Abstract
The Water Framework Directive (WFD) provides the legal basis for water management in the European Union (EU). Twelve years after it was passed, all but five EU Member States had phytobenthos assessment methods for rivers, whilst nine had methods for lakes. Most are based on diatoms, although a few are supplemented by evaluations of non-diatoms and some include macroalgae as part of parallel macrophyte assessments. Norway is the exception, with assessment based on non-diatom algae alone. Over half of all states have methods based wholly or partly on weighted average metrics developed before the onset of the WFD, with nine choosing the Indice de Polluosensibilité Specifique. Such metrics generally have high correlations with the predominant nutrient or organic pollution gradient and, as such, represent pragmatic solutions to ecological status assessment. However, their widespread use raises questions about what, exactly, ‘ecological status’ means. Strong relationships with chemical pressure gradients may be a mixed blessing as pressure gradients are often composed of several intercorrelated variables, making it difficult to disentangle correlation and causation in the absence of ecophysiological studies of individual species. Moreover, the focus on strong relationships with chemical gradients means that most phytobenthos metrics describe the scale of hazard at a site rather than the risk posed to other trophic levels and to ecosystem services. This first generation of phytobenthos assessment tools may be inadequate when catchment managers need guidance on remediation strategies for particular water bodies. A second generation of assessment tools, focused on the fitness of the phytobenthos as part of aquatic ecosystems, rather than just as indicators of chemical conditions, is needed if the goal of good ecological status around Europe is to be achieved.
Introduction: the Water Framework Directive in context
The Water Framework Directive (WFD: European Union, Citation2000), the legal basis for water management across the 27 states of the European Union, exists in a grey area between science, bureaucracy and the law. As the WFD requires member states to assess the state of rivers and lakes using a number of parameters, one of which is ‘phytobenthos’ (benthic algae), much new research has been published since its introduction. This review addresses this research partly in terms of how much more we know than in 2000 when the WFD was published, and partly in terms of how this science dovetails with the broader legal, bureaucratic and political climate. It asks, in other words, if this research is ‘fit-for-purpose’ when faced with the enormous challenges of improving Europe’s surface waters. It is not an exhaustive review of all papers published on phytobenthos assessment, as the unique demands of the WFD create a de facto filter that renders many valid scientific studies inappropriate for practical assessment in Europe. However, the references should enable readers to locate this broader literature.
The objective of the WFD is to protect inland and coastal waters and promote sustainable water use in Europe (Article 1) with ‘ecological status’ used as a benchmark against which progress towards this objective is assessed. Ecological status is defined as “an expression of the quality of the structure and functioning of aquatic ecosystems associated with surface waters …” (Article 2). This assumes that a healthy ecosystem is a good indicator that a water body is being exploited in a sustainable manner. Ecological status is a holistic concept, not confined to any single organism group (or ‘biological quality elements’ [BQEs] in the terminology of the WFD); the use of “functioning” in the definition suggests a need to understand both the state of a BQE and its interactions with other BQEs and the catchment. For rivers and lakes, four BQEs are defined, one of which is “macrophytes and phytobenthos”, although most member states have treated these as two separate sub-elements, with diatoms frequently employed as representatives of the phytobenthos.
These BQEs are used to place all water bodies into one of five ecological status classes, depending on the degree of deviation from the unimpacted “reference state” (). “Good ecological status” (GES) is defined as no more than a slight change from this reference state. If the water body fails to attain this, the WFD stipulates that a “programme of measures” needs to be applied in order to restore it to good ecological status. The science of the WFD therefore needs to be evaluated in terms of its ability to place a water body reliably into the appropriate status class and, if this is lower than good status, to guide catchment managers on the appropriate remediation steps. The WFD also stipulates that costs for water services need to be recovered in accordance with the ‘polluter pays principle’ (Article 9) which, as urban wastewater treatment and agriculture are major stresses, will result in knock-on costs for the entire population of Europe. On the other hand, the WFD also permits some discretion, since it gives the option of setting “less stringent objectives” (LSOs), if achieving good ecological status is deemed to be disproportionately expensive (Article 4 clause 5). Finally, Article 14 (“Public information and consultation”) encourages the “active involvement of all interested parties…” and an open and transparent approach to decision-making. In other words, we need to be able to explain the rationale behind any decision, and justify why citizens should have to pay higher costs for their water services.
Table 1. Normative definitions of ecological status for lakes and rivers. The general definitions refer to all biological quality elements in all water body types; definitions for ‘macrophytes and phytobenthos’ apply just to lakes and rivers. From Annex V of the WFD (European Union, Citation2000).
However, all of this needs to be placed into a broader political framework: the EU operates on a system of governance known as ‘subsidiarity’, which leaves the details of implementation up to individual member states. This means that two neighbouring states might have completely different (but equally valid) approaches to evaluating ecological status. In a few cases (e.g. Belgium), responsibility is delegated to regional governments within the state. Taxation policies vary between member states, leading to differences in the budgets available to public sector organisations such as environmental regulators. Finally, much of Europe is engulfed in a financial crisis with far-reaching ramifications for public spending, and investment. How does this change the way in which ecological tools should be evaluated?
Algal-based monitoring in Europe prior to the WFD
Algae have been used for environmental assessment since the 19th century (Hassall, Citation1850; Kolkwitz & Marsson, Citation1908), with a large literature developing from the 1970s onwards (reviewed by Cairns, Citation1980; Shubert, Citation1984; Whitton & Kelly, Citation1995; Rimet, Citation2012). Evaluation of this literature is complicated as it is not always easy to differentiate between papers that point out the theoretical potential of algae for environmental assessment and those that demonstrate integration of such approaches into a decision-making process.
The use of palaeoecology to establish the causes of acidification in north-west Europe stands as a case in point: an elegant demonstration of a link between declining ecological quality in softwater areas and atmospheric deposition of sulphur and nitrogen compounds (Renberg & Hellberg, Citation1982; Flower & Battarbee, Citation1983; Jones et al., Citation1989). This work directly influenced environmental policy in the UK, leading to tighter regulations on atmospheric emissions (Derwent & Wilson, Citation2012). Ongoing monitoring then demonstrated a measure of recovery in several of the original lakes (Lancaster et al., Citation1996; Battarbee et al., Citation2012). This, and similar work in north-east USA (Charles & Whitehead, Citation1986), was also significant in two other ways: first, because it demonstrated the tight coupling between diatom assemblages and their chemical environment, and second, because it showed that it was possible both to hindcast change by calibrating historical datasets against contemporary spatial datasets, and to predict the benefits of restoration strategies.
At about the same time, Descy (Citation1979) and Lange-Bertalot (Citation1979) were looking at ways in which diatoms could be used to assess river quality. Descy (Citation1979) adopted the weighted average equation of Zelinka & Marvan (Citation1961) to establish an index, which was calibrated against a gradient extending from unpolluted to heavily polluted rivers in Belgium. This approach was further developed by Coste (in CEMAGREF, Citation1982) into the Indice de Polluosensebilité Specifique (IPS) which, as we shall see, remains very popular. The IPS itself was adapted and adopted by the Agence de l’Eau Artois-Picardie in northern France as part of their routine environmental assessments (Prygiel & Coste, Citation1993). In order to make calculations more straightforward, a software package, Omnidia, was developed (Lecointe et al., Citation1993). As this includes not just the IPS but also other indices, it has become a popular means by which diatomists throughout Europe could check the potential of existing indices on their own datasets.
The situation changed in 1991 with the enactment of the Urban Wastewater Treatment Directive (UWWTD, European Community, Citation1991). This dealt with many aspects of sewage discharges to the marine and freshwater environments but included a specific requirement for phosphorus stripping to be installed at large sewage treatment works (STWs), if there was a risk of eutrophication arising from the discharge. This persuaded regulators to look again at methods that directly assessed the photosynthetic biota, and led to the development of a new generation of indices, optimized against an inorganic nutrient, rather than a ‘general pollution’ gradient (Kelly & Whitton, Citation1995; Coring et al., Citation1999; Rott et al., Citation1999). This more widespread use of diatoms also stimulated efforts to harmonize methods (Kelly et al., Citation1998; CEN, Citation2003, Citation2004).
