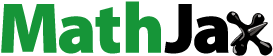
ABSTRACT
The ability to travel independently is a vital part of an autonomous life. It is important to investigate to what degree people with acquired brain injuries (ABI) suffer from navigation impairments. The aim of this study was to investigate the prevalence and characteristics of objective and subjective navigation impairments in the population of ABI patients. A large-scale online navigation study was conducted with 435 ABI patients and 7474 healthy controls. Participants studied a route through a virtual environment and completed 5 navigation tasks that assessed distinct functional components of navigation ability. Subjective navigation abilities were assessed using the Wayfinding questionnaire. Patients were matched to controls using propensity score matching. Overall, performance on objective navigation tasks was significantly lower in the ABI population compared to the healthy controls. The landmark recognition, route continuation and allocentric location knowledge tasks were most vulnerable to brain injury. The prevalence of subjective navigation impairments was higher in the ABI population compared to the healthy controls. In conclusion, a substantial proportion (39.1%) of the ABI population reports navigation impairments. We advocate the evaluation of objective and subjective navigation ability in neuropsychological assessments of ABI patients.
Introduction
Acquired brain injury (ABI) refers to brain injury following rapid onset damage to the brain after birth that is not caused by hereditary, congenital or degenerative events (Tibæk et al., Citation2018; Turner-Strokes, Citation2003). The most common types of ABIs result from cerebrovascular accidents (CVA), traumatic brain Injury (TBI) and brain tumours, whereas less common causes of ABIs include hypoxia, intoxication, and infection (Turner-Strokes, Citation2003). In the Netherlands, a country with 17.2 million inhabitants, approximately 645,900 people (38 out of 1000) suffer from an acquired brain injury (RIVM, Citation2016).
ABI can have a profound impact on a patient’s life. Patients often report cognitive impairments (e.g., working memory, executive functioning, attention) (Rees et al., Citation2007) in addition to social, emotional and behavioural problems (Cattelani et al., Citation2010; Milders et al., Citation2003). These impairments typically carry over to activities of daily living such as returning to work, doing groceries or maintaining a social network (Häggström & Lund, Citation2008; Schipper et al., Citation2011; Van Velzen et al., Citation2009; Wade et al., Citation2018).
Professional occupation, running a household and attending social events, often require us to travel between locations. As such, the ability to navigate plays a key role in maintaining an independent life (Sohlberg et al., Citation2005). The impact of navigation impairments on daily life has been shown to be substantial. In a sample of mild stroke patients, 29% of the participants reported navigation problems. The levels of reported impairments correlate strongly with psychosocial quality of life, stressing the importance of independent navigation for activities of daily living (van der Ham et al., Citation2013).
Navigation impairments are not limited to stroke patients. Neuropsychological case studies report navigation problems in TBI patients (Rosenbaum et al., Citation2000), brain tumour patients (van der Ham et al., Citation2010) and patients with brain injury as a result of hypoxia (Herdman et al., Citation2015), infections (Hirayama et al., Citation2003; Maguire et al., Citation2006) and intoxication (Turriziani et al., Citation2003). However, the prevalence of these problems among the ABI population at large is currently unknown. There is reason to expect that navigation impairments are common in patients with multiple types of ABI. Spatial navigation is a high level cognitive ability that is supported by a range of cognitive functions and brain networks (Boccia et al., Citation2014; Wolbers & Hegarty, Citation2010). As such, navigation ability should not be regarded as a singular cognitive function, but rather, as a synergy of distinct cognitive processes. Disruption of these systems as a result of brain injury can lead to a wide variety of difficulties when navigating in an environment.
To understand the nature of the navigation problems reported after ABI, it is important to investigate what component of navigation ability is afflicted in a patient. There have been several attempts to capture the components of navigation into a model. Siegel and White (Citation1975) proposed an influential framework in which spatial knowledge is subdivided in landmark, route and survey knowledge (L–R–S framework). According to this framework, spatial knowledge is acquired sequentially. First, fine-grained knowledge about landmarks in the environment is obtained. Then, spatial and temporal relations of landmarks along routes are learned. Finally, survey knowledge is formed, resembling a cognitive map of the environment, allowing navigators to take shortcuts or sketch maps of the environment. While the subdivision of knowledge types has not been disputed, later studies demonstrate that the spatial knowledge is not necessarily obtained in a sequential fashion, nor does extensive route knowledge always lead to survey knowledge (Ishikawa & Montello, Citation2006). Furthermore, several important aspects of navigation ability are not taken into account in this model, such as perspective taking and spatial updating (Blajenkova et al., Citation2005; Zhong & Kozhevnikov, Citation2016).
A different line of research has focussed on the formation and use of spatial memory. Core concepts to these studies include egocentric (self-centred) and allocentric (world-centred) representations (Klatzky, Citation1998). Egocentric and allocentric representations determine how people orientate themselves in an environment, how locations and places are memorized and what spatial strategies are used during navigation (Bullens et al., Citation2010; Burgess, Citation2006; Wen et al., Citation2013). Importantly, these studies show that egocentric and allocentric representations are constructed in parallel (Iglói et al., Citation2009). This suggests that a map-like understanding of the environment (allocentric representation) can be formed during initial exposure to an environment.
More recently, Claessen and van der Ham (Citation2017) have proposed a classification of navigation impairments based on the functional properties of navigation impairment found in neuropsychological case studies reported in the literature. This model combines elements from both the L–R–S framework and research on spatial memory. This classification entails three distinct functional domains of navigation ability that are particularly relevant for understanding navigation impairment: knowledge of landmarks, locations, and paths.
Landmarks serve as beacons and reference points in the environment, marking important decision points and allowing navigators to maintain oriented along a route (Chan et al., Citation2012; Sorrows & Hirtle, Citation1999). Landmark-based navigation impairments concern a defect in the ability to encode, retrieve or recognize salient objects (e.g., a statue or building) in an environment. The main neural correlates involved in landmark processing are the parahippocampal place area, and the retrosplenial complex and the prefrontal cortex (Epstein, Citation2008; Janzen & Jansen, Citation2010). In addition, lesions to the right medial occipito-temporal lobe are often associated with landmark impairments (Epstein et al., Citation2001; Landis et al., Citation1986; Mendez & Cherrier, Citation2003; Takahashi & Kawamura, Citation2002; van der Ham et al., Citation2010).
Location-based navigation impairments describe problems in remembering, processing and updating the locations of landmarks in an environment (Burgess, Citation2006). In order to understand the location of objects in an environment, one constructs a mental representation of space. Impairments occur in the construction of egocentric representations (understanding where objects are in relation to your own location) and allocentric representations (understanding the configuration of objects in the environment regardless of your own location) (Klatzky, Citation1998). The parietal cortex is the key neural correlate involved in processing egocentric references frames while the hippocampus, parahippocampal gyrus and thalamus are typically involved processing allocentric representations during navigation (Colombo et al., Citation2017; Johnson & Davis, Citation1998).
Path–based navigation impairments describe difficulties in understanding how locations in the environment are connected to each other. This includes the use and formation of route knowledge (Siegel & White, Citation1975). For example, understanding the order in which landmarks are encountered along a route or remembering what direction one should take at intersections to continue along a route. Path-based navigation impairments also describe survey knowledge; the ability to form and utilize a map-like understanding of an environment (Siegel & White, Citation1975). This allows navigators to take shortcuts, find novel routes, estimate the direction and distance between locations. The hippocampus is involved in both route and survey knowledge. In addition, route knowledge is supported by the medial temporal lobe whereas survey knowledge-based navigation is further supported by the inferior temporal cortex and the posterior superior parietal cortex (Brown et al., Citation2014; Shelton & Gabrieli, Citation2002).
