2022) presents certain important considerations that must be met to supplement continuous application as well as expand the knowledge to the other toxigenic fungi and their toxins. These considerations are digging information about the: (a) persistence of atoxigenic fungi under climatic stresses and particularly their vulnerability to climate change (if any); (b) genetic stability of non-aflatoxigenic strains, as fungi are existing and evolving since thousands of years, sexual interaction might result in production of other mycotoxins; (c) efforts/managemental practices to supplement the effectivity of atoxigenic fungi; and (d) outlining the precise mode of action of atoxigenic strains.
Apart from the application of non-aflatoxigenic A. flavus, atoxigenic strains of other fungi can be tested for their bio-preservation potential. For instance, Wani et al. (Citation2010) found the production of a volatile 2-PE by a strain of A. niger: this molecule is similar to the one produced by yeasts for the inhibition of toxigenic A. flavus, A. carbonarius, A. ochraceus (Chang et al., Citation2015; Farbo et al., Citation2018; Tilocca et al., Citation2019) and can potentially be used as biocontrol means. The exploration of non-toxigenic fungal strains from genus Aspergillus, Penicillium and Fusarium is continuing, with focus on their potential for commercial application such as aggressiveness, genetic stability, environmental impact and ease and suitability in application. Below is a tabulated summary of such efforts made in the years after 2000.
5.2. Volatilome of filamentous fungi: mode of action against toxigenic fungi
The precise mechanism of action of filamentous fungal volatiles in the control of toxigenic fungi and mycotoxins has not been fully elucidated. However, based on the nature of biocontrol strains and their reported in vitro and in vivo activities, some hypotheses can be inferred. The volatiles of biocontrol strains have shown genetic effects such as altered expression levels in the key genes involved in the mycotoxins synthesis (Moore et al., Citation2021) and those involved in cell membrane aging process and expression of chitin synthase (Yang et al., Citation2021). There are different patterns of genes alteration in the biocontrol strains, spanning from point mutation in a single gene (Ehrlich & Cotty, Citation2004), missing some pathway genes (Yin et al., Citation2008), or complete deletion of mycotoxin pathway gene cluster (Chang et al., Citation2005). Yet, there might be other possibilities, probably at post-transcriptional level, as several non-aflatoxigenic A. flavus strains are known to have intact gene clusters (Criseo et al., Citation2008; Scherm et al., Citation2005). Moreover, aggressive colonisation of the substrate by non-toxigenic strains suggests that competitive exclusion in terms of ‘fight for space and nutrients’ is also operative in these fungi-fungi interactions (Mauro et al., Citation2018; Yan et al., Citation2021).
Filamentous fungi may share with yeast the same antagonistic mechanisms of action because the nature of their biomolecules is not different (Moore et al., Citation2021; Moore et al., Citation2022; Rao et al., Citation2022; Yang et al., Citation2021). Wani et al. (Citation2010) reported the production of 2-PE (2-phenylethanol) by A. niger; whereby 2-PE-producing yeasts are known to affect the cellular metabolic processes and the expression of key mycotoxin synthesis pathway genes in the exposed fungi (Farbo et al., Citation2018; Tilocca et al., Citation2019). Another possible mechanism of action involves DNA-methylation as a result of the exposure to the volatile N-methyl-N-nitroso isobutyramide (MNIBA) released by M. albus (Alpha et al., Citation2015; Hutchings et al., Citation2017; Jimenez et al., Citation2012). The decomposition of MNIBA results in the production of methyl diazohydroxide and isobutyric acid: the first one is converted to methyl diazonium ion, which interacts with the nucleophilic site on DNA and leads to DNA-methylation. Consistently with this pathway, isobutyric acid (a derivative of MNIBA), has been found in the fungal volatiles of previous studies (Braun et al., Citation2012; Corcuff et al., Citation2011; Mercier & Jiménez, Citation2004). This mechanistic pathway is particularly relevant to the M. albus biocontrol activity, as isobutyric acid is not reported in the volatiles of non-aflatoxigenic A. flavus strains (Lucca et al., Citation2010; Moore et al., Citation2021).
Suppression of fungal spore production and germination (Li et al., Citation2015), alteration in plasma membrane permeability and hyphal cell respiration (Medina-Romero et al., Citation2017), accumulation of reactive oxygen species (Yang et al., Citation2021) are other mechanisms of action of fungal volatiles on toxigenic fungi. It is not unreasonable to hypothesise that non-toxigenic biocontrol strains are working in multidimensional ways to limit the growth and mycotoxins synthesis potential of the toxigenic strains. These approaches might include release of antifungal molecules (volatiles and diffusible), competition for the space and nutrients, lytic enzymes, etc.
6. Application of microbial volatiles in different scenarios for the protection of horticultural crops
There are promising evidences of bacterial, yeast and filamentous fungal strains possessing strong antifungal potentialities to limit the mycotoxins in food (Dopazo et al., Citation2022; Gallo et al., Citation2022; Medina-Romero et al., Citation2017; Moore et al., Citation2022, Citation2021; Saleh et al., Citation2021; Wang et al., Citation2021; Yang et al., Citation2021). A handful number of these strains are proven under in vitro and in vivo environment to retain antagonistic activities in limiting key toxigenic fungal species (Alasmar et al., Citation2021; Farbo et al., Citation2018; Galván et al., Citation2022; Gomomo et al., Citation2022; Moore, Citation2022). Fewer of these, however, were successfully tested in fructo/in planta (Bandyopadhyay et al., Citation2019; Gallo et al., Citation2022; Moore, Citation2022). Microbial volatile molecules can easily diffuse in the stored food commodities making it possible to ensure maximum protection by reaching every empty space and surface, at least during post-harvest storage and transportation (Lammers et al., Citation2022; Passone & Etcheverry, Citation2014). In most of the post-harvest closed environment studies, even smaller volumes were able to produce long-standing protection with an increasing shelf life of the biofumigated commodities (Aunsbjerg et al., Citation2015). The results obtained with low volumes of volatile molecules also present an economical and environmental advantage compared to their chemical fungicide counterpart.
Another approach could be incorporating VOCs in edible films, which are being used as smart food packaging material (Okcu et al., Citation2018; Sultan et al., Citation2021). These films can be used as a routine coating material for hard-textured fruits like avocado, mango and orange. These films may retain the molecules on the fruit for extended duration of time, ensuring protection against microbial spoilage during long-distance transportation and storage. However, this approach wouldn’t be viable for highly perishable fruits like strawberry, table grape, peach, as these are much prone to handling damages. In such cases, these fruits can be preserved by treating sterile gauze with antimicrobial VOCs and distributing them within the fruit packages this approach has been proven very effective for 56 days at 1°C (Valero et al., Citation2006; Valverde et al., Citation2005). Incorporating VOCs on specialised slow release material such as polyethylene terephthalate (PET), and packing in sachets or pouches to be inserted in the food storage boxes can effectively reduce the food spoilage during the transport and storage chain (Almenar et al., Citation2007).
The first successful biocontrol effort emerged with the commercialisation of Aflaguard® to limit the spread of toxigenic A. flavus and AFs in the peanut fields in U.S.A. (Dorner & Lamb, Citation2006; Moore et al., Citation2021). Alfagurad®, a formulation of non-toxigenic A. flavus coated on roasted sorghum seeds, is applied during the sowing of crops (Moore, Citation2022; Moore et al., Citation2022). Atoxigenic A. flavus mainly produce trans-2-methyl-2-butenal, 2,3-dihydrofuran and decane to control the growth of toxigenic A. flavus and/or AFs synthesis (Moore et al., Citation2021). An observation based on 10-years application of this product showed 70–90% reduction in AFB1 in the treated commodities (Bandyopadhyay et al., Citation2019; Moore, Citation2022; Moore et al., Citation2019). Despite this milestone success, antifungal products based on yeast and bacterial volatilome have not been achieved yet. In any case, before adopting such approaches it is absolutely necessary to correlate laboratory findings with commercial context in terms of physicochemical appearance, sensorial evaluation and quality standards of the product.
7. Concerns and limitations associated with the application of VOC
Application of microbial volatiles for the control of mycotoxigenic fungi and mycotoxins in food shows a significant potential to replace the synthetic fungicides, thereby avoiding associated environmental and health effects. Additionally, it adds significant economic benefits to the food and agriculture industry by controlling pre- and post-harvest losses at low cost. However, with increasing efforts in developing and application of VOC in commercial settings, some concerns and limitations were outlined by the scientific community as well as by consumers, namely: (a) chances of interaction of VOCs with food molecules, leading to the production of toxic products that might raise the regulatory issue; (b) VOCs might behave differentially in different food material, possibly inhibiting their diffusion and effectivity; (c) range, effectiveness, spectrum and safety of the VOC, as there is a common concern about microbial volatiles being not as effective as synthetic fungicides, particularly in the open environment and against a diversified range of spoilage microbes. In these scenarios, it is imperative to assess the effect of microbial volatiles in a real food protection system: the appropriate concentration of VOCs for optimal protection needs to be explored for each different food commodity under variable environmental conditions; (d) safety associated with fumigation of microbial volatile is another important consideration that can be enhanced with the application of proper concentration of the fumigant; (e) the registration process, as with any new products, registration process for the commercial application is not easy nor fast, (f) genetic stability of non-toxic microbes: this concern is particularly relevant to the use to non-toxigenic fungal strains for the control of toxigenic ones. There are chances that genetic makeup of the biocontrol strains (atoxigenic) will change with time, leading to emergence of super-toxigenic strains.
