ABSTRACT
Glycosaminoglycan (GAG), an important component of proteoglycan (PG), was biosynthesized with the initiation by peptide O-xylosyltransferase. O-xylosyltransferase activity was presented as a marker for increased PG synthesis. In the present study, a novel O-xylosyltransferase (OXT) gene was identified from Pinctada fucata martensii (PmOXT). The PmOXT-deduced protein sequence carried a typical water-soluble carbohydrate domain, branch domain, and xylosyltransferase domain. Homologous analysis of PmOXT presented the conserved DXD motif and catalytic structure characteristics. Phylogenetic tree analysis showed the traditional taxonomy and PmOXT clustered with Crassostrea gigas. PmOXT was expressed in all the detected tissues and developmental stages. PmOXT had a significantly higher expression level in the shell formation associated tissues and developmental stages. PmOXT expressed significantly decreased in the central zone of the mantle and marginal zone of the mantle after RNA interference; additionally, OXT activity and GAG content in the extrapallial fluid were significantly reduced compared with the control. Furthermore, the crystal tablets of prismatic layer displayed obvious holes and disordered crystals with obviously rough surface and irregular crystal tablets were observed in the nacre after RNAi. Results suggested that PmOXT affected the shell formation by influencing the formation of GAGs in the process of addition to the core proteins.
1. Introduction
Proteoglycans (PGs), which consist of a core protein and long glycosaminoglycan (GAG) chains, present as polyanionic molecules, as widely observed in the cells of most of the tissues (Ruoslahti Citation1989; Silbert and Sugumaran Citation1995). PGs as acidic macromolecules are used as a nucleation factor for mollusk shell formation (Furuhashi et al. Citation2010). Two kinds of acidic macromolecules, namely, acidic proteins (Weiner and Hood Citation1975) and sulfated sugars (Krampitz et al. Citation1983; Hao et al. Citation2018) are widely recognized. GAGs are an important component of PGs and widely exist in carbonate biominerals, including mollusks (Dauphin et al. Citation2005; Nudelman et al. Citation2006), corals (Cuif et al. Citation2003, Citation2005), and avian eggshells (Dauphin et al. Citation2006), and present potential in biomineralization materials.
O-xylosyltransferase participates in the catalyzation of the transfer process of the d-xylose group from uridine diphosphate (UDP)-D-xylose to serine residues of the core protein (Rodén Citation1980; Kuhn et al. Citation2001). GAG, as an important component of PGs, is biosynthesized with the initiation by peptide O-xylosyltransferases (OXT) (Kearns et al. Citation1991; Kjellen and Lindahl Citation1991). Xylosyltransferase (XYLT) activity has been observed as a marker for increased PG biosynthesis (Weilke et al. Citation1997). In mammals, two active xylosyltransferase isoenzymes (XYLT I and II) have been found, and both hold the function of catalyzation for the transfer of xylose to the PG core protein (Kuhn et al. Citation2015). Moreover, XYLT gene has a potential role in skeleton-associated diseases, such as cartilage matrix production, and ultimately regulates the timing of skeletal development (Eames et al. Citation2011) and systemic sclerosis (Gotting et al. Citation1999). XYLT activity variation in human serum present with direct association with various diseases, such as osteoarthritis (Kuhn et al. Citation2015). Compared with mammals, only one O-xylosyltransferase gene exists in Drosophila melanogaster, and considering the key roles of PGs, and we designated the oxt gene as essential for viability (Wilson Citation2002).
In the present study, a novel xylosyltransferase oxt gene, which is the only one in the genome of Pinctada fucata martensii (Du et al. Citation2017), was cloned and analyzed for its potential function in the pearl oyster in order to provide fundamental molecular knowledge regarding pearl production.
2. Materials and methods
2.1. Experimental animals
Pearl oysters P. f. martensii (approximately 2 years) were collected from a commercial farm in Xuwen, Guangdong Province, China (20°250′ N, 109°570′ E).
2.2. Cloning and bioinformatic analysis of Pinctada fucata martensii (PmOXT) gene
A partial sequence of the xylosyltransferase oxt gene used for cloning was acquired from P. f. martensii genome data (Du et al. Citation2017). The 5′ and 3′ ends of PmOXT were obtained by using the SMART RACE cDNA amplification kit (Takara, Dalian, China) using the method of RACE action. Primers used for the cloning of PmOXT are shown in .
Table 1 Primers used in this study
The full-length sequence of PmOXT was subjected for bioinformatic analysis, which included ORF Finder, domain analysis of SMART, multiple alignment with ClustalX and protein domain model construction of Swiss model. Protein sequence of PmOXT and OXT from Crassostrea gigas (EKC32292.1) were displayed as 3D models by using Chimera 1.8.1. Phylogenetic tree construction of xylosyltransferase sequences from different species was displayed by using MEGA 6.1 using the NJ (neighbour-joining) method.