By the close of the 20th century, therefore, phytobenthos assessment was well established in Europe, not just in the academic literature but also in agencies responsible for environmental regulation in several countries. It was based almost entirely, however, on diatoms (with a few exceptions, e.g. Austria: Rott et al., Citation1999) although some countries included larger benthic algae such as Cladophora and Vaucheria as part of their macrophyte assessment systems (see below). Moreover, phytobenthos assessment was establishing a particular role within the monitoring toolkits of these countries: focusing particularly on pressures such as eutrophication, and complementing, rather than competing with, the information regulators gained from other organisms.
How Europe’s states approach phytobenthos assessment for the WFD
Reference conditions and typologies
The major change that the WFD brought to ecological assessment in Europe was the requirement to express results in terms of deviation from an ideal state, termed the ‘reference’ condition, for a particular type of water body. The WFD itself is vague on the subject of reference conditions, referring to these only as “… representing the values of the biological quality elements … for that surface water body type at high ecological status …” (Annex 2, clause 1.3). The phrase “no, or only very minor, anthropogenic alterations” in the definition of high ecological status () offers many possible interpretations: it is certainly not the unambiguous statement of ‘pristine’ conditions that some (Moss, Citation2008) claim. The European landscape shows evidence of anthropogenic alteration extending back 9000 years (Behre, Citation1988) and very few aquatic sites may be said to be truly ‘unimpacted’ as a result. Reference conditions provide the theoretical end-points for potentially expensive ecosystem restoration projects and so need to be rooted in datasets large enough to provide robust inferences, necessitating a trade-off between the purity of the reference concept and pragmatism.
In environments where sediments accumulate, palaeolimnology should be an ideal tool for establishing reference conditions (Bennion et al., Citation2004; Clarke et al., Citation2006). However, diatoms (the most widely used taxonomic group in palaeolimnology) span two BQEs – phytoplankton and phytobenthos – although palaeolimnological methods do not coincide exactly with current assessment methods for either of these individually. Moreover, approaches to determining reference conditions need to be applicable across all BQEs in all water body types whilst many organisms do not preserve well and some habitat types do not allow sediments to accumulate. Palaeolimnology continues to provide many insights and allows reference concepts to be tested (Bennion et al., Citation2004); however, contemporary data from spatial networks of minimally disturbed sites have become the preferred basis for establishing reference conditions. The core principle is that reference conditions are defined by the absence of pressure rather than by the presence of a particular assemblage of organisms. Pressures are assessed as far as possible from land use data, using ‘no observable effect’ thresholds derived from the literature (Pardo et al., Citation2012; Feio et al., Citation2013). This is a pragmatic interpretation of the requirement for “no, or very minor … alteration” yet, even so, some countries can find no such sites in their territory or for certain water body types, necessitating alternative forms of validation (Vogel et al., Citation2005; Baattrup-Pedesen et al., 2008; Denys, Citation2009; Yallop et al., Citation2009; Kelly et al., Citation2012).
The search for a meaningful interpretation of reference conditions epitomizes many of the tensions inherent in WFD implementation. Mathematically, reference conditions provide the denominator in Ecological Quality Ratio (EQR) calculations (see below). Consequently, it is important that all member states share a common concept as this, in effect ‘anchors’ ecological status assessments, regardless of the type of water body or the region of Europe, at a common (and measurable) point on the impact scale. Yet reference conditions also provide us with a ‘vision’, albeit idealized, of how aquatic communities should function. Because some of man’s impacts on the landscape are irreversible, it is best to view reference conditions, in most cases, as ‘plagioclimaxes’ (Tansley, Citation1935), rather than searching for a prelapsarian ‘undisturbed’ state, as in Moss (Citation2008).
The WFD requires member states to express ecological status as an EQR, which is the observed value for a biological metric divided by the expected value (derived from values obtained from sites at reference conditions). This means that all ecological status assessments are expressed on a common scale, 0–1. EQR = 1 indicates observed = expected, whilst lower values indicate increasing levels of deviation from this state. In practice, most ‘expected’ values are means or medians of data from reference sites, meaning that values >1 have to be rounded down. A few methods use upper percentiles of reference datasets but such values are inherently less stable unless based on large datasets. Values of metrics at reference conditions will themselves vary depending upon physical and geological conditions (Tison et al., Citation2005), necessitating the establishment of ‘types’ within each category of water body (lakes, rivers etc.).
Assessment methods
All member states have now had over a decade in which to develop their own national assessment methods. These vary considerably, reflecting both biogeographical differences between states but also differences in environmental regulation and scientific practice between countries. The principle of subsidiarity allows such differences to be accommodated; however, there is a risk that these differences will also extend to the level of ambition that each member state sets for its water bodies. This was anticipated by the requirement for an intercalibration exercise (Annex V, Clause 1.4.1) to harmonize the position of the high–good and good–moderate status boundaries between states (Birk et al., Citation2013).
The formal outcome of intercalibration was two ‘Decisions’ listing the approved boundaries for all BQEs in all water body types for all member states in the EU (European Union, Citation2008, Citation2013). From these I have derived Tables 2 and 3, which summarize the state of freshwater phytobenthos assessment across the EU. All but five states now have phytobenthos methods for rivers that have been validated via the intercalibration exercise, and nine states also have methods for lakes.
The normative definitions for “macrophytes and phytobenthos” refer to taxonomic composition, abundance, “undesirable disturbances to the balance of organisms” and bacterial tufts and coats (). The phrase “undesirable disturbances” forms part of the formal definition of eutrophication in European Community directive 91/271/EEC (European Community, Citation1991) and implies a need to demonstrate consequences of nutrient enrichment beyond simply changes in photosynthetic biota (see Tett et al., Citation2006; European Commission, Citation2009). Most states have chosen to treat macrophytes and phytobenthos as two discrete sub-elements, and so it is not possible to assess full compliance with the WFD solely by looking at phytobenthos methods, most of which measure only taxonomic composition and relative abundance of diatoms. Absolute abundance of phytobenthos is, in any case, a spatially and temporally variable property (Biggs & Thomsen, Citation1995; Marker & Collett, Citation1997; Pan et al., Citation1999). Moreover, the division between macrophytes and phytobenthos is not always clear, with some states recording larger algae such as Lemanea, Cladophora and Vaucheria in macrophyte surveys. States that adopt this approach are able to report an approximation (e.g. via percent cover scales) of phytobenthos abundance.
Of those states that have now reported phytobenthos methods, 15 (58%) have chosen to use weighted average metrics developed prior to the WFD, with the IPS (9 states, 35%) being the most commonly employed (). The apparent popularity of the IPS, however, disguises many national variants and it is better to regard the IPS as a ‘family’ of closely related indices, rather than as a wholly consistent approach to ecological status assessment amongst these states.
Table 2. National approaches to fulfilling the WFD’s requirement to assess phytobenthos in rivers. Information from European Union (Citation2008, Citation2013) and www.wiser.eu. ‘No method’ means that the State has neither submitted a national method to intercalibration nor provided information to the WISER database.
Use of weighted average metrics in isolation assumes that nutrient or organic pollution is the main pressure encountered within the territory. Many combinations of taxa can yield the same index value for such metrics so weighted average metrics are not in strict compliance with the requirement to show no taxonomic change and there is also a risk of missing pressures that these indices do not detect (e.g. acidification: Schneider et al., Citation2013a).
These problems should be overcome by indices based on the actual species expected to be encountered in a particular water body type. Such metrics have been developed by six states (23%). The approach developed by Germany is a good example: this relates the cumulative frequency of species indicative of reference conditions to ecological status: >76% is characteristic of high status whilst 51–75% indicates good status and 26–50% characterizes moderate status. The list of reference species (Schaumburg et al., Citation2006) includes a list of ‘general reference species’ (mostly taxa associated with low nutrients), subdivided into groups characteristic of ‘siliceous’ and ‘calcareous’ conditions. This list is supplemented by further lists of species regarded as characteristic of particular types of river or stream.