8. Conclusions and perspectives
The antifungal spectrum of microbial volatiles is generally wider because of rapid conversion to gaseous state in water and air diffusion (Lammers et al., Citation2022; Passone & Etcheverry, Citation2014), and needs no physical contact with the treated commodity, hence organoleptic qualities of the food matrices are preserved (Valero et al., Citation2006; Valverde et al., Citation2005). Other restrictions posed by the chemical and physical methods of fungal control such as high cost of production, heavy equipment, denaturation of nutrition value and energy consumptions are overcome by microbial methods (Smaoui et al., Citation2022). All in all, it is not unreasonable to foresee a big scope and future of microbial VOC in the food and agriculture industry for the biological control of mycotoxins.
Acknowledgements
Open Access funding provided by the Qatar National Library. The statements here are solely the responsibility of authors.
Disclosure statement
No potential conflict of interest was reported by the authors.
Correction Statement
This article has been corrected with minor changes. These changes do not impact the academic content of the article.
References
- Abbas, H. K., Zablotowicz, R. M., Bruns, H. A., & Abel, C. A. (2006). Biocontrol of aflatoxin in corn by inoculation with non-aflatoxigenic Aspergillus flavus isolates. Biocontrol Science and Technology, 16(5), 437–449. https://doi.org/10.1080/09583150500532477
- Agirman, B., & Erten, H. (2020). Biocontrol ability and action mechanisms of Aureobasidium pullulans GE17 and Meyerozyma guilliermondii KL3 against Penicillium digitatum DSM2750 and Penicillium expansum DSM62841 causing postharvest diseases. Yeast, 37(9–10), 437–448. https://doi.org/10.1002/yea.3501
- Alasmar, R., Ul-Hassan, Z., Zeidan, R., Al-Thani, R., Al-Shamary, N., Alnaimi, H., Migheli, Q., & Jaoua, S. (2021). Isolation of a novel Kluyveromyces marxianus strain QKM-4 and evidence of its volatilome production and binding potentialities in the biocontrol of toxigenic fungi and their mycotoxins. ACS Omega, 5(28), 17637–17645. https://doi.org/10.1021/acsomega.0c02124
- Al Attiya, W., Hassan, Z. U., Al-Thani, R., & Jaoua, S. (2021). Prevalence of toxigenic fungi and mycotoxins in Arabic coffee (Coffea arabica): Protective role of traditional coffee roasting, brewing and bacterial volatiles. PLOS ONE, 16(10), e0259302. https://doi.org/10.1371/journal.pone.0259302
- Alberts, J. F., Lilly, M., Rheeder, J. P., Burger, H.-M., Shephard, G. S., & Gelderblom, W. C. A. (2017). Technological and community-based methods to reduce mycotoxin exposure. Food Control, 73, 101–109. https://doi.org/10.1016/j.foodcont.2016.05.029
- Alina, M. R. (2022). Implications of mycotoxins in food safety. In R. A. Marc (Ed.), Mycotoxins and food safety – recent advances. IntechOpen. https://doi.org/10.5772/intechopen.102495
- Alkuwari, A., Hassan, Z. U., Zeidan, R., Al-Thani, R., & Jaoua, S. (2022). Occurrence of mycotoxins and toxigenic fungi in cereals and application of yeast volatiles for their biological control. Toxins, 14(6), 404. https://doi.org/10.3390/toxins14060404
- Almeida, F., Rodrigues, M. L., & Coelho, C. (2019). The still underestimated problem of fungal diseases worldwide. Frontiers in Microbiology, 10, 214. https://doi.org/10.3389/fmicb.2019.00214
- Almenar, E., Auras, R., Rubino, M., & Harte, B. (2007). A new technique to prevent the main post harvest diseases in berries during storage: Inclusion complexes β-cyclodextrin-hexanal. International Journal of Food Microbiology, 118(2), 164–172. https://doi.org/10.1016/j.ijfoodmicro.2007.07.002
- Alpha, C. J., Campos, M., Jacobs-Wagner, C., & Strobel, S. A. (2015). Mycofumigation by the volatile organic compound-producing fungus Muscodor albus induces bacterial cell death through DNA damage. Applied and Environmental Microbiology, 81(3), 1147–1156. https://doi.org/10.1128/AEM.03294-14
- Alshannaq, A., & Yu, J. H. (2017). Occurrence, toxicity, and analysis of major mycotoxins in food. International Journal of Environmental Research and Public Health, 14(6), 632. https://doi.org/10.3390/ijerph14060632
- Ando, H., Hatanaka, K., Ohata, I., Yamashita-Kitaguchi, Y., Kurata, A., & Kishimoto, N. (2012). Antifungal activities of volatile substances generated by yeast isolated from Iranian commercial cheese. Food Control, 26(2), 472–478. https://doi.org/10.1016/j.foodcont.2012.02.017
- Apaliya, M. T., Zhang, H., Zheng, X., Yang, Q., Mahunu, G. K., & Kwaw, E. (2018). Exogenous trehalose enhanced the biocontrol efficacy of Hanseniaspora uvarum against grape berry rots caused by Aspergillus tubingensis and Penicillium commune. Journal of the Science of Food and Agriculture, 98(12), 4665–4672. https://doi.org/10.1002/jsfa.8998
- Arrarte, E., Garmendia, G., Rossini, C., Wisniewski, M., & Vero, S. (2017). Volatile organic compounds produced by Antarctic strains of Candida sake play a role in the control of postharvest pathogens of apples. Biological Control, 109, 14–20. https://doi.org/10.1016/j.biocontrol.2017.03.002
- Arrebola, E., Sivakumar, D., & Korsten, L. (2010). Effect of volatile compounds produced by Bacillus strains on postharvest decay in citrus. Biological Control, 53(1), 122–128. https://doi.org/10.1016/j.biocontrol.2009.11.010
- Aunsbjerg, S. D., Honoré, A. H., Marcussen, J., Ebrahimi, P., Vogensen, F. K., Benfeldt, C., Skov, T., & Knøchel, S. (2015). Contribution of volatiles to the antifungal effect of Lactobacillus paracasei in defined medium and yogurt. International Journal of Food Microbiology, 194, 46–53. https://doi.org/10.1016/j.ijfoodmicro.2014.11.007
- Bandyopadhyay, R., Atehnkeng, J., Ortega-Beltran, A., Akande, A., Falade, T. D. O., & Cotty, P. J. (2019). ‘Ground-Truthing’ Efficacy of biological control for aflatoxin mitigation in farmers’ fields in Nigeria: From field trials to commercial usage, a 10-year study. Frontiers in Microbiology, 10, 2528. https://doi.org/10.3389/fmicb.2019.02528
- Bandyopadhyay, R., Ortega-Beltran, A., Akande, A., Mutegi, C., Atehnkeng, J., Kaptoge, L., Senghor, A. L., Adhikari, B. N., & Cotty, P. J. (2016). Biological control of aflatoxins in Africa: Current status and potential challenges in the face of climate change. World Mycotoxin Journal, 9(5), 771–789. https://doi.org/10.3920/WMJ2016.2130
- BIOHAZ (EFSA Panel on Biological Hazards). (2012). Scientific opinion on the maintenance of the list of QPS biological agents intentionally added to food and feed (2012 update). EFSA J., 10(12), 3020. https://doi.org/10.2903/j.efsa.2012.302
- Black, B. A., Zannini, E., Curtis, J. M., & Gänzle, M. G. (2013). Antifungal hydroxy fatty acids produced during sourdough fermentation: Microbial and enzymatic pathways, and antifungal activity in bread. Applied and Environmental Microbiology, 79(6), 1866–1873. https://doi.org/10.1128/AEM.03784-12
- Bluma, R. V., & Etcheverry, M. G. (2006). Influence of Bacillus spp. isolated from maize agroecosystem on growth and aflatoxin B1 production by Aspergillus section Flavi. Pest Management Science, 62(3), 242–251. https://doi.org/10.1002/ps.1154
- Boukaew, S., & Prasertsan, P. (2020). Efficacy of volatile compounds from Streptomyces philanthi RL-1-178 as a biofumigant for controlling growth and aflatoxin production of the two aflatoxin-producing fungi on stored soybean seeds. Journal of Applied Microbiology, 129(3), 652–664. https://doi.org/10.1111/jam.14643
- Braun, G., Vailati, M., Prange, R., & Bevis, E. (2012). Muscodor albus volatiles control toxigenic fungi under controlled atmosphere (CA) storage conditions. International Journal of Molecular Sciences, 13(12), 15848–15858. https://doi.org/10.3390/ijms131215848
- Breuer, U., & Harms, H. (2006). Debaryomyces hansenii – An extremophilic yeast with biotechnological potential. Yeast (Chichester, England), 23(6), 415–437. https://doi.org/10.1002/yea.1374
- Broberg, A., Jacobsson, K., Ström, K., & Schnürer, J. (2007). Metabolite profiles of lactic acid bacteria in grass silage. Applied and Environmental Microbiology, 73(17), 5547–5552. https://doi.org/10.1128/AEM.02939-06
- Brock, N. L., Menke, M., Klapschinski, T. A., & Dickschat, J. S. (2014). Marine bacteria from the Roseobacter clade produce sulfur volatiles via amino acid and dimethylsulfoniopropionate catabolism. Organic & Biomolecular Chemistry, 12(25), 4318. https://doi.org/10.1039/c4ob00719k
- Bubici, G. (2018). Streptomyces spp. As biocontrol agents against fusarium species. CAB Reviews: Perspectives in Agriculture, Veterinary Science, Nutrition and Natural Resources, 13(050), https://doi.org/10.1079/PAVSNNR201813050