2.3. PmOXT expression pattern at tissues and developmental stages
Eight individuals were used for the analysis of expression pattern of PmOXT. Sampled tissues included adductor muscle (A), gill (GI), haemocyte (B), hepatopancreas (HE), marginal zone of mantle (ME), and central zone of mantle (MC). Samples were collected at different developmental stages with three replicates, which included fertilized egg, blastula, gastrula, trochophore, D-stage larvae, early umbolarvae, eyed larvae, and spat.
Total RNA of samples was extracted with Trizol reagent (Invitrogen, Carlsbad, CA, USA). The cDNA was synthesized with M-MLV First-strand cDNA Synthesis Kit (Invitrogen). Primers for the qRT-PCR analysis were presented in . The expression of PmOXT at tissues and development stages was calculated by using the 2−ΔCt method with glyceraldehyde 3-phosphate dehydrogenase (GAPDH) gene as reference gene.
2.4. PmOXT function analysis after RNA interference (RNAi)
RNAi experiment was performed following the procedures of Hao et al. (Citation2018) with some modifications. An experimental group (EG) and a control group (CG) were designed in the experiment. 10 oysters were used for each group. Primers were designed and listed in . dsRNAs were synthesized using T7 High-Efficiency Transcription Kit and EasyPure rRNA Purification Kit (Beijing TransGen Biotech Co., Ltd.), the dsRNAs were diluted to 60 μg/100 μL with RNase-free water.
100 μL of dsRNA-PmOXT and 100 μL dsRNA-RFP were separately injected into the adductor muscle of pearl oysters from EG and CG. On days 6 after injection, pearl oysters of each group were sacrificed and ME and MC were sampled for expression analysis of PmOXT.
Extrapallial fluid (EPF) of each oyster was collected on days 6 after injection (Yan et al. Citation2014). Changes of OXT activity and GAG content in the EPF after RNAi were determined by using microtitration assay via the EnSpire microplate spectrophotometer (PerkinElmer, USA). Changes of OXT activity in the EPF were tested by using a commercial kit (Beijing Dongge Weiye Technology Co., Ltd., Beijing, China). Changes of GAG content in the EPF were tested with Blyscan-sulfated glycosaminoglycan assay kit (Biocolor Life Science Assays; Labtek, West Ipswich, QLD, Australia) with absorbance set at 656 nm (Elder and Athanasiou Citation2008). Shells (0.6 cm × 0.6 cm) after RNAi were observed by scanning electron microscopy (JSM-6300 LV) without disease.
2.5. Statistical analysis
Expression levels of PmOXT at tissues and developmental stages were compared by one-way ANOVA. Differences in PmOXT expression, GAG content and OXT activity between the two groups were evaluated by T-test. All statistical analysis was conducted using SPSS 19.0 (IBM, Chicago, IL, USA). The significant level for these analyses was set at P < 0.05.
3. Results
3.1. Full length of PmOXT
The full-length of PmOXT was 3129 bp with 5′-(496 bp) and 3′-untranslated region (UTR) (431 bp). cDNA sequence of PmOXT held an ORF of 2202 bp and encoded a polypeptide of 733 residues. The estimated molecular mass and theoretical isoelectric point of PmOXT were 84.45 kDa and 8.24, respectively. The deduced protein sequence of PmOXT featured an xylosyltransferase domain (390–564 aa), a WSC domain (5–80 aa), and a branch domain (105–358 aa) ().
Figure 1 Nucleotide sequence analysis of PmOXT. (A) shows nucleotide sequence and deduced amino acids of PmOXT. 5′- and 3′-UTR are indicated by small letters. ORF and deduced amino acid (aa) sequences are indicated by capital letters. Nucleotides with borders correspond to the initiation codon (ATG) and stop codon (TGA). Sequence in yellow background represents the xylosetransferase domain. The aa in grey signifies branch domain. The underlined aa represents the WSC domain.
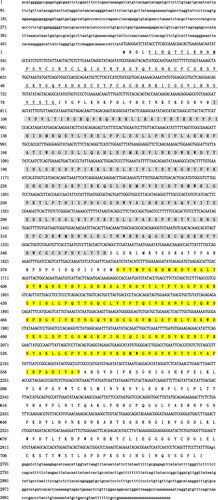
3.2. Homologous analysis and PmOXT structure
The PmOXT-deduced protein was homologous to the xylosyltransferase oxt family. Homologous analysis of PmOXT was performed and was compared with the genes of C. gigas oxt (EKC32292.1), Aplysia californica oxt (XP_012944960.1), Mizuhopecten yessoensis oxt (OWF50901.1), and Lingula anatina oxt (XP_013390783.1). The result indicated that PmOXT exhibited a conserved function domain with other OXT of other species (). Moreover, the PmOXT identities were 53%, 54%, 50%, and 46% compared with those of C. gigas, M. yessoensis, A. californica, and L. anatina, respectively. The predicted structural organization of OXT were performed among C. gigas [EKC32292.1], A. californica [XP_012944960.1], L. anatina [XP_013390783.1], Homo sapiens [NP_071449.1 and NP_071450.2], and Mus musculus [NP_783576.2 and NP_665827.2]. PmOXT protein sequence showed the conservation compared with other species mentioned above (). The protein sequences of PmOXT and OXT from C. gigas were submitted to Swiss-model model construction. Ribbon representation of full protein sequence represented the conservation of advanced structure ((A,B)). Comparation of the xylosyltransferase domain between the two species showed the catalytic domain held similar structure and DXD motif which indicated their function conservation ((C,D)).
