Abstract
Based on haemolytic activity and reactive oxygen species (ROS) production of Chattonella marina, Chattonella antiqua, Heterocapsa circularisquama, Alexandrium tamiyavanichii and Karenia mikimotoi, the species were categorized into four types. (1) H. circularisquama: haemolytic activity was detected in both cell suspension and cell-free culture supernatant, but with greater activity in cell suspension than in the supernatant suggesting the presence of both cell surface and secreted haemolytic agents. (2) A. tamiyavanichii: equal haemolytic activities were detected in both the cell suspension and cell-free culture supernatant suggesting the presence of only secreted haemolytic agents. (3) K. mikimotoi: haemolytic activity was detected only in the cell suspension, indicating haemolytic agents occur only on the cell surface. (4) C. marina and C. antiqua: no significant haemolytic activity was detected in either cell suspension or cell-free culture supernatant, but high ROS were detected in the cell suspensions. Heterocapsa circularisquama and K. mikimotoi showed lethal effects on rotifers (Brachionus plicatilis), whereas A. tamiyavanichii, C. marina and C. antiqua had no effect. Our results suggest that H. circularisquama, K. mikimotoi and A. tamiyavanichii produce haemolytic agents with distinct characteristics, whereas C. marina and C. antiqua have an extremely potent ability to produce ROS.
Introduction
The frequency, magnitude and duration of harmful algal blooms (HABs) are increasing as a result of global warming (Paerl & Huisman, Citation2008). HABs often cause mass mortality of wild and farmed fish and shellfish, resulting in serious economic losses and pollution of coastal marine habitats. In addition to such direct effects on marine organisms, some HAB-forming species produce toxic substances that can accumulate in shellfish and other marine organisms, which if consumed are a potential threat to human health. The mechanisms and impacts of toxicity tend to vary substantially among species. More than 100 species of toxic marine microalgae have been identified but the mechanisms causing toxicity in these HAB species are mostly still unclear. The major groups of organisms that form HABs are dinoflagellates (Heterocapsa circularisquama, Karenia mikimotoi and Alexandrium spp.) and raphidophytes (Chattonella spp.).
Chattonella spp. are highly toxic to fish, particularly yellowtail (Seriola quinqueradiata; Okaichi, Citation1989). Previous studies have shown that Chattonella spp. produce extremely high concentrations of reactive oxygen species (ROS) under normal growth conditions (Shimada et al., Citation1991; Oda et al., Citation1997; Kim et al., Citation2007) and several lines of evidence implicate these in fish mortality (Ishimatsu et al., Citation1996; Kim et al., Citation1999; Marshall et al., Citation2003, Citation2005; Hiroishi et al., Citation2005). Chattonella spp. are also known to produce several other toxic substances, such as neurotoxins, haemolytic agents and haemagglutinating agents (Ahmed et al., Citation1995; Khan et al., Citation1996; Kuroda et al., Citation2005). However, it has been reported that dead cells of C. antiqua and filtrate of the culture medium containing the algae were not toxic to red sea bream (Matsusato & Kobayashi, Citation1974). Ishimatsu et al. (Citation1996) also reported that ruptured C. marina cells showed no toxic effects on yellowtail, and there was a clear correlation between the cells’ ability to produce O2– and fish-killing activity (Ishimatsu et al., Citation1996). These findings suggest that live cells are essential for the toxicity of Chattonella spp., and any toxic factors may be quite unstable. Heterocapsa circularisquama can be lethal to shellfish, particularly bivalves such as pearl oysters, but is not known to be toxic to fish (Matsuyama, Citation2012). In a previous study, we showed that H. circularisquama has a haemolytic toxin that may be a cause of shellfish mortality (Oda et al., Citation2001). The bloom-forming K. mikimotoi is known to be toxic to both fish and shellfish (Honjo, Citation1994). Previous studies have demonstrated that K. mikimotoi produces various toxic agents, including haemolytic toxins and ROS (Jenkinson & Arzul, Citation2001; Yamasaki et al., Citation2004; Satake et al., Citation2005; Gentien et al., Citation2007). Some Alexandrium spp. are known to produce toxins that cause paralytic shellfish poisoning (PSP) in humans. In addition, some Alexandrium spp. are reported to have direct detrimental effects on several marine organisms (Sephton et al., Citation2007; Galimany et al., Citation2008; Teegarden et al., Citation2008). Previous work suggests that A. tamiyavanichii, A. tamarense and A. fraterculus produce cytotoxic agents (Katsuo et al., Citation2007; Yamasaki et al., Citation2008), whereas A. taylori secretes a proteinaceous haemolytic toxin (Emura et al., Citation2004).
These findings suggest that HAB toxicity results from a wide variety of toxic agents, which vary among species. Haemolytic agents and ROS seem to be important toxic factors. However, the characteristics and biochemical features of the haemolytic toxins and the importance of ROS as a toxic factor remain poorly understood. Furthermore, there is no comparative study on haemolytic and ROS-producing activities among different HAB species so far, so it is not clear whether or not the activity detected in a certain species is significant compared with other species. To gain insight into this point, comparative study on the various HAB species under the same experimental conditions is necessary, which may provide useful information for understanding the major toxic factors of HAB species. In this study, we measured and compared the haemolytic activity and ROS production of C. marina, C. antiqua, K. mikimotoi, A. tamiyavanichii and H. circularisquama to gain insights into the potentially toxic agents produced by these HAB species.
Materials and methods
Materials
Superoxide dismutase (Cu/Zn-SOD, 3800 U mg–1 of protein, from bovine erythrocytes), horseradish peroxidase (100 U mg–1 of protein), catalase (5000–15 000 U mg–1 of protein), and L-012 were purchased from Wako Pure Chemical Industries, Ltd, Osaka, Japan. P-hydroxyphenylacetic acid (PHPA) was obtained from Sigma-Aldrich Chemical Co. (St. Louis, Missouri, USA). Other chemicals were of the highest grade commercially available.