- Cai, S. T., Chiu, M. C., & Chou, J. Y. (2021). Broad-spectrum activity of volatile organic compounds from three yeast-like fungi of the Galactomyces genus against diverse plant pathogens. Mycobiology, 49(1), 69–77. https://doi.org/10.1080/12298093.2020.1857042
- Calvo, H., Mendiara, I., Arias, E., Gracia, A. P., Blanco, D., & Venturini, M. E. (2020). Antifungal activity of the volatile organic compounds produced by Bacillus velezensis strains against postharvest fungal pathogens. Postharvest Biology and Technology, 166, 111208. https://doi.org/10.1016/j.postharvbio.2020.111208
- Chalivendra, S., DeRobertis, C., Reyes Pineda, J., Ham, J., & Damann, K. (2018). Rice phyllosphere Bacillus species and their secreted metabolites suppress Aspergillus flavus growth and aflatoxin production in vitro and in maize seeds. Toxins, 10(4), 159. https://doi.org/10.3390/toxins10040159
- Chalivendra, S., & Ham, J. H. (2019). Bacilli in the biocontrol of mycotoxins. In M. T. Islam, M. M. Rahman, P. Pandey, M. H. Boehme, & G. Haesaert (Eds.), Bacilli and agrobiotechnology: Phytostimulation and biocontrol (pp. 49–62). Springer International. https://doi.org/10.1007/978-3-030-15175-1_3
- Chang, P.-K., Horn, B. W., & Dorner, J. W. (2005). Sequence breakpoints in the aflatoxin biosynthesis gene cluster and flanking regions in nonaflatoxigenic Aspergillus flavus isolates. Fungal Genetics and Biology, 42(11), 914–923. https://doi.org/10.1016/j.fgb.2005.07.004
- Chang, P.-K., Hua, S., Sarreal, S., & Li, R. (2015). Suppression of aflatoxin biosynthesis in Aspergillus flavus by 2-Phenylethanol is associated with stimulated growth and decreased degradation of branched-chain amino acids. Toxins, 7(10), 3887–3902. https://doi.org/10.3390/toxins7103887
- Chaves-López, C., Serio, A., Gianotti, A., Sacchetti, G., Ndagijimana, M., Ciccarone, C., Stellarini, A., Corsetti, A., & Paparella, A. (2015). Diversity of food-borne Bacillus volatile compounds and influence on fungal growth. Journal of Applied Microbiology, 119(2), 487–499. https://doi.org/10.1111/jam.12847
- Chen, J. H., Xiang, W., Cao, K. X., Lu, X., Yao, S. C., Hung, D., Huang, R. S., & Li, L. B. (2020). Characterization of volatile organic compounds emitted from endophytic Burkholderia cenocepacia ETR-B22 by SPME-GC-MS and their inhibitory activity against various plant fungal pathogens. Molecules, 25(17), 3765. https://doi.org/10.3390/molecules25173765
- Cho, G., Kim, J., Park, C. G., Nislow, C., Weller, D. M., & Kwak, Y. S. (2017). Caryolan-1-ol, an antifungal volatile produced by Streptomyces spp., inhibits the endomembrane system of fungi. Open Biology, 7(7), 170075. https://doi.org/10.1098/rsob.170075
- Choińska, R., Piasecka-Jóźwiak, K., Chabłowska, B., Dumka, J., & Łukaszewicz, A. (2020). Biocontrol ability and volatile organic compounds production as a putative mode of action of yeast strains isolated from organic grapes and rye grains. Antonie van Leeuwenhoek, 113(8), 1135–1146. https://doi.org/10.1007/s10482-020-01420-7
- Cimbalo, A., Alonso-Garrido, M., Font, G., & Manyes, L. (2020). Toxicity of mycotoxins in vivo on vertebrate organisms: A review. Food and Chemical Toxicology, 137, 111161. https://doi.org/10.1016/j.fct.2020.111161
- Contarino, R., Brighina, S., Fallico, B., Cirvilleri, G., Parafati, L., & Restuccia, C. (2019). Volatile organic compounds (VOCs) produced by biocontrol yeasts. Food Microbiology, 82, 70–74. https://doi.org/10.1016/j.fm.2019.01.008
- Corcuff, R., Mercier, J., Tweddell, R., & Arul, J. (2011). Effect of water activity on the production of volatile organic compounds by Muscodor albus and their effect on three pathogens in stored potato. Fungal Biology, 115(3), 220–227. https://doi.org/10.1016/j.funbio.2010.12.005
- Cordero, P., Príncipe, A., Jofré, E., Mori, G., & Fischer, S. (2014). Inhibition of the phytopathogenic fungus Fusarium proliferatum by volatile compounds produced by Pseudomonas. Archives of Microbiology, 196(11), 803–809. https://doi.org/10.1007/s00203-014-1019-6
- Corral, D. A. P., Paz, J., de, J. O., Orozco, G. I. O., Muñiz, C. H. A., Marina, MÁS, Cisneros, M. F. R., Corral, F. J. M., Pavía, S. P. F., & Velasco, C. R. (2020). Antagonistic effect of volatile and non-volatile compounds from Streptomyces strains on cultures of several phytopathogenic fungi. Emirates Journal of Food and Agriculture, 879, https://doi.org/10.9755/ejfa.2020.v32.i12.2222
- Cray, J. A., Connor, M. C., Stevenson, A., Houghton, J. D. R., Rangel, D. E. N., Cooke, L. R., & Hallsworth, J. E. (2016). Biocontrol agents promote growth of potato pathogens, depending on environmental conditions. Microbial Biotechnology, 9(3), 330–354. https://doi.org/10.1111/1751-7915.12349
- Criseo, G., Racco, C., & Romeo, O. (2008). High genetic variability in non-aflatoxigenic A. flavus strains by using Quadruplex PCR-based assay. International Journal of Food Microbiology, 125(3), 341–343. https://doi.org/10.1016/j.ijfoodmicro.2008.04.020
- Crowley, S., Mahony, J., & van Sinderen, D. (2013). Current perspectives on antifungal lactic acid bacteria as natural bio-preservatives. Trends in Food Science & Technology, 33(2), 93–109. https://doi.org/10.1016/j.tifs.2013.07.004
- DeFilippi, S., Groulx, E., Megalla, M., Mohamed, R., & Avis, T. J. (2018). Fungal competitors affect production of antimicrobial lipopeptides in Bacillus subtilis Strain B9–5. Journal of Chemical Ecology, 44(4), 374–383. https://doi.org/10.1007/s10886-018-0938-0
- de Paiva, E., Serradilla, M. J., Ruiz-Moyano, S., Córdoba, M. G., Villalobos, M. C., Casquete, R., & Hernández, A. (2017). Combined effect of antagonistic yeast and modified atmosphere to control Penicillium expansum infection in sweet cherries cv. Ambrunés. International Journal of Food Microbiology, 241, 276–282. https://doi.org/10.1016/j.ijfoodmicro.2016.10.033
- Dey, D. K., Kang, J. I., Bajpai, V. K., Kim, K., Lee, H., Sonwal, S., Simal-Gandara, J., Xiao, J., Ali, S., Huh, Y. S., Han, Y.-K., & Shukla, S. (2022). Mycotoxins in food and feed: Toxicity, preventive challenges, and advanced detection techniques for associated diseases. Critical Reviews in Food Science and Nutrition, 1–22. https://doi.org/10.1080/10408398.2022.2059650
- Diaz, D. G., Pizzolitto, R. P., Vázquez, C., Usseglio, V. L., Zunino, M. P., Dambolena, J. S., Zygadlo, J. A., & Merlo, C. (2021). Effects of the volatile organic compounds produced by Enterococcus spp. Strains isolated from maize grain silos on Fusarium verticillioides growth and fumonisin B1 production. Journal of Stored Products Research, 93, 101825. https://doi.org/10.1016/j.jspr.2021.101825
- Di Francesco, A., Ugolini, L., Lazzeri, L., & Mari, M. (2015). Production of volatile organic compounds by Aureobasidium pullulans as a potential mechanism of action against postharvest fruit pathogens. Biological Control, 81, 8–14. https://doi.org/10.1016/j.biocontrol.2014.10.004
- Dopazo, V., Luz, C., Quiles, J. M., Calpe, J., Romano, R., Mañes, J., & Meca, G. (2022). Potential application of lactic acid bacteria in the biopreservation of red grape from mycotoxigenic fungi. Journal of the Science of Food and Agriculture, 102(3), 898–907. https://doi.org/10.1002/jsfa.11422
- Dorner, J. W., & Cole, R. J. (2002). Effect of application of nontoxigenic strains of Aspergillus flavus and A. parasiticus on subsequent aflatoxin contamination of peanuts in storage. Journal of Stored Products Research, 38(4), 329–339. https://doi.org/10.1016/S0022-474X(01)00035-2
- Dorner, J. W., & Lamb, M. C. (2006). Development and commercial use of afla-Guard®, an aflatoxin biocontrol agent. Mycotoxin Research, 22(1), 33–38. https://doi.org/10.1007/BF02954555
- Ebert, B., Halbfeld, C., & Blank, L. M. (2017). Exploration and exploitation of the yeast volatilome. Current Metabolomics, 5(2), https://doi.org/10.2174/2213235X04666160818151119
- Effmert, U., Kalderás, J., Warnke, R., & Piechulla, B. (2012). Volatile mediated interactions between bacteria and fungi in the soil. Journal of Chemical Ecology, 38(6), 665–703. https://doi.org/10.1007/s10886-012-0135-5
- Ehrlich, K. C. (2014). Non-aflatoxigenic Aspergillus flavus to prevent aflatoxin contamination in crops: Advantages and limitations. Frontiers in Microbiology, 5. https://doi.org/10.3389/fmicb.2014.00050
- Ehrlich, K. C., & Cotty, P. J. (2004). An isolate of Aspergillus flavus used to reduce aflatoxin contamination in cottonseed has a defective polyketide synthase gene. Applied Microbiology and Biotechnology, 65(4), 473–478. https://doi.org/10.1007/s00253-004-1670-y