Figure 2 Multiple-sequence alignment of PmOXT aa sequences. Dark blue background indicates conserved aa; pink background indicates aa with strong similarity; light blue indicates aa with weak similarity; the right numbers show position of sequence alignment aa. Bracket presents DXD motif. The accession numbers of the sequences used in this alignment are as follows: Crassostrea gigas (EKC32292.1), Aplysia californica (XP_012944960.1), Mizuhopecten yessoensis (OWF50901.1), and Lingula anatina (XP_013390783.1).
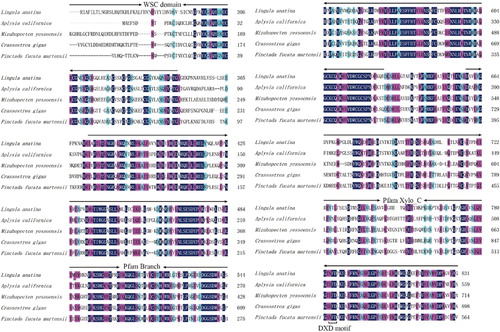
Figure 3 Predicted structural organization of XYLT among different species. Accession number for sequences used in this alignment are: Crassostrea gigas (EKC32292.1), A. californica (XP_012944960.1), L. anatina (XP_013390783.1), Homo sapiens (NP_071449.1 and NP_071450.2), and Mus musculus (NP_783576.2 and NP_665827.2).
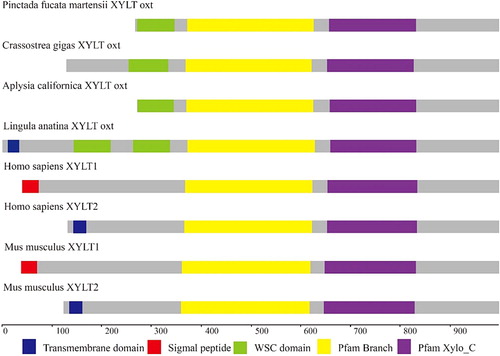
Figure 4 Ribbon representation of structures of PmOXT and OXT from C. gigas (EKC32292.1). (A) and (B) show the protein sequence of PmOXT and OXT of C. gigas, respectively. (C) and (D) represent the xylosyltransferase domain of PmOXT and OXT of C. gigas, respectively. WSC domain, branch domain, and xylosyltransferase domain are shown in green, yellow, and purple, respectively. DXD motif is represented in red. The C and N in the figures show the C- and N-terminal of sequences, respectively.
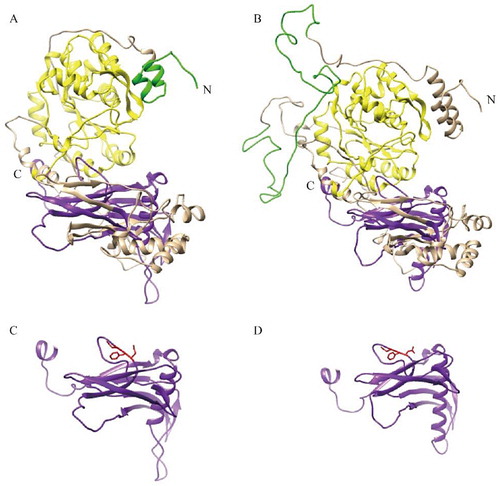
3.3. Phylogenetic analysis of XYLT
To examine the relationships among XYLT1, XYLT2, and OXT, phylogenetic trees were generated with 1000 bootstrap replicates. The tree showed that XYLT1 and XYLT2 from vertebrates and xylosyltransferase oxt from invertebrates were separated into two branches, which were consistent with traditional taxonomy. PmOXT clustered with C. gigas OXT ().
Figure 5 Phylogenetic tree of XYLT among different species. The phylogenetic tree is constructed using MEGA software 6.05 by the NJ method and 1000 replications of bootstrap. The scale bar indicates a branch length of 0.1. The XYLT protein sequences used for phylogenetic analysis include the following: Crassostrea gigas (EKC32292.1), A. californica (XP_012944960.1), L. anatina (XP_013390783.1), Homo sapiens (NP_071449.1 and NP_071450.2) and Mus musculus (NP_783576.2 and NP_665827.2), Danio rerio (NP_001165868.1 and NP_001124250.1), Gallus gallus (AAT44331.1 and NP_001001785.1), Lonchura striata domestica (OWK61928.1 and XP_021410469.1), Cimex lectularius (XP_014257441.1), Drosophila willistoni (XP_002067754.1), Bactrocera latifrons (JAI44494.1), Blattella germanica (PSN41238.1), and Drosophila erecta (XP_001971258.1).