Cultivation
HAB species were obtained from a variety of sources. Heterocapsa circularisquama Horiguchi and Alexandrium tamiyavanichii Balech were isolated from Fukuyama Bay and Hiroshima Bay, Japan, respectively. Chattonella marina (Subrahmanyan) Hara & Chihara and C. antiqua (Hada) Ono were isolated from Kagoshima Bay and Shimabara Bay, Japan, respectively. These Chattonella species were genetically closely related (Demura et al., Citation2009), but they were morphologically distinguishable and hence, we considered them as different species. Karenia mikimotoi (Miyake & Kominami ex Oda) Gert Hansen & Ø.Moestrup was isolated from Hiroshima Bay, Japan. Cultures of these species were maintained at 26°C in 100 ml flasks containing 60 ml of modified seawater medium (SWM-3) at a salinity of 25 psu (Yamasaki et al., Citation2007). The modified SWM-3 containing a Tris-HCl buffer system was autoclaved for 15 min at 121°C before use. The cultures were kept under a 12:12-h photoperiod regime using a cool-white fluorescent lamp (200 ± 5 µmol photons m–2 s–1). Cell numbers in the cultures were counted using a haemocytometer (Erma Inc., Tokyo, Japan). The rotifer Brachionus plicatilis Müller was provided by Dr A. Hagiwara (Faculty of Fisheries, Nagasaki University, Japan) and was cultured with Nannochloropsis oculata (Droop) D.J.Hibberd. All samples were cultured using sterilized instruments. Each culture was used throughout the experiments at late exponential growth phase unless otherwise specified.
Measurement of haemolytic activity
For each HAB species, a cell-free culture supernatant was obtained from 1 ml of cell suspension of each species in the late exponential growth phase by centrifugation at 5000 × g for 5 min at 4°C. Triplicate 50 µl aliquots of serial two-fold dilutions (using modified SWM-3) of intact cell suspension and cell-free culture supernatant were then added to round-bottom 96-well plates (Becton-Dickinson, Franklin Lakes, NJ, USA). Haemolytic activity was measured using rabbit erythrocytes isolated from rabbit blood (Nippon Bio-Test Laboratories, Tokyo, Japan). Erythrocytes were washed three times with phosphate-buffered saline (PBS) and adjusted to a final concentration of 4% (v/v) in modified SWM-3. To each well containing test samples, 50 µl of the 4% (v/v) erythrocyte suspension was added. The well plates were gently shaken. After incubation for 5 h at 26°C under illumination from a fluorescent lamp (200 µmol photons m–2 s–1), the plates were centrifuged at 900 × g for 5 min. Aliquots (70 µl) of supernatant were withdrawn from the wells and transferred to flat-bottom 96-well plates (Becton-Dickinson). Released haemoglobin was determined by measuring absorbance at 560 nm using a microplate reader (Multiskan GO, Fisher Scientific Inc., Massachusetts, USA). Negative controls (zero haemolysis) and positive controls (100% haemolysis) were prepared using erythrocytes suspended in modified SWM-3 alone and in modified SWM-3 containing 1% Triton X-100 (v/v), respectively.
To examine the effect of heat on haemolytic activity, cell-free supernatant prepared from a H. circularisquama culture (1×105 cells ml–1) and an A. tamiyavanichii culture (2×103 cells ml–1) was subjected to a range of temperatures (0, 27, 37 and 60°C) for 1, 2, 3 and 18 h. Following this, haemolytic activity was measured following the procedure described above. To estimate the molecular size of the haemolytic agents in the supernatants from H. circularisquama and A. tamiyavanichii, supernatants were passed through ultrafiltration units (3000~100,000 cut filters, Merck KGaA, Darmstadt, Germany). The haemolytic activity of each filtrate was measured as described above.
To gain insight into which molecules the haemolytic agents in the supernatants of H. circularisquama and A. tamiyavanichii recognized, haemolytic activity was examined in the presence of various carbohydrates (final 10 mM).
Rotifer exposure experiment
Rotifer exposure tests were conducted in 48-well plates (Becton-Dickinson). One hundred µl of modified SWM-3 containing 10 rotifers was added to each well in a 48-well plate which contained 900 µl of cell suspension from each HAB species. The final concentration of cells was adjusted to 1×104 cells ml–1 for C. marina, C. antiqua and K. mikimotoi, 2×103 cells ml–1 for A. tamiyavanichii and 1×105 cells ml–1 for H. circularisquama. As a negative control, 10 individual rotifers were cultured in 1 ml of the modified SWM-3 alone. The number of dead rotifers was counted every hour over the course of a 7-h incubation using a stereomicroscope.
Measurement of O2–
Production of superoxide anions (O2–) by each HAB species was measured at 26°C using the chemiluminescence method, with L-012 as a superoxide-specific chemiluminescent probe as described by Kadomura et al. (Citation2006). The probe was dissolved in distilled water and stored at −80°C until use. After the addition of L-012 to each flagellate cell suspension in the presence or absence of SOD, the chemiluminescence response was recorded immediately with a Bio-Orbit Luminometer (1254-001, Bio-Orbit Oy, Turku, Finland). The reaction mixtures typically consisted of 145 µl of each flagellate cell suspension, 50 µl of L-012 (final 10 µM) and 5 µl of SOD solution (final 100 U ml–1) or 5 µl of modified SWM-3. As a control, chemiluminescence response in modified SWM-3 containing each probe was measured in the absence of flagellate cells.