Currently, navigation impairments receive relatively little attention in clinical practise. Common tests employed during patient intake, such as the MMSE (Zwecker et al., Citation2002), the MOCA (Nasreddine et al., Citation2005) and CLCE-24 (Van Heugten et al., Citation2007) typically do not assess navigation ability. Moreover, navigation ability is not accounted for in elaborate testing batteries (e.g., WAIS (Wechsler, Citation1987), BADS (Wilson et al., Citation1997)) employed in more comprehensive neuropsychological assessments. As a result, few healthcare centres inventory navigation ability among ABI patients. In order to determine whether navigation impairments require more attention in healthcare centres, we aimed to provide an overview of the prevalence and characteristics of navigation impairments among the ABI patient population. To this end, three goals have been formulated.
The first goal of the current study was to assess the occurrence of objective and subjective navigation impairments in the population of patients with ABI. In order to investigate the effects of ABI on navigation abilities, objective and subjective measures of navigation ability were compared between a group of ABI patients and a group of healthy controls. The second goal of this study was to determine what component of navigation ability is most often impaired in the ABI population. We will examine what domains of navigation abilities, landmark, location or path, are most vulnerable to brain injury. The third goal of this study was to investigate the prevalence of the different types of navigation impairments and to what degree these depend on ABI type and the location (hemisphere) of the lesion.
Providing a clear understanding of the scope and severity of navigation impairments amongst the ABI population will aid healthcare professionals in detecting and understanding problems that patients might experience in daily life. Additionally, results might provide insight with regard to the most common navigation impairments that a therapist might encounter, as well as the type of patient that is at risk of suffering from navigation problems. Finally, information on the prevalence of the impairment might help decide whether navigation assessment should be part of patient intake procedures.
Methods
Recruitment
The experiment was hosted online on the websites “navigerenkunjeleren.nl” and “weekendvandewetenschap.nl”. Participants were invited to participate in the study through national, local and social media, organized by The Weekend of Science. This is a Dutch annual event organized by the Secretary of Education, Science and Culture, with the goal of promoting science to the general public. Additionally, “hersenonderzoek.nl” an online platform that promotes research to an interested audience, was used to invite people to participate in the study. Two versions of the experiment were available online: a version for healthy participants (van der Ham et al., Citation2020) and a version for people with ABI (the current experiment). Inclusion criteria for the ABI participants were (1) older than 16 years old, (2) acquired brain injury and (3) access to stable internet connection. Psychiatric disorders were an exclusion criteria for participation. Due to the open nature of the experiment, no official medical records were obtained. As such, adherence of the in- and exclusion criteria was not verified. The study was approved by the local ethical committee at Leiden University, and conducted in accordance with the declaration of Helsinki (2013). Each participant provided informed consent prior to participation.
Tasks
The design of the experiment paradigm was similar to that described by van der Ham et al. (Citation2020). The experiment consisted of a general questionnaire, an objective navigation assessment and a questionnaire that was used to assess subjective navigation ability.
General questionnaire
The experiment started with a general questionnaire in which participants provided demographic information, including age, gender, education level (scores ranging from 1, lowest, to 7, highest (Verhage, Citation1964)) and the province (within the Netherlands) they lived in. Additionally, participants provided information about their spatial experience (How often do you travel to places you have not visited before?), with response options (“never”, “several times a year”, “several times a month”, “weekly or more”), and the residence type (urban or rural). This was followed by three questions about the nature of their brain injury: type of brain injury, location of brain injury and onset of acquired brain injury.
Subjective navigation assessment
Subjective navigational ability was assessed using the Wayfinding questionnaire (Claessen et al., Citation2016). The Wayfinding questionnaire consists of 22 items that are rated on a 7-point Likert scale. The Wayfinding questionnaire contains 3 sub-scales of navigation ability: navigation & orientation (11 items), distance estimation (3 items) and spatial anxiety (8 items). Cut-off values for the three sub-scales have been determined to indicate an impaired score (navigation & orientation =< 32, distance estimation =< 6, spatial anxiety =>44).
Objective navigation assessment
The objective navigation assessment was identical to the experimental design described in van der Ham et al. (Citation2020). Participants watched a 69-s movie in which a virtual environment was explored. In the video, a path through a fictitious forest was traversed from a first-person perspective on normal walking speed. The environment consisted out of 8 intersection points (5 two-way intersections, 3 three-way intersections). The stroke of land alongside the path was filled with vegetation and dunes, making it impossible to see previous and upcoming components of the path. Along the path, participants would encounter 8 distinct landmarks (oil barrels, spaceship, science fiction crate, rowboat, car, container, buoy and a formation of crystals) ().
Figure 1. A screenshot of the navigation task indicating one of the eight landmarks in the environment.
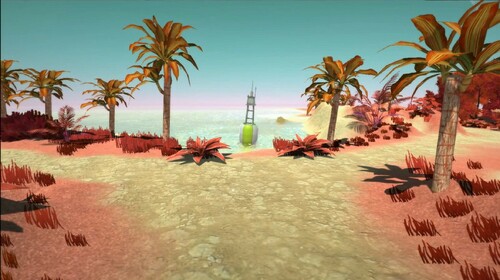
Following the demonstration route, participants completed five navigation tasks, each assessing a component of navigational ability: landmark recognition, allocentric location knowledge, egocentric location knowledge, route-based path knowledge, survey-based path knowledge.
In the landmark recognition task, participants were presented with eight images of landmarks and had to indicate whether the landmark was encountered along the route. Half of the landmarks that were shown to participants were not present in the environment. In the allocentric location task, participants were presented with a map of the environment. Participants were shown a landmark and had to indicate where on the map (location A, B, C or D) the landmark was encountered. In the egocentric location task, participants were presented with an image of an intersection point (including the landmark) shown from a first-person perspective. Six arrows were shown pointing to different directions with an interval of 60 degrees. Participants had to select which arrow pointed towards the ending location of the route. In the route-based path knowledge task, participant were shown an image of an intersection point (including the landmark), depicted from a first-person perspective. Participant had to indicate the direction of the route at each intersection point (left, right or straight). In the survey-based path knowledge task, participants were shown three landmarks. Participants had to indicate which two landmarks were closest to each other (beeline). A total score of 8 could be obtained in the landmark recognition tasks. In all other tasks, a score of 4 could be obtained. Scores were calculated separately for each of the five navigation tasks.
Procedure
Participants visited the website to partake in the experiment. First, an information letter and consent form was presented. Participants gave consent to participating in the experiment by checking a box indicating that they read the information, and a box stating that they agreed to participate. All data was gathered anonymously.
The experiment started with the general questionnaire, followed by the objective navigation assessment. During the objective navigation test, participants watched the video and completed the landmark recognition task. The order of the remaining four navigation tasks was randomized. The objective navigation assessment was followed by the Wayfinding questionnaire. After completing this part, participants received feedback on the objective navigation score in the form of a graph, indicating their performance on the landmark task, relative to the route knowledge path + egocentric location and the survey knowledge path + allocentric location tasks. Additionally, participants received general information about navigation strategy and tips to improve their navigation ability.
Statistics
Matching procedure
In order to investigate the effects of ABI on navigation abilities, objective and subjective measures of navigation ability were compared between the ABI group and a group of healthy controls. The sample size and demographics of the healthy participants (n = 7474) and ABI patients (n = 435) varied considerably (). To account for the differences between the two samples, a propensity score matching procedure was conducted using the “IBM SPSS Statistics Essentials for R” package and “SPSS PS Matching” plugin (Thoemmes, Citation2012). Propensity score matching allowed us to match individuals from the healthy control sample to the patients sample on the basis of a set of selected covariates, whilst minimizing selection bias.