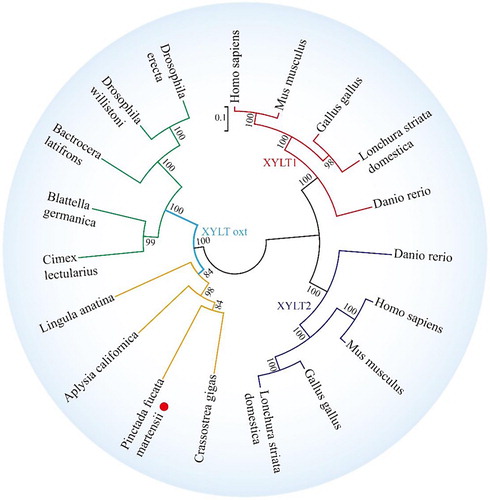
3.4. Expression pattern of PmOXT
PmOXT mRNA was ubiquitously expressed at sampled tissues ((A)). ME held significantly higher expression level than other tissues (P < 0.05). PmOXT was expressed at different developmental stages. PmOXT expression was significantly higher at trochophore and spat stages than other stages (P < 0.05) ((B)).
Figure 6 Expression pattern of PmOXT in P. f. martensii. (A) Expression pattern of PmOXT in different tissues. (B) Expression pattern of PmOXT in developmental stages. Notes: ME: Marginal zone of mantle, MC: Central zone of mantle, A: Adductor muscle, HE: Hepatopancreas, GI: Gill, B: Haemocyte, Fe: fertilized egg, B: blastula, G: gastrula, T: trochophore, D: D-stage larvae, EU: early umbo larvae, EL: eyed larvae, and S: spat. Mean values with different letters are significantly different as determined through one-way ANOVA. The vertical bar represents standard deviation (P < 0.05).
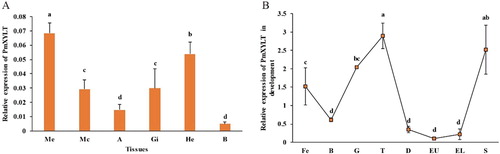
3.5. PmOXT function analysis in shell formation
A RNAi experiment was performed to evaluate the function of PmOXT in shell formation. PmOXT mRNA expression levels in the EG significantly decreased by 62.97% and 35.79% in the ME and MC, respectively, relative to the CG (P < 0.05) ((A,B)).
Figure 7 Influence of RNA interference on PmOXT and shell formation. (A) and (B) show PmOXT expression in ME and MC, respectively. (C) and (D) show the enzyme activity of OXT and content of GAGs in the extrapallial fluid after RNAi, respectively. Mean values with different letters are significantly different as determined through T-test. The vertical bar represents standard deviation (P < 0.05). (E) shows the microstructure of shells after RNAi. (E)-1 and (E)-2 show shell prismatic layer of dsRNA-RFP and dsRNA-PmOXT group after RNAi, respectively. (E)-3 and (E)-4 present shell nacre of dsRNA-RFP and dsRNA-PmOXT group after RNAi, respectively. Bars represent 50 and 5 µm scale in images of (E)-1, 2 and (E)-3, 4, respectively.
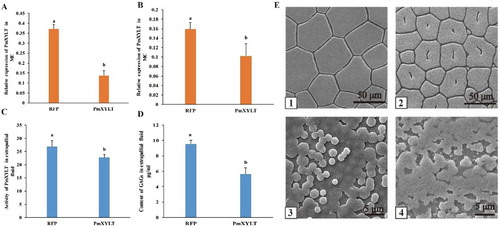
OXT activity was determined in the EPF at the end of RNAi experiment. OXT activity in the EG significantly decreased compared with the CG (P < 0.05) ((C)). Similarly, GAGs content in the EG significantly decreased compared with the CG (P < 0.05) ((D)).
Microstructure of shell inner surface was scanned after RNAi experiment. The prismatic layer and nacre in the CG displayed well-defined microstructure. Hexangular and pentangular column calcite crystals were highly well arranged in the structure of prismatic layer ((E-1)). In the nacre, hexagonal aragonite flat tablets were regularly packed together to form a stair-shaped structure ((E-3)). On the contrary, the crystal tablets of prismatic layer displayed obvious holes in the EG ((E-2)). Disordered crystals with obviously rough surface and irregular crystal tablets were observed in the nacre ((E-4)). These results indicated that PmOXT was involved in shell formation in the species.
4. Discussion
Xylosyltransferases catalyse the initiation of glycosaminoglycan chain addition to PG core proteins, which are important for normal PG function (Mis et al. Citation2014). Vertebrates have two similar genes that encode xylosyltransferase I and xylosyltransferase II. However, Caenorhabditis and Drosophila have only one peptide O-xylosyltransferase gene each (Wilson Citation2004). In the present study, a novel xylosyltransferase gene from P. f. martensii was cloned to show its versatile function in the GAG synthesis and shell formation in the pearl oyster.