Measurement of H2O2
The PHPA assay method at 26°C was used to detect H2O2 in the flagellate cell suspensions (Hyslop & Sklar, Citation1984). After the addition of PHPA (final 1 mM) and horseradish peroxidase (final 100 µg ml–1) to the flagellate cell suspension in the presence or absence of catalase (final 100 U ml–1), any increase in fluorescence intensity during the first min of incubation was measured with a fluorescent spectrophotometer (Model 650-60, Hitachi High-Tech Science Co., Tokyo, Japan) at an excitation wavelength of 317 nm and an emission wavelength of 400 nm. Each measurement was repeated three times. H2O2 concentration was estimated using a standard curve of H2O2 in cell-free modified SWM-3 medium. The standard solution of H2O2 in modified SWM-3 was prepared using reagent H2O2. Under these conditions the increase in fluorescence intensity was proportional to H2O2 concentration.
Statistical analysis
All experiments were carried out in triplicate. Data were expressed as mean ± standard deviation (SD), and paired Student’s t-tests were used to test for significant differences, with p = 0.05 considered to be the threshold for statistical significance.
Results
Haemolytic activity
Haemolytic activity in H. circularisquama cell suspension was positively related to cell concentration, approaching its maximum at 5×104 cells ml–1 (). The cell-free culture supernatant prepared from H. circularisquama culture showed reduced haemolytic activity in comparison. The cell concentration of A. tamiyavanichii at late exponential growth phase was around 2×103 cells ml–1, much lower than that of H. circularisquama. Despite this, significant cell-concentration dependent haemolytic activity was observed (). Haemolytic activity in A. tamiyavanichii cell-free culture supernatant differed little from that in the cell suspension. Karenia mikimotoi showed a positive relationship between cell concentration and haemolytic activity, although even at 2×104 cells ml–1 activity was less than that of H. circularisquama at 3×102 cells ml–1 or A. tamiyavanichii at 1.25×102 cells ml–1 (). No significant haemolytic activity was observed in either cell suspensions or cell-free supernatants of C. marina and C. antiqua at concentrations of up to 4×104 cells ml–1 (, ).
Fig. 1. Haemolytic activities of cell suspension (○) and cell-free culture supernatant (●) of Heterocapsa circularisquama (A), Alexandrium tamiyavanichii (B), Karenia mikimotoi (C), Chattonella marina (D) and C. antiqua (E). Cell suspensions or supernatants equivalent to the indicated cell concentration of each species were mixed with rabbit erythrocytes. After 5 h incubation at 26°C, the extents of haemolysis were measured. Each point represents the mean of triplicate measurements, ± SD (%).
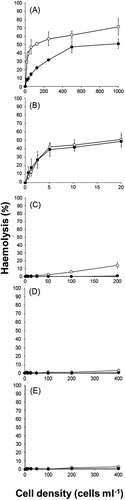
Biochemical characterization of H. circularisquama and A. tamiyavanichii haemolytic agents
The haemolytic constituents of cell-free supernatants from H. circularisquama (1×105 cells ml–1) and A. tamiyavanichii (2×103 cells ml–1) were heat labile; haemolysis was reduced by 70% after 1 h of incubation at 60°C (). The haemolytic properties of H. circularisquama were slightly more heat-stable than those of A. tamiyavanichii supernatant; H. circularisquama haemolysis showed almost no reduction after 18 h of incubation at 0°C, whereas that of A. tamiyavanichii decreased by nearly 50%. In both H. circularisquama and A. tamiyavanichii, haemolytic activities of the supernatants declined by 90% after being passed through the 100 000 cut-filter, suggesting that the haemolytic agents may have molecular size greater than 100 000. There was no haemolytic activity detected in the filtrates obtained from other filters.
Fig. 2. Heat-stability of the haemolytic activities of cell-free culture supernatant of A. tamiyavanichii (A) and H. circularisquama (B). The cell-free supernatant of each species was treated at 0 (○), 27 (△), 37 (□) and 60°C (◇) for the indicated periods of time, and then the haemolytic activities were measured. Each point represents the mean of triplicate measurements, ± SD (%).
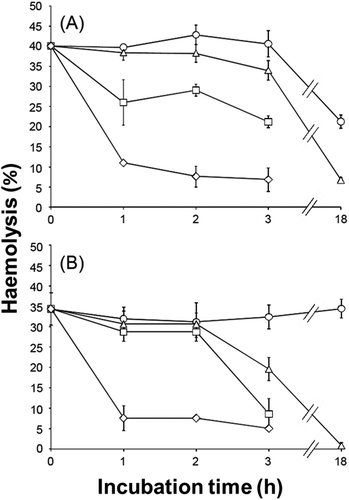
Effects of various saccharides on the haemolytic activities of the cell-free supernatants of H. circularisquama and A. tamiyavanichii
The inhibition profiles obtained in the presence of saccharides differed between H. circularisquama and A. tamiyavanichii (). Haemolysis of supernatant of A. tamiyavanichii was most inhibited by mannitol, whereas that of H. circularisquama was most inhibited by fructose, although this effect was comparatively small.
Fig. 3. Effects of various saccharides on the haemolytic activities of the cell-free culture supernatants of H. circularisquama (□) and A. tamiyavanichii (■). In the absence (control) or presence of the indicated saccharides at 10 mM, the haemolytic activities of the cell-free culture supernatants of each species were measured. The activity was expressed as % of control (100%) without the saccharides. Data are means ± SD (%) of triplicate measurements. Asterisks indicate significant differences between the absence (control) and presence of saccharides (*p<0.05).