Table 1. Demographics of ABI patients and sample of pre and post-matched healthy controls.
Before starting the matching procedure, the sample of healthy participants was trimmed based on participant age. The minimal age required for participation in the ABI population was 16, while the sample of healthy participants included participants younger than 16. As such, healthy participants younger than 16 were not included the analysis.
A propensity score was calculated by performing a logistic regression using a set of matching variables as predictors. The matching covariates used were age, gender, education, residence type and spatial experience.
Age and gender were included as matching variables as these variables are well known individual factors influencing navigation ability (Castelli et al., Citation2008; Grön et al., Citation2000; Moffat et al., Citation2001). Education level has been included as matching variable as there was a clear discrepancy between education levels in the healthy sample compared to the sample with ABI patients. Residence type has been included as matching variable as there are differences in navigation abilities and strategies that can be employed in dense urban environments compared to open rural environments (Juliani et al., Citation2016). For example, global landmarks might be more present in rural environments whereas navigation in dense urban towns might favour the use of local landmarks (Steck & Mallot, Citation2000). Spatial experience, measured by how often people visit novel environments, was included as matching variables in order to account for ABI patients that do not venture outside their residence too often (Logan et al., Citation2004; Qiu et al., Citation2010)
The propensity scores of healthy participants and ABI patients were matched using a 1-to-1 nearest neighbour matching algorithm without replacement. To limit inaccurate matching, a calliper with a width equal to 0.2 of the standard deviation of the logit of the propensity score was used (Austin, Citation2011b). The resulting matches were assessed for overall imbalance using Hansen and Bowers (Citation2008) imbalance test and the relative multivariate imbalance L1 test (Iacus et al., Citation2009).
Subjective navigational ability
Since healthy participants and ABI patients were matched using the propensity score matching procedure, the data was treated as paired for the main effects analysis. A repeated measures MANOVA analysis was performed with the scores on the three scales of the Wayfinding Questionnaire (navigation & orientation, distance estimation and spatial anxiety) as dependent variables and group (healthy vs. ABI) as within subject factor. The proportion of participants that reported impaired levels of subjective navigation ability on the Wayfinding questionnaire were calculated following the cut-off values described in Claessen et al. (Citation2016). The proportions of impaired individuals in the ABI group and the healthy controls were compared using the McNemar test.
To investigate the effect of ABI type and ABI location on self-reported navigation impairments, a (non-paired) MANOVA was conducted with the scores on the three scales of the Wayfinding Questionnaire (navigation & orientation, distance estimation and spatial anxiety) as dependent variables and ABI type as within-subject factor. Gender, education and age were included as covariates. The effect of ABI location was investigated using a sub-set of participants with ABI types that typically concern localized lesions: stroke, brain tumour, epilepsy. Only participants that knew the location of their lesion were included in this analysis. A (non-paired) MANOVA was performed with the scores of the WQ as depend variables and ABI location (left, right and bilateral) as between subject factor. Specifically, in a post-hoc analysis, performance differences between patients with left vs. right hemispherical damage were assessed.
Objective navigation ability
Since healthy participants and ABI patients were matched using the propensity score matching procedure, the data was treated as paired for the main effects analysis. To assess the differences in objective navigation ability between healthy participants and ABI patients, a repeated measures MANOVA analysis was performed with the 5 navigation tasks (landmark recognition, egocentric location knowledge, allocentric location knowledge, route-based path knowledge and survey-based path knowledge) as dependent variables and group (healthy vs. ABI) as within subject factor.
To assess the effect of ABI type and location on navigation ability, a (non-paired) MANCOVA was performed with the 5 navigation tasks scores as depend variables and ABI type as between subject factor and gender, age and education as covariates. To investigate an effect of ABI location, a sample of ABI participants with localized damage (stroke, brain tumour and epilepsy) was selected. Participants who did not know the location of their ABI were excluded from this analysis. A (non-paired) MANCOVA was performed with the 5 navigation task scores as dependent variables, ABI location as between subject factor and age, gender and education as covariates. Specifically, in a post-hoc analysis, performance differences between patients with left vs. right hemispherical damage was assessed.
Results
Participants
A total of 485 ABI patients completed both the Wayfinding questionnaire and all 5 navigation tasks. Out of this sample, 50 participants were excluded from analysis because they reported neurological, congenital, psychiatric or otherwise unclear medical conditions instead of ABIs (e.g., ADHD, Alzheimer’s disease, focal cortical dysplasia, tremors). One participant was excluded as the reported gender was unclear. In total, 435 participants with ABI were included in the analysis (). A variety of ABI types were reported by the ABI patients (). The largest proportion of ABI patients in this sample had experienced a stroke (45.1%) or traumatic brain injury (23.0%). Other types of ABI were reported less frequently (<10%). The locations of brain injuries were equally divided between the left and right hemispheres, although roughly a third of the ABI patients were unable to report the location of the lesion (37.7%). Most of the ABI patients in this sample were in the chronic stage of brain injury (86.9%), as the onset time of the injury was more than 12 months ago. The sample of healthy controls that completed the 5 navigation tasks, the Wayfinding questionnaire and were 16 years or older, consisted of 7474 participants ().
Table 2. Overview of subjective navigation impairments within subcategories of the ABI population.
Propensity score matching
Prior to the matching procedure, MANOVA analysis revealed significant differences (F (3, 7905) = 34.39; p < .001; η2 = .013) between the ABI and control group for the variables age (F (1, 7907) = 16.99; p < .001; η2 = .002), education (F (1, 7907) = 85.79; p < .001; η2 = .011), and spatial experience (F (1, 7907) = 27.27; p < .001; η2 = .003). Chi-squared tests show significant differences in gender (χ2 (1) = 7.66, p = .006) and residence type (χ2 (1) = 9.39, p = .002) between the two samples. Using propensity score matching, 435 healthy controls were matched to the ABI patient sample. Post matching balance checks revealed an increase in overall balance. Standardized mean differences (Cohen’s d) was lower than 0.1 after matching, indicating that none of the covariates exhibited a large unbalance after matching (Austin, Citation2011a). This was confirmed by subsequent balance assessments: the overall χ2 balance test was not significant, χ2 (5) = 3.08, p = .69, the relative multivariate imbalance L1 was larger in the unmatched sample (.46) than in the matched sample (.45). After the propensity score matching procedure, the ABI sample and the matched healthy controls were comparable in terms of age, education, gender, spatial experience, and residence type ().
Subjective navigation impairments
Overall, 39.1% of the ABI participants were impaired on any of the subscales of the Wayfinding questionnaire, compared to 19.3% in the control group. Proportional analysis using the McNemar tests revealed a significantly higher percentage of self-reported impairments in the ABI compared to the control group for the navigation & orientation (19.1% vs. 8.7%, p < .001), distance estimation (21.1% vs. 11.3%, p < .001) and spatial anxiety subscales (21.4% vs. 7.1%, p < .001).
Analysis of subscale scores on the Wayfinding Questionnaire using a paired MANOVA (repeated measures) revealed a main effect of group (control vs. ABI) on self-reported navigation ability (F (3, 432) = 24.11; p < 0.001; = .14). Univariate tests indicated a significant effect of group on navigation & orientation (F (1, 434) = 42.35; p < 0.001;
= .09), distance estimation (F (1, 434) = 25.63; p < 0.001; η2 = .06) and spatial anxiety (F (1, 434) = 65.36; p < 0.001;
= .13) (). Post-hoc paired t-test showed that the control group scored significantly higher on navigation & orientation (p < .001) and distance estimation (p < .001) (higher scores on these scales referred to higher self-reported spatial ability), whereas the ABI group scored higher on the spatial anxiety subscale (p < .001) (higher score referred to higher levels of spatial anxiety).