The DXD motif is conserved in glycosyltransferase families (Breton et al. Citation1998; Wiggins and Munro Citation1998) and is critical for enzyme activity (Busch et al. Citation1998; Munro and Freeman Citation2000). DXD motif with mutation analysis indicated that not all the DXD motifs hold identical functions for enzyme activity (Charnock and Davies Citation1999; Munro and Freeman Citation2000; Li et al. Citation2001). Gotting et al. (Citation2004) reported the potential function of two DXD motifs (314–316 and 745–747, located in the N- and C-terminal regions, respectively) in the XT-I. They found the changes in the 745DWD747 motif or the N-motif result in decreased enzyme activity (Gotting et al. Citation2004). In the present study, the DXD motif was observed in the C-terminal region in the multiple-sequence alignment and ribbon representation of PmOXT, which has been reported that involved in the enzyme activity (Gotting et al. Citation2004). For the analysis of sequence characteristics of PmOXT, its primary, secondary, and structures were presented. The primary structure showed that PmOXT held a combined organization that included the WSC, branch, and xylosyltransferase domains. This similar structure organization of XYLT from vertebrate and invertebrate animals revealed their potential conserved function. The phylogenetic analysis of XYLT protein sequences showed that PmOXT was clustered with C. gigas oxt. Our studies indicated that PmOXT might be a candidate gene for GAG and PG synthesis in the species.
PGs are an important component of the extracellular matrix and widely exist in various cells and basement membranes, which are necessary for holding the structural integrity of the connective tissue; moreover, PG participates in cell adhesion, motility, differentiation, and morphogenesis (Ruoslahti Citation1989). Xylosyltransferase is the initial enzyme for PG biosynthesis. The present results showed ubiquitous expression of PmOXT at sampled tissues, which indicated that the PGs may be multifunctional in the species. In mollusks, mantle epidermal cells secrete matrix proteins to control the CaCO3 polymorph (calcite, aragonite), the size, the shapes of the crystallites, and finally, the texture of the shell (Marin and Luquet Citation2004; Zheng et al. Citation2017). In terms of the shell structure of the bivalve pearl oyster, it includes two mineralized layers: The inner aragonite nacre and the outer calcite prismatic layer, which are mainly regulated by the matrix proteins secreted from the mantle central and the mantle edge, respectively (Kinoshita et al. Citation2011; Yan et al. Citation2014; Tian et al. Citation2015). Significantly, higher expression level of PmOXT in the ME and MC than other tissues indicate that PmOXT might play a potential role in the shell formation through influencing the cellular matrix of the mantle cell (Fang et al. Citation2011).
PGs are the main components of the extracellular matrix (ECM) (Mariman and Wang Citation2010; Hao et al. Citation2018). Integrins link the ECM to the cytoskeleton and play important roles in controlling various steps in the signalling pathways that regulate processes such as proliferation, differentiation, apoptosis, and cell migration and implantation, in various species (Bosman and Stamenkovic Citation2003; Reddy and Mangale Citation2003; Hao et al. Citation2019). During the development of P. f. martensii, PmOXT expressed in each stage which showed PGs had an important function in larvae growth and development. Transcriptome analysis of development from pearl oyster P. f. martensii and C. gigas represented the PGs syntheses associated genes widely expressed in the development of bivalves (Du et al. Citation2017; Zheng et al. Citation2019). Furthermore, Chondroitin and heparan sulfates have key functions in animal development, and their synthesis is initiated by the action of xylosyltransferase (Brunner et al. Citation2006). Normal foetal skeletal growth is dependent on the activity of xylosyltransferase in the cartilage during the last one-half of gestation (Morriss et al. Citation1985). During the development of P. f. martensii, prodissoconch formed at trochophore stage and dissoconch formed at juvenile stage (Kakoi et al. Citation2008; Miyazaki et al. Citation2010; Zheng et al. Citation2019). Genes, such as sulfotransferase, VWA (von Willebrand factor A)-containing protein, and chitin synthase genes, that have increased expression levels in both developmental stages (trochophore stage and spat stage) of P. f. martensii have been reported to have a potential role in the matrix protein and shell formation (Du et al. Citation2017). In the present study, PmOXT displayed similar expression pattern with above genes (sulfotransferase, VWA-containing protein, and chitin synthase genes) (Du et al. Citation2017; Hao et al. Citation2018). The present results suggested that PmOXT be a candidate gene involved in shell formation of the species.