Such metrics assume a clearly demarcated typology and a good understanding of the diatom assemblages encountered within each water body type. In theory, this approach avoids the problem that weighted average metrics are blind to situations where taxonomic change has occurred due to factors other than those for which the metric has been calibrated. However, this advantage has to be balanced against the uncertainty involved in capturing the whole range of variation (microhabitat, temporal etc.) encountered in stream types, particularly when reference sites for that type are rare.
In theory, a combination of the two approaches should overcome the limitations of both; this has been adopted by three states, each of which combines two weighted average metrics, the Trophic Index (TI: Rott et al., Citation1999) and the Saprobic Index (SI: Rott et al., Citation1997), with an evaluation of the percentage of ‘reference species’. The TI is most sensitive at low to moderate levels of enrichment (assumed to represent inorganic nutrients) and the SI gives extra sensitivity in organically enriched sites, while the proportion of reference species should ‘catch’ pressures missed by the other two.
Methods in 11 states (42%) are based solely on diatoms, with no parallel assessment of non-diatom phytobenthos, even in macrophyte surveys. This is potentially worrying, as it indicates that monitoring will fail to detect situations where pressures have resulted in shifts in the balance between different groups of phytobenthos. At the extreme, this means that programmes to detect eutrophication and organic enrichment in rivers, for example, will ignore Cladophora glomerata and other algae which can reach nuisance levels as a result of nutrient enrichment (Dodds & Gudder, Citation1992). The utility of diatoms as ‘indicators’ of particular trophic states needs to be set against the need to have a broader understanding of the condition of the phytobenthos as a basis for management. This, in turn, needs to recognize that shifts in the relative dominance of taxonomic groups can itself be a property of enrichment (Cattaneo, Citation1987; Carrick & Lowe Citation1988) as well as a response to other pressures (e.g. heavy metals; Whitton, Citation1980; ). Norway is the only state that has a phytobenthos assessment method not based on diatoms (Schneider & Lindstrøm, Citation2011), whilst Galicia (northern Spain) is the only region that includes a direct measurement of phytobenthos abundance (Delgardo et al., Citation2010). How other states assess the risks posed by eutrophication without any attempt to assess the quantity of algae present is not clear: the pessimistic assumption is simply that most do not do this at all.
Fig. 1. Schematic diagram illustrating how position on the Ecological Quality Ratio (EQR) scale can affect the confidence of an ecological status classification. It assumes ecological status boundaries are regularly arranged along the EQR gradient (bad status: 0–0.2; poor: 0.2–0.4; moderate: 0.4–0.6; good: 0.6–0.8; high: > 0.8). The upper plot (a) shows a (statistical) sample comprising 30 spatial and/or temporal replicates from a water body, with a mean EQR of 0.58 and standard deviation of 0.027. The mean value suggests moderate status; however, as this is close to the good/moderate boundary, 11 samples have EQRs ≥ 0.6, suggesting good status. There is, in other words, a 63% probability that the ‘true’ status is moderate and a 37% probability that it is, in fact, good status. By contrast, although the standard deviation is larger (0.046) in the lower plot (b), the confidence of class is greater as the mean corresponds to the middle of the class (0.3) and the distribution does not spill into adjacent classes.
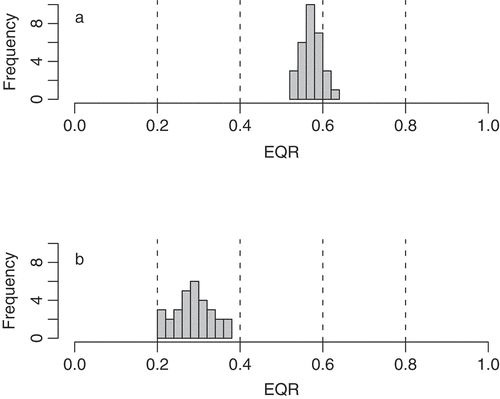
Fig. 2. The River Nent at Nentsberry, Cumbria, northern England (UK National Grid reference NY 766449). The green colour on the river bed is due to prolific growth of metal-tolerant populations of the chlorophyte Stigeoclonium tenue, which accounts for approximately 80% of the biovolume of algae in the river (Kelly, Citation2012).
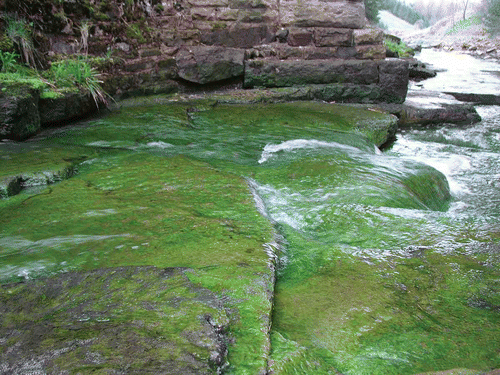
The capacity to detect pressures other than the nutrient- or organic gradients appears to be limited, apart from in those states that use ‘reference species’ approaches. In some cases, lack of capacity may reflect a low risk of such pressures occurring (why go to the expense of developing an acidification metric, for example, for a territory that lies mostly on calcareous geology?) or it may be that such pressures will be detected by other BQEs. Of those who do have additional diagnostic capacity, the German assessment system has procedures that detect acidification and salinity (Schaumburg et al., Citation2006), Norway and UK have special acidification metrics (Schneider & Lindstrøm, Citation2009; Juggins & Kelly, Citation2012; Schneider et al., Citation2013b), and Sweden has a metric that detects changes in pH (Andrén & Jarlman, Citation2009), although not, as yet, acidification.
The situation for lakes is broadly similar, except that only nine states have developed methods to the stage where they can be intercalibrated whilst three others include assessment of filamentous algae in their macrophyte assessments (). This leaves 15 states with no formal assessment of phytobenthos in lakes at all. Several states have argued that their macrophyte assessment systems are adequate to fulfil their obligations, although few have presented any data to support this assertion (Poikane, Citation2013). For such an assumption to be valid, a strong correlation between macrophytes and phytobenthos EQRs would need to be demonstrated and few states have produced such evidence (presumably believing that macrophytes alone are sufficient).
Table 3. National approaches to fulfilling the WFD’s requirement to assess phytobenthos in lakes. Information from European Union (Citation2008, in prep.) and www.wiser.eu. Only those states with formal assessment of phytobenthos or macroalgae other than charophytes are included in this table.
Dealing with variability
A phytobenthos sample represents a particular point in space and time whereas ‘ecological status’ applies to a broader area (a stretch of a river, or all or part of a lake) over a period of time (updates on status are required by the European Commission every three years). A sample, therefore, can only estimate the probability of a particular status class for a particular period and method (Clarke, Citation2012). Replicate sampling can refine the prediction but not eliminate uncertainty altogether. Yet sampling and analysis is expensive and regulators work within tight budgets, raising questions about how resources can best be used. Can uncertainty be reduced significantly by spending more time processing each sample, or should the same effort be divided between several replicate samples? And, if the latter, how should effort be split between spatial and temporal replicates from the same water body?
Pappas & Stoermer (Citation1996) showed that the number of taxa recorded increases as effort spent per sample increases. But does this extra information on biodiversity actually contribute to our knowledge about ecological status at a site? The nature of the metrics used in ecological assessments means that the amount of extra ecological information obtained from increased effort may be low (Lavoie et al., 2008a; Kelly & Ector, Citation2012; Rimet & Bouchez, Citation2012). If this is the case, then is there a case for more spatial and temporal replication at the expense of effort in the laboratory? Whilst there are many studies of variability in phytobenthos assemblages (e.g. Passy, Citation2001; Prygiel et al., Citation2002; Besse-Lotoskaya et al., Citation2006; Lavoie et al., Citation2008b), relatively few of these express results in terms that can be transposed to water quality management. Kelly et al. (Citation2009a) showed that repeated sampling at the same site reduces the risk of misclassification of river and lake sites appreciably. They also noted, too, that confidence of assignment to a class is necessarily reduced when mean EQR values are close to status class boundaries (). They recommended temporal replication within a water body (two samples per year over a three-year reporting period), rather than spatial replication, though others (e.g. Schaumburg et al., Citation2004) urge spatial as well as temporal sampling. More sophisticated approaches to evaluating optimal sampling strategies are now available (e.g. WISERBUGS: Clarke, Citation2012) but have yet to be applied to phytobenthos.
