ABSTRACT
The aim of experiment was to investigate the effect of feeding high level of zinc on cow production, and health and immunity of calves. The cows in each period of pre and post calving were allocated to two treatments containing 75 and 150 mg/kg Zn, respectively, as control, and containing 110 and 250 mg/kg, respectively, as second treatment. On days −25, −5, 7 and 21 relative to parturition, blood samples were obtained from 24 cows per each treatment. High level of zinc in the diet increased serum zinc, leptin and insulin in both periods but serum glucose was constant. Serum non-esterified fatty acids (NEFA) was increased by high Zn in diet, but beta hydroxybutyrate (BHBA) decreased on day 7 of lactation. In compared to the control, milk Zn on days 1 and 7 of lactation had a significant increase. High level of Zn increased colostrum IgG, calves serum IgG and total protein. Obese cows had more serum leptin and NEFA but BHBA was higher only on day 7 of lactation. In conclusion, increased serum Zn increases leptin and insulin before and after calving. It seems that their cumulative effect in spite of an increase in NEFA had a beneficial effect on milk production and calves immunity.
Introduction
Large regions of zinc (Zn)-deficient soils exist in many countries (McDowell Citation2003; Alloway Citation2008). NRC in last revised edition increased the recommended level of zinc in lactating dairy cow diets (NRC Citation1989, Citation2001). Improved dairy cow performance has been observed in cattle fed with supplemental zinc in excess of NRC (Citation2001) recommendations (Nayeri et al. Citation2014).
Leptin secretion is influenced by body fat and decreases appetite (Friedman and Halaas Citation1998), whereas zinc increases appetite (Brody Citation1999; McDowell Citation2003). However, Mangian et al. (Citation1998) and Chen et al. (Citation2000) reported that zinc supplementation increases plasma leptin level. Furthermore, zinc is essential for the synthesis and functioning of insulin and zinc deficiency decreases the amount and function of insulin (Roth and Kirchgessner Citation1981; McDowell Citation2003). Leptin is greatly influenced by insulin, and increased insulin increases the leptin (Mangian et al. Citation1998; Block et al. Citation2003; Niswender and Schwartz Citation2003). In the pre-parturition period, plasma leptin level decreases and continues till postcalving (Block et al. Citation2001; Leury et al. Citation2003). This reduction is combined with insulin resistance (Stanley Citation2005; Chalmeh et al. Citation2015). Due to the high concentration of zinc in colostrum (Kinkaid and Cronrath Citation1992; Kume et al. Citation1998), with the onset of udder activity for the synthesis of colostrum, blood zinc level is affected (such as calcium) which makes interpretation of the relationship among zinc, leptin and insulin more complicated. In this research, we investigated the effect of zinc supplementation in pre- and post-calving period on changes in zinc, leptin, and insulin, and according to the role of this three item on energy metabolism and immunity, plasma NEFA, BHBA and transfer of IgG to calves and their immunity were investigated.
Materials and methods
Animals, treatments and sampling
The present study was conducted from 23 July 2016 to 21 September 2016, at the Dam-e Asil farm located in west of Tehran-Iran. Seventy-one multiparous Holstein cows were assigned to one of two dietary treatments beginning from 25 ± 4 d before expected calving date. Cows were housed in group for each treatment on open shed wheat straw-bedded pack during the prepartum and postpartum phase of the experiment. Cows that were sick (n = 1), carrying twins (n = 2), had unfunctional mammary gland quarters (n = 4), had dystocia (n = 2), early parturition (n = 2), abortion (n = 1), lameness (n = 1), problems of sampling (n = 6) and loss of samples (n = 4) were excluded from the experiment. Only 48 cows were used for sampling and thirty-six calves of them were used for total protein (Atkinson et al. Citation2017) and IgG analysis and scoring health aspects.
Following dry-off, cows were fed with a total mixed ration (TMR) diet ad libitum. Formulated diets are shown in . Treatments were including two levels of zinc supplement in the transitional period. The cows in each period of pre and post calving were allocated to two treatments of Zn containing 75 and 150 mg/kg Zn, respectively, as control (CZn), and 110 and 250 mg/kg, respectively, as second treatment (HZn). With the exception of zinc level, all other dietary ingredients were the same within the prepartum and postpartum TMR across all treatments. Cows were fed in group ad libitum for each treatment. Feed was delivered twice daily to lactating cows (0700 and 1500 h) and once daily to prepartum cows (0900 h). Orts were measured, recorded, and discarded daily, before the morning feed delivery and amounts fed were adjusted to ensure 5% feed refusal. During the fresh period, cows were also fed a daily allowance of long-stem alfalfa (1.5 kg containing 14.4% CP in DM). Diets were formulated assuming that feedstuffs did not contribute Zn, Mn, Cu, and Co.