Mollusks shells contained calcium carbonate and organic matrix materials. Organic matrix is primarily essential for regulating the polymorph, size, and shape of crystallites (Marin and Luquet Citation2004). Organic matrices such as proteins, polysaccharides, and lipids, have been well studied (Farre and Dauphin Citation2009; Suzuki et al. Citation2009). Researchers reported several matrix proteins, which are helpful to understand the mechanism of biomineralization of shell and pearl formation (Suzuki et al. Citation2009; Fang et al. Citation2011). RNAi is a mechanism for the selective inhibition of expression of a gene (Kim and Rossi Citation2008). Previous studies reported that RNAi could reduce the mRNA expression levels of XYLT1 and XYLT2 and thus decreases the protein levels that these genes encode (Dziedzic et al. Citation2010). In the present study, RNAi was performed to explore the PmOXT function in the pearl oyster. The expression levels of PmOXT mRNA in the ME and MC significantly decreased after injection of dsRNA-PmOXT. In bivalves, mantle tissue secreted organic matrix and formed the EPF that participates in the biomineralization (Young et al. Citation1977). The inhibition of PmOXT mRNA decreased the OXT activity and GAG content in the extrapallial fluid and thus resulted in irregular shell microstructure. The pug (a recessive dwarf mouse mutant) mutation disrupted XT1 activity and subsequently resulted in a reduction of GAG chains (Mis et al. Citation2014). GAGs with numerous negative sulfate groups can enrich the content of Ca2+ and reach a state of supersaturation that is essential for nucleation on crystal formation (Addadi et al. Citation1987; Fernandez et al. Citation2007). The soluble matrical fraction from mollusks consists of sulfated groups and high molecular-weight glycoproteins; some of them participated in the process of binding calcium (Krampitz et al. Citation1983). Therefore, PmOXT inhibition resulted in decreased GAGs and affected the shell formation in pearl oysters. These results suggested the PmOXT be involved in shell formation in the pearl oyster P. f. martensii.
Disclosure statement
No potential conflict of interest was reported by the authors.
Additional information
Funding
References
- Addadi L , Moradian J , Shay E , Maroudas NG , Weiner S. 1987. A chemical model for the cooperation of sulfates and carboxylates in calcite crystal nucleation: relevance to biomineralization. Proc Natl Acad Sci USA. 84:2732–2736. doi: 10.1073/pnas.84.9.2732
- Bosman FT , Stamenkovic I. 2003. Functional structure and composition of the extracellular matrix. J Pathol. 200(4):423–428. doi: 10.1002/path.1437
- Breton C , Bettler E , Joziasse DH , Geremia RA , Imberty A. 1998. Sequence-function relationships of prokaryotic and eukaryotic galactosyltransferases. J Bio Chem. 123:1000–1009.
- Brunner A , Kolarich D , Voglmeir J , Paschinger K , Wilson IBH. 2006. Comparative characterisation of recombinant invertebrate and vertebrate peptide O-xylosyltransferases. Glycoconj J. 23:543–554. doi: 10.1007/s10719-006-7633-z
- Busch C , Hofmann F , Selzer J , Munro S , Jeckel D , Aktories K. 1998. A common motif of eukaryotic glycosyltransferases is essential for the enzyme activity of large clostridial cytotoxins. J Biol Chem. 273:19566–19572. doi: 10.1074/jbc.273.31.19566
- Charnock SJ , Davies GJ. 1999. Structure of the nucleotide-diphospho-sugar transferase, SpsA from Bacillus subtilis, in native and nucleotide-complexed forms. Biochemistry. 38:6380–6385. doi: 10.1021/bi990270y
- Cuif JP , Dauphin Y. 2005. The Environment Recording Unit in coral skeletons – a synthesis of structural and chemical evidences for a biochemically driven, stepping-growth process in fibres. Biogeosciences. 2:61–73. doi: 10.5194/bg-2-61-2005
- Cuif JP , Dauphin Y , Doucet J , Salome M , Susini J. 2003. XANES mapping of organic sulfate in three scleractinian coral skeletons. Geochim Cosmochim Acta. 67:75–83. doi: 10.1016/S0016-7037(02)01041-4
- Dauphin Y , Cuif JP , Salome M , Susini J , Williams CT. 2006. Microstructure and chemical composition of giant avian eggshells. Anal Bioanal Chem. 386:1761–1771. doi: 10.1007/s00216-006-0784-8
- Dauphin Y , Cuif JP , Salome C , Susini J. 2005. Speciation and distribution of sulfur in a mollusk shell as revealed by in situ maps using X-ray absorption near-edge structure (XANES) spectroscopy at the SK-edge. Am Mineral. 90:1748–1758. doi: 10.2138/am.2005.1640
- Du X , Fan G , Jiao Y , Zhang H , Guo X , Huang R , Zheng Z , Bian C , Deng Y , Wang Q , et al. 2017. The pearl oyster Pinctada fucata martensii genome and multi-omic analyses provide insights into biomineralization. Gigascience. 6:1–12. doi: 10.1093/gigascience/gix059
- Dziedzic D , Wegrzyn G , Jakobkiewicz-Banecka J. 2010. Impairment of glycosaminoglycan synthesis in mucopolysaccharidosis type IIIA cells by using siRNA: a potential therapeutic approach for Sanfilippo disease. Eur J Hum Genet. 18:200–205. doi: 10.1038/ejhg.2009.144
- Eames BF , Yan Y , Swartz ME , Levic DS , Knapik EW , Postlethwait JH , Kimmel CB. 2011. Mutations in fam20b and xylt1 reveal that cartilage matrix controls timing of endochondral ossification by inhibiting chondrocyte maturation. Plos Genet. 7:e10022468. doi: 10.1371/journal.pgen.1002246
- Elder BD , Athanasiou KA. 2008. Synergistic and additive effects of hydrostatic pressure and growth factors on tissue formation. Plos One. 3:e23416. doi: 10.1371/journal.pone.0002341
- Fang D , Xu G , Hu Y , Pan C , Xie L , Zhang R. 2011. Identification of genes directly involved in shell formation and their functions in pearl oyster, Pinctada fucata . Plos One. 6:e218607.