The final estimate of uncertainty that should be considered is that of how diatom-based assessments relate to the entire phytobenthos community, bearing in mind that only three states make a thorough assessment of diatoms and non-diatoms (the opposite issue, of course, applies to Norway, which only assesses non-diatoms). Available evidence (Kelly, Citation2006; Kelly et al., Citation2008b; Schneider et al., Citation2013a) suggests that diatoms (or, in Norway, non-diatoms) are good proxies for the entire phytobenthos assemblage. This means that, with some reservations (Schneider et al., Citation2012), diatoms and non-diatoms can be substituted for one another without significant loss of information. Caution, however, is needed in situations where one consequence of a pressure is a shift in dominance between algal groups. Analysis of diatoms alone cannot tell you that one consequence of heavy metal pollution in the River Nent (Cumbria, UK) is massive growths of the green alga Stigeoclonium, due to the loss of grazers (Armitage, Citation1979; Kelly, Citation2012).
Critique
What do we mean by ‘ecological status’?
Ecological status is a legal expression of how entire aquatic ecosystems are structured and function; the principle, given in Article 2, is elaborated in detail in Annex V (), and it is easy to lose sight of the core principles amidst the verbiage. ‘Structure’, for example, is too easily reduced to lists of species names whilst ‘function’ is mostly ignored both in Annex V and in national methods. In theory, lists of species drawn from several trophic levels should imply a set of interactions from which ecosystem functioning can be deduced. In practice, separate methods have been developed for each BQE, each giving a generalized view of ecological status rather than detailed information of the fitness of any particular BQE to service other parts of the ecosystem. The expectation is that all BQEs will change as the level of pressure changes, and the assumption is always that a high correlation between metric and pressure gradient is desirable. Yet, paradoxically, such attitudes could result in high levels of redundancy between metrics, with none offering unique information over and above chemical analyses.
An alternative view is that both phytobenthos metrics and chemical pressure gradients are correlated with an underlying ‘true’ status gradient that encapsulates both structure and function. Kelly et al. (Citation2012) noted Achnanthidium minutissimum sensu lato to be the most commonly reported species at reference sites around Europe. Species in this aggregate generally indicate low levels of nutrient- or organic pressure although, as it is known to develop heavy metal tolerance (Watanabe et al., Citation1988), it is not an unambiguous indicator of pristine conditions. How might a better understanding of the ecology of A. minutissimum sensu lato lead us to a more holistic understanding of ecological status? Biggs et al. (Citation1998) regard A. minutissimum sensu lato as an indicator of disturbed conditions, caused either by hydrological factors or grazing. This species complex is very common in moderately to well-buffered UK streams and rivers free from significant pressures during the summer, when flow levels are mostly low (discounting physical disturbance). It is also often associated with invertebrate grazers such as Chironomidae and Trichoptera. The designation of good ecological status therefore implies the presence of an assemblage that contains many of the characteristics of assemblages found at reference conditions (i.e. healthy ‘structure’), along with healthy interactions with other trophic levels (healthy ‘function’). Moderate status, by contrast, implies a breakdown in grazer–periphyton relationships (see Sturt et al., Citation2011, for an example of how grazers can control nuisance growths of filamentous algae). A few species in the A. minutissimum complex (A. saprophilum, A. eutrophilum) appear to thrive at higher pressure levels. However, without a fuller understanding of the autecology of all species within this complex and, in particular, how they interact with grazers, it is impossible to know whether the presence of A. saprophilum or A. eutrophilum represents a breakdown in function within a stream. That there is far more variation within the A. minutissimum complex than was hitherto suspected seems clear (see Ector, Citation2011, and references therein) but how much of this genuinely helps us to understand ecological status (rather than just reflecting biogeographical and typological variation) remains to be seen.
Phytobenthos assessment and eutrophication management
The counter-argument to having phytobenthos metrics optimized against generalized pressure gradients that are also captured by other groups of organisms is to focus on those pressures to which phytobenthos should be expected to be particularly responsive. Eutrophication is frequently highlighted as one pressure where phytobenthos should contribute (e.g. Besse-Lotoskoya et al., 2011) although current methods are far from adequate.
Understanding their limitations requires recognition of the differences between hazards and risks. ISO (Citation1999) defines ‘hazard’ as a “potential source of harm” and ‘risk’ as the “combination of the probability of occurrence of harm and the severity of that harm”. In the context of eutrophication, the enrichment of waters constitutes the hazard whilst the risk combines this hazard along with the factors that allow the accelerated growth of algae and other plants, leading to the manifestation of undesirable disturbances. These factors may include retention time (in the case of slow-flowing rivers), frequency of scouring spates, light, substratum stability, temperature (Dodds, Citation2006; Hilton et al., Citation2006; Page et al., Citation2012). Seen from this perspective, most of the phytobenthos assessment systems listed in Tables 2 and 3 are no more than biological correlates of the scale of (chemical) hazard, a property defined by Schneider & Melzer (Citation2003) as ‘nutrient potential’; they are not themselves expressions of the risk of eutrophication. Yet, as we have seen, abundance is a property often ignored as part of status assessment using phytobenthos.
On the other hand, high biomasses of algae and plants can develop, apparently naturally, in rivers and lakes far from obvious human influences. Examples include the diatom Didymosphenia geminata (Skulberg & Lillehammer, Citation1984), a rush, Juncus bulbosus (Schneider et al., Citation2013b), the chrysophyte Hydrurus foetidus, and filamentous green algae (my unpublished observations; John et al., Citation2011). Such phenomena have been observed for a long time (Hassall, Citation1852; West & Fritsch, Citation1927) and there is no reason to assume that they are not natural properties of these water bodies. Nor can we presume that these high biomasses do not sometimes have negative effects on other trophic levels (James et al., Citation2010). Rather than argue that abundance is not necessary for ecological status assessment, it is probably more honest to say that the problems of distinguishing between ‘natural’ and anthropogenic high biomass, coupled with the spatial and temporal variability associated with biomass estimates (see above), have led those responsible for developing phytobenthos assessment methods to sidestep the issue and to focus instead on taxonomic composition.
At high or good status it is reasonable to assume that a low level of ‘hazard’ translates into a low risk of developing undesirable disturbances. The inability to differentiate between ‘hazard’ and ‘risk’ becomes a more significant issue for water bodies that are classified at moderate, poor or bad status. Consider two situations – a shaded site whose flora indicates ‘bad status’ but with an unstable substratum and frequent scouring spates versus one whose flora indicates ‘moderate status’ but at an unshaded site with a stable substratum and few spates – where should resources be focused? In the former case, significant alteration from the expected state has occurred but other factors at the site (shade, substratum) may preclude this ‘hazard’ from being converted into a high ‘risk’. Though the ‘hazard’ appears to be lower in the latter scenario, local circumstances increase the risk of a high biomass creating ‘undesirable disturbances’ such as night-time anoxia leading to the death of fish. One possible answer may be to qualify the face-value assessment based on composition with a risk assessment. This may be biological (evidence of high biomass of nuisance algae) or physical (substratum composition, degree of shading) and could result in the classification based on composition being modified by additional evidence to give a better estimate of the risks of undesirable effects of eutrophication at a site.
Integrating phytobenthos into the decision-making process
At the most basic level, most states now fulfil their obligations for surveillance of phytobenthos status in rivers across their territories (albeit with the qualifications described above) and will be producing evidence that, combined with data from other BQEs, will enable them to identify water bodies where programmes of measures are needed. It is at this point that the weaknesses of these methods may start to become apparent. This is partly because most phytobenthos assessment methods are based on associations between groups of organisms and abiotic variables with little supporting experimental evidence, and partly because these pressure gradients are in many cases composed of co-correlated variables. In very polluted waters, restoration steps may be obvious (build or improve a sewage works, for example) but, as the water body approaches moderate status, catchment managers will need clear guidance on which of a number of expensive options (install P stripping, control diffuse inputs of N, etc.) will bring the greatest likelihood of ecological benefits.