Table 1. Nutrient composition of prepartum and postpartum diets.
Cows body condition were scored (Wildman et al. Citation1982) by two trained individuals (scores averaged) on the first day and repeated on calving day and at 21 days of postpartum. Cows with BCS > 4 were considered as fat and cows with BCS < 3 were considered as thin. At least eight cows were in each group of fat and thin. This was used to compare the effect of BCS on hormones and metabolites. It is clear that also eight cows had 3 ≤ BCS ≤ 4 in each treatment.
Immediately postcalving, each calf was weighed and removed from the dam to prevent nursing, and cow was moved to a maternity barn, milked, and then a colostrum sample was collected. Each calf was then placed in an individual pen, measuring 0.8 × 1.2 m for 30 days. The colostrum fed to each calf after birth was obtained from the first milking postcalving of its own dam. Ingestion of colostrum was repeated 2 h later. Colostrum samples were frozen at −20°C and retained until all samples had been collected.
After first milking, cows were moved into a preparation pen in the lactation barn at least for four milking (twice daily). Twenty-four hours after birth, the blood sample was taken from the jugular vein of each calf. Samples were centrifuged (2000 × g for 10 min) and used for determination of IgG and total protein. California mastitis test was used for all cows and only after passing this test, cows were moved into a fresh barn and milked three times daily at 0700, 1500 and 2200 h for the remainder of the experiment. Weekly samples were obtained for milk composition analysis (24 cows per treatments) beginning at seven and ending at 21 days in milk (DIM) at the afternoon milking. Two samples were obtained from each cow, one of the samples was immediately sent for analysis and the other was frozen at −20°C. Blood samples were obtained from 24 cows for each treatment on d −25, −5, 7 and 21 DIM based on calving date. Blood samples of these same 48 cows were taken in each day of sampling. Blood samples were collected via coccygeal vein into vacutainers within 1 h after the morning feeding. Samples were kept on ice until centrifuged at 2000 × g for 10 min, and plasma was split into four aliquots and frozen at −20°C until analysis. We did not observe clinical problems in any of the groups.
Zinc quantified using air-acetylene flame atomic absorption spectrophotometry (PYE UNICAN, Spg, UK). Plasma leptin and insulin determined by ELISA using commercially available kits, GmbH, pharmaceuticals, Germany, with inter- and intraassay coefficients of variation of 5.4, 6.8 and 2.57, 2.19%, respectively. Plasma glucose, BHBA and NEFA concentrations were determined enzymatically using commercially available kits [glucose (GOD-PAP, Pishtaz teb, Iran), NEFA (NEFA assay, Randox, England), BHBA (Ranbut, Randox, England)] using Autoanalyser BT 1200. The inter- and intraassay coefficients of variation for the glucose, NEFA and BHBA assay were 1.35, 1.35 and 4.51, 4.32 and 4.71, 4.44%, respectively. Milk SCC analyzed by Ekomilk scan, EON Inc. Bulgaria. Milk compositions were determined by ultrasonic milk analyzer (Lactoscan, milktronic Ltd., Bulgaria). Plasma IgG concentration determined by auto analyzer (abbot-alcion, USA) and Pars Azmoon kits. Total protein samples tested by zinc sulfate turbidity test using procedure described by McEwan et al. (Citation1970) with the modification that the concentration of the zinc sulfate solution used was 250 mg/L rather than 208 mg/L, as employed by Hudgens et al. (Citation1996).
Collection of calf health data
Individual calf health scores were assigned twice weekly by a single trained person. Calves were scored from birth to 31 days old. Health scores were assigned by using a calf health-scoring system developed by the School of Veterinary Medicine, University of Wisconsin–Madison (http://www.vetmed.wisc.edu/dms/fapm/fapmtools/8calf/calf_health_scoring_chart.pdf). Calves were scored on four different aspects of health: nasal, eye and ear, fecal, and cough. A score from zero to three was given to each health aspect, zero representing normal and three representing the most severely affected.
An on-farm expert daily examined all calves. Assessment was made of general demeanor, respiratory rate, and fecal consistency. Calves were examined in order to determine the presence of ocular and nasal discharge and cough. Any calf that was exhibiting any abnormal clinical sign was subjected to a complete clinical examination that included determination of temperature and auscultation of the lungs. Ill calves received the appropriate care and treatment.
Statistical analysis
To analyze milk yield, all data were conducted to weekly means (4 days record in a week) before analysis and milk composition values were assigned to this means. Energy corrected milk was calculated as described by Hutjens (Citation2010).
Milk yield, energy corrected milk (ECM), milk components and blood serum hormones and metabolites were analyzed by repeated measures, using PROC MIXED of SAS (SAS Version 9.1, SAS Institute, Inc., Cary, NC). Mean separations were analyzed with the PDIFF statement in SAS. Serum IgG, total protein, and milk IgG were analyzed by PROC ANOVA (SAS Version 9.1). Correlation was analyzed by PROC CORR (SAS Version 9.1) and for testing normality, PROC VAR (SAS Version 9.1) was used.