- Ehrlich, K. C., Wei, Q., Brown, R. L., & Bhatnagar, D. (2011). Inverse correlation of ability to produce aflatoxin and Aspergillus colonization of maize seed. Food and Nutrition Sciences, 02(05), 486–489. https://doi.org/10.4236/fns.2011.25070
- Elshafie, H., Camele, I., Racioppi, R., Scrano, L., Iacobellis, N., & Bufo, S. (2012). In vitro antifungal activity of Burkholderia gladioli pv. Agaricicola against some phytopathogenic fungi. International Journal of Molecular Sciences, 13(12), 16291–16302. https://doi.org/10.3390/ijms131216291
- Ezra, D., & Strobel, G. A. (2003). Effect of substrate on the bioactivity of volatile antimicrobials produced by Muscodor albus. Plant Science, 165(6), 1229–1238. https://doi.org/10.1016/S0168-9452(03)00330-3
- Farbo, M. G., Urgeghe, P. P., Fiori, S., Marcello, A., Oggiano, S., Balmas, V., Hassan, Z. U., Jaoua, S., & Migheli, Q. (2018). Effect of yeast volatile organic compounds on ochratoxin A-producing Aspergillus carbonarius and A. ochraceus. International Journal of Food Microbiology, 284, 1–10. https://doi.org/10.1016/j.ijfoodmicro.2018.06.023
- Fiori, S., Urgeghe, P. P., Hammami, W., Razzu, S., Jaoua, S., & Migheli, Q. (2014). Biocontrol activity of four non- and low-fermenting yeast strains against Aspergillus carbonarius and their ability to remove ochratoxin A from grape juice. International Journal of Food Microbiology, 189, 45–50. https://doi.org/10.1016/j.ijfoodmicro.2014.07.020
- Fisher, M. C., Alastruey-Izquierdo, A., Berman, J., Bicanic, T., Bignell, E. M., Bowyer, P., Bromley, M., Brüggemann, R., Garber, G., Cornely, O. A., Gurr, S. J., Harrison, T. S., Kuijper, E., Rhodes, J., Sheppard, D. C., Warris, A., White, P. L., Xu, J., Zwaan, B., & Verweij, P. E. (2022). Tackling the emerging threat of antifungal resistance to human health. Nature Reviews Microbiology, https://doi.org/10.1038/s41579-022-00720-1
- Freimoser, F. M., Rueda-Mejia, M. P., Tilocca, B., & Migheli, Q. (2019). Biocontrol yeasts: Mechanisms and applications. World Journal of Microbiology and Biotechnology, 35(10), 154. https://doi.org/10.1007/s11274-019-2728-4
- Gallo, A., Fancello, F., Ghilardelli, F., Zara, S., Froldi, F., & Spanghero, M. (2021). Effects of several lactic acid bacteria inoculants on fermentation and mycotoxins in corn silage. Animal Feed Science and Technology, 277, 114962. https://doi.org/10.1016/j.anifeedsci.2021.114962
- Gallo, A., Fancello, F., Ghilardelli, F., Zara, S., & Spanghero, M. (2022). Effects of several commercial or pure lactic acid bacteria inoculants on fermentation and mycotoxin levels in high-moisture corn silage. Animal Feed Science and Technology, 286, 115256. https://doi.org/10.1016/j.anifeedsci.2022.115256
- Galván, A. I., Hernández, A., Córdoba, M., de, G., Martín, A., Serradilla, M. J., López-Corrales, M., & Rodríguez, A. (2022). Control of toxigenic Aspergillus spp. In dried figs by volatile organic compounds (VOCs) from antagonistic yeasts. International Journal of Food Microbiology, 376, 109772. https://doi.org/10.1016/j.ijfoodmicro.2022.109772
- Gil-Serna, J., Patiño, B., Cortés, L., González-Jaén, M. T., & Vázquez, C. (2011). Mechanisms involved in reduction of ochratoxin A produced by Aspergillus westerdijkiae using Debaryomyces hansenii CYC 1244. International Journal of Food Microbiology, 151(1), 113–118. https://doi.org/10.1016/j.ijfoodmicro.2011.08.012
- Gil-Serna, J., Patiño, B., González-Jaén, M. T., & Vázquez, C. (2009). Biocontrol of Aspergillus ochraceus by yeast. In A. Mendez-Vilas (Ed.), Current research topics in applied microbiology and microbial biotechnology. World Scientific.
- Gómez-Albarrán, C., Melguizo, C., Patiño, B., Vázquez, C., & Gil-Serna, J. (2021). Diversity of mycobiota in Spanish grape berries and selection of Hanseniaspora uvarum U1 to prevent mycotoxin contamination. Toxins, 13(9), 649. https://doi.org/10.3390/toxins13090649
- Gomomo, Z., Fanadzo, M., Mewa-Ngongang, M., Hoff, J., Van der Rijst, M., Okudoh, V., Kriel, J., & du Plessis, H. (2022). Control of mould spoilage on apples using yeasts as biological control agents. Polish Journal of Food and Nutrition Sciences, 119–128. https://doi.org/10.31883/pjfns/147913
- Gong, A. D., Dong, F. Y., Hu, M. J., Kong, X. W., Wei, F. F., Gong, S. J., Zhang, Y. M., Zhang, J. B., Wu, A. B., & Liao, Y. C. (2019). Antifungal activity of volatile emitted from Enterobacter asburiae Vt-7 against Aspergillus flavus and aflatoxins in peanuts during storage. Food Control, 106, 106718. https://doi.org/10.1016/j.foodcont.2019.106718
- Groenhagen, U., Baumgartner, R., Bailly, A., Gardiner, A., Eberl, L., Schulz, S., & Weisskopf, L. (2013). Production of bioactive volatiles by different Burkholderia ambifaria strains. Journal of Chemical Ecology, 39(7), 892–906. https://doi.org/10.1007/s10886-013-0315-y
- Hameed, A. R., Al-Qaysi, S. A. S., & Hameed, S. T. (2019). Killer activity of Hanseniaspora uvarum isolated from dates vinegar: Partially purification and characterization of killer toxin. Baghdad Science Journal, 16(1(Suppl.)), 0140. https://doi.org/10.21123/bsj.2019.16.1(Suppl.).0140
- Hassan, Z. U., Al Thani, R., Balmas, V., Migheli, Q., & Jaoua, S. (2019). Prevalence of Fusarium fungi and their toxins in marketed feed. Food Control, 104, 224–230. https://doi.org/10.1016/j.foodcont.2019.04.045
- Hassan, Z. U., Al-Thani, R. F., Migheli, Q., & Jaoua, S. (2018). Detection of toxigenic mycobiota and mycotoxins in cereal feed market. Food Control, 84, 389–394. https://doi.org/10.1016/j.foodcont.2017.08.032
- He, C. N., Ye, W. Q., Zhu, Y. Y., & Zhou, W. W. (2020). Antifungal activity of volatile organic compounds produced by Bacillus methylotrophicus and Bacillus thuringiensis against five common spoilage fungi on loquats. Molecules, 25(15), 3360. https://doi.org/10.3390/molecules25153360
- Horn, B. W., & Dorner, J. W. (2009). Effect of nontoxigenic Aspergillus flavus and A. parasiticus on aflatoxin contamination of wounded peanut seeds inoculated with agricultural soil containing natural fungal populations. Biocontrol Science and Technology, 19(3), 249–262. https://doi.org/10.1080/09583150802696541
- Hranilovic, A., Albertin, W., Capone, D. L., Gallo, A., Grbin, P. R., Danner, L., Bastian, S. E. P., Masneuf-Pomarede, I., Coulon, J., Bely, M., & Jiranek, V. (2021). Impact of Lachancea thermotolerans on chemical composition and sensory profiles of Merlot wines. Food Chemistry, 349, 129015. https://doi.org/10.1016/j.foodchem.2021.129015
- Hranilovic, A., Albertin, W., Capone, D. L., Gallo, A., Grbin, P. R., Danner, L., Bastian, S. E. P., Masneuf-Pomarede, I., Coulon, J., Bely, M., & Jiranek, V. (2022). Impact of Lachancea thermotolerans on chemical composition and sensory profiles of Viognier wines. Journal of Fungi, 8(5), 474. https://doi.org/10.3390/jof8050474
- Hua, S. S. T., Beck, J. J., Sarreal, S. B. L., & Gee, W. (2014). The major volatile compound 2-phenylethanol from the biocontrol yeast, Pichia anomala, inhibits growth and expression of aflatoxin biosynthetic genes of Aspergillus flavus. Mycotoxin Research, 30(2), 71–78. https://doi.org/10.1007/s12550-014-0189-z
- Huang, C., Zhang, L., Johansen, P. G., Petersen, M. A., Arneborg, N., & Jespersen, L. (2021). Debaryomyces hansenii strains isolated from Danish cheese brines act as biocontrol agents to inhibit germination and growth of contaminating molds. Frontiers in Microbiology, 12, 662785. https://doi.org/10.3389/fmicb.2021.662785
- Hutchings, M. L., Alpha-Cobb, C. J., Hiller, D. A., Berro, J., & Strobel, S. A. (2017). Mycofumigation through production of the volatile DNA-methylating agent N-methyl-N-nitrosoisobutyramide by fungi in the genus Muscodor. Journal of Biological Chemistry, 292(18), 7358–7371. https://doi.org/10.1074/jbc.M117.779009
- Insam, H., & Seewald, M. S. A. (2010). Volatile organic compounds (VOCs) in soils. Biology and Fertility of Soils, 46(3), 199–213. https://doi.org/10.1007/s00374-010-0442-3
- Jaibangyang, S., Nasanit, R., & Limtong, S. (2020). Biological control of aflatoxin-producing Aspergillus flavus by volatile organic compound-producing antagonistic yeasts. BioControl, 65(3), 377–386. https://doi.org/10.1007/s10526-020-09996-9
- Ji, C., Fan, Y., & Zhao, L. (2016). Review on biological degradation of mycotoxins. Animal Nutrition, 2(3), 127–133. https://doi.org/10.1016/j.aninu.2016.07.003
- Jimenez, J. I., Margolis, J. S., Baird, J. K., & Lego, S. F. (2012). Compounds derived from Muscodor fungi. U. S. Patent, 20120058058 A1.