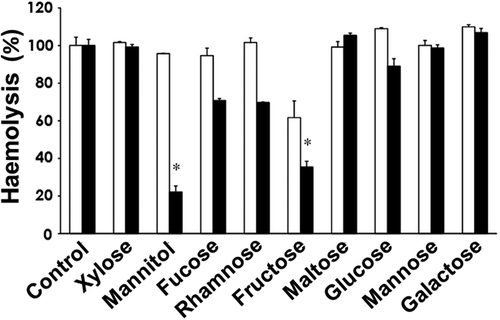
Superoxide (O2–) and hydrogen peroxide (H2O2)
The strongest chemiluminescence response was observed in C. antiqua (1×104 cells ml–1), and a weaker but significant response was observed in C. marina (1×104 cells ml–1) (). In the presence of SOD (100 U ml–1), these responses decreased to the background level observed in the medium alone. A weak response, only slightly above the background level, was observed in K. mikimotoi (). In H. circularisquama and A. tamiyavanichii, the responses were almost background level ().
Fig. 4. L-012-mediated chemiluminescence responses of C. marina (△, ▲), C. antiqua (○, ●) and H. circularisquama (□, ■) (A), K. mikimotoi (□, ■) and A. tamiyavanichii (○, ●) (B). Cell suspensions of C. marina (1×104 cells ml–1), C. antiqua (1×104 cells ml–1), H. circularisquama (105 cells ml–1), A. tamiyavanichii (2×103 cells ml–1), and K. mikimotoi (1×104 cells ml–1) were subjected to L-012-mediated chemiluminescence analysis in the presence (●,▲,■) or absence (○,△,□) of SOD (100 U ml–1).
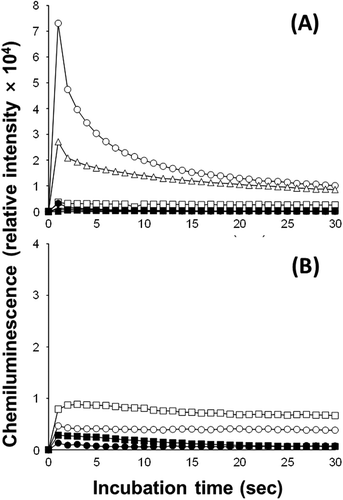
The highest H2O2 concentration was observed in C. marina cell suspension, and lower but significant concentration was detected in that of C. antiqua (). H2O2 concentrations in cell suspensions of H. circularisquama and K. mikimotoi were low compared with those of Chattonella spp. H2O2 was undetectable in A. tamiyavanichii cell suspension ().
Fig. 5. Hydrogen peroxide (H2O2) levels in the cell suspensions of C. marina (1×104 cells ml–1), C. antiqua (1×104 cells ml–1), H. circularisquama (1×105 cells ml–1), K. mikimotoi (1×104 cells ml–1) and A. tamiyavanichii (2×103 cells ml–1). Cell suspension of each species was subjected to PHPA-H2O2 detection assay in the absence (□) or presence (■) of catalase (100 U ml–1). Each column represents the mean of triplicate measurements ± SD.
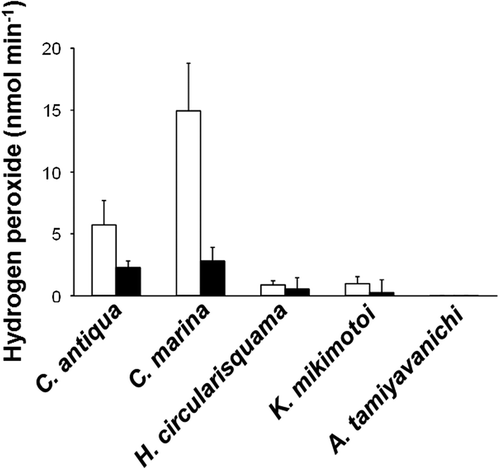
Effects on rotifers
As shown in , H. circularisquama had a potent lethal effect on rotifers; the number of live rotifers gradually decreased with incubation time until all rotifers were dead after 6 h. Karenia mikimotoi also showed toxic effects on rotifers. In contrast, C. marina, C. antiqua and A. tamiyavanichii showed no significant toxic effects on rotifers.
Fig. 6. Effects of C. marina, C. antiqua, H. circularisquama, K. mikimotoi and A. tamiyavanichii on the rotifer Brachionus plicatilis. After exposure to cell suspension of C. marina (1×104 cells ml–1) (○), C. antiqua (1×104 cells ml–1) (△), H. circularisquama (1×105 cells ml–1) (■), A. tamiyavanichii (2×103 cells ml–1) (□) or K. mikimotoi (1×104 cells ml–1) (▲), the number of viable rotifers was counted at the indicated times. Each point represents the mean of triplicate measurements ± SD.