- Farre B , Dauphin Y. 2009. Lipids from the nacreous and prismatic layers of two Pteriomorphia mollusc shells. Comp Biochem Physiol B Biochem Mol Biol. 152:103–109. doi: 10.1016/j.cbpb.2008.10.003
- Fernandez MS , Arriagada K , Arias JL. 2007. SEM localization of proteoglycans in abalone shell (Haliotis rufescens). Microsc Microanal. 13:1462–1463. doi: 10.1017/S1431927607071747
- Furuhashi T , Miksik I , Smrz M , Germann B , Nebija D , Lachmann B , Noe C. 2010. Comparison of aragonitic molluscan shell proteins. Comp Biochem Physiol B Biochem Mol Biol. 155:195–200. doi: 10.1016/j.cbpb.2009.11.007
- Gotting C , Muller S , Schottler M , Schon S , Prante C , Brinkmann T , Kuhn J , Kleesiek K. 2004. Analysis of the DXD motifs in human xylosyltransferase I required for enzyme activity. J Biol Chem. 279:42566–42573. doi: 10.1074/jbc.M401340200
- Gotting C , Sollberg S , Kuhn J , Weilke C , Huerkamp C , Brinkmann T , Krieg T , Kleesiek K. 1999. Serum xylosyltransferase: a new biochemical marker of the sclerotic process in systemic sclerosis. J Invest Dermatol. 112:919–924. doi: 10.1046/j.1523-1747.1999.00590.x
- Hao R , Zheng Z , Wang Q , Du X , Deng Y , Huang R. 2018. Molecular and functional analysis of PmCHST1b in nacre formation of Pinctada fucata martensii . Comp Biochem Physiol B Biochem Mol Biol. 225:13–20. doi: 10.1016/j.cbpb.2018.06.007
- Hao R , Zheng Z , Wang Q , Du X , Deng Y. 2019. Integrated application of transcriptomics and metabolomics provides insights into unsynchronized growth in pearl oyster Pinctada fucata martensii . Sci Total Environment. 666:46–56. doi: 10.1016/j.scitotenv.2019.02.221
- Kakoi S , Kin K , Miyazaki K , Wada H. 2008. Early development of the Japanese spiny oyster (Saccostrea kegaki): characterization of some genetic markers. Zoolog Sci. 25:455–464. doi: 10.2108/zsj.25.455
- Kearns AE , Campbell SC , Westley J , Schwartz NB. 1991. Initiation of chondroitin sulfate biosynthesis: a kinetic analysis of UDP-D-xylose: core protein beta-D-xylosyltransferase. Biochemistry. 30:7477–7483. doi: 10.1021/bi00244a016
- Kim DH , Rossi JJ. 2008. RNAi mechanisms and applications. Biotechniques. 44:613–616. doi: 10.2144/000112792
- Kinoshita S , Wang N , Inoue H , Maeyama K , Okamoto K , Nagai K , Kondo H , Hirono I , Asakawa S , Watabe S. 2011. Deep sequencing of ESTs from nacreous and prismatic layer producing tissues and a screen for novel shell formation-related genes in the pearl oyster. Plos One. 6:e212386.
- Kjellen L , Lindahl U. 1991. Proteoglycans: structures and interactions. Annu Rev Biochem. 60:443–475. doi: 10.1146/annurev.bi.60.070191.002303
- Krampitz G , Drolshagen H , Häusle J , Hof-Irmscher K. 1983. Organic matrices of mollusc shells. Biominer Biol Metal Accumulation. 231–247. doi: 10.1007/978-94-009-7944-4_20
- Kuhn J , Goetting C , Beahm BJ , Bertozzi CR , Faust I , Kuzaj P , Knabbe C , Hendig D. 2015. Xylosyltransferase II is the predominant isoenzyme which is responsible for the steady-state level of xylosyltransferase activity in human serum. Biochem Biophys Res Commun. 459:469–474. doi: 10.1016/j.bbrc.2015.02.129
- Kuhn J , Gotting C , Schnolzer M , Kempf T , Brinkmann T , Kleesiek K. 2001. First isolation of human UDP-D-xylose: proteoglycan core protein beta-D-xylosyltransferase secreted from cultured JAR choriocarcinoma cells. J Biol Chem. 276:4940–4947. doi: 10.1074/jbc.M005111200
- Li J , Rancour DM , Allende ML , Worth CA , Darling DS , Gilbert JB , Menon AK , Young WWJ. 2001. The DXD motif is required for GM2 synthase activity but is not critical for nucleotide binding. Glycobiology. 11:217–229. doi: 10.1093/glycob/11.3.217
- Mariman ECM , Wang P. 2010. Adipocyte extracellular matrix composition, dynamics and role in obesity. Cell Mol Life Sci. 67(8):1277–1292. doi: 10.1007/s00018-010-0263-4
- Marin F , Luquet G. 2004. Molluscan shell proteins. CR Palevol. 3:469–492. doi: 10.1016/j.crpv.2004.07.009