- Kagot, V., Okoth, S., De Boevre, M., & De Saeger, S. (2019). Biocontrol of Aspergillus and Fusarium mycotoxins in Africa: Benefits and limitations. Toxins, 11(2), 109. https://doi.org/10.3390/toxins11020109
- Kolosova, A., & Stroka, J. (2012). Evaluation of the effect of mycotoxin binders in animal feed on the analytical performance of standardised methods for the determination of mycotoxins in feed. Food Additives & Contaminants: Part A, 29(12), 1959–1971. https://doi.org/10.1080/19440049.2012.720035
- Lal, M., Kumar, A., Chaudhary, S., Singh, R. K., Sharma, S., & Kumar, M. (2022). Antagonistic and growth enhancement activities of native Pseudomonas spp. against soil and tuber-borne diseases of potato (Solanum tuberosum L.). Egyptian Journal of Biological Pest Control, 32(1), 22. https://doi.org/10.1186/s41938-022-00522-w
- Lammers, A., Lalk, M., & Garbeva, P. (2022). Air ambulance: Antimicrobial power of bacterial volatiles. Antibiotics, 11(1), 109. https://doi.org/10.3390/antibiotics11010109
- Lavkor, I., Arioglu, H., Var, I., & Oztemiz, S. (2019). Biological control of aflatoxigenic fungi on peanuts: For the pre-harvest approach. Turkish Journal of Field Crops, 24(1), 21–27. https://doi.org/10.17557/tjfc.562634
- Lee, S. O., Kim, H. Y., Choi, G. J., Lee, H. B., Jang, K. S., Choi, Y. H., & Kim, J. C. (2009). Mycofumigation with Oxyporus latemarginatus EF069 for control of postharvest apple decay and Rhizoctonia root rot on moth orchid. Journal of Applied Microbiology, 106(4), 1213–1219. https://doi.org/10.1111/j.1365-2672.2008.04087.x
- Li, Q., Ning, P., Zheng, L., Huang, J., Li, G., & Hsiang, T. (2010). Fumigant activity of volatiles of streptomyces globisporus JK-1 against Penicillium italicum on Citrus microcarpa. Postharvest Biology and Technology, 58(2), 157–165. https://doi.org/10.1016/j.postharvbio.2010.06.003
- Li, Q., Wu, L., Hao, J., Luo, L., Cao, Y., & Li, J. (2015). Biofumigation on post-harvest diseases of fruits using a new volatile-producing fungus of Ceratocystis fimbriata. PLOS ONE, 10(7), e0132009. https://doi.org/10.1371/journal.pone.0132009
- Lim, S. M., Yoon, M. Y., Choi, G. J., Choi, Y. H., Jang, K. S., Shin, T. S., Park, H. W., Yu, N. H., Kim, Y. H., & Kim, J. C. (2017). Diffusible and volatile antifungal compounds produced by an antagonistic Bacillus velezensis G341 against various phytopathogenic fungi. The Plant Pathology Journal, 33(5), 488–498. https://doi.org/10.5423/PPJ.OA.04.2017.0073
- Liu, P., Cheng, Y., Yang, M., Liu, Y., Chen, K., Long, C., & Deng, X. (2014). Mechanisms of action for 2-phenylethanol isolated from Kloeckera apiculata in control of Penicillium molds of citrus fruits. BMC Microbiology, 14(1), 242. https://doi.org/10.1186/s12866-014-0242-2
- Lucca, A. J. D., Boué, S. M., Carter-Wientjes, C. H., Bland, J. M., Bhatnagar, D., & Cleveland, T. E. (2010). Volatile profiles of toxigenic and non-toxigenic Aspergillus flavus using SPME for solid phase extraction. Annual of Agriculture, Environment and Medicine, 17(2), 301–308.
- Lutz, M. C., Lopes, C. A., Rodriguez, M. E., Sosa, M. C., & Sangorrín, M. P. (2013). Efficacy and putative mode of action of native and commercial antagonistic yeasts against postharvest pathogens of pear. International Journal of Food Microbiology, 164(2–3), 166–172. https://doi.org/10.1016/j.ijfoodmicro.2013.04.005
- Lyu, A., Yang, L., Wu, M., Zhang, J., & Li, G. (2020). High efficacy of the volatile organic compounds of Streptomyces yanglinensis 3-10 in suppression of Aspergillus contamination on peanut kernels. Frontiers in Microbiology, 11, 142. https://doi.org/10.3389/fmicb.2020.00142
- Mannaa, M., & Kim, K. D. (2018). Biocontrol activity of volatile-producing Bacillus megaterium and Pseudomonas protegens against Aspergillus and Penicillium spp. Predominant in stored rice grains: Study II. Mycobiology, 46(1), 52–63. https://doi.org/10.1080/12298093.2018.1454015
- Mannaa, M., Oh, J. Y., & Kim, K. D. (2017). Biocontrol activity of volatile-producing Bacillus megaterium and Pseudomonas protegens against Aspergillus flavus and aflatoxin production on stored rice grains. Mycobiology, 45(3), 213–219. https://doi.org/10.5941/MYCO.2017.45.3.213
- Mari, M., Bautista-Baños, S., & Sivakumar, D. (2016). Decay control in the postharvest system: Role of microbial and plant volatile organic compounds. Postharvest Biology and Technology, 122, 70–81. https://doi.org/10.1016/j.postharvbio.2016.04.014
- Mari, M., Martini, C., Spadoni, A., Rouissi, W., & Bertolini, P. (2012). Biocontrol of apple postharvest decay by Aureobasidium pullulans. Postharvest Biology and Technology, 73, 56–62. https://doi.org/10.1016/j.postharvbio.2012.05.014
- Masoud, W., & Kaltoft, C. H. (2006). The effects of yeasts involved in the fermentation of Coffea arabica in East Africa on growth and ochratoxin A (OTA) production by Aspergillus ochraceus. International Journal of Food Microbiology, 106(2), 229–234. https://doi.org/10.1016/j.ijfoodmicro.2005.06.015
- Masoud, W., Poll, L., & Jakobsen, M. (2005). Influence of volatile compounds produced by yeasts predominant during processing of Coffea arabica in East Africa on growth and ochratoxin A (OTA) production by Aspergillus ochraceus. Yeast, 22(14), 1133–1142. https://doi.org/10.1002/yea.1304
- Mauro, A., Garcia-Cela, E., Pietri, A., Cotty, P., & Battilani, P. (2018). Biological control products for aflatoxin prevention in Italy: Commercial field evaluation of atoxigenic Aspergillus flavus active ingredients. Toxins, 10(1), 30. https://doi.org/10.3390/toxins10010030
- Maxwell, L. A., Callicott, K. A., Bandyopadhyay, R., Mehl, H. L., Orbach, M. J., & Cotty, P. J. (2021). Degradation of aflatoxins B1 by Atoxigenic Aspergillus flavus biocontrol agents. Plant Disease, 105(9), 2343–2350. https://doi.org/10.1094/PDIS-01-21-0066-RE
- Medina-Córdova, N., López-Aguilar, R., Ascencio, F., Castellanos, T., Campa-Córdova, A. I., & Angulo, C. (2016). Biocontrol activity of the marine yeast Debaryomyces hansenii against phytopathogenic fungi and its ability to inhibit mycotoxins production in maize grain (Zea mays L.). Biological Control, 97, 70–79. https://doi.org/10.1016/j.biocontrol.2016.03.006
- Medina-Córdova, N., Rosales-Mendoza, S., Hernández-Montiel, L. G., & Angulo, C. (2018). The potential use of Debaryomyces hansenii for the biological control of pathogenic fungi in food. Biological Control, 121, 216–222. https://doi.org/10.1016/j.biocontrol.2018.03.002
- Medina-Romero, Y. M., Roque-Flores, G., & Macías-Rubalcava, M. L. (2017). Volatile organic compounds from endophytic fungi as innovative postharvest control of Fusarium oxysporum in cherry tomato fruits. Applied Microbiology and Biotechnology, 101(22), 8209–8222. https://doi.org/10.1007/s00253-017-8542-8
- Mercier, J., & Jiménez, J. I. (2004). Control of fungal decay of apples and peaches by the biofumigant fungus Muscodor albus. Postharvest Biology and Technology, 31(1), 1–8. https://doi.org/10.1016/j.postharvbio.2003.08.004
- Moore, G. G. (2022). Practical considerations will ensure the continued success of pre-harvest biocontrol using non-aflatoxigenic Aspergillus flavus strains. Critical Reviews in Food Science and Nutrition, 62(15), 4208–4225. https://doi.org/10.1080/10408398.2021.1873731