Figure 2. Scores on the subscales of the Wayfinding questionnaire. Error bars represent standard error of the mean. * Indicates a significant difference between the control and ABI populations.
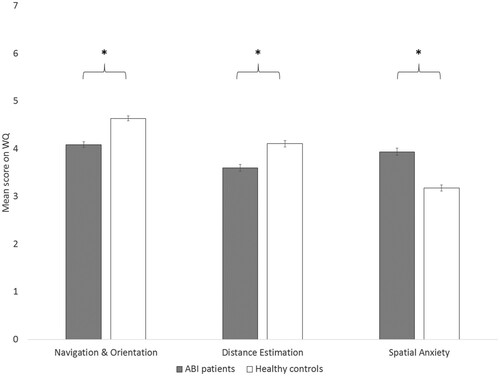
Exploratory analyses were conducted to determine whether there was an effect of type and location of ABI on self-reported navigation abilities. A MANOVA revealed a main effect of ABI type (F (27, 2571) = 3.931; p < 0.001; = 0.40) on subjective navigation performance. Post-hoc pairwise comparison revealed that compared to the control group, stroke and TBI scored significantly lower on navigation & orientation and distance estimation subscales. Patients with stroke, TBI, hypoxia and intoxication scored significantly higher on spatial anxiety compared to the control group. No effect of ABI location was found on subjective navigation abilities.
Objective navigation impairments
A paired MANOVA (repeated measures) revealed a main effect of group (control vs. ABI) on objective navigation ability (F (5, 430) = 2.53; p = 0.029; = .03). Univariate tests showed a significant effect of group on performance on the landmark recognition task (F (1, 434) = 4.33; p = 0.038;
= .01), allocentric location knowledge task (F (1, 434) = 4.41; p = 0.036;
= .01) and route-based path knowledge task (F (1, 434) = 7.35; p = 0.007;
= .01). Post-hoc analysis showed that ABI patient scored significantly lower on landmark recognition, allocentric location knowledge and route-based path knowledge compared to the control group ().
Table 3. Comparison of objective navigation impairment between the control and ABI population.
Exploratory analyses were performed to assess the effect of ABI type and ABI location on objective navigation ability (). While the group analysis revealed a differences between the control and ABI group, no specific effects of ABI type were found (F (45, 4285) = 1.166, p = .209, = .012). The analysis of ABI location demonstrated a trend-level effect of ABI location on objective navigation performance (F (15, 1836) = 1.589, p = .069,
= .013). Further investigation of this trend suggested that this effect was specifically present in the landmark recognition task (F (3, 621) = 4.2, p = .01,
= .018), in which patients with right hemisphere ABIs scored significantly lower compared to controls (p = 0.01) and patients with left hemisphere lesion (p = .029).
Table 4. Overview of objective navigation score for the 5 subtasks per brain injury type, onset time and location of brain injury.
Discussion
Little is known about the prevalence of navigation impairments among patients with ABI. The aim of this study was to provide an overview of navigation impairments in this population to inform rehabilitation specialist about prevalence and characteristics of navigation problems. Results can help clinicians to make informed decision about whether or not to adopt navigation ability assessment in clinical intake procedures.
The first goal of this study was to determine the occurrence of subjective and objective navigation impairments in ABI patients compared to a group of healthy participants that were matched in terms of gender, age, education, resident type (urban or rural) and spatial experience. Compared to the control group, ABI patients scored significantly lower on self-reported navigation ability and objective navigation ability. ABI patients are 2.03 times more likely to report subjective navigation impairments compared to healthy participants. In the current sample, 39.1% of the patients had an impaired score on at least one scale of the Wayfinding questionnaire (compared to 19.3% in the control group). The percentage of self-reported navigation impairments is substantially higher than the proportion found in previous research with solely mild stroke patients (29%) (van der Ham et al., Citation2013). We expect that the higher level of impairments found in the current study is the result of the relatively loose inclusion criteria for ABI patients. In the current study, all ABI patients were allowed to participate whereas van der Ham et al. (Citation2013) included only patients that scored high on independent living indexes. The self-reported impairments were reflected in the lowered performance on the objective navigation assessments. The increased impairment levels are not as high (31% to 86%, depending on the location of the lesion), as reported earlier studies that included relatively large samples of ABI patients (Barrash et al., Citation2000). Barrash et al. (Citation2000) investigated route learning in a real environment, using an 8 min route and used 3 consecutive trials to assess route knowledge. In contrast, the current study was concerned with a more general assessment of navigation ability spanning over 5 domains. Furthermore, this study consisted of a shorter route (69 s) and did not include repetition of tasks. As such, we suspect that the proportion of impaired ABI patients in the current study reflects a conservative number.
The second goal of the study was to explore what domain of navigation ability (landmark, location or path) was most vulnerable to acquired brain injury (Claessen & van der Ham, Citation2017). The results show that ABI patients scored lower on the landmark recognition task, allocentric location task and the route-based path knowledge task compared to the control group.
The ability to encode and recognize landmarks can be regarded as one of the most fundamental components of navigation ability. Landmarks serve as beacons, associative and directional cues (Chan et al., Citation2012). As such, other components of navigation ability, location and path, partially rely on intact landmark memory. Because of its importance to navigation, the human brain is highly effective at detecting and encoding objects at key decision points in the environment to the point that this occurs independent of attention to the object (Janzen & Van Turennout, Citation2004). As such, impairments in the ability to recognize landmarks can have detrimental effects on navigation. It should be noted that the landmark recognition task used in the current paradigm only reflects one component of landmark knowledge: the encoding and recall of novel landmarks. Different subcategories of landmark impairments, such as the ability to recognize familiar and famous landmarks or the ability to recognize scenes rather than specific objects, were not assessed in this task.
ABI patients scored lower on the route-based path knowledge task. Here, we assessed the ability to remember what direction to take when standing at an intersection point, in order to replicate a route. Route continuation ability allows navigators to form an understanding of paths between important locations in an environment. Route continuation is a prominent navigation ability that arises early during development and is relatively well preserved with regard to aging (Nys et al., Citation2018; Wiener et al., Citation2019). While route continuation appears to be a stable and enduring ability, the current study shows it is vulnerable to acquired brain injury. This result supports earlier findings on the vulnerability of route learning after ABI (Barrash et al., Citation2000).
Lower scores on the allocentric location task show that ABI patients in general have more difficulty remembering where landmarks were located when presented with a map. This task required participants to convert knowledge obtained egocentrically, to an allocentric reference frame. It is well known that the switch between perspectives is difficult. Furthermore, increased difficulty with perspective switching is observed after aging and in patients with neurodegenerative diseases such as Alzheimer’s disease and patients with mild cognitive impairments (Colombo et al., Citation2017). These difficulties are also observed in the ABI population.
Lastly, we assessed whether the type of ABI and location (hemisphere) of the injury would affect the occurrence of objective and subjective navigation impairments. Earlier research has established that subjective navigation impairments are often reported by stroke patients (van der Ham et al., Citation2013). Importantly, our results show that navigation impairments are not limited to stroke patients, but are also reported by people with traumatic brain injury, intoxication and hypoxia. No effect of hemispherical location of the brain injury on subjective navigation impairments was found.
