ABSTRACT
A widely accepted view is that errorless learning is essential for supporting new learning in people with anterograde amnesia, but findings are mixed for those with a broader range of memory impairments. People at a chronic stage of recovery from brain injury (BI) with impaired memory and executive function (N = 26) were compared with adults in a comparison group without any known risks to brain function (N = 25). Learning techniques were compared using a “Generate-and-correct” and “Read-only” condition when learning novel word pairs. At test, both groups scored above chance and showed benefits of Generate-and-correct (errorful learning). Poor learners in the BI group were classified from “flat” learning slopes extracted from an independent word-pair learning task. Critically, poor learners showed no benefit, but also no decrement to learning, using the Generate-and-correct method. No group was harmed by errorful learning; all, except the poorest learners, benefitted from errorful learning. This study indicates, that in some rehabilitation settings, encouraging clients to guess the meaning of unfamiliar material (e.g., from cards, magazines, newspapers) and then correct their errors, could have benefits for recognition memory. Determining when and how errorful learning benefits learning is a key aim for future research.
Brain injury (BI) has been increasing worldwide over the past few decades, with a concurrent reduction in mortality rate by 30–40% (Rosenfeld et al., Citation2012). For example, within the period of one year in the UK, there were 356,699 hospital admissions for acquired BI in 2019–2020 (a 12% increase since 2005–2006), with 158,255 from traumatic BI (TBI) and 137,403 from stroke (Headway, Citation2023). This burgeoning population of survivors of TBI need prolonged rehabilitation and care (Faul et al., Citation2007) to cope with the cognitive, physical, and psychological consequences of BI. Moderate to Severe BI, whether from closed or penetrating insult, will incur cognitive sequalae lasting over six months, to years after the original injury (Dikmen et al., Citation2009; Ruet et al., Citation2019), and even mild BI impacts on cognitive, somatic, and well-being outcomes (Polinder et al., Citation2018). Episodic memory and dysexecutive impairment are pervasive consequences of BI that influence recovery (Jennett et al., Citation1981; Ruet et al., Citation2019; Vakil, Citation2007; Vakil et al., Citation2019). As BI tends to be diffuse, rather than focal, white matter integrity and patterns of hypo/hyperactivity across networks are of interest (e.g., Chiou et al., Citation2016; Ramage et al., Citation2019), especially in relation to memory impairment (Gillis & Hampstead, Citation2015; Mallas et al., Citation2021; Palacios et al., Citation2013; Strangman et al., Citation2008). To meet the ongoing need for effective rehabilitation, there is a need for research to identify the optimal way for individuals to learn new information under different circumstances. Targeted intervention is essential given the heterogeneity of individual cognitive profiles (Chiou et al., Citation2016; Mallas et al., Citation2021).
Cognitive recovery can lead to broad-based benefits for quality-of-life outcomes (Novack et al., Citation2001). With more efficient memory and learning, recovery rates from BI can be optimized and lead to stronger outcomes (Ownsworth et al., Citation2010, Citation2013; Skidmore, Citation2015). Finding ways to improve memory and learning has been the focus of numerous cognitive interventions post-injury, even when cognitive impairment (CI) is severe (Page et al., Citation2006; Tailby & Haslam, Citation2003; Wilson et al., Citation2010). One approach has been to introduce errorless learning techniques (Terrace, Citation1963; see also Baddeley & Wilson, Citation1994) that aim to avoid introducing errors that could be subsequently mis recalled (Middleton & Schwartz, Citation2012; Tailby & Haslam, Citation2003). Typically, these techniques focus on learning within a single domain, where learning is broken down to simple steps, and there is little flexibility in responding; people only attend to the correct response. It originally targeted individuals with severe anterograde amnesia (Middleton & Schwartz, Citation2012), but benefits of errorless techniques have been identified across numerous populations with varying degrees of memory impairment (Clare & Jones, Citation2008; Hunkin et al., Citation1998; Middleton & Schwartz, Citation2012; Page et al., Citation2006; Squires et al., Citation1997; Tailby & Haslam, Citation2003; Wilson et al., Citation2010). In other groups with concomitant executive dysfunction and impaired memory, errorless techniques still have demonstrable benefits for memory (Cohen et al., Citation2010; Pitel et al., Citation2006). In cases of amnestic Mild Cognitive Impairment (MCI), errorless learning benefits recall of word-pairs and word-wordlists (Callahan & Anderson, Citation2019; Lubinsky et al., Citation2009; Roberts et al., Citation2018). For people with and without memory impairment, introducing errors as a response to a memory test can influence learning. By introducing errorless learning techniques, the aim is to reduce the likelihood of generating mistakes that could subsequently be recalled at a later point (Wilson et al., Citation2010). One technique is to use “vanishing cues” to target cue reduction (Glisky et al., Citation1986). Glisky et al. manipulated the cues for new vocabulary by gradually reducing their availability over repeated learning opportunities for each new word. As noted by Clare and Jones (Citation2008), this, and similar techniques, were generally superior over shorter intervals, but has relatively weaker retention over longer intervals, relative to alternative techniques involving trial and error (guessing). From a practical perspective, there are questions regarding the likelihood of achieving genuinely “errorless” learning, given the preponderance of mistakes in a memory-impaired population. Within classic models of memory (see Atkinson & Shiffrin’s multi-modal model amended by Hildebrandt, Citation2019), eliminating error cues could align with simple, repetition techniques to support encoding. Other factors may contribute to the distinction, notably through a reduction in self-initiated effort to produce responses or by reducing the demands of monitoring guess responses, especially given high levels of fatigue (Ramage et al., Citation2019). If response generation is required during encoding, then resource allocation will need to increase accordingly (e.g., Schmidt, Citation1990; Slamecka & Graf, Citation1978).
A critical feature of the earlier literature on errorless learning is the focus on the benefits for recall, rather than for recognition. Being able to recognize words, people, and places is instrumental for effective daily functioning; people prefer familiar material. For example, when accessing information such as current news and events, advice from health professionals, and when contacting friends and family, familiar information can feel comforting and easier to access. The familiarity of an item can support recognition for amnestic individuals (Giovanello et al., Citation2006). According to the dominant theoretical framework, two different processes are thought to result in successful recognition (e.g., Aggleton & Brown, Citation1999; Yonelinas, Citation2002). Recognition memory has long thought to be the result of “remembering”, that is, actually recollecting the stimulus, and “knowing”, that is the feeling of familiarity accompanied by the presentation of a given stimulus (Tulving, Citation1985). Aggleton and Brown (Citation1999) posited selective, but interlinked, structures of the medial temporal lobe (MTL) that support these two aspects of recognition. Whereas remembering (i.e., recollection) is typically hippocampal-dependent, the feeling of knowing (i.e., familiarity) is instead reliant on the perirhinal cortex (PrC; Brodmann 35 and 36) that lies adjacent to the hippocampus (although see Bussey and Saksida (Citation2005) for the involvement of more posterior, ventral-visual pathways). Whilst errorless techniques can offset the misleading familiarity of generated error responses, these techniques also offer fewer recollective opportunities (e.g., Anderson & Craik, Citation2006). Damage to the hippocampal region of MTL can occur after traumatic BI (e.g., Ariza et al., Citation2006), although white matter integrity and disrupted connectivity are also linked to memory impairment (e.g., Palacios et al., Citation2013). If damage to these regions compromises recollection, then familiarity of the item can support recognition (Giovanello et al., Citation2006).
By contrast, errorful learning techniques involve encouraging participants to generate or guess responses, which typically results in making multiple errors in the learning process, for example, by generating a meaning for an unknown foreign word, or other trial and error techniques (e.g., Baddeley & Wilson, Citation1994; McKissock & Ward, Citation2007). Errorful learning is typically explored in the context of new word learning, although nonverbal material may also benefit (e.g., Evans et al., Citation2000; Lloyd et al., Citation2009). An early interpretation was that response generation/guessing only improves the retrieval of word pairs when there is a semantic association, such as in frog-pond (e.g., Grimaldi & Karpicke, Citation2012). In this way, the errors draw on existing semantic networks that activate related concepts; these serve as additional cues for mediating retrieval (e.g., Mera et al., Citation2022). However, one can distinguish the mediating role of semantic relatedness between cues, targets and guesses, from the benefits to learning when corrective feedback is given (e.g., Huelser & Metcalfe, Citation2012; Zawadzka & Hanczakowski, Citation2019). In healthy adults, providing this feedback immediately after generating a guess on a trial-by-trial basis benefits subsequent recall and recognition of word-pairs (e.g., Overman et al., Citation2021). The value of generating guess responses has been shown for novel word learning using recognition paradigms (Potts & Shanks, Citation2014; Seabrooke et al., Citation2019a; Seabrooke et al., Citation2021). For example, Potts and Shanks (Citation2014) conducted a series of experiments involving multiple choice recognition which identified that response generation (regardless of semantic association to the cue) was an advantage for subsequent memory recognition, over studying the cue-target in a Read-only (errorless) condition. Over five experiments conducted by Seabrooke et al. (Citation2019a), errorful learning induced by guessing a response (compared to read-only) strengthened the recognition of both the cue and the target on tests of item recognition, and on multiple choice of targets from unfamiliar foils, but not on tests of associative recognition and cued recall. Response generation favours enhanced recognition of the memory target, possibly by enhanced allocation of attention to the target on receipt of corrective feedback. In related work, Seabrooke et al. (Citation2021) further showed the memory benefit as linked to the target itself, rather than retrieving incidental contextual detail. Together, this body of work highlights the potential benefits of errorful learning for healthy participants, at least for verbal learning under specific test conditions.
Determining the best method of instruction (whether errorful or errorless) has important consequences for rehabilitation of people with memory impairment. For these populations, the assumed benefits of traditional errorless learning approaches have come into question in recent years (see Clare & Jones, Citation2008; Middleton & Schwartz, Citation2012, for extended discussion). Errorful learning seems just as, or more, effective at improving recall and recognition in some cases (Evans et al., Citation2000; Hunkin et al., Citation1998; Squires et al., Citation1997). For example, Squires et al. (Citation1997) compared errorful and errorless techniques with amnestic patients learning verbal paired associates and found significant forgetting using errorless techniques after a delay; this was not seen for errorful learning. However, using novel associates benefited from errorless learning. In other work by Metzler-Baddeley and Snowden (Citation2005), errorless learning is argued to be beneficial only for those with severe memory impairment, with significant benefits of errorful learning when both the task and residual memory capacity allows in a wider range of memory impairment. Broader cognitive processes than hippocampal-dependent memory can support learning, potentially through frontally mediated (executive) activities such as error detection, goal monitoring, response inhibition, and compensatory behaviours. Invoking error monitoring is one aspect of errorful learning that can diminish the relative benefit of errorless learning, when recollection is impaired (e.g., Roberts et al., Citation2018).