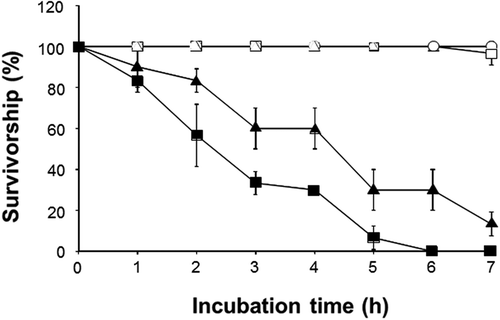
Discussion
Several haemolytic compounds have been isolated from phytoplankton, most of which are fatty acids, such as polyunsaturated fatty acids (PUFAs) (Landsberg, Citation2002). Examples include digalactosyl monoacylglycerol and octadecapentaenoic acid, which were isolated from K. mikimotoi and shown to be haemolytic and ichthyotoxic (Yasumoto et al., Citation1990). Eicosapentaenoic acid (EPA) is the most potent of PUFAs (Takagi et al., Citation1984; Jüttner, Citation2001; Marshall et al., Citation2003). It has been reported that EPA causes harmful effects on zooplankton (Jüttner, Citation2001), phytoplankton (Arzul et al., Citation1998), and fish (Marshall et al., Citation2003). These findings suggest that haemolytic fatty acids may play important roles in the harmful effects of HABs on aquatic organisms. However, these fatty acid-related haemolytic agents are generally isolated and identified by organic solvent-extraction from the cells. Hence, the mechanism of how hydrophobic water-insoluble fatty acids can release from HAB species and attach to the surrounding organisms in the seawater environment is still unclear. Previous studies on the haemolytic properties of dinoflagellate cell suspensions suggested the presence of water-soluble high molecular weight haemolytic compounds that may be involved in the toxicity (Emura et al., Citation2004; Yamasaki et al., Citation2008), although there is only a limited available information on the high molecular weight haemolytic agents. It seems likely that assays that directly detect haemolytic activity in phytoplankton cell suspensions or cell-free supernatants, without the need for extraction or isolation procedures, may provide important tools for finding the toxic factors truly responsible for the harmful effects of phytoplankton.
In this study, we found that the strength of the haemolytic effects of cell suspensions of H. circularisquama, A. tamiyavanichii and K. mikimotoi on rabbit erythrocytes depended on cell density and varied among species. In contrast, C. marina and C. antiqua showed no significant haemolytic activity (). Although cell size differs among species, A. tamiyavanichii showed significant haemolytic activity even at quite low cell densities (less than 1×103 cells ml–1) compared with the other species (around 1×104–1×105 cells ml–1). Comparison of the haemolytic activity of the cell suspensions and the cell-free supernatants suggests that the haemolytic properties of the HAB species studied can be divided into four categories, defined in terms of location and activity (). There seem to be two types of haemolytic agents, those occurring on the cell surface and those secreted extracellularly. Category 1 contains both secreted and cell surface-associated haemolytic agents and is represented by H. circularisquama. Category 2 contains only secreted agents, and is represented by A. tamiyavanichii. Category 3 is typified by K. mikimotoi, and contains only cell surface-associated haemolytic agents. The 4th category is represented by C. marina and C. antiqua, which have no haemolytic activity. Although the exact biological significance of these categories is still unclear, H. circularisquama and K. mikimotoi, which have cell surface-associated haemolytic agents, are known to be toxic to shellfish and zooplankton (Zou et al., Citation2010; Matsuyama, Citation2012). Previous studies have suggested that direct contact between H. circularisquama cells and the soft tissues of bivalve molluscs (Matsuyama et al., Citation1997) or microzooplankton (Kamiyama, Citation1997; Kamiyama & Arima, Citation1997) is important for a lethal effect to occur. Our previous study also showed that K. mikimotoi exerted a lethal effect on zooplankton rotifers through direct contact (Zou et al., Citation2010). Consistent with these findings, H. circularisquama and K. mikimotoi had potent lethal effects on rotifers in exposure experiments, whereas A. tamiyavanichii, C. marina and C. antiqua had no effect (). Taken together, these results suggest that cell-surface associated haemolytic agents observed in H. circularisquama and K. mikimotoi may play an important role in their toxicity to bivalves and zooplankton. Furthermore, A. tamiyavanichii had no toxic effect on rotifers in spite of having far higher haemolytic activity than K. mikimotoi. This suggests that the type of haemolytic agent (i.e. extracellular vs. surface associated) may have a greater influence on toxicity of dinoflagellates than haemolytic potency, at least in the species analysed in this study. In addition, the mechanisms by which bivalves and zooplankton recognize HAB species may be related to cell contact with phytoplankton (Matsuyama et al., Citation1997; Kim et al., Citation2000; Zou et al., Citation2010). Probably, the toxic mechanisms of dinoflagellates are complicated and quite different depending on species, and even on the victimized organisms. Further studies are required to clarify these points.
Fig. 7. Schematic illustrations of the presence of the haemolytic agents and ROS production in H. circularisquama (A), A. tamiyavanichii (B), K. mikimotoi (C) and Chattonella spp. (D). In H. circularisquama, some of the haemolytic agents are secreted extracellularly and some are bound on the cell surface. In A. tamiyavanichii, almost all the haemolytic agents are secreted extracellularly. In K. mikimotoi, almost all the haemolytic agents are bound on the cell surface, and low levels of ROS are produced. In Chattonella spp., there is no haemolytic agent, but high levels of ROS are produced.
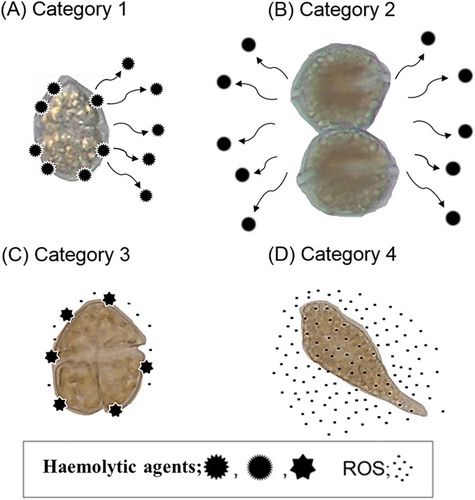
The inhibitory effects of various saccharides on the haemolytic activity of cell-free supernatants differed between H. circularisquama and A. tamiyavanichii (), suggesting molecular recognition mechanisms differ between these two HAB species. It seems likely that the haemolytic agents of these species may recognize different saccharide chains in the rabbit erythrocytes. Mannitol had a large inhibitory effect on the haemolytic activity of A. tamiyavanichii, but not on that of H. circularisquama (). Heat-stability and ultrafiltration analyses indicate that the haemolytic agents secreted from H. circularisquama and A. tamiyavanichii are heat-labile, high molecular weight compounds ().