Furthermore, no effect of ABI type was found on the objective navigation impairments. However, a trend effect of brain injury location was observed. ABI patients with right hemispherical damage scored lower on the landmark recognition task compared to the controls and patients with left hemispherical damage. This result is in line with a wealth of fMRI and lesion studies that have shown that networks in the right hemisphere are of particular importance for navigation and spatial memory (Ekstrom et al., Citation2003; Gramann et al., Citation2006; Iaria et al., Citation2007; Jacobs et al., Citation2010; Maguire et al., Citation1997).
Of note are the relatively large differences between ABI patients and controls on the mean scores of the subjective (self-reported) task and the small differences between the means of the objective navigation scores. This discrepancy suggests a limited selectivity of the objective assessment. This can in part be explained by the design of the study, which was constrained to be short and accessible online, rather than a complete diagnostic assessment (Claessen et al., Citation2017). A more thorough assessment of each domain is likely to raise the selectivity to impairments in each task. However, the discrepancy between self-reported and objectively measured cognitive problems is a well-known phenomenon in neuropsychological assessments of ABI patients. Many studies fail to find a clear relation between self-reported cognitive complaints and objective performance (Aben et al., Citation2011; Duits et al., Citation2008; Lamb et al., Citation2013; Spencer et al., Citation2010; Stulemeijer et al., Citation2007; Winkens et al., Citation2009). Cognitive problems experienced in daily life are often influenced by subtle factors such as fatigue, reduced mental effort capacity and personal factors (e.g., emotional functioning), that are often not registered by neuropsychological assessments (Borgaro et al., Citation2005; Riese, Citation1999).
Overall, our results show that almost half of the patients with ABI reported navigation impairments. Subjective impairments occur roughly two times more often in the ABI population. Furthermore, navigation impairments are prominent in all types of ABI and can be observed in patients with left, right and bilateral brain injuries. Specific components of navigation ability, landmark recognition, route continuation and allocentric location knowledge are most vulnerable to brain injury. Therefore, we strongly recommend that screening and treatment of navigation impairments are included in clinical practice guidelines at rehabilitation treatment centres. Awareness and recognition of patient’s daily navigation problems is an important step in starting potential treatment. We encourage healthcare professionals to discuss potential difficulties in spatial navigation a patient might experience following brain injury. Practitioners could ask about a patient’s ability to remember landmarks, describe a route or ask patients to use a map. In case of complaints, a patient can be asked to fill in the Wayfinding questionnaire to determine the presence of subjective navigation complains. Finally, the domain of navigation impairment should be assessed. The navigation test used here provides a suitable solution for a standardized diagnostic tool to provide an objective measure of such complaints, as a specific reference group can be constructed from the large control group.
While large-scale online assessments have many advantages, several important limitations should be noted. First, participants performed the experiment unsupervised. This will have introduced a level of uncertainty and noise in the dataset. For example, participants might not have understood all questions or might have had a bad internet connection. Second, because this study was part of a public science event, an open web link to the experiment was used rather than a unique personalized code that could be traced back to an individual. It was therefore impossible to verify the characteristics of the participants in this study. Consequently, we did not obtain medical records, nor were we able to inspect any underlying cognitive processes that might have contributed to the reported navigation problems. As such, we relied on self-identification of acquired brain injury. While a large proportion of patients were able to provide detailed information on their brain injury (94%), this could not be validated. Third, the online availability of the study might have attracted a population of participants that are not necessarily representative of the ABI population as a whole. Patients who experienced difficulties during navigation might have been more inclined to participate in the experiment. As such, the level of self-reported impairments might have been slightly biased towards higher levels of navigation impairments. Additionally, seeking out and performing this online study requires a certain degree of cognitive functioning, posing a potential bias towards ABI patients with sufficiently intact cognitive abilities. However, it is likely that the impact of this bias is diminished by the large number of participants in this study. We also identified limitation of a more methodological nature, as the experiment was short and broad (focussing on a variety of domains). Most components of the objective navigation assessment contained only 4 multiple choice questions. This allowed us to investigate the general effect of ABI types on all components of navigation in a single test. The disadvantage of this approach was that the differences between groups are only apparent on group levels, as no cut-off threshold for task specific impairment levels could be formulated. Lastly, the current navigation task utilized a video from which a route was learned instead of real-world route that was traversed. Several studies show that movement information (e.g., vestibular and proprioceptive signals) obtained during real-world navigation contributes to the formation of spatial knowledge. Loss of movement information negatively affects egocentric navigation, the formation of survey knowledge and knowledge about route order (Chrastil & Warren, Citation2013, Citation2015; Sorita et al., Citation2013; Xie et al., Citation2017). As such, the current assessment of navigation ability is likely to underestimate the performance of participants when compared to real-life navigation. However, as the purpose of the study was to compare the performance of ABI patients to healthy controls, this limitation is not likely to effect the current results. It should be noted that this approach has advantages: the environment is novel to all participants, disrupting factors are removed (e.g., traffic, whether conditions) and exposure of the environment is kept constant amongst participants. Furthermore, there are studies that suggest that navigation performance in real and virtual environments is highly comparable (Lloyd et al., Citation2009; Richardson et al., Citation1999) and that transfer of information between real and virtual environments is possible (Péruch et al., Citation2000).
When placing all results in context, we conclude that navigation impairments are common amongst patients with all types of acquired brain injuries. Neurologists, rehabilitation specialists, neuropsychologists, occupational therapists, and general practitioners are encouraged to ask all ABI patients about potential changes in their navigation abilities after the incident. Especially participants with right hemispherical damage should be inspected. Patients with self-reported navigation impairments should be referred to neuropsychologists for further diagnosis of the type of impairment and develop suitable treatments for these patients.
Author contributions
Conceptualization: MvdK, AE, AVM & IvdH; Methodology: MvdK & IvdH; Formal analysis and investigation: MvdK; Writing original draft preparation MvdK, AE, AVM & IvdH; Funding acquisition: IvdH & AE; Supervision: AE, AVM & IvdH.
Disclosure statement
The authors declared that they had no conflicts of interest with respect to their authorship or the publication of this article.