There are various accounts of why and how errorful techniques hold benefits for learning (see Mera et al., Citation2022, for a review). According to additional-cue theory, the additional “guess” response acts as an additional retrieval cue, leading to an increase in available cues to guide retrieval (Grimaldi & Karpicke, Citation2012), especially if self-initiated (Metcalfe & Xu, Citation2018). A second account points to the value of error correction (e.g., Rescorla & Wagner, Citation1972) which is grounded in the long-standing idea that learning results from the difference between what is expected (guess) and what actually happens (target) on a given trial (e.g., Kornell et al., Citation2009). A third account is based on the motivational mediation of learning (e.g., Potts & Shanks, Citation2014). If there is a stronger motivational, or emotive, aspect to learning the correct answer after generating a guess, then this results in a higher motivation to learn the correct word. This view aligns with evidence (e.g., McKissock & Ward, Citation2007) that being able to monitor errors holds benefits for learners who can retain insight to their own performance, especially after receiving corrective feedback. For example, McKissock and Ward (Citation2007) found that feedback maintained similar benefits (whether errorful or errorless) for anomic learners, and these benefits for learning were stronger than errorful instruction without feedback. Clare and Jones (Citation2008) postulated that errorful learning is supported by processes beyond hippocampal-dependent remembering, such as the allocation of attention during encoding, or the ability to draw on frontally mediated control processes. They highlighted the possible benefits of a broader focus of attention with the need to retain, or rehearse, the guessed response, and engage with other contextual factors (see also Hildebrandt’s, Citation2019), putative amendments to multi-modal model).
Taken together, the uncertainty about the benefits of errorless learning in healthy participants and in brain-injured participants means that it is crucial to find out how and when these techniques are beneficial. We pick up on the proposition of Clare and Jones (Citation2008) that errorful learning benefits people with mild-to-moderate memory impairment, compared to the more restricted training methods used for those with severe memory impairment (following Baddeley & Wilson, Citation1994). The primary aim of the present study is to compare the benefits of errorful and errorless techniques for verbal learning in people living with long-term brain injury. We used a simple experimental word-pair learning task that required participants to draw on errorful and errorless techniques to examine potential benefits on recognizing the correct target word in a multiple-choice recognition test with matched lures. We posit that errorful learning can offer greater resource allocation to individual item recognition after receiving corrective feedback. Putative mechanisms could arise from increased strength of encoding of the memory target when attention is directed by corrective feedback, or by engaging control processes that support semantic selection of the memory target, post-retrieval.
A secondary aim of this study was to explore the potential benefits of these techniques in the context of targeted rehabilitation. First, we selected accessible, neuropsychological markers that would characterize patterns of impaired cognition in this population. For example, standard neuropsychological tests such as the Montreal Cognitive Assessment (MoCA; Julayanont et al., Citation2012; Nasreddine et al., Citation2005) and the Wechsler Memory Scales (e.g., Wechsler Memory Scale-Fourth Edition; WMS-IV; Wechsler, Citation2009) are commonly used in rehabilitation settings. A recent large-scale study of mild and moderate cases of traumatic BI (Panwar et al., Citation2019), showed four subscales of the MoCA (Language, Executive/visuospatial, Attention and Delayed Recall) were the most sensitive descriptors of cognitive impairment and discriminated mild from moderate traumatic BI. We refer to this as a Language, Executive, Attention, Delayed Recall (LEADeR) profile, which concurs with reports that impaired memory and attention lapses are common sequela of BI, often linked to frontally mediated, or diffuse, brain damage (Shah et al., Citation2017). To characterize the cognitive impairment of our BI group, we used the MoCA to compare and evaluate the LEADeR profile previously reported in traumatic BI.
Finding simple measures that enable targeted intervention is important to increase the likelihood of intervention success. We used measures of verbal fluency (e.g., phonemic and category fluency) that are sensitive to frontal lobe dysfunction (e.g., Alvarez & Emory, Citation2006), plus measures of semantic cognition (e.g., picture naming and word-picture matching) that capture the control and co-ordination of semantic knowledge. As memory impairments of varying severity are a well-established sequelae of brain injury linked to the medial temporal lobe (TL) memory system (Ariza et al., Citation2006), we took measures of word-pair learning over repeat trials and a short delay to establish deficits in associative memory. Word list memory tests are increasingly acknowledged as having multiple outcome measures e.g., intrusion errors, proactive interference, learning slopes, that can classify early MCI -, or more severe memory impairment (Clark et al., Citation2012; Gifford et al., Citation2015). Moreover, capturing learning slopes across repeated trials can be more sensitive to severe impairment (Gifford et al., Citation2015), at least compared to summative measures of memory that can mask poor performance (Delis et al., Citation1988; Nettelbeck et al., Citation1996). In Chiou et al. (Citation2016), a distinction was made between “learners” who were trained to criterion on a word list learning task (i.e., they recalled two perfect lists of 10 items, over 15 repeat trials), compared to “poor learners” who did not achieve criterion recall. Notably, these learners had markers of white matter integrity (fractional ansiotropy values) that were higher for learners than non-learners. Specifically, white matter integrity was greater within the anterior thalamic and the inferior fronto-occipital fasiculus (iFOF) tracts. The integrity of thalamic tracts has been linked to executive deficits after traumatic BI (Little et al., Citation2010). In addition, the compromised integrity of iFOF tracts in Chiou’s “non-learners” points to the interface of semantic processing with goal-oriented behaviour as significant to learning. Similar to the Chiou et al. (Citation2016) study, we sought to classify “poor learners” and “learners” after BI. Regression of learning slopes were extracted from four trials of word-pair associate learning to target the potential benefits of different learning techniques to learners of differing ability. In this way, we extend Chiou’s findings by identifying non-learners through a relative disadvantage in recalling familiar word-pairs (not learning individual words to criterion). Like the amnestic MCI cases of Roberts et al. (Citation2018), we assume that better error monitoring should diminish benefits of errorless learning in learners.
The current study sampled people only from rehabilitation settings to represent the wide spectrum of people living with the cognitive sequelae of BI from months to decades later. There were two aims; first, to identify the benefits of errorless and errorful learning techniques after BI, and second, to characterize cognitive impairment after BI in order to explore more effective targeting of learning techniques within the BI group. We anticipated a likely range of (recollective) memory and cognitive impairments after BI that can impact on learning. We predict an advantage for errorful over errorless learning since selection and monitoring demands (post-retrieval) are reduced in single item recognition, but this predicted benefit is likely to be stronger for learners than non-learners. By comparison to healthy young adults, we anticipated better errorful learning for item recognition in these highly competent learners.
Materials and methods
Participants
Two groups of participants were recruited: Individuals living with chronic state BI and a comparison group living without known clinical or neurological risk to brain function.
Twenty-six participants living with chronic state acquired brain injury (BI group: 17 males: 9 females; Mage = 50.3, Rangeage = 25–67 years) were recruited via opportunity sampling of individuals and families supported by Headway (UK); a voluntary charitable organization providing social interaction and support for people affected long-term by brain injury. All participants were native English speakers, and from mixed educational backgrounds of which 54% were educated for less than or equal to twelve years and 46% were educated to degree level. On subjective measures of memory and attention (see supplementary materials for full detail of scores from the Everyday Memory Questionnaire-Revised, Royle & Lincoln, Citation2008, and the Attention Process Training Questionnaire-II, Sohlberg et al., Citation2000), this BI group reported highly frequent memory and attentional lapses consistent with poor recollective memory, as illustrated in Supplemental Materials.
Full ethical approval for the study was obtained from the relevant University of Bristol, Faculty of Science Research Ethics Committee. Initial contact was made with clients via a specialist gatekeeper, followed by telephone contact with clients and their carers to ascertain suitability for inclusion in the study, prior to obtaining consent for participation in accordance with the ethics protocol. Participants were excluded from the study if they were diagnosed with a progressive brain condition (including dementia, multiple sclerosis, myalgic encephalomyelitis, motor neurone disease, Parkinson’s disease, or Huntington’s disease) or with a significant speech and language impairment (e.g., in stroke-related aphasia). As part of the consenting process, participants completed a short questionnaire (with family/carer support for completion, if needed) that asked for details of the nature and location of their BI, with any arising difficulties, and their current coping strategies. In response to a request to provide a brief explanation of their brain injury (including how, where, when this occurred), approximately half of this group reported traumatic BI originating from road traffic accidents (n = 11), assault (n = 2) or a fall (n = 1), with the remainder reporting acquired BI after a stroke (n = 4), tumour (n = 4), associated with infection (n = 1), brain surgery (n = 1), hypoxia (n = 1) or from calcium deposits in the brain (n = 1). When asked to provide details of the areas of the brain affected by injury, participants self-reported mostly diffuse axonal injury and/or global insult (n = 5), or global insult affecting either one, or both, hemispheres (left n = 4, right n = 2, both n = 2), or diffuse insult across the frontal lobes (n = 6). A few individuals reported more focal damage to the limbic system (n = 1), left temporal lobe (n = 1) or the cerebellum and all remaining participants were either unsure (n = 1) or were unable to specify their brain injury (n = 4). No participant was in the initial (post-acute) stages of recovery, the duration since injury ranged from 2 to 41 years, where seven participants were within five years post-injury.
Short-term memory problems were reported by all but two cases in the BI group, and often reported as co-occurring with long-term memory difficulties (n = 14; 54%). Several cases (n = 6) reported their difficulties clinically as an anterograde amnesia, with one case preferring this description alone. Dysregulation of executive cognition in the BI group was reported as lack of inhibition, poor executive function, and/or confusion by fourteen cases (54%), typically in conjunction with other cognitive and emotional difficulties. Only one case described their difficulties as exclusively linked to executive function without any reference to poor memory.
As a comparison group, a further twenty-eight participants (Comparison group: 4 male, 24 female; Mage = 27 years, Rangeage = 18–63 years) were recruited by volunteer sampling at the University of Bristol, either by word-of-mouth with entry into a small cash prize draw, or from an undergraduate population with participation in lieu of course credit. Most participants in this group were native English speakers (n = 22), and with strong educational backgrounds, educated for at least twelve years or more, and with 43% of the group educated to degree level. Similar to the BI group, participants were excluded if diagnosed with a progressive brain condition, or any form of neurological or congenital disorder, or living with a significant speech and language/ hearing impairment or had previously experienced a traumatic life event with risk of acquired BI or had undergone brain surgery.