- Mis EK , Jr Liem KE , Kong Y , Schwartz NB , Domowicz M , Weatherbee SD. 2014. Forward genetics defines Xylt1 as a key, conserved regulator of early chondrocyte maturation and skeletal length. Dev Biol. 385:67–82. doi: 10.1016/j.ydbio.2013.10.014
- Miyazaki Y , Nishida T , Aoki H , Samata T. 2010. Expression of genes responsible for biomineralization of Pinctada fucata during development. Comp Biochem Physiol B Biochem Mol Biol. 155:241–248. doi: 10.1016/j.cbpb.2009.11.009
- Morriss FHJ , Fitzgerald B , Riddle LM. 1985. Fetal cartilage xylosyltransferase activity and skeletal growth in sheep. Pediatr Res. 19:1240–1243. doi: 10.1203/00006450-198512000-00003
- Munro S , Freeman M. 2000. The notch signalling regulator fringe acts in the Golgi apparatus and requires the glycosyltransferase signature motif DXD. Curr Biol: CB. 10:813–820. doi: 10.1016/S0960-9822(00)00578-9
- Nudelman F , Gotliv BA , Addadi L , Weiner S. 2006. Mollusk shell formation: mapping the distribution of organic matrix components underlying a single aragonitic tablet in nacre. J Struct Biol. 153:176–187. doi: 10.1016/j.jsb.2005.09.009
- Reddy KVR , Mangale SS. 2003. Integrin receptors: the dynamic modulators of endometrial function. Tissue Cell. 35(4):260–273. doi: 10.1016/S0040-8166(03)00039-9
- Rodén L. 1980. Structure and metabolism of connective tissue proteoglycans. Biochem Glycoproteins Proteoglycans. 267–271. doi: 10.1007/978-1-4684-1006-8_7
- Ruoslahti E. 1989. Proteoglycans in cell regulation. J Biol Chem. 264:13369–13372.
- Silbert JE , Sugumaran G. 1995. Intracellular membranes in the synthesis, transport, and metabolism of proteoglycans. Biochim Biophys Acta. 1241:371–384. doi: 10.1016/0304-4157(95)00011-9
- Suzuki M , Saruwatari K , Kogure T , Yamamoto Y , Nishimura T , Kato T , Nagasawa H. 2009. An acidic matrix protein, Pif, is a key macromolecule for nacre formation. Science. 325:1388–1390. doi: 10.1126/science.1173793
- Tian R , Zheng Z , Huang R , Jiao Y , Du X. 2015. miR-29a participated in nacre formation and immune response by targeting Y2R in Pinctada martensii . Int J Mol Sci 16:29436–29445. doi: 10.3390/ijms161226182
- Weilke C , Brinkmann T , Kleesiek K. 1997. Determination of xylosyltransferase activity in serum with recombinant human Bikunin as acceptor. Clin Chem. 43:45–51.
- Weiner S , Hood L. 1975. Soluble protein of the organic matrix of mollusk shells: a potential template for shell formation. Science. 190:987–989. doi: 10.1126/science.1188379
- Wiggins CA , Munro S. 1998. Activity of the yeast MNN1 alpha-1,3-mannosyltransferase requires a motif conserved in many other families of glycosyltransferases. P Natl Acad Sci Usa. 95:7945–7950. doi: 10.1073/pnas.95.14.7945
- Wilson I. 2002. Functional characterization of Drosophila melanogaster peptide O-xylosyltransferase, the key enzyme for proteoglycan chain initiation and member of the core 2/I N-acetylglucosaminyltransferase family. J Biol Chem. 277:21207–21212. doi: 10.1074/jbc.M201634200
- Wilson I. 2004. The never-ending story of peptide O-xylosyltransferase. Cell Mol Life Sci. 61:794–809. doi: 10.1007/s00018-003-3278-2
- Yan F , Lu S , Jiao Y , Deng Y , Du X , Huang R , Wang Q , Chen W. 2014. Molecular characterization of the BMP7 gene and its potential role in shell formation in Pinctada martensii . Int J Mol Sci. 15:21215–21228. doi: 10.3390/ijms151121215
- Young SD , Crenshaw MA , King DB. 1977. Mantle protein excretion and calcification in the hardshell clam Mercenaria mercenaria . II. Protein Synthesis Excretion Isolated Mantle Mar Biol. 41:259–262.
- Zheng Z , Du X , Xiong X , Jiao Y , Deng Y , Wang Q , Huang R. 2017. Pmrunt regulated by Pm-miR-183 participates in nacre formation possibly through promoting the expression of collagen VI-like and Nacrein in pearl oyster Pinctada martensii . Plos One. 12(6):e0178561. doi: 10.1371/journal.pone.0178561
- Zheng Z , Hao R , Xiong X , Jiao Y , Deng Y , Du X. 2019. Developmental characteristics of pearl oyster Pinctada fucata martensii: insight into key molecular events related to shell formation, settlement and metamorphosis. BMC Genomics. 20:122. doi: 10.1186/s12864-019-5505-8