References
- Aben, L., Ponds, R. W., Heijenbrok-Kal, M. H., Visser, M. M., Busschbach, J. J., & Ribbers, G. M. (2011). Memory complaints in chronic stroke patients are predicted by memory self-efficacy rather than memory capacity. Cerebrovascular Diseases, 31(6), 566–572. https://doi.org/10.1159/000324627
- Austin, P. C. (2011a). An introduction to propensity score methods for reducing the effects of confounding in observational studies. Multivariate Behavioral Research, 46(3), 399–424. https://doi.org/10.1080/00273171.2011.568786
- Austin, P. C. (2011b). Optimal caliper widths for propensity-score matching when estimating differences in means and differences in proportions in observational studies. Pharmaceutical Statistics, 10(2), 150–161. https://doi.org/10.1002/pst.433
- Barrash, J., Damasio, H., Adolphs, R., & Tranel, D. (2000). The neuroanatomical correlates of route learning impairment. Neuropsychologia, 38(6), 820–836. https://doi.org/10.1016/S0028-3932(99)00131-1
- Blajenkova, O., Motes, M. A., & Kozhevnikov, M. (2005). Individual differences in the representations of novel environments. Journal of Environmental Psychology, 25(1), 97–109. https://doi.org/10.1016/j.jenvp.2004.12.003
- Boccia, M., Nemmi, F., & Guariglia, C. (2014). Neuropsychology of environmental navigation in humans: Review and meta-analysis of FMRI studies in healthy participants. Neuropsychology Review, 24(2), 236–251. https://doi.org/10.1007/s11065-014-9247-8
- Borgaro, S. R., Baker, J., Wethe, J. V., Prigatano, G. P., & Kwasnica, C. (2005). Subjective reports of fatigue during early recovery from traumatic brain injury. The Journal of Head Trauma Rehabilitation, 20(5), 416–425. https://doi.org/10.1097/00001199-200509000-00003
- Brown, T. I., Hasselmo, M. E., & Stern, C. E. (2014). A high-resolution study of hippocampal and medial temporal lobe correlates of spatial context and prospective overlapping route memory. Hippocampus, 24(7), 819–839. https://doi.org/10.1002/hipo.22273
- Bullens, J., Iglói, K., Berthoz, A., Postma, A., & Rondi-Reig, L. (2010). Developmental time course of the acquisition of sequential egocentric and allocentric navigation strategies. Journal of Experimental Child Psychology, 107(3), 337–350. https://doi.org/10.1016/j.jecp.2010.05.010
- Burgess, N. (2006). Spatial memory: How egocentric and allocentric combine. Trends in Cognitive Sciences, 10(12), 551–557. https://doi.org/10.1016/j.tics.2006.10.005
- Castelli, L., Corazzini, L. L., & Geminiani, G. C. (2008). Spatial navigation in large-scale virtual environments: Gender differences in survey tasks. Computers in Human Behavior, 24(4), 1643–1667. https://doi.org/10.1016/j.chb.2007.06.005
- Cattelani, R., Zettin, M., & Zoccolotti, P. (2010). Rehabilitation treatments for adults with behavioral and psychosocial disorders following acquired brain injury: A systematic review. Neuropsychology Review, 20(1), 52–85. https://doi.org/10.1007/s11065-009-9125-y
- Chan, E., Baumann, O., Bellgrove, M., & Mattingley, J. (2012). From objects to landmarks: The function of visual location information in spatial navigation. Frontiers in Psychology, 3(304), https://doi.org/10.3389/fpsyg.2012.00304
- Chrastil, E. R., & Warren, W. H. (2013). Active and passive spatial learning in human navigation: Acquisition of survey knowledge. Journal of Experimental Psychology: Learning, Memory, and Cognition, 39(5), 1520. https://doi.org/10.1037/a0032382
- Chrastil, E. R., & Warren, W. H. (2015). Active and passive spatial learning in human navigation: Acquisition of graph knowledge. Journal of Experimental Psychology: Learning, Memory, and Cognition, 41(4), 1162. https://doi.org/10.1037/xlm0000082
- Claessen, M. H., & van der Ham, I. J. (2017). Classification of navigation impairment: A systematic review of neuropsychological case studies. Neuroscience & Biobehavioral Reviews, 73, 81–97. https://doi.org/10.1016/j.neubiorev.2016.12.015
- Claessen, M. H., Visser-Meily, J. M., de Rooij, N. K., Postma, A., & van der Ham, I. J. (2016). The wayfinding questionnaire as a self-report screening instrument for navigation-related complaints after stroke: Internal validity in healthy respondents and chronic mild stroke patients. Archives of Clinical Neuropsychology, 31(8), 839–854. https://doi.org/10.1093/arclin/acw044
- Claessen, M. H., Visser-Meily, J. M., Meilinger, T., Postma, A., de Rooij, N. K., & van der Ham, I. J. (2017). A systematic investigation of navigation impairment in chronic stroke patients: Evidence for three distinct types. Neuropsychologia, 103, 154–161. https://doi.org/10.1016/j.neuropsychologia.2017.07.001
- Colombo, D., Serino, S., Tuena, C., Pedroli, E., Dakanalis, A., Cipresso, P., & Riva, G. (2017). Egocentric and allocentric spatial reference frames in aging: A systematic review. Neuroscience & Biobehavioral Reviews, 80, 605–621. https://doi.org/10.1016/j.neubiorev.2017.07.012
- Duits, A., Munnecom, T., van Heugten, C., & van Oostenbrugge, R. J. (2008). Cognitive complaints in the early phase after stroke are not indicative of cognitive impairment. Journal of Neurology, Neurosurgery & Psychiatry, 79(2), 143–146. https://doi.org/10.1136/jnnp.2007.114595
- Ekstrom, A. D., Kahana, M. J., Caplan, J. B., Fields, T. A., Isham, E. A., Newman, E. L., & Fried, I. (2003). Cellular networks underlying human spatial navigation. Nature, 425(6954), 184–188. https://doi.org/10.1038/nature01964
- Epstein, R. A. (2008). Parahippocampal and retrosplenial contributions to human spatial navigation. Trends in Cognitive Sciences, 12(10), 388–396. https://doi.org/10.1016/j.tics.2008.07.004
- Epstein, R., DeYoe, E. A., Press, D. Z., Rosen, A. C., & Kanwisher, N. (2001). Neuropsychological evidence for a topographical learning mechanism in parahippocampal cortex. Cognitive Neuropsychology, 18(6), 481–508. https://doi.org/10.1080/02643290125929
- Gramann, K., Müller, H. J., Schönebeck, B., & Debus, G. (2006). The neural basis of ego- and allocentric reference frames in spatial navigation: Evidence from spatio-temporal coupled current density reconstruction. Brain Research, 1118(1), 116–129. https://doi.org/10.1016/j.brainres.2006.08.005
- Grön, G., Wunderlich, A. P., Spitzer, M., Tomczak, R., & Riepe, M. W. (2000). Brain activation during human navigation: Gender-different neural networks as substrate of performance. Nature Neuroscience, 3(4), 404. https://doi.org/10.1038/73980
- Häggström, A., & Lund, M. L. (2008). The complexity of participation in daily life: A qualitative study of the experiences of persons with acquired brain injury. Journal of Rehabilitation Medicine, 40(2), 89–95. https://doi.org/10.2340/16501977-0138
- Hansen, B. B., & Bowers, J. (2008). Covariate balance in simple, stratified and clustered comparative studies. Statistical Science, 23(2), 219–236. https://doi.org/10.1214/08-STS254
- Herdman, K. A., Calarco, N., Moscovitch, M., Hirshhorn, M., & Rosenbaum, R. S. (2015). Impoverished descriptions of familiar routes in three cases of hippocampal/medial temporal lobe amnesia. Cortex, 71, 248–263. https://doi.org/10.1016/j.cortex.2015.06.008
- Hirayama, K., Taguchi, Y., Sato, M., & Tsukamoto, T. (2003). Limbic encephalitis presenting with topographical disorientation and amnesia. Journal of Neurology, Neurosurgery & Psychiatry, 74(1), 110–112. https://doi.org/10.1136/jnnp.74.1.110
- Iacus, S. M., King, G., & Porro, G. (2009). CEM: software for coarsened exact matching.