This group outperformed the BI group on pre-morbid IQ (The National Adult Reading Test, Nelson & Willison, Citation1991; MBI = .46, SD = .24 vs MCOMP = .61, SD = .12; t = 2.79; p < .001, d = .77) and fluid IQ (Raven's Coloured Progressive Matrices, Raven et al., Citation1998; MdnBI = .87, vs MdnCOMP = .97; U = 547, p < .001; rpb = .67), with scores indicative of the normative population of cognitively intact, young adults. No attempt was made to match this group to the BI group across demographic or cognitive indices of performance.
Design and measures
An experimental word-learning task was investigated within a 2 (Group: BI vs comparison) x 2 (Learning Technique: Errorful vs Errorless) mixed design with Group as a between-subjects factor and Learning Technique as a repeated measure, alongside assessments of general cognitive ability.
Experimental word-learning task
This task was based on the design of a previous study of unfamiliar word-learning (Potts & Shanks, Citation2014; Experiment 2b), learning unfamiliar (Euskara) words and their translations. Participants engaged in two learning techniques: a Read-only method (errorless) and a Generate-and-correct method (errorful). In this design, error generation on a single trial was more similar to “pre-testing” effects in healthy adults (e.g., Potts & Shanks, Citation2014; Seabrooke et al., Citation2019a, Citation2021).
The word-learning task involved three phases: an encoding phase, a distractor task, and a test phase for 60 Euskara-English word-pairs (from Experiment 2b of Potts & Shanks, Citation2014, and Experiment 5 of Seabrooke et al., Citation2019a). In the encoding phase, trials were administered in four inter-mixed blocks that were counterbalanced to control for order effects. Participants therefore received four blocks either in “Read-Generate-Read-Generate” order or in “Generate-Read-Generate-Read” order. Each block contained 15 trials in a fixed sequence, within and across blocks, so that all participants received all 60 word-pairs in the same fixed sequence.
Each encoding trial consisted of onscreen presentation of a single slide that displayed an unfamiliar (Euskara) word, either with its translation (in the Read-only condition e.g., aker = ram), or with a question mark (in the Generate condition e.g., aker = ?). On all encoding trials the words were displayed centrally in black lowercase font (Calibri Headings; size 72) on a white background, using a 15.6-inch screen. Each slide was displayed for a maximum of 20 secs so there was sufficient time to either read or generate a possible guess. Immediately after a guess response, corrective feedback was provided as a single slide with a cue – target pair (e.g., oihan = jungle) displayed centrally onscreen for 20 secs (i.e., identical to the Read-only condition). The experimenter read aloud both words in the Read-only condition, and read the Euskara work followed by a prompt in the Generate condition, and both words once a guess had been generated.
In the distractor phase, all participants took part in a search for a cartoon character hidden amongst a dense, visually complex display for 3 mins (a “Where’s Wally” task). In the test phase, all participants completed a 60 item-recognition test, with a fixed order of presentation. Each test trial consisted of onscreen presentation of a single slide displaying a Euskara word cue at the top of the screen, with four word choices below, including one target translation (correct) and three lures (incorrect). All test trials displayed the words centrally in black lowercase font (Calibri Body; size 66) on a white background. In this test phase, the experimenter did not read the words aloud.
Screening for cognitive impairment
To characterize the clinical risk of cognitive impairment in a heterogeneous BI group, the Montreal Cognitive Assessment (MoCA) provides a quick screening assessment of cognitive ability within a cluster of 12 tests contributing to 7 functional aspects of cognition (Visuospatial/Executive, Attention, Delayed Recall, Language, Naming, Abstraction, and Orientation). With a maximum summative score of 30, a score < 26 indicates cognitive impairment. Since the MoCA is a tool that is commonly used in rehabilitation settings, we probed scores for the seven sub-tests as a proxy measure of cognitive variation in the absence of a full neuropsychological test battery. We identified the LEADeR profile across subtests for comparison with other cohorts, and for comparing different groups of learners.
Assessment of verbal learning, semantic cognition and verbal fluency
Verbal learning
To supplement the Delayed Recall subtests from the MoCA, we administered the Verbal Paired Associates (VPA) sub-test of the WMS-IV (Wechsler, Citation2009). This required memorizing four lists of 10 word-pairs (presented in different order within each list) for immediate cued recall, followed by recall and recognition after a short delay. This indexed supraspan learning of the pairwise association between words and their subsequent recall. This contrasts with the Delayed Recall subtest of the MoCA that required memorizing five words in two trials, presented with a 5-minute delay. For the purposes of the present study, we considered that supra-span learning over repeat trials on the VPA was a more optimal way to distinguish verbal learning deficits linked to a broad range of memory impairment, than learning words in isolation (list learning). Proportional accuracy scores were calculated for each of four immediate recall trials, plus the subsequent delayed recall and recognition trials. Learning (regression) slopes were calculated from the four sequential presentations of word-pairs. Slope scores (of 0.08 or less) were used to identify poor learners and determine the relative benefits of learning technique in relation to the cognitive profile of different learners.
Semantic cognition
To complement the use of the Naming and Abstraction subtests of the MoCA that tapped semantic knowledge and conceptual thinking, two subtests of the Cambridge Semantic Memory Test Battery (SMTB; Adlam et al., Citation2010) were administered to further assess the integrity of semantic cognition. Picture-naming and word-picture matching in the Camel and Cactus Test (e.g., Bozeat et al., Citation2000) can capture semantic dysfunction linked to degraded conceptual knowledge stored in the anterior lobes (e.g., Hodges & Patterson, Citation1997; Visser & Lambon-Ralph, Citation2011). However, naming can also draw on frontally mediated pathways, including the left-inferior frontal gyrus, that underpin selection and retrieval of semantic competitors in healthy participants (e.g., Thompson-Schill et al., Citation1997). Total scores for each subtest were compared across different learners to consider relative strengths and weaknesses in semantic cognition.
Verbal fluency
To supplement fluency tests in the MoCA (Language subscale), we used the Verbal Fluency (VF) subtest from the Delis–Kaplan Executive Function System (D-KEFS; Delis et al., Citation2001). Verbal fluency engages task monitoring (updating) as participants need to retrieve and generate words consistent with task instruction (Shao et al., Citation2014). Phonemic fluency measures are highly sensitive to frontal lobe dysfunction (Alvarez & Emory, Citation2006), whereas category fluency draws on frontally mediated neural pathways to anterior portions of the temporal lobe that facilitates the control and co-ordination of semantic knowledge. Scores included totals of words retrieved with the same specified phoneme, from the same category and when switching between two semantic categories, in total, and without error. Scores were compared for different learners to identify whether flexible allocation of resources within a generative word retrieval task converged with any observed benefit for errorful learning in item recognition.
Procedure
Participants were provided with the details of the study in advance. After consenting to the study, questionnaires were provided to participants and, for the BI group participants, carers, prior to the test sessions; the BI group were offered further assistance in questionnaire completion. In the BI group, participants attended three test sessions (of approximately 30 mins each) held in a familiar daycentre, of which the first two sessions included screening for general cognitive ability, memory and attention, and the third session included the experimental measure of unfamiliar word-learning. Tasks were administered in approximately the same fixed order, as follows: background questionnaire, EMQ, APTQ, VPA-immediate, MoCA, NART, VPA-delay, Verbal Fluency subtest of the D-KEFS, picture naming and word-picture matching, RCPM, followed by the experimental word learning task, a distraction task, and finally the word recognition task. For the comparison group, an identical set of measures were administered in one single session (approximately 90 mins) and in the same order as the BI group.
For the VPA (immediate and delayed), the MoCA, NART, Verbal Fluency, and the RCPM tests, participants followed the standard test protocol. For the experimental word-learning task, all participants were initially instructed to learn translations for “foreign” words using two different techniques (Read-only and Generate-and-correct) and that they should try to remember the correct translations of the words for a subsequent memory test. At the start of each block of 15 trials, onscreen instructions were provided to offset the potential difficulty of remembering the task instructions for individuals in the BI group. The instructions for the Read-only condition were:
You will be shown an unfamiliar word with the correct translation next to it. Read the word and translation and try to remember them for later. Each slide will change after 20 s but you may move on before that if you are ready.
You will be shown an unfamiliar word and asked to guess what you think it could mean. Remember, you are not expected to get these right! You will then be shown the correct translation, try to remember this for later. Each slide will change after 20 s but you may move on before that if you are ready.
With the BI group, the idea of generating an “incorrect guess” was difficult for a few individuals; they reported that they did not “see the point” in getting the answer wrong. These participants needed extra encouragement and reminding that they were not being scored for the guessed response. As a self-paced encoding task, participants were able to move to the next trial, when ready to do so. However, some BI participants needed a few seconds longer than the allocated 20 s to generate a guess. After completing all 60 trials in the encoding phase, participants were instructed to search for a cartoon character hidden amongst a dense, visually complex display. Finally, for the test phase, participants were asked to select and read aloud the word they believed to be the correct translation of the cue. If they were uncertain, they were encouraged to guess. There was no time limit for responding to the test trials.
Results
Screening for cognitive impairment
Given the routine and well-justified use of the MoCA as a screening test for MCI in rehabilitation settings, total MoCA scores were calculated for participants in both groups. In the BI group, most cognitive profiles were classified below the cut-off for MCI (MoCA total score < 26; N = 23), of which the majority met more stringent criteria associated with more severe CI (MoCA total score < 21; N = 12). Of these, three had difficulty with temporal orientation (to the month or year) that is highly sensitive to dementia pathology. Only three individuals in the BI group appeared to be un-impaired (MoCA total scores > 26). In the comparison group, three participants also met criteria for MCI (MoCA total scores < 26) and so were excluded from further analysis, leaving a group of 25 participants in this group (4 male; 22 female, Mage = 27, Range = 18–63 years).
To characterize the profile of cognitive impairment, shows the distribution of proportional correct scores across seven cognitive functions of the MoCA for the BI group (left panel) and the Comparison group (right panel).
Figure 1. Scores for subscales of the MoCA in the Brain-injured (left panel) and Comparison group (right panel). Note: The distribution of proportional accuracy scores across the seven functional scales of the MoCA shown for the Brain-Injured group (left panel) and the Comparison group (right panel).
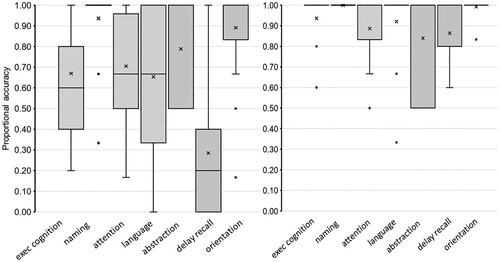
From the MoCA, naming and orientation were relatively well-preserved in both the BI and Comparison groups as demonstrated by means of approximately .90 or above (apart from 2–3 exceptions of cases with severe cognitive impairment in the BI group). In addition, group comparisons revealed that scores for the abstraction sub-scale were well-matched across groups (Abstraction; MdnBI = 1.0, vs MdnCOMP = 1.0; U = 367; p = .18). This apparent integrity of semantic and conceptual knowledge on the MoCA was also confirmed by two supplementary measures of semantic cognition. Across groups, mean performance was well-matched for Picture-Naming (MBI = .96, SD = .08 vs MCOMP = .97, SD = .05; t = .81; p = .21, d = .23) and for Picture-Matching-(MdnBI = .89, vs MdnCOMP = .89; U = 341, p = .38; rpb = .05).