- Moore, G. G., Lebar, M. D., & Carter-Wientjes, C. H. (2019). The role of extrolites secreted by nonaflatoxigenic Aspergillus flavus in biocontrol efficacy. Journal of Applied Microbiology, 126(4), 1257–1264. https://doi.org/10.1111/jam.14175
- Moore, G. G., Lebar, M. D., & Carter-Wientjes, C. H. (2022). Cumulative effects of non-aflatoxigenic Aspergillus flavus volatile organic compounds to abate toxin production by mycotoxigenic Aspergilli. Toxins, 14(5), 340. https://doi.org/10.3390/toxins14050340
- Moore, G. G., Lebar, M. D., Carter-Wientjes, C. H., & Gilbert, M. K. (2021). The potential role of fungal volatile organic compounds in Aspergillus flavus biocontrol efficacy. Biological Control, 160, 104686. https://doi.org/10.1016/j.biocontrol.2021.104686
- Moral, J., Garcia-Lopez, M. T., Camiletti, B. X., Jaime, R., Michailides, T. J., Bandyopadhyay, R., & Ortega-Beltran, A. (2020). Present status and perspective on the future use of aflatoxin biocontrol products. Agronomy, 10(4), 491. https://doi.org/10.3390/agronomy10040491
- Morita, T., Tanaka, I., Ryuda, N., Ikari, M., Ueno, D., & Someya, T. (2019). Antifungal spectrum characterization and identification of strong volatile organic compounds produced by Bacillus pumilus TM-R. Heliyon, 5(6), e01817. https://doi.org/10.1016/j.heliyon.2019.e01817
- Nally, M. C., Pesce, V. M., Maturano, Y. P., Rodriguez Assaf, L. A., Toro, M. E., Castellanos de Figueroa, L. I., & Vazquez, F. (2015). Antifungal modes of action of Saccharomyces and other biocontrol yeasts against fungi isolated from sour and grey rots. International Journal of Food Microbiology, 204, 91–100. https://doi.org/10.1016/j.ijfoodmicro.2015.03.024
- Nasrollahzadeh, A., Mokhtari, S., Khomeiri, M., & Saris, P. E. J. (2022). Antifungal preservation of food by lactic acid bacteria. Foods (Basel, Switzerland), 11(3), 395. https://doi.org/10.3390/foods11030395
- Nazik, H., Sass, G., Déziel, E., & Stevens, D. A. (2020). Aspergillus is inhibited by Pseudomonas aeruginosa volatiles. Journal of Fungi, 6(3), 118. https://doi.org/10.3390/jof6030118
- Nešić, K., Habschied, K., & Mastanjević, K. (2021). Possibilities for the biological control of mycotoxins in food and feed. Toxins, 13(3), 198. https://doi.org/10.3390/toxins13030198
- Núñez, F., Lara, M. S., Peromingo, B., Delgado, J., Sánchez-Montero, L., & Andrade, M. J. (2015). Selection and evaluation of Debaryomyces hansenii isolates as potential bioprotective agents against toxigenic penicillia in dry-fermented sausages. Food Microbiology, 46, 114–120. https://doi.org/10.1016/j.fm.2014.07.019
- Okcu, Z., Yavuz, Y., & Kerse, S. (2018). Edible film and coating applications in fruits and vegetables. Alınteri Zirai Bilimler Dergisi, 221–226. https://doi.org/10.28955/alinterizbd.368362
- Omotayo, O. P., Omotayo, A. O., Mwanza, M., & Babalola, O. O. (2019). Prevalence of mycotoxins and their consequences on human health. Toxicological Research, 35(1), 1–7. https://doi.org/10.5487/TR.2019.35.1.001
- Ons, L., Bylemans, D., Thevissen, K., & Cammue, B. P. A. (2020). Combining biocontrol agents with chemical fungicides for integrated plant fungal disease control. Microorganisms, 8(12), 1930. https://doi.org/10.3390/microorganisms8121930
- Oro, L., Feliziani, E., Ciani, M., Romanazzi, G., & Comitini, F. (2018). Volatile organic compounds from Wickerhamomyces anomalus, Metschnikowia pulcherrima and Saccharomyces cerevisiae inhibit growth of decay causing fungi and control postharvest diseases of strawberries. International Journal of Food Microbiology, 265, 18–22. https://doi.org/10.1016/j.ijfoodmicro.2017.10.027
- Osaki, C., Yamaguchi, K., Urakawa, S., Nakashima, Y., Sugita, K., Nagaishi, M., Mitsuiki, S., Kuraoka, T., Ogawa, Y., & Sato, H. (2019). The bacteriological properties of Bacillus strain TM-I-3 and analysis of the volatile antifungal compounds emitted by this bacteria. Biocontrol Science, 24(3), 129–136. https://doi.org/10.4265/bio.24.129
- Ossowicki, A., Jafra, S., & Garbeva, P. (2017). The antimicrobial volatile power of the rhizospheric isolate Pseudomonas donghuensis P482. PLOS ONE, 12(3), e0174362. https://doi.org/10.1371/journal.pone.0174362
- Palumbo, J. D., O’Keeffe, T. L., & Abbas, H. K. (2007). Isolation of maize soil and rhizosphere bacteria with antagonistic activity against Aspergillus flavus and Fusarium verticillioides. Journal of Food Protection, 70(7), 1615–1621. https://doi.org/10.4315/0362-028X-70.7.1615
- Papp, L. A., Horváth, E., Peles, F., Pócsi, I., & Miklós, I. (2021). Insight into yeast–mycotoxin relations. Agriculture, 11(12), 1291. https://doi.org/10.3390/agriculture11121291
- Passone, M. A., & Etcheverry, M. (2014). Antifungal impact of volatile fractions of Peumus boldus and Lippia turbinata on Aspergillus section Flavi and residual levels of these oils in irradiated peanut. International Journal of Food Microbiology, 168–169, 17–23. https://doi.org/10.1016/j.ijfoodmicro.2013.10.009
- Pennerman, K. K., Scarsella, J. B., Yin, G.-H., Hua, S.-S. T., Hartman, T. G., & Bennett, J. W. (2019). Volatile 1-octen-3-ol increases patulin production by Penicillium expansum on a patulin-suppressing medium. Mycotoxin Research, 35(4), 329–340. https://doi.org/10.1007/s12550-019-00348-w
- Polizzi, V., Fazzini, L., Adams, A., Picco, A. M., De Saeger, S., Van Peteghem, C., & De Kimpe, N. (2011). Autoregulatory properties of (+)-Thujopsene and influence of environmental conditions on its production by Penicillium decumbens. Microbial Ecology, 62(4), 838. https://doi.org/10.1007/s00248-011-9905-9
- Rao, Y., Zeng, L., Jiang, H., Mei, L., & Wang, Y. (2022). Trichoderma atroviride LZ42 releases volatile organic compounds promoting plant growth and suppressing Fusarium wilt disease in tomato seedlings. BMC Microbiology, 22(1), 88. https://doi.org/10.1186/s12866-022-02511-3
- Reis, T. A., Oliveira, T. D., Zorzete, P., Faria, P., & Corrêa, B. (2020). A non-toxigenic Aspergillus flavus strain prevents the spreading of Fusarium verticillioides and fumonisins in maize. Toxicon, 181, 6–8. https://doi.org/10.1016/j.toxicon.2020.04.091
- Rouissi, W., Ugolini, L., Martini, C., Lazzeri, L., & Mari, M. (2013). Control of postharvest fungal pathogens by antifungal compounds from Penicillium expansum. Journal of Food Protection, 76(11), 1879–1886. https://doi.org/10.4315/0362-028X.JFP-13-072
- Ryu, C. M., Farag, M. A., Hu, C. H., Reddy, M. S., Wei, H. X., Paré, P. W., & Kloepper, J. W. (2003). Bacterial volatiles promote growth in Arabidopsis. Proceedings of the National Academy of Sciences, 100(8), 4927–4932. https://doi.org/10.1073/pnas.0730845100
- Sadiq, F. A., Yan, B., Tian, F., Zhao, J., Zhang, H., & Chen, W. (2019). Lactic acid bacteria as antifungal and anti-mycotoxigenic agents: A comprehensive review. Comprehensive Reviews in Food Science and Food Safety, 18(5), 1403–1436. https://doi.org/10.1111/1541-4337.12481
- Salas, M., Mounier, J., Valence, F., Coton, M., Thierry, A., & Coton, E. (2017). Antifungal microbial agents for food biopreservation – A review. Microorganisms, 5(3), 37. https://doi.org/10.3390/microorganisms5030037