- Iaria, G., Chen, J., Guariglia, C., Ptito, A., & Petrides, M. (2007). Retrosplenial and hippocampal brain regions in human navigation: Complementary functional contributions to the formation and use of cognitive maps. European Journal of Neuroscience, 25(3), 890–899. https://doi.org/10.1111/j.1460-9568.2007.05371.x
- Iglói, K., Zaoui, M., Berthoz, A., & Rondi-Reig, L. (2009). Sequential egocentric strategy is acquired as early as allocentric strategy: Parallel acquisition of these two navigation strategies. Hippocampus, 19(12), 1199–1211. https://doi.org/10.1002/hipo.20595
- Ishikawa, T., & Montello, D. R. (2006). Spatial knowledge acquisition from direct experience in the environment: Individual differences in the development of metric knowledge and the integration of separately learned places. Cognitive Psychology, 52(2), 93–129. https://doi.org/10.1016/j.cogpsych.2005.08.003
- Jacobs, J., Korolev, I. O., Caplan, J. B., Ekstrom, A. D., Litt, B., Baltuch, G., Fried, I., Schulze-Bonhage, A., Madsen, J. R., & Kahana, M. J. (2010). Right-lateralized brain oscillations in human spatial navigation. Journal of Cognitive Neuroscience, 22(5), 824–836. https://doi.org/10.1162/jocn.2009.21240
- Janzen, G., & Jansen, C. (2010). A neural wayfinding mechanism adjusts for ambiguous landmark information. Neuroimage, 52(1), 364–370. https://doi.org/10.1016/j.neuroimage.2010.03.083
- Janzen, G., & Van Turennout, M. (2004). Selective neural representation of objects relevant for navigation. Nature Neuroscience, 7(6), 673. https://doi.org/10.1038/nn1257
- Johnson, K., & Davis, P. K. (1998). A supported relationships intervention to increase the social integration of persons with traumatic brain injuries. Behavior Modification, 22(4), 502–528. https://doi.org/10.1177/01454455980224004
- Juliani, A. W., Bies, A. J., Boydston, C. R., Taylor, R. P., & Sereno, M. E. (2016). Navigation performance in virtual environments varies with fractal dimension of landscape. Journal of Environmental Psychology, 47, 155–165. https://doi.org/10.1016/j.jenvp.2016.05.011
- Klatzky, R. L. (1998). Allocentric and egocentric spatial representations: Definitions, distinctions, and interconnections. In C. Freksa, C. Habel, & K. Wender (Eds.), Spatial cognition (pp. 1–17). Springer. https://doi.org/10.1007/3-540-69342-4_1
- Lamb, F., Anderson, J., Saling, M., & Dewey, H. (2013). Predictors of subjective cognitive complaint in postacute older adult stroke patients. Archives of Physical Medicine and Rehabilitation, 94(9), 1747–1752. https://doi.org/10.1016/j.apmr.2013.02.026
- Landis, T., Cummings, J. L., Benson, D. F., & Palmer, E. P. (1986). Loss of topographic familiarity: An environmental agnosia. Archives of Neurology, 43(2), 132–136. https://doi.org/10.1001/archneur.1986.00520020026011
- Lloyd, J., Persaud, N. V., & Powell, T. E. (2009). Equivalence of real-world and virtual-reality route learning: A pilot study. Cyberpsychology & Behavior, 12(4), 423–427. https://doi.org/10.1089/cpb.2008.0326
- Logan, P. A., Gladman, J. R. F., Avery, A., Walker, M. F., Dyas, J., & Groom, L. (2004). Randomised controlled trial of an occupational therapy intervention to increase outdoor mobility after stroke. BMJ (Clinical Research ed.), 329(7479), 1372–1375. https://doi.org/10.1136/bmj.38264.679560.8F
- Maguire, E. A., Frackowiak, R. S., & Frith, C. D. (1997). Recalling routes around London: Activation of the right hippocampus in taxi drivers. Journal of Neuroscience, 17(18), 7103–7110. https://doi.org/10.1523/JNEUROSCI.17-18-07103.1997
- Maguire, E. A., Nannery, R., & Spiers, H. J. (2006). Navigation around London by a taxi driver with bilateral hippocampal lesions. Brain, 129(11), 2894–2907. https://doi.org/10.1093/brain/awl286
- Mendez, M. F., & Cherrier, M. M. (2003). Agnosia for scenes in topographagnosia. Neuropsychologia, 41(10), 1387–1395. https://doi.org/10.1016/S0028-3932(03)00041-1
- Milders, M., Fuchs, S., & Crawford, J. R. (2003). Neuropsychological impairments and changes in emotional and social behaviour following severe traumatic brain injury. Journal of Clinical and Experimental Neuropsychology, 25(2), 157–172. https://doi.org/10.1076/jcen.25.2.157.13642
- Moffat, S. D., Zonderman, A. B., & Resnick, S. M. (2001). Age differences in spatial memory in a virtual environment navigation task. Neurobiology of Aging, 22(5), 787–796. https://doi.org/10.1016/S0197-4580(01)00251-2
- Nasreddine, Z. S., Phillips, N. A., Bédirian, V., Charbonneau, S., Whitehead, V., Collin, I., Cummings, J. L., & Chertkow, H. (2005). The Montreal Cognitive Assessment, MoCA: A brief screening tool for mild cognitive impairment. Journal of the American Geriatrics Society, 53(4), 695–699. https://doi.org/10.1111/j.1532-5415.2005.53221.x
- Nys, M., Hickmann, M., & Gyselinck, V. (2018). The role of verbal and visuo-spatial working memory in the encoding of virtual routes by children and adults. Journal of Cognitive Psychology, 30(7), 710–727. https://doi.org/10.1080/20445911.2018.1523175
- Péruch, P., Belingard, L., & Thinus-Blanc, C. (2000). Transfer of spatial knowledge from virtual to real environments. In C. Freksa, C. Bauer, C. Habel, & K. Wender (Eds.), Spatial Cognition II, LNAI 1849 (pp. 253–264). Springer. https://doi.org/10.1007/3-540-45460-8_19
- Qiu, W. Q., Dean, M., Liu, T., George, L., Gann, M., Cohen, J., & Bruce, M. L. (2010). Physical and mental health of homebound older adults: An overlooked population. Journal of the American Geriatrics Society, 58(12), 2423–2428. https://doi.org/10.1111/j.1532-5415.2010.03161.x
- Rees, L., Marshall, S., Hartridge, C., Mackie, D., & Weiser, M. (2007). Cognitive interventions post acquired brain injury. Brain Injury, 21(2), 161–200. https://doi.org/10.1080/02699050701201813
- Richardson, A. E., Montello, D. R., & Hegarty, M. (1999). Spatial knowledge acquisition from maps and from navigation in real and virtual environments. Memory & Cognition, 27(4), 741–750. https://doi.org/10.3758/BF03211566
- Riese, H. (1999). Mental fatigue after very severe closed head injury: Sustained performance, mental effort, and distress at two levels of workload in a driving simulator. Neuropsychological Rehabilitation, 9(2), 189–205. https://doi.org/10.1080/713755600
- RIVM. (2016). Overzicht hersenaandoeningen. https://www.volksgezondheidenzorg.info/bestanden/documenten/overzichthersenaandoeningendefinitiefxlsx
- Rosenbaum, R. S., Priselac, S., Köhler, S., Black, S. E., Gao, F., Nadel, L., & Moscovitch, M. (2000). Remote spatial memory in an amnesic person with extensive bilateral hippocampal lesions. Nature Neuroscience, 3(10), 1044–1048. https://doi.org/10.1038/79867
- Schipper, K., Visser-Meily, J. M., Hendrikx, A., & Abma, T. A. (2011). Participation of people with acquired brain injury: Insiders perspectives. Brain Injury, 25(9), 832–843. https://doi.org/10.3109/02699052.2011.589796
- Shelton, A. L., & Gabrieli, J. D. E. (2002). Neural correlates of encoding space from route and survey perspectives. The Journal of Neuroscience, 22(7), 2711–2717. https://doi.org/10.1523/jneurosci.22-07-02711.2002
- Siegel, A. W., & White, S. H. (1975). The development of spatial representations of large-scale environments. In H. W. Reese (Ed.), Advances in Child Development and Behavior (Vol. 10) (pp. 9–55). JAI.