A marked impairment of the BI group on the MoCA was evident on four remaining sub-scales of the MoCA (Language, Executive, Attention and Delayed Recall). The comparison group significantly outperformed the BI group on Language (MdnBI = .67, vs MdnCOMP = 1.0; U = 487, p < .001, rpb = .49), Executive/visuospatial cognition (MdnBI = .66, vs MdnCOMP = .94; U = 539, p < .001, rpb = .66) and Attention- (MdnBI = .67, vs MdnCOMP = 1.0; U = 484; p < .01, rpb = .49). This dysexecutive impairment in the BI group concurred with a sizeable disadvantage on supplementary measures of verbal fluency. The BI group had significantly weaker scores relative to the comparison group, whether retrieving words by letter (MBI = 7.50, SD = 3.72 vs MCOMP = 12.56, SD = 2.88; t = 5.41; p < .001, d = 1.51) or by category membership (MBI = 7.85, SD = 3.79 vs MCOMP = 14.64, SD = 4.07; t = 6.17; p < .001, d = 1.73). Fewer words were retrieved when switching between semantic categories (MBI = 5.92, SD = 3.98 vs MCOMP = 11.92, SD = 3.62; t = 5.63; p < .001, d = 1.56) and category switching led to less accurate performance (MBI = 5.92, SD = 3.98 vs. MCOMP = 11.16, SD = 3.24; t = 5.54; p < .001, d = 1.55). Of the BI group, 18 (69%) had at least one verbal fluency score that was abnormally low relative to the general population (scaled score = 5 or less, Crawford et al., Citation2011).
Finally, the BI group had markedly depressed scores on the delayed recall subscale of the MoCA (MdnBI = .29, vs MdnCOMP = .86; U = 608, p < .001, rpb = .87) with an average score of 1.42 nouns recalled out of a possible 5 and where 9 participants (34.6%) scored zero. 34.6% participants were at floor despite trials with multiple choice options. In addition, the BI group recalled fewer word-pairs after a short delay on a supplementary memory test (MdnBI = .42, vs MdnCOMP = .89; U = 605, p < .001; rpb = .86). Both groups performed better on the delayed recognition of word-pairs relative to their recall, the BI group recognized fewer items than the comparison group (MdnBI = .84, vs MdnCOMP = .98; U = 596, p < .001; rpb = .84).
Experimental manipulation of unfamiliar word-learning
To address the primary aim of this study, two different learning techniques were contrasted for supporting learning unfamiliar word-pairs. Overall, recognition performance (as measured by proportion correct: the sum of correctly selected target words divided by the total number of trials) was above chance (.25) in the Read-only and Generate-and-correct conditions (MREAD = .65; one-way t = 13.15, p < .001 and MGENERATE = .74; one-way t = 16.12, p < .001), indicating learning was effective under both techniques. displays the mean proportion correct recognition scores by group.
Figure 2. Recognition scores of Brain-injured and Comparison groups. Note: Proportions of correct item recognition in the Read-only and Generate-and-correct conditions, displayed separately for the Brain-injured and Comparison groups. Error bars displayed as 95% Confidence Intervals.
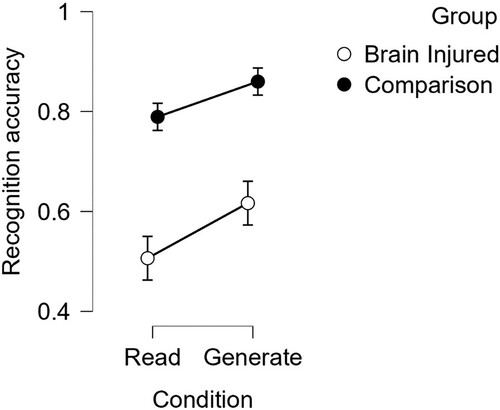
As displayed in , a mixed 2 (Group: BI vs. comparison) * 2 (learning technique; Read-only vs. Generate-and-correct) ANOVA indicated that word recognition was poorer in the BI group overall, F(1, 49) = 35.3; p < .001; η2 = .42, and there was a clear benefit of the Generate-and-correct over the Read-only condition in both the BI and comparison groups, F(1, 49) = 25.8; p < .001; η2 = .34. The advantage for the Generate-and-correct condition was comparable in both groups, F (1, 49) = 1.23; p = .27; η2 = .02. One assumption of the Generate-and-correct condition is that participants generate a “guess” response relative to the cue that is subsequently corrected. In these data, the two groups varied slightly in the likelihood of generating cues; 95% of cues generated a “guess” response in the BI group compared to 99% of cues generating these responses in the comparison group. Excluding trials where a response was omitted made virtually no difference to the quantitative pattern of results.
Closer scrutiny of the content of “guess” responses generated by the BI group indicated that retrieved answers varied. Some answers repeated the targets from a previously presented cue, although participants did not explicitly acknowledge the repetition of target items. In an exploratory analysis, using Levensthein’s distance (which provides an index of the orthographic similarity of two words), we compared guessed responses to cues to determine whether these guessed responses were orthographically similar to the unfamiliar cue (e.g., untxi = taxi). A larger score indicates more distance (lower orthographic similarity) between the target and response. No difference was observed between the BI group and the comparison group (mean distanceBI = 5.76, mean distanceComp = 5.60) in terms of orthographic distance between guesses and cues.
Targeting poor learners from verbal learning and memory profiles
A secondary aim of this study was to characterize poor learners in the BI group from their verbal memory and learning profile, in order to target learning techniques.
For more formal memory assessment, supra-span learning of word-pairs was evaluated using the VPA-I test. A 2 (group; BI vs comparison) * 4 (retrieval attempt; trials 1–4) mixed ANOVA was conducted on proportional accuracy scores. The BI group retrieved fewer word pairs overall, MBI = .31, SD = .24 vs MCOMP = .71, SD = .23; F (1, 49) = 61.4; p < .001; η2 = .41. Although recall scores increased over subsequent retrieval attempts in both groups, F (2.2, 108.5) = 124.19; p < .001; η2 = .17, the mean difference between groups increased significantly over repeated retrieval attempts on the four trials, F (2.2, 108.5) = 13.06; p < .001; η2 = .02, as displayed in .
Figure 3. Immediate recall of word pairs. Note: Proportional accuracy of word pair recall on consecutive retrieval attempts over four trials, displayed for the Brain-injured and Comparison groups. Error bars displayed as 95% Confidence Intervals.
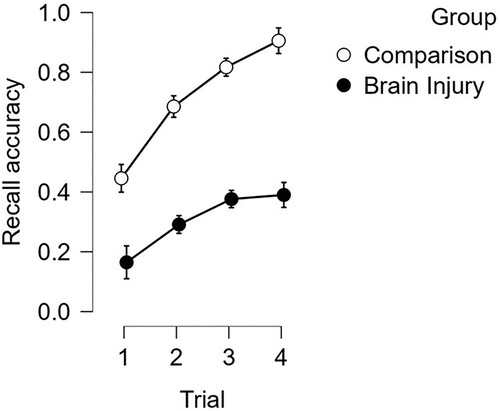
The small, but significant, decrement to learning was indicative of variable learning within the BI group. Learning (regression) slopes were then calculated from the four sequential presentations of word-pairs in the VPA-I test. Close inspection indicated that 9 participants from the BI group had extremely low retention of word pairs (i.e., slope scores of 0.08 or less) characterizing a marked deficit of new learning, that is, a poor learners sub-group. To characterise the profile of cognitive impairment in these poor learners, a shows the distribution of proportional correct scores across six cognitive functions of the MoCA for the BI-learners and the BI-poor learners sub-groups.
Figure 4. a. Proportional accuracy scores for six subscales of the MoCA, shown for the Brain-injured Learners and Poor learners sub-groups. b. Proportional accuracy scores for the language, picture naming and picture matching tests (top panel, left to right) and total scores for letter, category and switching fluency subtests of the D-KEFS (bottom panel, left to right), displayed for Brain-injured Learners and Poor learners sub-groups.
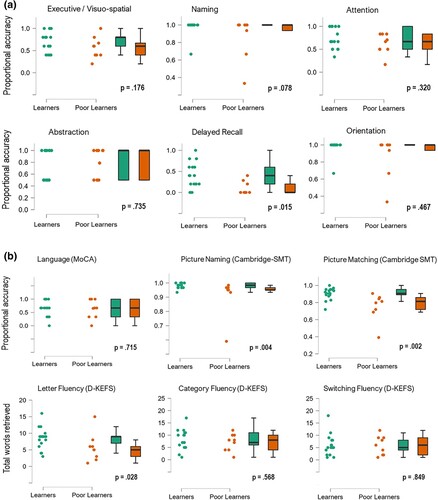
As shown in a, there was a range in cognitive impairment on six subscales of the MoCA (excluding language) within small sub-groups, so direct comparisons of sub-groups were not conclusive. One exception was delayed recall (middle, bottom row) where the poor learners appeared to have more severe impairment on this subscale (Mdnpoor learners = .00, vs MdnBI-learners = .04; U = 121, p = .015; rpb = .58). Distributions of scores on all other subscales were not suggestive of robust group differences; no further tests reached significance.
b displayed performance of subgroups across tests of executive and semantic cognition, including the language subscale of the MoCA (left, top row). In the top row, distributions were well-matched on the language sub-test of the MoCA, and highly similar on the two measures of semantic cognition, despite one markedly impaired individual in the poor learner group. Across the bottom row, total fluency scores were widely distributed in both sub-groups. Overall, there was a slight tendency for more severe impairment in the picture matching scores (Mdnpoor learners = .81, vs MdnBI-learners = .91; U = 133.5, p = .002; rpb = .74) and in generating words for letter fluency (Mdnpoor learners = 5, vs MdnBI-learners = 9; U = 117.5, p = .028; rpb = .54) in the poor learners sub-group. While strong conclusions cannot be drawn from these minor differences, they are suggestive of executive-semantic impairment in the poor learners.
Targeting learning techniques within the brain injury group
To explore the benefits of learning techniques for use in rehabilitation settings, we considered whether both techniques were effective for the poorest learners. Using slope scores as a classifier of poor learners distinct from BI learners, the relative benefit of different learning techniques was compared for cases with different patterns of CI after brain injury. displays the mean proportion correct scores for item recognition in the Read and Generate-and-correct conditions for the two sub-groups.