In practice, phytobenthos will be one of a number of strands of evidence available to managers. The phytobenthos expert will be part of a team of specialists working to prescribe a catchment-specific remediation. One task will be to optimize experimental designs (location of sampling sites, number and spacing of temporal replicates in order to maximize statistical power). Another may be to recommend supplementary measurements in order to confirm effects (in situ bioassays to determine limiting nutrients, for example: Bowes et al., Citation2007). However, decision-making for the WFD has to consider the socio-economic context, with options for setting “less stringent objectives” (i.e. targets of less than GES) if achievement of GES would be “infeasible or disproportionately expensive” (Article 4, Clause 5). This again highlights the need for phytobenthos assessment to consider the risks and consequences of particular assemblages occurring. Yet this also has implications under Article 14: how well can phytobenthos experts express the concerns that they may have about a water body in terms that a lay person may understand, bearing in mind that it is the water users who have to fund the improvements (Article 9)? States that fail to meet their obligations could be prosecuted in the European Court of Justice, where case law has established the need to demonstrate “undesirable disturbances” as well as “enrichment” and “accelerated growth” as evidence of eutrophication, without any mention of composition (European Court of Justice, Citation2009). Once again, a discipline that evolved using diatoms to indicate chemical conditions may now need to refocus if it is to demonstrate the benefits of a correctly functioning phytobenthos assemblage in sustainable water management.
Summary: what needs to be done
There is no cause for complacency amongst Europe’s phytobenthos specialists after just over a decade of WFD implementation. Whilst progress has been made in some areas (e.g. defining reference conditions), most national methods are at best fit-for-purpose for the basic task of classifying Europe’s fresh waters and probably inadequate for giving guidance to local catchment managers who need to prioritize failing water bodies for action and design appropriate programmes of measures.
The first is that phytobenthos assessment needs deeper roots in functional ecology and, in particular, better conceptual models to relate cause and effect. Current approaches are based mostly on associations derived from analyses of spatial datasets, with relatively little support from experimental studies. Progress is being made (Dodds, Citation2006; Hilton et al., Citation2006; Kelly et al., Citation2009c) but this contrasts with the situation for benthic invertebrates in rivers, for example, where there is a long tradition of relating traits such as respiration and feeding strategies to pollution tolerance and where, as a result, correlations tend to confirm the validity of indices which are rooted in functional ecology. For diatoms, in particular, there is a leap directly from a list of names to an index, via statistical modelling, with relatively little conceptual underpinning (e.g. Besse-Lotoskaya et al., Citation2011; Kelly, Citation2011). There are, for example, simply too few detailed studies on traits of phytobenthos to be able to make authoritative statements on why some species thrive in organic pollution whilst others do not.
The second area of concern is that phytobenthos assessment needs better integration into the decision-making process at the catchment scale. This needs to be understood in the context of the financial circumstances that have engulfed much of Europe: how should a catchment manager divide his or her limited budget amongst various potential strands of evidence? If phytobenthos is to be used, how many temporal and spatial replicates are needed to provide a robust case? How are phytobenthos results communicated to stakeholders? This area seems to be receiving relatively little attention in the peer-reviewed literature, although it is possible that such guidance exists in internal documents in government agencies in some countries, as it does already in New Zealand (Biggs & Kilroy, Citation2000).
Although this review has focused on the limitations of approaches based purely on composition, future approaches will need to consider composition and new technologies such as DNA barcoding, which holds great promise (Mann et al., Citation2010; Zimmermann et al., Citation2011; Kermarrec et al., 2013) as a means of collecting basic information on composition with greater precision and lower cost than was hitherto the case. We should, however, be wary of those (Baird & Hajibabaei, Citation2012) who would bypass conventional taxonomy altogether.
The next decade sees important deadlines approaching for achieving GES and the challenge now is to refine phytobenthos assessment tools so that they can play their role in this. The biggest challenge may be to encourage some of us to step back from our preoccupations with long lists of names derived from examination of empty frustules and to broaden our horizons to consider the role of phytobenthos (from all divisions) in ecosystem functioning.
Martyn Kelly
Bowburn Consultancy, 11 Monteigne Drive, Bowburn, Durham DH6 5QB, UK
E-mail: [email protected]
Acknowledgements
Thanks to the organizers of the 22nd International Diatom Symposium, Ghent, Belgium for the invitation to present a plenary lecture, which provided the impetus to write this review. Discussions with many colleagues have helped shape my views: especial thanks to Geoff Phillips (Environment Agency, UK) and Nigel Willby (University of Stirling, UK). Susanne Schneider (NIVA, Norway), Lydia King (Germany), Aleksandra Zgrundo (University of Gdansk, Poland), along with the editor and three anonymous reviewers, gave helpful feedback on drafts of this manuscript.
References
- Andrén, C. & Jarlman, A. (2009). Benthic diatoms as indicators of acidity in streams. Fundamental and Applied Limnology, 173: 237–253.
- Armitage, P.D. (1979). The effects of mine drainage and organic enrichment on benthos in the River Nent system, Northern Pennines. Hydrobiologia, 74: 119–128.
- Baattrup-Pedersen, A., Springe, G., Riis, T., Larsen, S.E., Sand-Jensen, K. & Kjellerup-Larsen, L.M. (2008). The search for reference conditions for stream vegetation in northern Europe. Freshwater Biology, 53: 1890–1901.
- Baird, D.J. & Hajibabaei, M. (2012). Biomonitoring 2.0: a new paradigm in ecosystem assessment made possible by next-generation DNA sequencing. Molecular Ecology, 21: 2039–2044.
- Battarbee, R.W., Simpson, G.L., Shilland, E.M., Flower, R.J., Kreiser, A., Yang, H. & Clarke, G. (2012). Recovery of UK lakes from acidification: an assessment using combined palaeoecological and contemporary diatom assemblage data. Ecological Indicators, http://dx.doi.org/10.1016/j.ecolind.2012.10.024.
- Behre, K.-E. (1988). The rôle of man in European vegetation history. In Vegetation history (Huntley, B. & Webb, T, editors), 633–672. Kluwer, Dordrecht.
- Bennion, H., Fluin, J. & Simpson, G.L. (2004). Assessing eutrophication and reference conditions for Scottish freshwater lochs using subfossil diatoms. Journal of Applied Ecology, 41: 124–138.
- Bennion, H., Kelly, M.G., Juggins, S., Yallop, M.L., Burgess, A., Reddihough, G., Jamieson, J., & Krokowski, J. (2014). Assessment of ecological status in UK lakes using benthic diatoms. Freshwater Science, (in press).
- Besse-Lotoskaya, A., Verdonschot, P.F.M. & Sinkeldam, J.A. (2006). Uncertainty in diatom assessment: sampling, identification and counting variation. Hydrobiologia, 566: 247–260.
- Besse-Lotoskaya, A., Verdonschot, P., Coste, M. & Van de Vijver, B. (2011). Evaluation of European diatom trophic indices. Ecological Indicators, 11: 456–467.
- Biggs, B.J.F. & Kilroy, C. (2000). Stream periphyton monitoring manual. NIWA, Christchurch, New Zealand.
- Biggs, B.J.F. & Thomsen, H.A. (1995). Disturbance of stream periphyton by perturbations in shear stress:time to structural failure and differences in community resistance. Journal of Phycology, 31: 233–241.
- Biggs, B.J.F., Stevenson, R.J. & Lowe, R.L. (1998). A habitat matrix conceptual model for stream periphyton. Archiv für Hydrobiologie, 143: 21–56.
- Birk, S., Willby, N.J., Kelly, M.G., Bonne, W., Borja, A., Poikane, S. & van de Bund, W. (2013). Intercalibrating classifications of ecological status: Europe’s quest for common management objectives of aquatic ecosystems. Science of the Total Environment, 454-455: 490–499.
- Bowes, M.J., Smith, J.T., Hilton, J., Sturt, M.M. & Armitage, P.D. (2007). Periphyton biomass response to changing phosphorus concentrations in a nutrient-impacted river: a new methodology for phosphorus target setting. Canadian Journal of Fisheries and Aquatic Science, 64: 227–238.