Individually for each health aspect, the probability of a calf having a higher welfare score (i.e. worse score) within the study period was modeled by ordinal regression in PROC GENMOD (SAS Version 9.1) applying a cumulative logit link function and a multinomial distribution (Conneely et al. Citation2014).
Due to normalizing of data for SCC, Log10 of SCC was used.
Results
Hormones and metabolites
The amount of serum Zn was similar between treatments at the beginning of the trial ( and ). However, higher level of zinc in the diet increased blood serum zinc before and after parturition (). Zinc was increased in the serum under the influence of HZn five days precalving and 7 and 21 days postcalving ( and ), respectively 19.4, 18.36 and 15.36% (P = 0.01, P = 0.04 and P = 0.04, respectively). Blood serum zinc was significantly lower in obese cows in contrast to thin cows (P < 0.05) and it was alike in all periods of sampling even at the first day of research before zinc supplementation.
Figure 1. Means of milk yield of cows supplemented by 75 and 110 mg/kg zinc before and after calving, respectively, as CZn and 150 and 250 mg/kg zinc before and after calving, respectively, as HZn for 21 days.
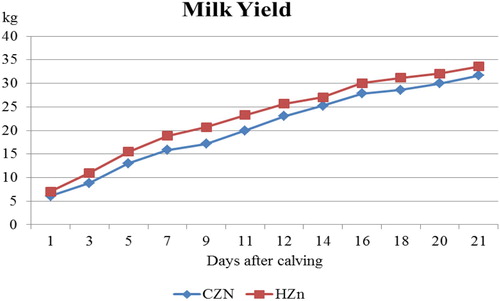
Table 2. Least square means of blood serum hormones and metabolites of cows fed two levels of zinc.
No difference was observed on day −25 for concentration of serum leptin level ( and ). However increased level of zinc in the diet, increased leptin level in the serum compared between treatments on days −5 and 7 relative to parturition (P = 0.04, P = 0.04, respectively). BCS considerably affected leptin level and in all comparisons, obese cows had higher leptin in contrast to low BCS cows (P = 0.0001). HZn significantly increased NEFA ( and ), 5 days before and 7 days after calving (P = 0.04 and P = 0.05 respectively).
HZn increased insulin ( and ). Increase in insulin was significant compared between treatments only on day 21 of lactation (P = 0.03) and on days −5 and 7, despite its increase under the effect of HZn, this was not significant (P = 0.06). The effect of BCS caused to increase serum insulin level on days −5 and 7 compared between treatments and obese cows significantly had higher insulin (P < 0.05).
HZn treatment increased serum glucose only on the 5th day of preparturition (P = 0.02) and no difference was observed on the other days. In addition, BCS had no effect on serum glucose.
At the beginning of the trial, NEFA and BHBA had no difference between treatments (), but HZn resulted in increased NEFA before and after parturition. On days −5, 7 and 21 relative to parturition, NEFA concentrations compared between treatment groups was significantly different (P < 0.05). On day 7 of lactation, BHBA decreased by high level of zinc supplementation compared to control (P = 0.0008). NEFA was influenced by BCS On days −5, 7 and 21, reduction in serum NEFA were observed in thin cows (P < 0.01). The effect of low BCS on the reduction of BHBA was significant only on day 7 of lactation (P = 0.001 and P = 0.04, respectively, for CZn and HZn).
Milk yield and composition
More zinc in the diet increased milk yield (). In the first 3 weeks of lactation, more milk (19.19, 13.18 and 7.41%) was produced by HZn treatment (P = 0.04, P = 0.009 and P = 0.04, respectively) (). Similar trend was observed for ECM and high level of zinc increased ECM production by 22.83, 16.63 and 9.26%, respectively in the first 3 weeks after parturition (P = 0.03, P = 0.002 and P = 0.02, respectively). BCS had no effect on milk production.
Figure 2. The effect of 75 and 110 mg/kg zinc supplementation before and after calving, respectively, as CZn and 150 and 250 mg/kg zinc before and after calving, respectively, as HZn on days −25, −5, 7, and 21 relative to parturition, on serum leptin (a), insulin (b), glucose (c), Zn (d), NEFA (e), and BHBA (f) of Holstein cows.