Figure 5. Mean recognition accuracy of Brain-injured sub-groups, with error bars shown as 95% Confidence Intervals. The average number of items recognized in the poor learners sub-group is approximately .4 for both Read-only and Generate-and-correct learning techniques. Recognition scores are higher for the learners amongst the Brain-injured group, and highest when they were previously invited to make a guessed response in the Generate-and-correct condition.
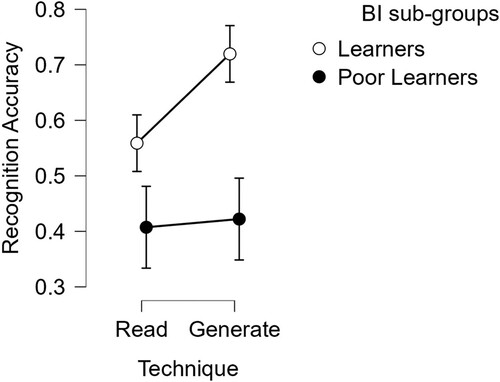
Recognition scores of brain-injured sub-groups
As can be seen in , both sub-groups were able to recognize items with scores above chance (> .25), however, only those who were learners showed benefits in the Generate-and-correct condition relative to the Read-only condition, resembling the benefit of errorful learning observed in the comparison group. By contrast, for the poor-learner sub-group, there was minimal difference between the mean averages for the two learning techniques, at 0.01. A mixed 2 (learning technique; Read-only vs. Generate-and-correct) * 2 (sub-groups; poor learners vs. learners) ANOVA confirmed that item recognition scores were significantly worse overall for the poor learners, F (1, 24) = 13.04, p = .001, ηp2 = .35. Although there was a significant benefit for item recognition in the Generate-and-correct condition, F (1, 24) = 9.45, p = .005, ηp2 = .28, this was qualified by a significant interaction with sub-group, F (1, 24) = 6.53, p = .017, ηp2 = .21. For individuals with flat learning slopes after brain injury, their recognition performance was compromised and they showed no benefits in the Generate-and-correct condition compared with the Read-only condition, however, importantly they also showed no detriment to learning when using this errorful learning technique.
Discussion
Using a common neuro-psychological screening tool, the MoCA, we identified impaired language, executive, attention, and delayed recall in the BI group, relative to a well-educated comparison group. For both groups, we found a large effect of learning technique so that response generation with corrective feedback (errorful learning) produced significantly more correct recognitions of Euskara translations, than errorless learning. In the BI group, this benefit for errorful learning occurred despite highly frequent memory and attentional lapses, and dysexecutive impairment. To explore a more targeted intervention for sub-groups with varying learning ability, learning slopes were extracted from repeat measures of word-pair recall, to distinguish learners from “poor learners” who showed “flat” slopes. Again, a large effect of learning technique showed a benefit for errorful learning techniques that was present for the learners, although poor learners showed no benefit and no cost of using an errorful technique compared with an errorless technique. As memory and dysexecutive impairment was distributed across both sub-groups post-injury, there was no strong basis for ruling in more severe memory impairment in the poor learners.
The primary aim of the present study was to evaluate the efficacy of two contrasting learning techniques in people living with cognitive impairment after BI. On the novel word learning task that used multiple-choice recognition, the BI group had depressed scores relative to the comparison group, with both groups performing above chance. This test does not differentiate between two distinct memory operations, those that generate cue-target associations and those implicated in single item recognition (e.g., Buchler et al., Citation2008). With healthy participants, Seabrooke et al. (Citation2019a) identified the locus of error as a failure to learn the cue and target in isolation, and not a difficulty in learning the cue-target associations on a variant of our word-learning task. Participants occasionally mentioned that they did not remember seeing the cue before but did point to the correct target. Therefore, the relative integrity of performance in both groups seemed linked to the familiarity of isolated cues and targets. That is, baseline recognition above chance could be relatively preserved familiarity through the involvement of peri-rhinal cortex (Aggleton & Brown, Citation1999) and/or engaging in pattern completion operations that support the reinstatement of the original item (Bussey & Saksida, Citation2005). Since our BI group also evidenced relative preservation of recognition (relative to delayed recall) on the VPA task, it was likely that recognition-oriented operations supported single item encoding to some extent. More support for this view comes from Arenth et al. (Citation2012) who observed familiarity-oriented operations during encoding elicited greater activity in MR imaging of a brain-injured group. In their study, controls generated more retrieval-oriented activity, but both groups achieved similar levels of performance using different operations (e.g., Arenth et al., Citation2012).
An impediment to new learning is a sequela of BI that is observed with varying degrees of memory impairment and executive dysfunction. We employed memory for word-pair associates to probe verbal learning, mirroring the cue-target pairings learned within the experimental task. Scores on all four trials of the VPA-immediate were relatively depressed for the BI group, although depressed scores in the BI group did not remain parallel to the comparison group over all trials. The comparison group had much greater learning gains for recall of familiar word-pairs over trials three and four, consistent with wider evidence of learning impairment after BI (De Luca et al., Citation2000; Strangman et al., Citation2008; Wright & Schmitter-Edgecombe, Citation2011). Critically, our manipulation of two techniques to support learning suggested that both groups benefited overall from the opportunity to generate target responses by guessing prior to receiving a correct translation (errorful learning). That is, errorful learning as a technique generated higher recognition accuracy in the final test of items that were foreign translations, compared to the errorless read-only technique. This converges with claims made by Potts and Shanks (Citation2014) insofar as guessing can improve target recognition, at least for neurologically intact adults. Importantly, this finding contradicts the current literature, and the advice for rehabilitation, that states that errorless learning is especially advantageous for individuals with anterograde amnesia (Baddeley & Wilson, Citation1994; Middleton & Schwartz, Citation2012).
Our data points to the benefits of errorful learning using a “Generate-and-correct” manipulation for recognition. Whilst item familiarity might play a role in supporting target recognition, the putative familiarity of errors also increases in errorful learning. One hypothesis in the wider literature is that the benefit for errorless learning (over “trial and error”) arises from the increased demands of distinguishing familiar errors (from targets) so that error monitoring is required to verify responses. That said, this advantage is most clearly observed for cued and associative recall measures, rather than recognition (e.g., Evans et al., Citation2000; Roberts et al., Citation2018). In our design, the likely impact of self-generating familiar errors was reduced in two ways. First, participants were presented with different alternative choices at test; this reduces the selection demands of competition from self-generated (familiar) errors. Second, participants self-generated only one response compared to multiple responses under “trial-and-error” conditions, for example, guessing on stem completion tasks. Our “Generate-and-correct” design has more in common with the first “short interval” retrieval opportunity in “spaced” or “practiced” retrieval (e.g., Haslam et al., Citation2011; Sumowski et al., Citation2010). From Haslam et al.’s data, the retrieval opportunities within spaced retrieval were more beneficial to face-name learning than errorless learning. Perhaps then, reducing the error load is critical to reducing the competition for selecting familiar candidates, post-retrieval.
Our second aim sought to distinguish learning profiles for more effective targeting of learning techniques in the BI group. The heterogeneity of learning outcomes is critical to supporting rehabilitation (Chiou et al., Citation2016; Mallas et al., Citation2021). In Chiou et al.’s study of moderate-to-severe BI, learners were differentiated from “non-learners” through their ability to reach a specific performance criterion. These non-learners had reduced white matter integrity, notably within right-sided, dorso-lateral PFC. In our analysis of VPA learning, “flat” regression slopes differentiated non-learners from learners. Only the learners benefited from the opportunity to generate “guess” responses. These findings are contrary to many previous studies that favour errorless learning (e.g., Middleton & Schwartz, Citation2012; Page et al., Citation2006; Wilson et al., Citation2010), even for the poor learners where no decrement of errorful learning was observed. Following Chiou et al., if impaired connectivity from dorso-lateral prefrontal to other cortical or subcortical regions (e.g., thalamus) in the non-learners impedes semantic regulation, then this sub-group could lack capacity for self-initiated elaborative semantic encoding to support “guessing”. In our study, despite one “poor learner” having excessively high omissions when asked to guess, people in both groups were mostly able to provide a guess response, implying the benefits of guessing are derived after the response, and not from an absence of a response. Exploratory analysis of executive-semantic cognition indicated that the poor learners in the BI group were worse on letter fluency and picture matching, with more severe impairment of delayed recall than learners. This was notable, as picture matching deficits contrasted with more typical preservation of semantic memory observed post-injury (e.g., Mallas et al., Citation2021).
More broadly, errorful learning provided specific benefits in the present study since novel verbal material was used (Potts & Shanks, Citation2014; Vannest et al., Citation2012). From earlier work with healthy adults, it is also clear that a more effortful encoding process results in better engagement with the task (Dunn & Clare, Citation2007; Seabrooke et al., Citation2019a; Tailby & Haslam, Citation2003). Previous studies report an advantage for self-generated responses (under constrained conditions of errorless learning) over simple repetition methods of learning (Laffan et al., Citation2010; Lubinsky et al., Citation2009). In Lubinsky et al.’s work with cases of amnestic MCI, self-generation of target words enhanced the benefit of errorless learning for cued recall and recognition, but not for item recognition. Of course, the capacity to generate an incorrect answer was problematic for some individuals in the current study, leading to slightly more failed attempts to guess in the BI group, but on the whole all participants were able to generate a guess on the majority of trials (with the exception of one BI participant who had 65% of trials without a guess), and even on trials with no guess generated, participants were still clearly engaging in the effortful process of trying to generate a guess. For example, participants were often still trying to generate a guess when they ran out of time. So, even though they failed to choose one word to generate, the covert operations underlying learning was still very much the same. As self-generation can be extended to errorful and errorless learning, future research should contrast the relative benefits of using self-generation within errorless learning, alongside “Generate-and-correct” in errorful learning to give more clarity over the factors critical for learning.
One candidate explanation of these covert operations is the additional-cue theory (Grimaldi & Karpicke, Citation2012; also, the Mediator Effectiveness hypothesis, Mera et al., Citation2022). On this account, the incorrect guess is the first target covertly recalled, and this “guess” then provides an additional cue for the correct target (Soraci et al., Citation1994). Indeed, participants made comments about multiple associations that led them to the answer. For example, the cue “untxi = ?” could remind the participant of their guess “taxi”, which reminds them of “myxy” (myxomatosis – a disease that affects rabbits), providing a cue to the correct target: “rabbit”. It is possible these additional (semantic) cues were compromised in poor learners. A second candidate explanation is that affective or motivational mediation of decisions during encoding gives rise to more effortful processing (Seabrooke et al., Citation2019b). That is, participants often stated that they did not know the correct answer, made a guess, and still scored highly, suggesting a covert operation that made one item more familiar to them – relying on a “gut feeling”. Affective decision making can lead to more accurate decisions, compared to deliberate decision making (Mikels et al., Citation2011). Finally, the apparent discrepancy between the guessed target and the correct target may be significant by using this discrepancy to cue attentional resources towards encoding (e.g., Kornell et al., Citation2009; Potts & Shanks, Citation2014; see also Error Prediction theory, Mera et al., Citation2022). A critical feature of the current design was the use of corrective feedback that could guide attentional resources to enhance encoding of the memory target and support item recognition in learners. Regardless of the exact operations, the benefits of errorful learning should not be overlooked in either healthy participants, or those with BI.