- Cairns, J., Jr (1980). The ABCs of biological monitoring. In Biological monitoring of fish (Hocault, C.H. & Stauffer, J.R. Jr, editors), 43–58. D.C. Heath, Lexington, MA.
- Carrick, H.J. & Lowe, R.L. (1988). Response of Lake Michigan benthic algae to in situ enrichment with Si, N, and P. Canadian Journal of Fisheries and Aquatic Sciences, 45: 271–279.
- Cattaneo, A. (1987). Periphyton in lakes of different trophy. Canadian Journal of Fisheries and Aquatic Sciences, 44: 296–303.
- CEMAGREF (1982). Etude de méthodes biologiques quantitatives d‘appreciation de la qualité des eaux. Rapport Q.E. Lyon-A.F.B. Rhône-Mediterranée-Corse.
- CEN (2003). Water quality – guidance standard for the surveying of aquatic macrophytes in running waters. EN 14184: 2003. Comité European de Normalisation, Geneva.
- CEN (2004). Water quality – Guidance standard for the identification, enumeration and interpretation of benthic diatom samples from running waters. EN 14407:2004. Comité European de Normalisation, Geneva.
- Charles, D.F. & Whitehead, D.R. (1986). The PIRLA project: paleoecological investigation of recent lake acidification. Hydrobiologia, 143: 13–20.
- Clarke, A.L., Weckström, K., Conley, D.J., Anderson, N.J., Adser, F., Andrén, E., de Jonge, V.N., Ellegaard, M., Juggins, S., Kauppila, P., Korhola, A., Reuss, N., Telford, R.J. & Vaalgamaa, S. (2006). Long-term trends in eutrophication and nutrients in the coastal zone. Limnology and Oceanography, 51: 385–397.
- Clarke, R.T. (2012). Estimating confidence of European WFD ecological status class and WISERBUGS (WISER Bioassessment Uncertainty Guidance Software). Hydrobiologia, 704: 39–56.
- Coring, E., Schneider, S., Hamm, A. & Hofmann, G. (1999). Durchgehendes Trophiesystem auf der Grundlage der Trophieindikation mit Kieselalgen. Deutscher Verband für Wasserwirtschaft und Kulturbau e.V., Koblenz.
- Coste, M., Boutry, S., Tison-Rosebery, J. & Delmas, F. (2009). Improvements of the Biological Diatom Index (BDI): description and efficiency of the new version (BDI-2006). Ecological Indicators, 9: 621–650.
- Delgardo, C., Pardo, I. & Liliana, G. (2010). A multimetric diatom index to assess the ecological status of coastal Galician rivers (NW Spain). Hydrobiologia, 644: 371–384.
- Dell’Uomo, A. (1998). Use of algae for monitoring rivers in Italy: current situation and perspectives. In Use of algae for monitoring rivers III (Prygiel, J., Whitton, B.A. & Bukowska, J., editors), 17–25. Agence de l’Eau Artois-Picardie, Douai.
- Denys, L. (2009). Palaeolimnology without a core: 153 years of diatoms and cultural environmental change in a shallow lowland lake (Belgium). Fottea, 9: 317–332.
- Derwent, R.G. & Wilson, R.B. (2012). Acidification research: evaluation and policy applications, a United Kingdom policy response. In Acidification research: evaluation and policy applications (Schneider, T., editor), 253–256. Elsevier, Amsterdam.
- Descy, J-P. (1979). A new approach to water quality estimation using diatoms. Nova Hedwigia, 64: 305–323.
- Descy, J-P. & Coste, M. (1991). A test of methods for assessing water quality based on diatoms. Verhandlungen, Internationale Vereinigung für Theoretische und Angewandte Limnologie, 24: 2112–2116.
- Dodds, W.K. (2006). Eutrophication and trophic state in rivers and streams. Limnology and Oceanography, 51: 671–680.
- Dodds, W.K. & Gudder, D.A. (1992). The ecology of Cladophora. Journal of Phycology, 28: 415–427.
- Ector, L. (2011). 1st European Workshop on Diatom Taxonomy. Algological Studies, 136/137: 1–4.
- European Commission (2009). Guidance document on eutrophication assessment in the context of European water policies. Guidance Document 23, version 12. Directorate-General for the Environment, Brussels.
- European Community (1991). Council directive of 21 May 1991 concerning urban waste water treatment (91/271/EEC). Official Journal of the European Community, Series L, 135: 40–52.
- European Court of Justice (2009). Judgment of the Court (Third Chamber) of 10 December 2009 (Case C-390/07). European Commission v United Kingdom of Great Britain and Northern Ireland. Failure of a Member State to fulfil obligations – Environment – Directive 91/271/EEC – Urban waste water treatment – Article 3(1) and (2), Article 5(1) to (3) and (5) and Annexes I and II – Initial failure to identify sensitive areas – Concept of ‘eutrophication’ – Criteria – Burden of proof – Relevant date when considering the evidence – Implementation of collection obligations – Implementation of more stringent treatment of discharges into sensitive areas. Official Journal of the European Union, C 24/4.
- European Union (2000). Directive 2000/60/EC of the European Parliament and of the Council of 23 October 2000 establishing a framework for Community action in the field of water policy. Official Journal of the European Communities, Series L, 327: 1–73.
- European Union (2008). Commission Decision of 30 October 2008 establishing, pursuant to Directive 2000/60/EC of the European Parliament and of the Council, the values of the Member State monitoring system classifications as a result of the intercalibration exercise. Official Journal of the European Union, Series L, 332: 20–44.
- European Union (2013). Commission Decision of 20 September 2013 establishing, pursuant to Directive 2000/60/EC of the European Parliament and of the Council, the values of the Member State monitoring system classifications as a result of the intercalibration exercise and repealing Decision 2008/915/EC. Official Journal of the European Union, Series L, 266: 1–47.
- Feio, M.J., Aguiar, F.C., Almeida, S.F., Ferreira, J., Ferreira, M.T., Elias, C., Serra, S.R., Buffagni, A., Cambra, J., Chauvin, C., Delmas, F., Dörflinger, G., Erba, S., Flor, N., Ferréol, M., Germ, M., Mancini, L., Manolaki, P., Marcheggiani, S., Minciardi, M.R., Munné, A., Papastergiadou, E., Prat, N., Puccinelli, C., Rosebery, J., Sabater, S., Ciadamidaro, S., Tornés, E., Tziortzis, I., Urbanič, G. & Vieira, C. (2013). Least disturbed condition for European Mediterranean rivers. Science of the Total Environment. doi: 10.1016/j.scitotenv.2013.05.056.
- Flower, R.J. & Battarbee, R.G. (1983). Diatom evidence for recent acidification of two Scottish lochs. Nature, 305: 130–133.
- Hajnal, E., Stenger-Kovács, C., Ács, É. & Padisak, J. (2009). DILSTORE software for ecological status assessment of lakes based on benthic diatoms. Fottea, 9: 351–354.
- Hassall, A.H. (1850). Memoir on the organic analysis or microscopic examination of water supplied to the inhabitants of London and the suburban districts. Lancet, 1: 230–235.
- Hassall, A.H. (1852). A history of the British freshwater algae, including descriptions of the Desmidacea and Diatomacea. 1. (Text). Taylor, Walton and Maberley, London.
- Hilton, J., O‘Hare, M., Bowes, M.J. & Jones, J.I. (2006). How green is my river? A new paradigm of eutrophication in rivers. Science of the Total Environment, 365: 66–83.
- Hofmann, G. (1999). Trophiebewertung von Seen anhand von Aufwuchsdiatomeen. In Biologische Gewässeruntersuchung, vol. 2 (Tümpling. W. & Friedrich, G., editors), 319–333. Gustav Fischer, Jena.
- ISO (1999). Safety aspects – guidelines for their inclusion in standards. ISO/IEC Guide 51. International Standards Organisation, Geneva.
- James, D.A., Ranney, S.H., Chipps, S.R. & Spindler, B.D. (2010). Invertebrate composition and abundance associated with Didymosphenia geminata in a montane stream. Journal of Freshwater Ecology, 25: 235–241.