- Saleh, A. E., Ul-Hassan, Z., Zeidan, R., Al-Shamary, N., Al-Yafei, T., Alnaimi, H., Higazy, N. S., Migheli, Q., & Jaoua, S. (2021). Biocontrol activity of Bacillus megaterium BM344-1 against toxigenic fungi. ACS Omega, 6(16), 10984–10990. https://doi.org/10.1021/acsomega.1c00816
- Santiago, R., Cao, A., & Butrón, A. (2015). Genetic factors involved in fumonisin accumulation in maize kernels and their implications in maize agronomic management and breeding. Toxins, 7(8), 3267–3296. https://doi.org/10.3390/toxins7083267
- Scherm, B., Palomba, M., Serra, D., Marcello, A., & Migheli, Q. (2005). Detection of transcripts of the aflatoxin genes aflD, aflO, and aflP by reverse transcription–polymerase chain reaction allows differentiation of aflatoxin-producing and non-producing isolates of Aspergillus flavus and Aspergillus parasiticus. International Journal of Food Microbiology, 98(2), 201–210. https://doi.org/10.1016/j.ijfoodmicro.2004.06.004
- Schmidt, R., Cordovez, V., de Boer, W., Raaijmakers, J., & Garbeva, P. (2015). Volatile affairs in microbial interactions. The ISME Journal, 9(11), 2329–2335. https://doi.org/10.1038/ismej.2015.42
- Schmidt, R., Etalo, D. W., de Jager, V., Gerards, S., Zweers, H., de Boer, W., & Garbeva, P. (2016). Microbial small talk: Volatiles in fungal–bacterial interactions. Frontiers in Microbiology, 6. https://doi.org/10.3389/fmicb.2015.01495
- Schulz-Bohm, K., Martín-Sánchez, L., & Garbeva, P. (2017). Microbial volatiles: Small molecules with an important role in intra- and inter-Kingdom Interactions. Frontiers in Microbiology, 8, 2484. https://doi.org/10.3389/fmicb.2017.02484
- Scott, J., Sueiro-Olivares, M., Ahmed, W., Heddergott, C., Zhao, C., Thomas, R., Bromley, M., Latgé, J.-P., Krappmann, S., Fowler, S., Bignell, E., & Amich, J. (2019). Pseudomonas aeruginosa-derived volatile sulfur compounds promote distal Aspergillus fumigatus growth and a synergistic pathogen-pathogen interaction that increases pathogenicity in ao-infection. Frontiers in Microbiology, 10, 2311. https://doi.org/10.3389/fmicb.2019.02311
- Settier-Ramírez, L., López-Carballo, G., Hernández-Muñoz, P., Fontana, A., Strub, C., & Schorr-Galindo, S. (2021). New isolated Metschnikowia pulcherrima strains from apples for postharvest biocontrol of Penicillium expansum and patulin accumulation. Toxins, 13(6), 397. https://doi.org/10.3390/toxins13060397
- Shabeer, S., Asad, S., Jamal, A., & Ali, A. (2022). Aflatoxin contamination, its impact and management strategies: An updated review. Toxins, 14(5), 307. https://doi.org/10.3390/toxins14050307
- Shehata, M. G., Badr, A. N., El Sohaimy, S. A., Asker, D., & Awad, T. S. (2019). Characterization of antifungal metabolites produced by novel lactic acid bacterium and their potential application as food biopreservatives. Annals of Agricultural Sciences, 64(1), 71–78. https://doi.org/10.1016/j.aoas.2019.05.002
- Shi, C., Yan, P., Li, J., Wu, H., Li, Q., & Guan, S. (2014). Biocontrol of Fusarium graminearum growth and deoxynivalenol production in wheat kernels with bacterial antagonists. International Journal of Environmental Research and Public Health, 11(1), 1094–1105. https://doi.org/10.3390/ijerph110101094
- Simoncini, N., Pinna, A., Toscani, T., & Virgili, R. (2015). Effect of added autochthonous yeasts on the volatile compounds of dry-cured hams. International Journal of Food Microbiology, 212, 25–33. https://doi.org/10.1016/j.ijfoodmicro.2015.06.024
- Singh, D., & Lee, C. H. (2018). Intraspecies volatile interactions affect growth rates and exometabolomes in Aspergillus oryzae KCCM 60345. Journal of Microbiology and Biotechnology, 28(2), 199–209. https://doi.org/10.4014/jmb.1711.11005
- Sipos, P., Peles, F., Brassó, D. L., Béri, B., Pusztahelyi, T., Pócsi, I., & Győri, Z. (2021). Physical and chemical methods for reduction in aflatoxin content of feed and food. Toxins, 13(3), 204. https://doi.org/10.3390/toxins13030204
- Smaoui, S., Agriopoulou, S., D’Amore, T., Tavares, L., & Mousavi Khaneghah, A. (2022). The control of Fusarium growth and decontamination of produced mycotoxins by lactic acid bacteria. Critical Reviews in Food Science and Nutrition, 1–28. https://doi.org/10.1080/10408398.2022.2087594
- Spadaro, D., & Droby, S. (2016). Development of biocontrol products for postharvest diseases of fruit: The importance of elucidating the mechanisms of action of yeast antagonists. Trends in Food Science & Technology, 47, 39–49. https://doi.org/10.1016/j.tifs.2015.11.003
- Ström, K., Sjögren, J., Broberg, A., & Schnürer, J. (2002). Lactobacillus plantarum MiLAB 393 produces the antifungal cyclic dipeptides Cyclo(l -Phe- l -Pro) and Cyclo(l -Phe- trans -4-OH- l -Pro) and 3-Phenyllactic acid. Applied and Environmental Microbiology, 68(9), 4322–4327. https://doi.org/10.1128/AEM.68.9.4322-4327.2002
- Sultan, M., Hafez, O. M., Saleh, M. A., & Youssef, A. M. (2021). Smart edible coating films based on chitosan and beeswax–pollen grains for the postharvest preservation of Le Conte pear. RSC Advances, 11(16), 9572–9585. https://doi.org/10.1039/D0RA10671B
- Suwannarach, N., Kumla, J., Bussaban, B., Nuangmek, W., Matsui, K., & Lumyong, S. (2013). Biofumigation with the endophytic fungus Nodulisporium spp. CMU-UPE34 to control postharvest decay of citrus fruit. Crop Protection, 45, 63–70. https://doi.org/10.1016/j.cropro.2012.11.015
- Tamreihao, K., Ningthoujam, D. S., Nimaichand, S., Singh, E. S., Reena, P., Singh, S. H., & Nongthomba, U. (2016). Biocontrol and plant growth promoting activities of a Streptomyces corchorusii strain UCR3-16 and preparation of powder formulation for application as biofertilizer agents for rice plant. Microbiological Research, 192, 260–270. https://doi.org/10.1016/j.micres.2016.08.005
- Tejero, P., Martín, A., Rodríguez, A., Galván, A. I., Ruiz-Moyano, S., & Hernández, A. (2021). In vitro biological control of Aspergillus flavus by Hanseniaspora opuntiae L479 and Hanseniaspora uvarum L793, producers of antifungal volatile organic compounds. Toxins, 13(9), 663. https://doi.org/10.3390/toxins13090663
- Tenorio-Salgado, S., Tinoco, R., Vazquez-Duhalt, R., Caballero-Mellado, J., & Perez-Rueda, E. (2013). Identification of volatile compounds produced by the bacterium Burkholderia tropica that inhibit the growth of fungal pathogens. Bioengineered, 4(4), 236–243. https://doi.org/10.4161/bioe.23808
- Tilocca, B., Balmas, V., Hassan, Z. U., Jaoua, S., & Migheli, Q. (2019). A proteomic investigation of Aspergillus carbonarius exposed to yeast volatilome or to its major component 2-phenylethanol reveals major shifts in fungal metabolism. International Journal of Food Microbiology, 306, 108265. https://doi.org/10.1016/j.ijfoodmicro.2019.108265
- Tilocca, B., Cao, A., & Migheli, Q. (2020). Scent of a killer: Microbial volatilome and its role in the biological control of plant pathogens. Frontiers in Microbiology, 11, 41. https://doi.org/10.3389/fmicb.2020.00041
- Toral, L., Rodríguez, M., Martínez-Checa, F., Montaño, A., Cortés-Delgado, A., Smolinska, A., Llamas, I., & Sampedro, I. (2021). Identification of volatile organic compounds in extremophilic bacteria and their effective use in biocontrol of postharvest fungal phytopathogens. Frontiers in Microbiology, 12, 773092. https://doi.org/10.3389/fmicb.2021.773092