Our focus on recognition is a relative strength of this study, along with the more constrained error load and the use of corrective feedback to enable learning. However, there are significant limitations of the design that should not be overlooked in the translation to rehabilitation settings. First, our exclusion of other memory measures (such as cued recall), the absence of manipulations of corrective feedback or multiple retrieval opportunities make it unclear which factor was most critical. Second, our neuropsychological assessment battery was limited to quick and accessible screening tools that constrained a fuller understanding on the distinction between learners and poor learners. A more comprehensive investigation of executive cognition would allow more direct testing of the putative role of error monitoring in learning, compared to other measures. For example, Roberts et al. (Citation2018) found the errorless learning advantage diminished when the capacity for error monitoring increased in cases of amnestic MCI. Poor memory and executive dysregulation were characterizing features of our cognitive profile of the BI group, but the study lacked insight to the relative strengths and weaknesses across broader aspects, such as response inhibition, motivation and self-initiation, set-shifting and error monitoring. Although our BI group presented with executive (fluency) deficits, similar to Roberts et al.’s (Citation2018) data, verbal fluency only requires task monitoring and shifting attention to align with task instructions (without error correction). Although better error monitoring could be the basis for the advantage for errorful learning in learners, compared to poor learners, this is hypothetical at best. Our exploratory work on comparative profiling points to a broad distribution of cognitive impairment in both sub-groups, with the exception of executive-semantic cognition that appeared more impaired in poor learners. Future work should focus on whether poor learners were less able to benefit from elaborative semantic cues and corrective feedback to enhance encoding. Also, whether learners engaged in a monitoring process to enable selection from a small set of familiar and meaningful candidates, post-retrieval (see also Rodriguez-Fornells et al., Citation2004).
For rehabilitation purposes, the ability to self-initiate a guessed response in the first place seemed to enable an elaborative “retrieval” operation, possibly from additional cues (e.g., Carpenter, Citation2009; Citation2011; Soraci et al., Citation1994), that enhances the recognition of single target items without necessarily relying on associative memory. The role of cognitive effort is significant for enhancing memory, whether this invokes active monitoring of retrieval success and elimination of error, or incidental engagement with motivational and emotional reponses that influence metacognitive monitoring of the learning experience (Baumeister et al., Citation2007; Tailby & Haslam, Citation2003). In principle, a motivational account could also mediate learning in the brain-injured population. That is, people who have lived with substantial cognitive and memory impairment for a long period of time may not be as motivated to learn, as in a healthy sample. Patients with TBI could have low motivation for a wide variety of reasons, ranging from more apathy and depression as sequelae of their adaptive functioning to brain damage, to frontal lobe insult that directly affects goal setting and execution. As our data implied that even “poor learners” did generate guesses, our data points more to the relationship of learning with somatic fatigue and cognitive effort, rather than assuming motivation is poor. More insight to the integrity and functional connectivity of white matter after brain injury will help to clarify the role of cognitive effort and other operations that underpin errorful learning.
Memory and learning impairments, from mild to severe, are an obvious long-term sequela of BI that generate long-term needs for support and rehabilitation. The use of errorful learning facilitates a greater cognitive recovery in those with capacity to learn and did not generate significant costs for poor learners. Practitioners should be made aware that our findings did not provide support for widely advocated use of errorless learning with survivors of brain injury, at least for recognition; an individual’s learning capacity, and other factors (e.g., constraining error load and using corrective feedback) will influence learning outcomes. To conclude, it is clear that errorful learning can benefit broader aspects of recovery, whilst reducing the personal and economic costs of living with chronic injury (e.g., Novack et al., Citation2001; Skidmore, Citation2015). Given the increasing awareness of the impact of SARS-COVID-19 on brain health (e.g., Spudich & Nath, Citation2022), these findings also offer an accessible opportunity for tracking the long-term sequelae of acquired neurological insult from viral infection, with implications for supporting rehabilitation on a global scale.
errorful learning-supp_materials_resub.docx
Download MS Word (31.2 KB)Acknowledgements
We gratefully acknowledge the support of all clients, their families and the staff at Headway – the brain injury association (UK) who generously offered time and effort in support of this project. Headway is a registered charitable company whose mission is to promote understanding of all aspects of brain injury and provide information, support and services to survivors, their families and carers, as well as campaign to reduce the incidence of brain injury.
Disclosure statement
No potential conflict of interest was reported by the author(s).
Data availability statement
Data sets supporting the analyzes within the folder “open data” can be found at the Open Science Framework [https://osf.io/atcqe/?view_only=270107c0328648eb8fe9708b04c93a0e].
Additional information
Funding
References
- Adlam, A.-L. R., Patterson, K., Bozeat, S., & Hodges, J. R. (2010). The Cambridge semantic memory test battery: Detection of semantic deficits in semantic dementia and alzheimers disease. Neurocase, 16(3), 193–207. https://doi.org/10.1080/13554790903405693
- Aggleton, J. P., & Brown, M. W. (1999). Episodic memory, amnesia, and the hippocampal-anterior thalamic axis. The Behavioral and Brain Sciences, 22(3), 425–489.
- Alvarez, J. A., & Emory, E. (2006). Executive function and the frontal lobes: A meta-analytic review. Neuropsychology Review, 16, 17–42. https://doi.org/10.1007/s11065-006-9002-x
- Anderson, N. D., & Craik, F. I. M. (2006). The mnemonic mechanisms of errorless learning. Neuropsychologia, 44, 2806–2813. https://doi.org/10.1016/j.neuropsychologia.2006.05.026
- Arenth, P., Russell, K., Scanlon, J., Kessler, L., & Ricker, J. (2012). Encoding and recognition after traumatic brain injury: Neuropsychological and functional magnetic resonance imaging findings. Journal of Clinical And Experimental Neuropsychology, 34(4), 333–344. https://doi.org/10.1080/13803395.2011.633896
- Ariza, M., Serra-Grabulosa, J. M., Junqué, C., Ramírez, B., Mataró, M., Poca, A., & Sahuquillo, J. (2006). Hippocampal head atrophy after traumatic brain injury. Neuropsychologia, 44(10), 1956–1961. https://doi.org/10.1016/j.neuropsychologia.2005.11.007
- Baddeley, A., & Wilson, B. A. (1994). When implicit learning fails: Amnesia and the problem of error elimination. Neuropsychologia, 32(1), 53–68.
- Baumeister, R. F., Vohs, K. D., & Tice, D. M. (2007). The strength model of self-control. Current Directions in Psychological Science, 16(6), 351–355. https://doi.org/10.1111/j.1467-8721.2007.00534.x
- Bozeat, S., Ralph, M. A. L., Patterson, K., Garrard, P., & Hodges, J. R. (2000). Non-verbal semantic impairment in semantic dementia. Neuropsychologia, 38(9), 1207–1215. https://doi.org/10.1016/s0028-3932(00)00034-8
- Buchler, N. G., Light, L. L., & Reder, L. M. (2008). Memory for items and associations: Distinct representations and processes in associative recognition. Journal of Memory and Language, 59(2), 183–199. https://doi.org/10.1016/j.jml.2008.04.001
- Bussey, T. J., & Saksida, L. M. (2005). Object memory and perception in the medial temporal lobe: An alternative approach. Current Opinion in Neurobiology, 15, 1–8.
- Callahan, B. L., & Anderson, N. D. (2019). Effect of conceptual and lexical errorless versus trial-and-error learning in amnestic mild cognitive impairment. Neuropsychological Rehabilitation, 29(6), 969–982. https://doi.org/10.1080/09602011.2017.1361843
- Carpenter, S. K. (2009). Cue strength as a moderator of the testing effect: The benefits of elaborative retrieval. Journal of Experimental Psychology: Learning, Memory, and Cognition, 35(6), 1563–1569. https://doi.org/10.1037/a0017021
- Carpenter, S. K. (2011). Semantic information activated during retrieval contributes to later retention: Support for the mediator effectiveness hypothesis of the testing effect. Journal of Experimental Psychology: Learning, Memory, and Cognition, 37(6), 1547–1552. https://doi.org/10.1037/a0024140
- Chiou, K. S., Genova, H. M., & Chiaravalloti, N. D. (2016). Structural white matter differences underlying heterogeneous learning abilities after TBI. Brain Imaging and Behavior, 10, 1274–1279. https://doi.org/10.1007/s11682-015-9497
- Clare, L., & Jones, R. S. (2008). Errorless learning in the rehabilitation of memory impairment: A critical review. Neuropsychology Review, 18(1), 1–23. https://doi.org/10.1007/s11065-008-9051-4
- Clark, L. R., Stricker, N. H., Libon, D. J., Delano-Wood, L., Salmon, D. P., Delis, D. C., & Bondi, M. W. (2012). Yes/no versus forced-choice recognition memory in mild cognitive impairment and Alzheimer's disease: Patterns of impairment and associations with dementia severity. The Clinical Neuropsychologist, 26(7), 1201–1216. https://doi.org/10.1080/13854046.2012.728626
- Cohen, M., Ylvisaker, M., Hamilton, J., Kemp, L., & Claiman, B. (2010). Errorless learning of functional life skills in an individual with three aetiologies of severe memory and executive function impairment. Neuropsychological Rehabilitation, 20(3), 355–376. https://doi.org/10.1080/09602010903309401
- Crawford, J. R., Garthwaite, P. H., Sutherland, D., & Borland, N. (2011). Some supplementary methods for the analysis of the delis–kaplan executive function system. Psychological Assessment, 23, 888–898. https://doi.org/10.1037/a0023712
- Delis, D., Freeland, J., Kramer, J., & Kaplan, E. (1988). Integrating clinical assessment with cognitive neuroscience: Construct validation of the California verbal learning test. Journal of Consulting And Clinical Psychology, 56(1), 123–130. https://doi.org/10.1037//0022-006x.56.1.123
- Delis, D. C., Kaplan, E., & Kramer, J. H. (2001). Delis-Kaplan executive function system. PsycTESTS Dataset. https://doi.org/10.1037/t15082-000.