- John, D.M., Whitton, B.A. & Brook, A.J. (2011). The freshwater algal flora of the British Isles. Second Edition. Cambridge University Press, Cambridge.
- Jones, V.J., Stevenson, A.C. & Battarbee, R.W. (1989). Acidification of lakes in southwest Scotland: a diatom and pollen study of the post-glacial history of the Round Loch of Glenhead. Journal of Ecology, 77: 1–23.
- Juggins, S. & Kelly, M. (2012). A WFD-compatible approach to assess acidification using diatoms in UK and Irish rivers. Report SC070034/TR2, Environment Agency, Bristol.
- Kelly, M.G. (2006). A comparison of diatoms with other phytobenthos as indicators of ecological status in streams in northern England. In Proceedings of the 18th International Diatom Symposium 2004 (Witkowski, A., editor), 139–151. Biopress, Bristol.
- Kelly, M.G. (2011). The Emperor’s new clothes? A comment on Besse-Lototskaya et al. (2011). Ecological Indicators, 11: 1492–1494.
- Kelly, M.G. (2012). The semiotics of slime: visual representation of phytobenthos as an aid to understanding ecological status. Freshwater Reviews, 5: 105–119.
- Kelly, M.G. & Ector, L. (2012). Effect of streamlining taxa lists on diatom-based indices: implications for intercalibrating ecological status. Hydrobiologia, 695: 253–263.
- Kelly, M.G. & Whitton, B.A. (1995). The Trophic Diatom Index: a new index for monitoring eutrophication in rivers. Journal of Applied Phycology, 7: 433–444.
- Kelly, M.G., Cazaubon, A., Coring, E., Dell’Uomo, A., Ector, L., Goldsmith, B., Guasch, H., Hürlimann, J., Jarlman, A., Kawecka, B., Kwandrans, J., Laugaste, R., Lindstrøm, E.-A., Leitao, M., Marvan, P., Padisák, J., Pipp, E., Prygiel, J., Rott, E., Sabater, S., van Dam, H. & Vizinet, J. (1998). Recommendations for the routine sampling of diatoms for water quality assessments in Europe. Journal of Applied Phycology, 10: 215–224.
- Kelly, M., Juggins, S., Guthrie, R., Pritchard, S., Jamieson, J., Rippey, B., Hirst, H. & Yallop, M. (2008a). Assessment of ecological status in U.K. rivers using diatoms. Freshwater Biology, 53: 403–422.
- Kelly, M.G., King, L., Jones, R.I., Barker, P.A. & Jamieson, B.J. (2008b). Validation of diatoms as proxies for phytobenthos when assessing ecological status in lakes. Hydrobiologia, 610: 125–129.
- Kelly, M.G., Bennion, H., Burgess, A., Ellis, J., Juggins, S., Guthrie, R., Jamieson, J., Adriaenssens, V. & Yallop, M. (2009a). Uncertainty in ecological status assessments of lakes and rivers using diatoms. Hydrobiologia, 633: 5–15.
- Kelly, M.G., Bennett, C., Coste, M., Delgado, C., Delmas, F., Denys, L., Ector, L., Fauvlle, C., Ferreol, M., Golub, M., Jarlman, A., Kahlert, M., Lucey, J., ní Chatháin, B., Pardo, I., Pfister, P., Picinska-Faltynowicz, J., Rosebery, J., Schranz, C., Schaumburg, J., van Dam, H. & Vilbaste, S. (2009b). A comparison of national approaches to setting ecological status boundaries in phytobenthos assessment for the European Water Framework Directive: results of an intercalibration exercise. Hydrobiologia, 621: 169–182.
- Kelly, M.G., King, L. & Ní Chatháin, B. (2009c). The conceptual basis of ecological status assessments using diatoms. Biology and Environment: Proceedings of the Royal Irish Academy, 109B: 175–189.
- Kelly, M.G., Gómez-Rodríguez, C., Kahlert, M., Almeida, S.F.P., Bennett, C., Bottin, M., Delmas. F., Descy, J.-P., Dörflinger, G., Kennedy, B., Marvan, P., Opatrilova, L., Pardo, I., Pfister, P., Rosebery, J., Schneider, S. & Vilbaste, S. (2012). Establishing expectations for pan-European diatom based ecological status assessments. Ecological Indicators, 20: 177–186.
- Kermarrec, L., Franc, A., Rimet, F., Chaumeil, P., Humber, J.F. & Bouchez, A. (2013). Next-generation sequencing to inventory taxonomic diversity in eukaryotic communities: a test for freshwater diatoms. Molecular Ecology Resources. doi: 10.1111/1755-0998.12105.
- Kolkwitz, R. & Marsson, M. (1908). Ökologie der pflanzliche Saprobien. Berichte der Deutschen Botanischen Gesellschaft, 26: 505–519.
- Lancaster, J., Real, M., Juggins, S., Monteith, D.T., Flower, R.J. & Beaumont, W.R.C. (1996). Monitoring temporal changes in the biology of acid waters. Freshwater Biology, 36: 179–201.
- Lange-Bertalot, H. (1979). Pollution tolerance as a criterion for water quality estimation. Nova Hedwigia, 64: 285–304.
- Lavoie, I., Dillon, P.J. & Campeau, S. (2008a). The effect of excluding diatom taxa and reducing taxonomic resolution on multivariate analyses and stream bioassessment. Ecological Indicators, 9: 213–225.
- Lavoie, I., Campeau, S., Darchambeau, F., Cabana, G. & Dillon, P. (2008b). Are diatoms good integrators of temporal variability in stream water quality? Freshwater Biology, 53: 827–841.
- Lecointe, C., Coste, M. & Prygiel, J. (1993). Omnidia: software for taxonomy, calculation of diatom indices and inventories management. Hydrobiologia, 269/270: 509–513.
- Mann, D.G., Sato, S., Trobajo, R., Vanormelingen, P. & Souffreau, C. (2010). DNA barcoding for species identification and discovery in diatoms. Cryptogamie, Algologie, 31: 557–577.
- Marker, A.F.H. & Collett, G.D. (1997). Spatial and temporal characteristics of algae in the River Great Ouse. II. The epiphytic algal flora. Regulated Rivers: Research & Management, 13: 235–244.
- Moss, B. (2008). The Water Framework Directive: total environment or political compromise? Science of the Total Environment, 400: 32–41.
- Page, T., Heathwaite, A.L., Moss, B., Reynolds, C., Beven, K.J., Pope, L. & Willows, R. (2012). Managing the impacts of nutrient enrichment on river systems: dealing with complex uncertainties in risk analysis. Freshwater Biology, 57: 108–123.
- Pan, Y., Stevenson, R.J., Hill, B.H., Kaufmann, P.R. & Herlihy, A.T. (1999). Spatial patterns and ecological determinants of benthic algal assemblages in mid-Atlantic streams, USA. Journal of Phycology, 35: 460–468.
- Pappas, J.L. & Stoermer, E.F. (1996). Quantitative method for determining a representative algal count. Journal of Phycology, 32: 693–696.
- Pardo, I., Gómez-Rodríguez, C., Wasson, J-G., Owen, R., van de Bund, W., Kelly, M., Bennett, C., Birk, S., Buffagni, A., Erba, S., Mengin, N., Murray-Bligh, J. & Ofenböeck, G. (2012). The European reference condition concept: a scientific and technical approach to identify minimally-impacted river ecosystems. Science of the Total Environment, 420: 33–42.
- Passy, S.I. (2001). Spatial paradigms of lotic diatom distribution: a landscape ecology perspective. Journal of Phycology, 37: 370–378.
- Picinska-Faltynowicz, J. & Blachuta, J. (2008). Zasady poboru i opracowania prób fitobentosu okrzemkowego z rzek i jezior. Przewodnik metodyczny, Warsaw, Poland.
- Poikane, S. (2013). Intercalibration of biological elements for lake water bodies. Contributions to the Common Implementation Strategy for the Water Framework Directive. JRC scientific and policy reports, European Commission, Luxembourg.