- Sohlberg, M. M., Todis, B., Fickas, S., Hung, P.-F., & Lemoncello, R. (2005). A profile of community navigation in adults with chronic cognitive impairments. Brain Injury, 19(14), 1249–1259. https://doi.org/10.1080/02699050500309510
- Sorita, E., N’Kaoua, B., Larrue, F., Criquillon, J., Simion, A., Sauzéon, H., Joseph, P.-A., & Mazaux, J.-M. (2013). Do patients with traumatic brain injury learn a route in the same way in real and virtual environments? Disability and Rehabilitation, 35(16), 1371–1379. https://doi.org/10.3109/09638288.2012.738761
- Sorrows, M. E., & Hirtle, S. C. (1999, August). The nature of landmarks for real and electronic spaces. In C. Freksa & D. M. Mark (Eds.), Spatial information theory. Cognitive and computational foundations of geographic information science (pp. 37–50). Springer. https://doi.org/10.1007/3-540-48384-5_3
- Spencer, R. J., Drag, L. L., Walker, S. J., & Bieliauskas, L. A. (2010). Self-reported cognitive symptoms following mild traumatic brain injury are poorly associated with neuropsychological performance in OIF/OEF veterans. Journal of Rehabilitation Research & Development, 47(6). https://doi.org/10.1682/JRRD.2009.11.0181
- Steck, S. D., & Mallot, H. A. (2000). The role of global and local landmarks in virtual environment navigation. Presence: Teleoperators & Virtual Environments, 9(1), 69–83. https://doi.org/10.1162/105474600566628
- Stulemeijer, M., Vos, P. E., Bleijenberg, G., & Van der Werf, S. P. (2007). Cognitive complaints after mild traumatic brain injury: Things are not always what they seem. Journal of Psychosomatic Research, 63(6), 637–645. https://doi.org/10.1016/j.jpsychores.2007.06.023
- Takahashi, N., & Kawamura, M. (2002). Pure topographical disorientation—the anatomical basis of landmark agnosia. Cortex, 38(5), 717–725. https://doi.org/10.1016/S0010-9452(08)70039-X
- Thoemmes, F. (2012). Propensity score matching in SPSS. arXiv preprint arXiv:1201.6385.
- Tibæk, M., Kammersgaard, L. P., Johnsen, S. P., Dehlendorff, C., & Forchhammer, H. B. (2018). Long-term return to work after acquired brain injury in young danish adults: A nation-wide registry-based cohort study. Frontiers in Neurology, 9, 1180. https://doi.org/10.3389/fneur.2018.01180
- Turner-Strokes, L. (2003). Rehabilitation following acquired brain injury: national clinical guidelines.
- Turriziani, P., Carlesimo, G. A., Perri, R., Tomaiuolo, F., & Caltagirone, C. (2003). Loss of spatial learning in a patient with topographical disorientation in new environments. Journal of Neurology, Neurosurgery & Psychiatry, 74(1), 61–69. https://doi.org/10.1136/jnnp.74.1.61
- van der Ham, I. J., Claessen, M. H., Evers, A. W., & van der Kuil, M. N. (2020). Large-scale assessment of human navigation ability across the lifespan. Scientific Reports, 10(1), 1–12. https://doi.org/10.1038/s41598-020-60302-0
- van der Ham, I. J., Kant, N., Postma, A., & Visser-Meily, J. (2013). Is navigation ability a problem in mild stroke patients? Insights from self-reported navigation measures. Journal of Rehabilitation Medicine, 45(5), 429–433. https://doi.org/10.2340/16501977-1139
- van der Ham, I. J., van Zandvoort, M. J., Meilinger, T., Bosch, S. E., Kant, N., & Postma, A. (2010). Spatial and temporal aspects of navigation in two neurological patients. Neuroreport, 21(10), 685–689. https://doi.org/10.1097/WNR.0b013e32833aea78
- Van Heugten, C., Rasquin, S., Winkens, I., Beusmans, G., & Verhey, F. (2007). Checklist for cognitive and emotional consequences following stroke (CLCE-24): development, usability and quality of the self-report version. Clinical Neurology and Neurosurgery, 109(3), 257–262. https://doi.org/10.1016/j.clineuro.2006.10.002
- Van Velzen, J. M., Van Bennekom, C. A. M., Edelaar, M. J. A., Sluiter, J. K., & Frings-Dresen, M. H. W. (2009). How many people return to work after acquired brain injury?: A systematic review. Brain Injury, 23(6), 473–488. https://doi.org/10.1080/02699050902970737
- Verhage, F. (1964). Intelligence and age: Study with Dutch people aged 12-77. Van Gorcum.
- Wade, S. L., Bedell, G., King, J. A., Jacquin, M., Turkstra, L. S., Haarbauer-Krupa, J., Johnson, J., Salloum, R., & Narad, M. E. (2018). Social participation and navigation (SPAN) program for adolescents with acquired brain injury: Pilot findings. Rehabilitation Psychology, 63(3), 327. https://doi.org/10.1037/rep0000187
- Wechsler, D., & Scale—Revised, WMS-R Wechsler Memory. (1987). Manual. The Psychological Corporation. In: Harcourt Brace Jovanovich Inc.
- Wen, W., Ishikawa, T., & Sato, T. (2013). Individual differences in the encoding processes of egocentric and allocentric survey knowledge. Cognitive Science, 37(1), 176–192. https://doi.org/10.1111/cogs.12005
- Wiener, J. M., Carroll, D., Moeller, S., Bibi, I., Ivanova, D., Allen, P., & Wolbers, T. (2019). A novel virtual-reality-based route-learning test suite: Assessing the effects of cognitive aging on navigation. Behavior Research Methods. https://doi.org/10.3758/s13428-019-01264-8
- Wilson, B. A., Evans, J. J., Alderman, N., Burgess, P. W., & Emslie, H. (1997). Behavioural assessment of the dysexecutive syndrome. In P. Rabbit (Ed.), Methodology of frontal and executive function (pp. 239–250). Psychology Press Ltd.
- Winkens, I., Van Heugten, C. M., Fasotti, L., & Wade, D. T. (2009). Reliability and validity of two new instruments for measuring aspects of mental slowness in the daily lives of stroke patients. Neuropsychological Rehabilitation, 19(1), 64–85. https://doi.org/10.1080/09602010801913650
- Wolbers, T., & Hegarty, M. (2010). What determines our navigational abilities? Trends in Cognitive Sciences, 14(3), 138–146. https://doi.org/10.1016/j.tics.2010.01.001
- Xie, Y., Bigelow, R. T., Frankenthaler, S. F., Studenski, S. A., Moffat, S. D., & Agrawal, Y. (2017). Vestibular loss in older adults is associated with impaired spatial navigation: Data from the triangle completion task. Frontiers in Neurology, 8, 173. https://doi.org/10.3389/fneur.2017.00173
- Zhong, J. Y., & Kozhevnikov, M. (2016). Relating allocentric and egocentric survey-based representations to the self-reported use of a navigation strategy of egocentric spatial updating. Journal of Environmental Psychology, 46, 154–175. https://doi.org/10.1016/j.jenvp.2016.04.007
- Zwecker, M., Levenkrohn, S., Fleisig, Y., Zeilig, G., Ohry, A., & Adunsky, A. (2002). Mini-mental state examination, cognitive FIM instrument, and the Loewenstein occupational therapy cognitive assessment: Relation to functional outcome of stroke patients. Archives of Physical Medicine and Rehabilitation, 83(3), 342–345. https://doi.org/10.1053/apmr.2002.29641