- De Luca, J., Schultheis, M. T., Madigan, N. K., Christodoulou, C., & Averill, A. (2000). Acquisition versus retrieval deficits in traumatic brain injury: Implications for memory rehabilitation. Archives of Physical Medicine and Rehabilitation, 81(10), 1327–1333. https://doi.org/10.1053/apmr.2000.9390
- Dikmen, S. S., Corrigan, J. D., Levin, H. S., Machamer, J., Stiers, W., & Weisskopf, M. G. (2009). Cognitive outcome following traumatic brain injury. Journal of Head Trauma Rehabilitation, 24, 430–438. https://doi.org/10.1097/HTR.0b013e3181c133e9
- Dunn, J., & Clare, L. (2007). Learning face–name associations in early-stage dementia: Comparing the effects of errorless learning and effortful processing. Neuropsychological Rehabilitation, 17(6), 735–754. https://doi.org/10.1080/09602010701218317
- Evans, J. J., Wilson, B. A., Schuri, U., Andrade, J., Baddeley, A., Bruna, O., & Taussik, I. (2000). A comparison of “errorless” and “trial-and-error” learning methods for teaching individuals with acquired memory deficits. Neuropsychological Rehabilitation, 10(1), 67–101.
- Faul, M., Wald, M. M., Rutland-Brown, W., Sullivent, E. E., & Sattin, R. W. (2007). Using a cost-benefit analysis to estimate outcomes of a clinical treatment guideline: Testing the brain trauma foundation guidelines for the treatment of severe traumatic brain injury. The Journal of Trauma and Acute Care Surgery, 63(6), 1271–1278. https://doi.org/10.1097/TA.0b013e3181493080
- Gifford, K. A., Phillips, J. S., Samuels, L. R., Lane, E. M., Bell, S. P., Liu, D., Hohman, T. J., Romano, R., Fritzsche, R., R, L., Lu, Z., & Jefferson, A. L. (2015). Associations between verbal learning slope and neuroimaging markers across the cognitive aging spectrum. Journal of the International Neuropsychological Society, 21(6), 455–467. https://doi.org/10.1017/s1355617715000430
- Gillis, M. M., & Hampstead, B. M. (2015). A two-part preliminary investigation of encoding-related activation changes after moderate to severe traumatic brain injury: Hyperactivation, repetition suppression, and the role of the prefrontal cortex. Brain Imaging and Behavior, 9, 801–820. https://doi.org/10.1007/s11682-014-9337-5
- Giovanello, K. S., Keane, M. M., & Verfaellie, M. (2006). The contribution of familiarity to associative memory in amnesia. Neuropsychologia, 44(10), 1859–1865. https://doi.org/10.1016/j.neuropsychologia.2006.03.004
- Glisky, E. L., Schacter, D. L., & Tulving, E. (1986). Computer learning by memory-impaired patients: Acquisition and retention of complex knowledge. Neuropsychologia, 24(3), 313–328. https://doi.org/10.1016/0028-3932(86)90017-5
- Grimaldi, P. J., & Karpicke, J. D. (2012). When and why do retrieval attempts enhance subsequent encoding? Journal of Applied Research in Memory and Cognition, 40(4), 505–513. https://doi.org/10.3758/s13421-011-0174-0
- Haslam, C., Hodder, K. I., & Yates, P. J. (2011). Errorless learning and spaced retrieval: How do these methods fare in healthy and clinical populations? Journal of Clinical and Experimental Neuropsychology, 33, 432–447. https://doi.org/10.1080/13803395.2010.533155
- Headway. (2023). Retrieved January 31, 2023, from www.headway.org.uk/about-brain-injury/further-information/statistics/statistics-resources/
- Hildebrandt, H. (2019). Cognitive Rehabilitation of Memory: A Clinical-Neuropsychological Introduction (pp. 71–147). Academic Press. https://doi.org/10.1016/C2018-0-00397-7
- Hodges, J. R., & Patterson, K. (1997). Semantic memory disorders. Trends in Cognitive Sciences, 1(2), 68–72. https://doi.org/10.1016/s1364-6613(97)01022-x
- Huelser, B. J., & Metcalfe, J. (2012). Making related errors facilitates learning, but learners do not know it. Journal of Applied Research in Memory and Cognition, 40(4), 514–527. https://doi.org/10.3758/s13421-011-0167-z
- Hunkin, N. M., Squires, E. J., Parkin, A. J., & Tidy, J. A. (1998). Are the benefits of errorless learning dependent on implicit memory? Neuropsychologia, 36, 25–36.
- Jennett, B., Snoek, J., Bond, M., & Brooks, N. (1981). Disability after severe head injury: Observations on the use of the Glasgow outcome scale. Journal of Neurology, Neurosurgery, and Psychiatry, 44(4), 285–293. https://doi.org/10.1136/jnnp.44.4.285
- Julayanont, P., Phillips, N., Chertkow, H., & Nasreddine, Z. S. (2012). Montreal cognitive assessment (MoCA): concept and clinical review. Cognitive Screening Instruments, 111–151. https://doi.org/10.1007/978-1-4471-2452-8_
- Kornell, N., Hays, M. J., & Bjork, R. A. (2009). Unsuccessful retrieval attempts enhance subsequent learning. Journal of Experimental Psychology: Learning, Memory and Cognition, 35(4), 989–998. https://doi.org/10.1037/a0015729
- Laffan, A. J., Metzler-Baddeley, C., Walker, I., & Jones, R. W. (2010). Making errorless learning more active: Self-generation in an error free learning context is superior to standard errorless learning of face-name associations in people with Alzheimer's disease. Neuropsychological Rehabilitation, 20, 197–211.
- Little, D. M., Kraus, M. F., Joseph, J., Geary, E. K., Susmaras, T., Zhou, X. J., Pliskin, N., & Gorelick, P. B. (2010). Thalamic integrity underlies executive dysfunction in traumatic brain injury. Neurology, 74(7), 558–564. https://doi.org/10.1212/WNL.0b013e3181cff5d5
- Lloyd, J., Riley, G., & Powell, T. (2009). Errorless learning of novel routes through a virtual town in people with acquired brain injury. Neuropsychological Rehabilitation, 19(1), 98–109. https://doi.org/10.1080/09602010802117392
- Lubinsky, T., Rich, J. B., & Anderson, N. D. (2009). Errorless learning and elaborative self-generation in healthy older adults and individuals with amnestic mild cognitive impairment: Mnemonic benefits and mechanisms. Journal of the International Neuropsychological Society, 15, 704–716. https://doi.org/10.1017/S1355617709990270
- Mallas, E.-J., De Simoni, S., Scott, G., Jolly, A.-E., Hampshire, A., Li, L. M., Bourke, N., Roberts, S. A. G., Gorgoraptis, N., & Sharp, D. J. (2021). Abnormal dorsal attention network activation in memory impairment after traumatic brain injury. Brain, 144, 114–127. https://doi.org/10.1093/brain/awaa380
- McKissock, S., & Ward, J. (2007). Do errors matter? Errorless and errorful learning in anomic picture naming. Neuropsychological Rehabilitation, 17(3), 355–373. https://doi.org/10.1080/09602010600892113
- Mera, Y., Rodríguez, G., & Marin-Garcia, E. (2022). Unravelling the benefits of experiencing errors during learning: Definition, modulating factors, and explanatory theories. Psychonomic Bulletin and Review, 29, 753–765. https://doi.org/10.3758/s13423-021-02022-8
- Metcalfe, J., & Xu, J. (2018). Learning from one’s own errors and those of others. Psychonomic Bulletin and Review, 25, 402–408. https://doi.org/10.3758/s13423-017-1287-7
- Metzler-Baddeley, C., & Snowden, J. S. (2005). Brief report: Errorless versus errorful learning as a memory rehabilitation approach in Alzheimer's disease. Journal of Clinical and Experimental Neuropsychology, 27(8), 1070–1079. https://doi.org/10.1080/13803390490919164
- Middleton, E. L., & Schwartz, M. F. (2012). Errorless learning in cognitive rehabilitation: A critical review. Neuropsychological Rehabilitation, 22(2), 138–168. https://doi.org/10.1080/09602011.2011.639619
- Mikels, J. A., Maglio, S. J., Reed, A. E., & Kaplowitz, L. J. (2011). Should I go with my gut? Investigating the benefits of emotion-focused decision making. Emotion, 11(4), 743–753. https://doi.org/10.1037/a0023986
- Nasreddine, Z., Phillips, N., Bedirian, V., Charbonneau, S., Whitehead, V., Collin, I., Cummings, J.L., Chertkow, H. (2005). The Montreal cognitive assessment, MoCA: A brief screening tool for mild cognitive impairment. Journal of The American Geriatrics Society, 53(4), 695–699. https://doi.org/10.1111/j.1532-5415.2005.53221.x
- Nelson, H., & Willison, J. (1991). National adult Reading test (NART) test manual (part II).. NFER-NELSON.