- Prygiel, J. & Coste, M. (1993). The assessment of water quality in the Artois-Picardie water basin (France) by the use of diatom indices. Hydrobiologia, 269/270: 343–349.
- Prygiel, J., Carpentier, P., Almeida, S., Coste, M., Druart, J.-C., Ector, L., Guillard, D., Honoré, M.-A., Iserentant, R., Ledeganck, P., Lalanne-Cassou, C., Lesniak, C., Mercier, I., Moncaut, P., Nazart, M., Nouchet, N., Peres, F., Peeters, V., Rimet, F., Rumeau, A., Sabater, S., Straub, F., Torrisi, M., Tudesque, L., van der Vijver, B., Vidal, H., Vizinet, J. & Zydek, N. (2002). Determination of the biological diatom index (IBD NF T 90-354): results of an intercomparison exercise. Journal of Applied Phycology, 14: 27–39.
- Renberg, I. & Hellberg, T. (1982). The pH history of lakes in south-western Sweden, as calculated from the subfossil diatom flora of the sediments. Ambio, 11: 30–33.
- Rimet, F. (2012). Recent views on river pollution and diatoms. Hydrobiologia, 683: 1–24.
- Rimet, F. & Bouchez, A. (2012). Biomonitoring river diatoms: implications of taxonomic resolution. Ecological Indicators 15: 92–99.
- Rott, E., Hofmann, G., Pall, K., Pfister, P. & Pipp, E. (1997). Indikationslisten für Aufwuchsalgen. Teil 1: Saprobielle Indikation. Bundesministerium für Land- und Forstwirtschaft, Vienna.
- Rott, E., Pipp, E., Pfister, P., van Dam, H., Ortler, K., Binder, N. & Pall, K. (1999). Indikationslisten für Aufwuchsalgen in Österreichischen Fliessgewassern. Teil 2: Trophieindikation. Bundesministerium für Land- und Forstwirtschaft, Vienna.
- Schaumburg, J., Schranz, C., Hofmann, G., Stelzer D., Schneider, S. & Schmedtje, U. (2004). Macrophytes and phytobenthos as indicators of ecological status in German lakes – a contribution to the implementation of the Water Framework Directive. Limnologica, 34: 302–314.
- Schaumburg, J., Schranz, C., Stelzer, D., Hofmann, G., Gutowski, A. & Foerster, J. (2006). Instruction protocol for the ecological assessment of running waters for implementation of the EC Water Framework Directive: macrophytes and phytobenthos. Bavarian Environment Agency, Augsburg.
- Schneider, S. & Lindstrøm, E.-A. (2009). Bioindication in Norwegian rivers using non-diatomaceous benthic algae: the acidification index periphyton (AIP). Ecological Indicators, 9: 1206–1211.
- Schneider, S. & Lindstrøm, E.-A. (2011). The periphyton index of trophic status PIT: a new eutrophication metric based on non-diatomaceous benthic algae in Nordic rivers. Hydrobiologia, 665: 143–155.
- Schneider, S. & Melzer, A. (2003). The trophic index of macrophytes (TIM) – a new tool for indicating the trophic state of running waters. International Review of Hydrobiology, 88: 49–67.
- Schneider, S.C., Lawniczak, A.E., Picińska-Faltynowicz, J. & Szoszkiewicz, K. (2012). Do macrophytes, diatoms and non-diatom benthic algae give redundant information? Results from a case study in Poland. Limnologica, 43: 204–211.
- Schneider, S.C., Kahlert, M. & Kelly, M.G. (2013a). Nutrient supply and pH interact in determining benthic algal assemblages in streams: consequences for biodiversity and ecological assessment. Science of the Total Environment, 444: 73–84.
- Schneider, S.C., Moe, T.F., Hessen, D.O. & Kaste, Ø (2013b). Juncus bulbosus nuisance growth in oligotrophic freshwater ecosystems: different triggers for the same phenomenon in rivers and lakes? Aquatic Botany, 104: 15–24.
- Schönfelder, I. (2005). Analyse von planktischen und benthischen Diatomeen in ausgewähltenFließgewässern und Seen im Raum Berlin-Brandenburg im Rahmen eines Praxistests des Bewertungsverfahrens PHYLIB zur Umsetzung der EU-Wasserrahmenrichtlinie. Abschlussbericht im Auftrag des Berliner Betriebes für Zentrale Gesundheitliche Aufgaben und dem Institut für Lebensmittel, Arzneimittel und Tierseuchen Berlin (ILAT).
- Shubert, L.E. (1984). Algae as ecological indicators. Academic Press, London.
- Skulberg, O.M. & Lillehammer, A. (1984). Glåma. In Ecology of European rivers (Whitton, B.A., editor), 469–498. Blackwell Scientific Publications, Oxford.
- Stenger-Kovács, C., Buczkó, K., Hajnal, E. & Padisák, J. (2007). Epiphytic, littoral diatoms as bioindicators of shallow lake trophic status: Trophic Diatom Index for Lakes (TDIL) developed in Hungary. Hydrobiologia, 589: 141–154.
- Sturt, M.M., Jansen, M.A.K. & Harrison, S.S.C. (2011). Invertebrate grazing and riparian shade as controllers of nuisance algae in a eutrophic river. Freshwater Biology, 56: 2580–2593.
- Tansley, A.G. (1935). The use and abuse of vegetational concepts and terms. Ecology, 16: 284–307.
- Tett, P., Gowen, R., Mills, D., Fernandes, T., Gilpin, L., Huxham, M., Kennington, K., Read, P., Service, M., Wilkinson, M. & Malcolm, S. (2006). Defining and detecting undesirable disturbance in the context of marine eutrophication. Marine Pollution Bulletin, 55: 282–297.
- Tison, J., Park, Y.-S., Coste, M., Wasson, J.G., Ector, L., Rimet, F. & Delmas, F. (2005). Typology of diatom communities and the influence of hydro-ecoregions: a study on the French hydroecosystem scale. Water Research, 39: 3177–3188.
- Várbiró, G., Borics, G., Csányi, B., Fehér, G., Grigorszky, I., Kiss, K.T., Tóth, A. & Ács, É. (2012). Improvement of the ecological water qualification system of rivers based on the first results of the Hungarian phytobenthos surveillance monitoring. Hydrobiologia, 695: 125–135.
- VMM (2009). Biological assessment of the natural, heavily modified and artificial surface water bodies in Flanders according to the European Water Framework Directive. Vlaamse Milieumaatschappij, Erembodegem, Belgium.
- Vogel, A., Beier, T., Braun, J. & Raeder, U. (2005). Does the process of drying submerged macrophytes affect community structure and composition of epiphytic diatoms? Hydrobiologia, 541: 237–240.
- Watanabe, M.M., Takeuchi, Y. & Takamura, N. (1988). Cu tolerance of a freshwater benthic diatom, Achnanthes minutissima. In Biological monitoring of environmental pollution (Yasuno, M. & Whitton, B.A., editors), 171–177. Tokai University Press, Tokyo.
- West, G.S. & Fritsch, F.E. (1927). A treatise on the British freshwater algae. Cambridge University Press, Cambridge.
- Whitton, B.A. (1980). Zinc and plants in rivers and streams. In Zinc in the environment. Part II: Health effects. (Nriagu, J.O., editor), 363–400. Wiley–Interscience, New York.
- Whitton, B.A. & Kelly, M.G. (1995). Use of algae and other plants for monitoring rivers. Australian Journal of Ecology, 20: 45–56.
- Yallop, M., Hirst, H., Kelly, M., Juggins, S., Jamieson, J. & Guthrie, R. (2009). Validation of ecological status concepts in UK rivers using historic diatom samples. Aquatic Botany, 90: 289–295.
- Zelinka, M. & Marvan, P. (1961). Zur Präzisierung der biologischen Klassifikation des Reinheit fliessender Gewässer. Archiv für Hydrobiologie, 57: 389–407.
- Zimmermann, J., Jahn, R. & Gemeinholzer, B. (2011). Barcoding diatoms: evaluation of the V4 subregion on the 18S rRNA gene, including new primers and protocols. Organisms, Diversity and Evolution, 11: 173–193.