In the case of unstable exotoxins like haemolytic agents of these dinoflagellates, it is essential to keep the activity stable for purification and characterization. Further studies are required to identify the molecules in erythrocytes important for recognition in these dinoflagellates as well as the biochemical characterization of the haemolytic agents. In that context, it is advantageous that the activity of the haemolytic agent secreted from H. circularisquama was stable at 0°C at least for 18 h. We are now planning to purify the haemolytic agent from H. circularisquama by a combination of several chromatographic procedures at 0°C.
Previous studies demonstrated that Chattonella spp. produce significant levels of ROS and suggested that these might be responsible for fish mortality, although this is still debated (Ishimatsu et al., Citation1996). Chemiluminescence and fluorescence analyses confirmed that C. marina and C. antiqua produce ROS in far greater quantities than the other species tested. Other species of raphidophycean flagellates, such as Heterosigma akashiwo, Fibrocapsa japonica and Olisthodiscus luteus, are known to produce ROS (Oda et al., Citation1997). Hence, ROS production may be a common characteristic of raphidophycean flagellates. Chattonella antiqua produced more superoxide, but less hydrogen peroxide than C. marina (, ). The reason for this is still unclear, but our previous study indicated that the locations and underlying mechanisms of superoxide and hydrogen peroxide generation were different (Kim et al., Citation2007). Fluorescent microscopic analysis using ROS-specific probes suggested that superoxide is produced on the surface of Chattonella cells, while hydrogen peroxide is produced in intracellular compartments (Kim et al., Citation2007). In addition, it has been reported that Hymenomonas carterae, a marine phytoplankter, produces extracellular hydrogen peroxide without involvement of superoxide generation (Palenik et al., Citation1987). Hence it seems likely that the metabolic or enzymatic systems responsible for hydrogen peroxide and superoxide generation are independent, and they may differ somehow between C. marina and C. antiqua. Since these raphidophytes showed no toxic effects on rotifers, it is unlikely that ROS are toxic to rotifers.
Recently we have found that Chattonella marina and the Chattonella antiqua used in this study showed different fish-killing activities and ROS-producing activities, and both activities were well correlated (Cho et al., Citation2016), suggesting that ROS may be a factor responsible for the fish-killing activity of Chattonella spp.
Our results, together with previous findings, suggest that the cell surface structures of HAB species, which may be deeply involved in the haemolytic activity in some dinoflagellates or superoxide production in raphidophytes, play an important role in the toxicity to surrounding organisms. Based on the results obtained in this study, we consider that detailed analysis of live cell suspension or culture supernatant, especially focusing on cell surface structure or high molecular weight compounds, is necessary to clarify the toxic mechanisms of HAB species.
Disclosure statement
No potential conflict of interest was reported by the authors.
Additional information
Funding
Notes on contributors
Kichul Cho
K. Cho: wrote the paper. T. Kasaoka and M. Ueno: performed the experiments and analysed the data; revised the final version of the manuscript. L. Basti and Y. Yamasaki: revised the final version of the manuscript. D. Kim and T. Oda: original concept; revised final version of the manuscript.
Takuya Kasaoka
K. Cho: wrote the paper. T. Kasaoka and M. Ueno: performed the experiments and analysed the data; revised the final version of the manuscript. L. Basti and Y. Yamasaki: revised the final version of the manuscript. D. Kim and T. Oda: original concept; revised final version of the manuscript.
Mikinori Ueno
K. Cho: wrote the paper. T. Kasaoka and M. Ueno: performed the experiments and analysed the data; revised the final version of the manuscript. L. Basti and Y. Yamasaki: revised the final version of the manuscript. D. Kim and T. Oda: original concept; revised final version of the manuscript.
Leila Basti
K. Cho: wrote the paper. T. Kasaoka and M. Ueno: performed the experiments and analysed the data; revised the final version of the manuscript. L. Basti and Y. Yamasaki: revised the final version of the manuscript. D. Kim and T. Oda: original concept; revised final version of the manuscript.
Yasuhiro Yamasaki
K. Cho: wrote the paper. T. Kasaoka and M. Ueno: performed the experiments and analysed the data; revised the final version of the manuscript. L. Basti and Y. Yamasaki: revised the final version of the manuscript. D. Kim and T. Oda: original concept; revised final version of the manuscript.
Daekyung Kim
K. Cho: wrote the paper. T. Kasaoka and M. Ueno: performed the experiments and analysed the data; revised the final version of the manuscript. L. Basti and Y. Yamasaki: revised the final version of the manuscript. D. Kim and T. Oda: original concept; revised final version of the manuscript.
Tatsuya Oda
K. Cho: wrote the paper. T. Kasaoka and M. Ueno: performed the experiments and analysed the data; revised the final version of the manuscript. L. Basti and Y. Yamasaki: revised the final version of the manuscript. D. Kim and T. Oda: original concept; revised final version of the manuscript.
References
- Ahmed, M.S., Khan, S., Arakawa, O. & Onoue, Y. (1995). Properties of hemagglutinins newly separated from toxic phytoplankton. Biochimica et Biophysica Acta (BBA)-General Subjects, 1243: 509–512.
- Arzul, G., Bodennec, G., Gentien, P., Bornens, P. & Crassous, M.P. (1998). The effect of dissolved oxygen on the haemolytic property of Gymnodinium ichthyotoxins. In Harmful Algae (Reguera, B., Blanco, J., Fernandez, M.L. & Wyatt, T., editors), 611–614. Xunta de Galicia and Intergovernmental Oceanographic Commission of UNESCO, Vigo.