- Nettelbeck, T., Rabbitt, P., Wilson, C., & Batt, R. (1996). Uncoupling learning from initial recall: The relationship between speed and memory deficits in old age. British Journal of Psychology, 87(4), 593–607. https://doi.org/10.1111/j.2044-8295.1996.tb02610.x
- Novack, T. A., Bush, B. A., Meythaler, J. M., & Canupp, K. (2001). Outcome after traumatic brain injury: Pathway analysis of contributions from premorbid, injury severity, and recovery variables. Archives of Physical Medicine and Rehabilitation, 82(3), 300–305. https://doi.org/10.1053/apmr.2001.18222
- Overman, A. A., Stephens, J. D. W., & Bernhadt, M. F. (2021). Enhanced memory for context associated with corrective feedback: Evidence for episodic processes in errorful learning. Memory (Hove, England), 29, 1017–1042. https://doi.org/10.1080/09658211.2021.1957937
- Ownsworth, T., Fleming, J., Tate, R., Shum, D., Griffin, J., Schmidt, J., Lane-Brown, A., Kendall, M., & Chevignard, M. (2013). Comparison of error-based and errorless learning for people with severe traumatic brain injury: Study protocol for a randomized control trial. Trials, 14(1), 369. https://doi.org/10.1186/1745-6215-14-369
- Ownsworth, T., Quinn, H., Fleming, J., Kendall, M., & Shum, D. (2010). Error self-regulation following traumatic brain injury: A single case study evaluation of metacognitive skills training and behavioural practice interventions. Neuropsychological Rehabilitation, 20(1), 59–80. https://doi.org/10.1080/09602010902949223
- Page, M., Wilson, B. A., Shiel, A., Carter, G., & Norris, D. (2006). What is the locus of the errorless-learning advantage? Neuropsychologia, 44(1), 90–100. https://doi.org/10.1016/j.neuropsychologia.2005.04.004
- Palacios, E. M., Sala-Llonch, R., Junque, C., Roig, T., Tormos, J. M., Bargallo, N., & Vendrell, P. (2013). Resting-State functional magnetic resonance imaging activity and connectivity and cognitive outcome in traumatic brain injury. JAMA Neurology, 70, 845–851. https://doi.org/10.1001/jamaneurol.2013.38
- Panwar, N., Purohit, D., Sinha, V. D., & Joshi, M. (2019). Evaluation and extent of neurocognitive functions in mild and moderate traumatic brain injury patients by using Montreal cognitive assessment (MoCA) score as a screening tool: An observational study from India. Asian Journal of Psychiatry, 41, 60–65. https://doi.org/10.1016/j.ajp.2018.08.007
- Pitel, A. L., Beaunieux, H., Lebaron, N., Joyeux, F., Desgranges, B., & Eustache, F. (2006). Two case studies in the application of errorless learning techniques in memory impaired patients with additional executive deficits. Brain Injury, 20, 1099–1110. https://doi.org/10.1080/02699050600909961
- Polinder, S., Cnossen, M. C., Real, R. G. L., Covic, A., Gorbunova, A., Voormolen, D. C., Master, C. L., Haagsma, J. A., Diaz-Arrastia, R., & von Steinbuechel, N. (2018). A multidimensional approach to post-concussion symptoms in mild traumatic brain injury. Frontiers in Neurology, 9, 1113. https://doi.org/10.3389/fneur.2018.01113
- Potts, R., & Shanks, D. R. (2014). The benefit of generating errors during learning. Journal of Experimental Psychology: General, 143(2), 644–667. https://doi.org/10.1037/a0033194
- Ramage, A. E., Tate, D. F., New, A. B., Lewis, J. D., & Robin, D. A. (2019). Effort and fatigue-related functional connectivity in mild traumatic brain injury. Frontiers in Neurology, 9, 1–11. https://doi.org/10.3389/fneur.2018.01165
- Raven, J., Raven, J. C., & Court, J. H. (1998). Raven manual: Section 4, advanced progressive matrices, 1998 edition. Oxford Psychologists Press.
- Rescorla, R. A., & Wagner, A. R. (1972). A theory of pavlovian conditioning: Variations in the effectiveness of reinforcement and nonreinforcement. In A. H. Black, & W. F. Prokasy (Eds.), Classical conditioning II: Current research and theory (pp. 66–99). Appleton Century Crofts.
- Roberts, J. L., Anderson, N. D., Guild, E., Cyr, A. A., Jones, R. S. P., & Clare, L. (2018). The benefits of errorless learning for people with amnestic mild cognitive impairment. Neuropsychological Rehabilitation, 28, 984–996. https://doi.org/10.1080/09602011.2016.1216000
- Rodriguez-Fornells, A., Kofidis, C., & Münte, T. F. (2004). An electrophysiological study of errorless learning. Cognitive Brain Research, 19, 160–173.
- Rosenfeld, J. V., Maas, A. I., Bragge, P., Morganti-Kossmann, M. C., Manley, G. T., & Gruen, R. L. (2012). Early management of severe traumatic brain injury. Lancet, 380(9847), 1088–1098. https://doi.org/10.1016/s0140-6736(12)60864-2
- Royle, J., & Lincoln, N. B. (2008). The everyday memory questionnaire-revised: Development of a 13-item scale. Disability Rehabilitation, 30(2), 114–121. https://doi.org/10.1080/09638280701223876
- Ruet, A., Bayen, E., Jourdan, C., Ghout, I., Meaude, L., Lalanne, A., … Azouvi, P. (2019). A detailed overview of long-term outcomes in severe traumatic brain injury eight years post-injury. Frontiers in Neurology, 10, 120.
- Schmidt, S. R. (1990). A test of resource-allocation explanations of the generation effect. Bulletin of the Psychonomic Society, 28(2), 93–96. https://doi.org/10.3758/BF03337658
- Seabrooke, T., Hollins, T. J., Kent, C., Wills, A. J., & Mitchell, C. J. (2019a). Learning from failure: Errorful generation improves memory for items, not associations. Journal of Memory and Language, 104, 70–82. https://doi.org/10.1016/j.jml.2018.10.001
- Seabrooke, T., Mitchell, C. J., & Hollins, T. J. (2021). Pretesting boosts item but not source memory. Memory (Hove, England), 29, 1245–1253. https://doi.org/10.1080/09658211.2021.1977328
- Seabrooke, T., Mitchell, C. J., Wills, A. J., Waters, J. L., & Hollins, T. J. (2019b). Selective effects of errorful generation on recognition memory: The role of motivation and surprise. Memory (Hove, England), 27(9), 1250–1262. https://doi.org/10.1080/09658211.2019.1647247
- Shah, S. A., Goldin, Y., Conte, M. M., Goldfine, A. M., Mohamadpour, M., Fidali, B. C., Cicerone, K., & Schiff, N. D. (2017). Executive attention deficits after traumatic brain injury reflect impaired recruitment of resources. NeuroImage: Clinical, 14, 233–241. https://doi.org/10.1016/j.nicl.2017.01.010
- Shao, Z., Janse, E., Visser, K., & Meyer, A. S. (2014). What do verbal fluency tasks measure? Predictors of verbal fluency performance in older adults. Frontiers in Psychology, 5. https://doi.org/10.3389/fpsyg.2014.00772
- Skidmore, E. R. (2015). Training to optimize learning after traumatic brain injury. Current Physical Medicine and Rehabilitation Reports, 3(2), 99–105. https://doi.org/10.1007/s40141-015-0081-6
- Slamecka, N. J., & Graf, P. (1978). The generation effect: Delineation of a phenomenon. Journal of Experimental Psychology: Human Learning and Memory, 4(6), 592–604. https://doi.org/10.1037/0278-7393.4.6.592
- Sohlberg, M. M., McLaughlin, K. A., Pavese, A., Heidrich, A., & Posner, M. I. (2000). Evaluation of attention process training and brain injury education in persons with acquired brain injury. Journal of Clinical and Experimental Neuropsychology, 22(5), 656–676. https://doi.org/10.1076/1380-3395(200010)
- Soraci, S. A., Jr., Franks, J. J., Bransford, J. D., Chechile, R. A., Belli, R. F., Carr, M., & Carlin, M. (1994). Incongruous item generation effects: A multiple-cue perspective. Journal of Experimental Psychology: Learning, Memory and Cognition, 20(1), 67–78.
- Spudich, S., & Nath, A. (2022). Nervous system consequences of COVID-19. Science, 375, 6578. https://doi.org/10.1126/science.abm2052
- Squires, E. J., Hunkin, N. M., & Parkin, A. J. (1997). Errorless learning of novel associations in amnesia. Neuropsychologia, 35(8), 1103–1101.
- Strangman, G., O'Neil-Pirozzi, T., Goldstein, R., Kelkar, K., Katz, D., Burke, D., Rauch, S., Savage, C., & Glenn, M. (2008). Prediction of memory rehabilitation outcomes in traumatic brain injury by using functional magnetic resonance imaging. Archives of Physical Medicine and Rehabilitation, 89(5), 974–981. https://doi.org/10.1016/j.apmr.2008.02.011
- Sumowski, J., Wood, H., Chiaravalloti, N., Wylie, G., Lengenfelder, J., & Deluca, J. (2010). Retrieval practice: A simple strategy for improving memory after traumatic brain injury. Journal of the International Neuropsychological Society, 16(6), 1147–1150. https://doi.org/10.1017/S1355617710001128
- Tailby, R., & Haslam, C. (2003). An investigation of errorless learning in memory-impaired patients: Improving the technique and clarifying theory. Neuropsychologia, 41(9), 1230–1240. https://doi.org/10.1016/S0028-3932(03)00036-8
- Terrace, H. S. (1963). Errorless transfer of a discrimination across two continua. Journal of the Experimental Analysis of Behavior, 6(2), 223–232. https://doi.org/10.1901/jeab.1963.6-223
- Thompson-Schill, S. L., D'Esposito, M., Aguirre, G. K., & Farah, M. J. (1997). Role of left inferior prefrontal cortex in retrieval of semantic knowledge: A reevaluation. Proceedings of the National Academy of Sciences, 94(26), 14792–14797. https://doi.org/10.1073/pnas.94.26.14792
- Tulving, E. (1985). Memory and consciousness. Canadian Psychology/Psychologie Canadienne, 26(1), 12. https://doi.org/10.1037/h0080017
- Vakil, E. (2007). The effect of moderate to severe traumatic brain injury (TBI) on different aspects of memory:A selective review. Journal of Clinical and Experimental Neuropsychology, 27(8), 977–1021. https://doi.org/10.1080/13803390490919245
- Vakil, E., Greenstein, Y., Weiss, I., & Shtein, S. (2019). The effects of moderate-to-severe traumatic brain injury on episodic memory: A meta-analysis. Neuropsychology Review, 29(3), 270–287. https://doi.org/10.1007/s11065-019-09413-8
- Vannest, J., Eaton, K. P., Henkel, D., Siegel, M., Tsevat, R. K., Allendorfer, J. B., & Szaflarski, J. P. (2012). Cortical correlates of self-generation in verbal paired associate learning. Brain Research, 1437, 104–114. https://doi.org/10.1016/j.brainres.2011.12.020
- Visser, M. A. L., & Lambon-Ralph, M. A. (2011). Differential contributions of bilateral ventral anterior temporal lobe and left anterior superior temporal gyrus to semantic processes. Journal of Cognitive Neuroscience, 23(10), 3121–3131. https://doi.org/10.1162/jocn_a_00007
- Wechsler Memory Scale-Fourth Edition. (2009). PsycTESTS dataset. https://doi.org/10.1037/t15175-000.
- Wilson, B. A., Baddeley, A., Evans, J., & Shiel, A. (2010). Errorless learning in the rehabilitation of memory impaired people. Neuropsychological Rehabilitation, 4(3), 307–326. https://doi.org/10.1080/09602019408401463
- Wright, M. J., & Schmitter-Edgecombe, M. (2011). The impact of verbal memory encoding and consolidation deficits during recovery from moderate-to-severe traumatic brain injury. Journal of Head Trauma Rehabilitation, 26(3), 182–191. https://doi.org/10.1097/HTR.0b013e318218dcf9
- Yonelinas, A. P. (2002). The nature of recollection and familiarity: A review of 30 years of research. Journal of Memory and Language, 46(3), 441–517. https://doi.org/10.1006/jmla.2002.2864
- Zawadzka, K., & Hanczakowski, M. (2019). Two routes to memory benefits of guessing. Journal of experimental psychology: Learning. Memory, and Cognition, 45(10), 1748–1760. https://doi.org/10.1037/xlm0000676