- Ul Hassan, Z., Al Thani, R., Alnaimi, H., Migheli, Q., & Jaoua, S. (2019). Investigation and application of Bacillus licheniformis volatile compounds for the biological control of toxigenic Aspergillus and Penicillium spp. ACS Omega, 4(17), 17186–17193. https://doi.org/10.1021/acsomega.9b01638
- Ul Hassan, Z., Al Thani, R., Atia, F. A., Al Meer, S., Migheli, Q., & Jaoua, S. (2018). Co-occurrence of mycotoxins in commercial formula milk and cereal-based baby food on the Qatar market. Food Additives & Contaminants: Part B, 11(3), 191–197. https://doi.org/10.1080/19393210.2018.1437785
- Ul Hassan, Z., Al Thani, R., Atia, F. A., Alsafran, M., Migheli, Q., & Jaoua, S. (2021). Application of yeasts and yeast derivatives for the biological control of toxigenic fungi and their toxic metabolites. Environmental Technology & Innovation, 22, 101447. https://doi.org/10.1016/j.eti.2021.101447
- Valerio, F., Di Biase, M., Lattanzio, V. M. T., & Lavermicocca, P. (2016). Improvement of the antifungal activity of lactic acid bacteria by addition to the growth medium of phenylpyruvic acid, a precursor of phenyllactic acid. International Journal of Food Microbiology, 222, 1–7. https://doi.org/10.1016/j.ijfoodmicro.2016.01.011
- Valero, D., Valverde, J. M., Martínez-Romero, D., Guillén, F., Castillo, S., & Serrano, M. (2006). The combination of modified atmosphere packaging with eugenol or thymol to maintain quality, safety and functional properties of table grapes. Postharvest Biology and Technology, 41(3), 317–327. https://doi.org/10.1016/j.postharvbio.2006.04.011
- Valverde, J. M., Guillén, F., Martínez-Romero, D., Castillo, S., Serrano, M., & Valero, D. (2005). Improvement of table grapes quality and safety by the combination of modified atmosphere packaging (MAP) and eugenol, menthol, or thymol. Journal of Agricultural and Food Chemistry, 53(19), 7458–7464. https://doi.org/10.1021/jf050913i
- van Egmond, H. P., Schothorst, R. C., & Jonker, M. A. (2007). Regulations relating to mycotoxins in food: Perspectives in a global and European context. Analytical and Bioanalytical Chemistry, 389(1), 147–157. https://doi.org/10.1007/s00216-007-1317-9
- Varsha, K. K., Devendra, L., Shilpa, G., Priya, S., Pandey, A., & Nampoothiri, K. M. (2015). 2,4-Di-tert-butyl phenol as the antifungal, antioxidant bioactive purified from a newly isolated Lactococcus sp. International Journal of Food Microbiology, 211, 44–50. https://doi.org/10.1016/j.ijfoodmicro.2015.06.025
- Verma, D. K., Thyab Gddoa Al-Sahlany, S., Kareem Niamah, A., Thakur, M., Shah, N., Singh, S., Baranwal, D., Patel, A. R., Lara Utama, G., & Noe Aguilar, C. (2022). Recent trends in microbial flavour compounds: A review on chemistry, synthesis mechanism and their application in food. Saudi Journal of Biological Sciences, 29(3), 1565–1576. https://doi.org/10.1016/j.sjbs.2021.11.010
- Veselova, M. A., Plyuta, V. A., & Khmel, I. A. (2019). Volatile compounds of bacterial origin: Structure, biosynthesis, and biological activity. Microbiology, 88(3), 261–274. https://doi.org/10.1134/S0026261719030160
- Wang, C., Wang, Z., Qiao, X., Li, Z., Li, F., Chen, M., Wang, Y., Huang, Y., & Cui, H. (2013). Antifungal activity of volatile organic compounds from Streptomyces alboflavus TD-1. FEMS Microbiology Letters, 341(1), 45–51. https://doi.org/10.1111/1574-6968.12088
- Wang, Z., Zhong, T., Chen, K., Du, M., Chen, G., Chen, X., Wang, K., Zalán, Z., Takács, K., & Kan, J. (2021). Antifungal activity of volatile organic compounds produced by Pseudomonas fluorescens ZX and potential biocontrol of blue mold decay on postharvest citrus. Food Control, 120, 107499. https://doi.org/10.1016/j.foodcont.2020.107499
- Wani, M. A., Sanjana, K., Kumar, D. M., & Lal, D. K. (2010). GC-MS analysis reveals production of 2–Phenylethanol from Aspergillus niger endophytic in rose. Journal of Basic Microbiology, 50(1), 110–114. https://doi.org/10.1002/jobm.200900295
- Watve, M., Tickoo, R., Jog, M., & Bhole, B. (2001). How many antibiotics are produced by the genus Streptomyces ? Archives of Microbiology, 176(5), 386–390. https://doi.org/10.1007/s002030100345
- Weaver, M. A., & Abbas, H. K. (2019). Field displacement of aflatoxigenic Aspergillus flavus strains through repeated biological control applications. Frontiers in Microbiology, 10, 1788. https://doi.org/10.3389/fmicb.2019.01788
- Weisskopf, L., Schulz, S., & Garbeva, P. (2021). Microbial volatile organic compounds in intra-kingdom and inter-kingdom interactions. Nature Reviews Microbiology, 19(6), 391–404. https://doi.org/10.1038/s41579-020-00508-1
- Xu, R., Kiarie, E. G., Yiannikouris, A., Sun, L., & Karrow, N. A. (2022). Nutritional impact of mycotoxins in food animal production and strategies for mitigation. Journal of Animal Science and Biotechnology, 13(1), 69. https://doi.org/10.1186/s40104-022-00714-2
- Yan, L., Song, W., Chen, Y., Kang, Y., Lei, Y., Huai, D., Wang, Z., Wang, X., & Liao, B. (2021). Effect of non-aflatoxigenic strains of Aspergillus flavus on aflatoxin contamination of pre-harvest peanuts in fields in China. Oil Crop Science, 6(2), 81–86. https://doi.org/10.1016/j.ocsci.2021.04.004
- Yang, E. J., & Chang, H. C. (2010). Purification of a new antifungal compound produced by Lactobacillus plantarum AF1 isolated from kimchi. International Journal of Food Microbiology, 139(1–2), 56–63. https://doi.org/10.1016/j.ijfoodmicro.2010.02.012
- Yang, M., Huang, C., Xue, Y., Li, S., Lu, L., & Wang, C. (2018). Biofumigation with volatile organic compounds from Streptomyces alboflavus TD-1 and pure chemicals to control Aspergillus ochraceus. Annual of Applied Biology, 173(3), 313–322. https://doi.org/10.1111/aab.12465
- Yang, M., Lu, L., Pang, J., Hu, Y., Guo, Q., Li, Z., Wu, S., Liu, H., & Wang, C. (2019). Biocontrol activity of volatile organic compounds from Streptomyces alboflavus TD-1 against Aspergillus flavus growth and aflatoxin production. Journal of Microbiology, 57(5), 396–404. https://doi.org/10.1007/s12275-019-8517-9
- Yang, Y., Chen, Y., Cai, J., Liu, X., & Huang, G. (2021). Antifungal activity of volatile compounds generated by endophytic fungi Sarocladium brachiariae HND5 against Fusarium oxysporum f. Sp. Cubense. PLOS ONE, 16(12), e0260747. https://doi.org/10.1371/journal.pone.0260747
- Yin, Y., Yan, L., Jiang, J., & Ma, Z. (2008). Biological control of aflatoxin contamination of crops. Journal of Zhejiang University SCIENCE B, 9(10), 787–792. https://doi.org/10.1631/jzus.B0860003
- You, J., Li, G., Li, C., Zhu, L., Yang, H., Song, R., & Gu, W. (2022). Biological control and plant growth promotion by volatile organic compounds of Trichoderma koningiopsis T-51. Journal of Fungi, 8(2), 131. https://doi.org/10.3390/jof8020131
- Yuan, J., Raza, W., Shen, Q., & Huang, Q. (2012). Antifungal activity of Bacillus amyloliquefaciens NJN-6 volatile compounds against Fusarium oxysporum f. sp. Cubense. Applied and Environmental Microbiology, 78(16), 5942–5944. https://doi.org/10.1128/AEM.01357-12
- Zeidan, R., Ul-Hassan, Z., Al-Thani, R., Balmas, V., & Jaoua, S. (2018). Application of low-fermenting yeast Lachancea thermotolerans for the control of toxigenic fungi Aspergillus parasiticus, Penicillium verrucosum and Fusarium graminearum and their mycotoxins. Toxins, 10(6), 242. https://doi.org/10.3390/toxins10060242