- Cho, K., Sakamoto, J., Noda, T., Nishiguchi, T., Ueno, M., Yamasaki, Y., Yagi, M., Kim, D. & Oda, T. (2016). Comparative studies on the fish-killing activities of Chattonella marina isolated in 1985 and Chattonella antiqua isolated in 2010, and their possible toxic factors. Bioscience, Biotechnology, and Biochemistry, 4: 811–817.
- Emura, A., Matsuyama, Y. & Oda, T. (2004). Evidence for the production of a novel proteinaceous haemolytic exotoxin by dinoflagellate Alexandrium taylori. Harmful Algae, 3: 29–37.
- Demura, M., Noël, M.-H., Kasai, F., Watanabe, M.M. & Kawachi, M. (2009). Taxonomic revision of Chattonella antiqua, C. marina and C. ovata (Raphidophyceae) based on their morphological characteristics and genetic diversity. Phycologia, 48: 518–535.
- Galimany, E., Sunila, I., Hégaret, H., Ramón, M. & Wikfors, G.H. (2008). Experimental exposure of the blue mussel (Mytilus edulis, L.) to the toxic dinoflagellate Alexandrium fundyense: histopathology, immune responses, and recovery. Harmful Algae, 7: 702–711.
- Gentien, P., Lunven, M., Lazure, P., Youenou, A. & Crassous, M.P. (2007). Motility and autotoxicity in Karenia mikimotoi (Dinophyceae). Philosophical Transactions of the Royal Society B, 362: 1937–1946.
- Hiroishi, S., Okada, H., Imai, I. & Yoshida, T. (2005). High toxicity of the novel bloom-forming species Chattonella ovata (Raphidophyceae) to cultured fish. Harmful Algae, 4: 783–787.
- Honjo, T. (1994). The biology and prediction of representative red tides associated with fish kills in Japan. Reviews in Fisheries Science, 2: 65–104.
- Hyslop, P.A. & Sklar, L.A. (1984). A quantitative fluorimetric assay for the determination of oxidant production by polymorphonuclear leukocytes: its use in the simultaneous fluorimetric assay of cellular activation processes. Analytical Biochemistry, 141: 280–286.
- Ishimatsu, A., Oda, T., Yoshida, M. & Ozaki, M. (1996). Oxygen radicals are probably involved in the mortality of yellowtail by Chattonella marina. Fisheries Science (Tokyo), 62: 836–837.
- Jenkinson, I.R. & Arzul, G. (2001). Mitigation by cysteine compounds of rheotoxicity, cytotoxicity and fish mortality caused by the dinoflagellates, Gymnodinium mikimotoi and G. cf. maguelonnense. In Harmful Algal Blooms 2000 (Hallegraeff, G.M., Blackburn, S.I., Bolch, C.J. and Lewis, R.J., editors), 71–84. IOC, Paris.
- Jüttner, F. (2001). Liberation of 5,8,11,14,17-eicosapentaenoic acid and other polyunsaturated fatty acids from lipids as a grazer defense reaction in epilithic diatom biofilms. Journal of Phycology, 37: 744–755.
- Kadomura, K., Nakashima, T., Kurachi, M., Yamaguchi, K. & Oda, T. (2006). Production of reactive oxygen species (ROS) by devil stinger (Inimicus japonicus) during embryogenesis. Fish and Shellfish Immunology, 21: 209–214.
- Kamiyama, T. (1997). Growth and grazing responses of tintinnid ciliates feeding on the toxic dinoflagellate Heterocapsa circularisquama. Marine Biology, 128: 509–515.
- Kamiyama, T. & Arima, S. (1997). Lethal effect of the dinoflagellate Heterocapsa circularisquama upon the tintinnid ciliate Favella taraikaensis. Marine Ecology Progress Series, 160: 27–33.
- Katsuo, D., Kim, D., Yamaguchi, K., Matsuyama, Y. & Oda, T. (2007). A new simple screening method for the detection of cytotoxic substances produced by harmful red tide phytoplankton. Harmful Algae, 6: 790–798.
- Khan, S., Arakawa, O. & Onoue, Y. (1996). A toxicological study of the marine phytoflagellate, Chattonella antiqua (Raphidophyceae). Phycologia, 35: 239–244.
- Kim, C.S., Lee, S.G., Lee, C.K., Kim, H.G. & Jung, J. (1999). Reactive oxygen species as causative agents in the ichthyotoxicity of the red tide dinoflagellate Cochlodinium polykrikoides. Journal of Plankton Research, 21: 2105–2115.
- Kim, D., Sato, Y., Oda, T., Muramatsu, T., Matsuyama, Y. & Honjo, T. (2000). Specific toxic effect of dinoflagellate Heterocapsa circularisquama on the rotifer Brachionus plicatilis. Bioscience, Biotechnology, and Biochemistry, 64: 2719–2722.
- Kim, D., Nakashima, T., Matsuyama, Y., Niwano, Y., Yamaguchi, K. & Oda, T. (2007). Presence of the distinct systems responsible for superoxide anion and hydrogen peroxide generation in red tide phytoplankton Chattonella marina and Chattonella ovata. Journal of Plankton Research, 29: 241–247.
- Kuroda, A., Nakashima, T., Yamaguchi, K. & Oda, T. (2005). Isolation and characterization of light-dependent haemolytic cytotoxin from harmful red tide phytoplankton Chattonella marina. Comparative Biochemistry and physiology-Part C: Toxicology & Pharmacology, 141: 297–305.
- Landsberg, J.H. (2002). The effects of harmful algal blooms on aquatic organisms. Reviews in Fisheries Science, 10: 113–390.
- Marshall, J.A., Nichols, P.D., Hamilton, B., Lewis, R.J. & Hallegraeff, G.M. (2003). Ichthyotoxicity of Chattonella marina (Raphidophyceae) to damselfish (Acanthochromis polycanthus): the synergistic role of reactive oxygen species and free fatty acids. Harmful Algae, 2: 273–281.
- Marshall, J.A., Ross, T., Pyecroft, S. & Hallegraeff, G.M. (2005). Superoxide production by marine microalgae. II. Towards understanding ecological consequences and possible functions. Marine Biology, 147: 541–549.
- Matsusato, T. & Kobayashi, H. (1974). Studies on death of fish caused by red tide. Bulletin of the Nansei Regional Fisheries Research Laboratory, 7: 43–67 (in Japanese with English abstract).
- Matsuyama, Y. (2012). Impacts of the harmful dinoflagellate Heterocapsa circularisquama bloom on shellfish aquaculture in Japan and some experimental studies on invertebrates. Harmful Algae, 14: 144–155.
- Matsuyama, Y., Uchida, T. & Honjo, T. (1997). Toxic effects of the dinoflagellate Heterocapsa circularisquama on clearance rate of the blue mussel Mytilus galloprovincialis. Marine Ecology Progress Series, 146: 73–80.
- Oda, T., Nakamura, A., Shikayama, M., Kawano, I., Ishimatsu, A. & Muramatsu, T. (1997). Generation of reactive oxygen species by raphidophycean phytoplankton. Bioscience, Biotechnology, and Biochemistry, 61: 1658–1662.
- Oda, T., Sato, Y., Kim, D., Muramatsu, T., Matsuyama, Y. & Honjo, T. (2001). Hemolytic activity of Heretocapsa circularisquama (Dinophyceae) and its possible involvement in shellfish toxicity. Journal of Phycology, 37: 509–516.
- Okaichi, T. (1989). Red tide problems in the Seto Inland Sea, Japan. In Red Tides – Biology, Environmental Science, and Toxicology (Okaichi, T., Anderson, D.M. & Nemoto, T., editors), 137–142. Elsevier, New York.
- Paerl, H.W. & Huisman, J. (2008). Blooms like it hot. Science, 320: 57–58.
- Palenik, B.P., Zafiriou, O.C. & Morel, F.M.M. (1987). Hydrogen peroxide production by a marine phytoplankter. Limnology and Oceanography, 32: 1365–1369.
- Satake, M., Tanaka, Y., Ishikura, Y., Oshima, Y., Naoki, H. & Yasumoto, T. (2005). Gymnocin-B with the largest contiguous polyether rings from the red tide dinoflagellate. Karenia (formerly Gymnodinium) mikimotoi. Tetrahedron Letters, 46: 3537–3540.
- Sephton, D.H., Haya, K., Martin, J.L., LeGresley, M.M. & Page, F.H. (2007). Paralytic shellfish toxins in zooplankton, mussels, lobsters and caged Atlantic salmon, Salmo salar, during a bloom of Alexandrium fundyense off Grand Manan Island, in the Bay of Fundy. Harmful Algae, 6: 745–758.
- Shimada, M., Akagi, N., Nakai, Y., Goto, H., Watanabe, M., Watanabe, H., Nakanishi, M., Yoshimatsu, S. & Ono, C. (1991). Free radical production by the red tide alga, Chattonella antiqua. Histochemical Journal, 23: 361–365.
- Takagi, T., Hayashi, K. & Itabashi, Y. (1984). Toxic effect of free unsaturated fatty acids in the mouse assay of diarrhetic shellfish toxin by intraperitoneal injection. Bulletin of the Japanese Society for the Science of Fish, 50: 1413–1418.
- Teegarden, G.J., Campbell, R.G., Anson, D.T., Ouellett, A., Westman, B.A. & Durbin, E.G. (2008). Copepod feeding response to varying Alexandrium spp. cellular toxicity and cell concentration among natural plankton samples. Harmful Algae, 7: 33–44.
- Yamasaki, Y., Kim, D.I., Matsuyama, Y., Oda, T. & Honjo, T. (2004). Production of superoxide anion and hydrogen peroxide by the red tide dinoflagellate Karenia mikimotoi. Journal of Bioscience and Bioengineering, 97: 212–215.
- Yamasaki, Y., Nagasoe, S., Matsubara, T., Shikata, T., Shimasaki, Y., Oshima, Y. & Honjo, T. (2007). Allelopathic interactions between the bacillariophyte Skeletonema costatum and the raphidophyte Heterosigma akashiwo. Marine Ecology Progress Series, 339: 83–92.
- Yamasaki, Y., Katsuo, D., Nakayasu, S., Salati, C., Duan, J., Zou, Y., Matsuyama, Y., Yamaguchi, K. & Oda, T. (2008). Purification and characterization of a novel high molecular weight exotoxin produced by red tide phytoplankton, Alexandrium tamarense. Journal of Biochemical and Molecular Toxicology, 22: 405–415.
- Yasumoto, T., Underdal, B., Aune, T., Hormazabal, V., Skulberg, O.M. & Oshima, Y. (1990). Screening for haemolytic and ichthyotoxic components of Chrysochromulina polylepis and Gymnodinium aureolum from Norwegian coastal waters. In Toxic Marine Phytoplankton (Granéli, E., Sundstrom, B., Edler, L. & Anderson, D.M., editors), 436–440. Elsevier, New York.
- Zou, Y., Yamasaki, Y., Matsuyama, Y., Yamaguchi, K., Honjo, T. & Oda, T. (2010). Possible involvement of haemolytic activity in the contact-dependent lethal effects of the dinoflagellate Karenia mikimotoi on the rotifer Brachionus plicatilis. Harmful Algae, 9: 367–373.