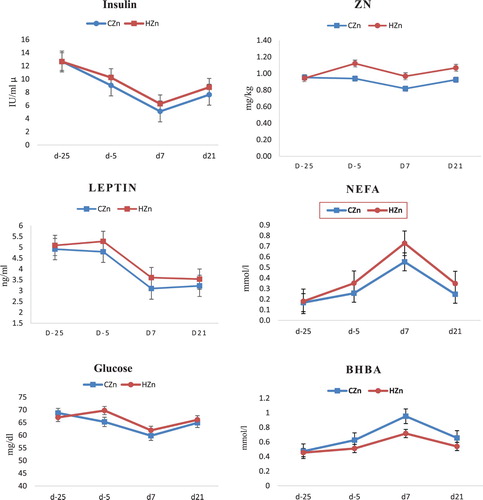
Table 3. Least square means of milk yield, milk composition and BCS of cows fed two levels of zinc.
HZn treatment mildly increased milk lactose but it was not significant (). BCS had significantly increased lactose only on day 7 and only on CZn. Neither milk fat nor milk protein was affected by high level of zinc in diet or by BCS.
Increase in milk Zn was observed compared between treatments on calving day and the 7th day of lactation (P < 0.001 and P < 0.05 respectively). Increase in zinc was not significant on days 14 and 21 of lactation. BCS had a significant effect on milk Zn. In CZn treatment on days 1 and 7 (P < 0.05), and in HZn treatment on days 1, 7 and 14 (P < 0.02), obese cows had lower milk Zn.
Calves IgG and immunity
Serum IgG and total protein had difference (P < 0.05) between the groups (). Calves serum mean IgG were 17.93 and 19.57 mg/ml and total protein means were 6.94 and 7.80, respectively for calves, which their dams fed the control and HZn treatments. High zinc in the diet increased colostral IgG by 17.5% (P = 0.04) and positive correlation was observed between serum and colostrum IgG. This correlation was greater in the control treatment (0.69, P < 0.05) and decrease by an increased amount of zinc (0.59, P = 0.07). Calves health score were not significant for any aspects of health. A number of 98, 95, 94, 94 and 99% of scores were zero (i.e. health score) for nasal, eye and ear, cough, fecal and temperature, respectively. Only one observation in CZn group and two observation in HZn group had severely abnormal score (score 3) which was in fecal aspect of health. We had no mortality during the study in both treatments.
Table 4. Mean concentration of serum IgG and serum total protein of calves and colostrum IgG fed to calves.
SCC
Somatic cell count was affected by dietary high amount of zinc (P = 0.001). However, its effectiveness was reduced by time. On the first and second week, SCC decreased (P = 0.02) in HZn treatment, but on the third week, reduction in SCC was not significant compared between treatments (). The effect of BCS was not significant on milk SCC.
Body condition score did not changed by HZn. However, BCS decreased after parturition irrespective of treatment.
Discussion
Hormones and metabolites
As our data represents, serum zinc concentration is markedly influenced by consumption level of zinc in the diet. Decreasing dietary level reduces plasma level and vice versa (Roth and Kirchgessner Citation1981). However, Marreiro et al. (Citation2006) reported that supplemented dietary zinc did not change serum and erythrocyte concentration of zinc.
Martino et al. (Citation1993) reported that feeding hypocaloric diet to obese humans increases the plasma level of zinc. They described that in obese people, zinc possibly would be accumulated in fatty tissue and during treatment by low-calorie diet, fatty tissue mobilization would release this mineral and would lead to an increase in plasma, and maybe this is the reason for lower plasma zinc concentration in obese group in our research and that of Marreiro et al. (Citation2004).
Leptin and insulin
It has been reported that leptin and insulin are adiposity signals (Niswender and Schwartz Citation2003; Schwartz Citation2006). Administration of leptin or insulin into the brain decreases feed intake, and lack of either, increases it (Schwartz et al. Citation2000). Decrease in dry matter intake precalving and two months postcalving may cause regression of pancreas and reduction in plasma insulin level (Hayirli Citation2006). Leptin decreases in the periparturient period and continues after parturition (Block et al. Citation2001; Leury et al. Citation2003) and in all probability, the low energy available for periparturient cows causes a sustained decrease in plasma leptin and may relate to the contrasting priorities of pregnancy and lactation (Block et al. Citation2001). The decrease also occurs as changes in profile of plasma insulin (Block et al. Citation2001). Insulin could increase plasma leptin but has little effect in early lactation (Leury et al. Citation2003; Niswender and Schwartz Citation2003). It is proposed that during negative energy balance in early lactation in cows, a decrease in plasma insulin could be a mediator of decrease in plasma leptin concentration (Mangian et al. Citation1998; Stanley Citation2005). Increasing appetite is a result of the decrease in the secretion of these hormones and probability this is a way to regulate dairy cows energy balance (Block et al. Citation2003; Leury et al. Citation2003).
Leptin levels are regulated by many factors, viz. Insulin, Glucocorticoids, Testosterone, Beta-adrenergic agonists, Thiazolidinediones, Thyroid hormone, Glucosamine, Glucose, Lipids (Ahima and Flier Citation2000), central nervous system (Hardie et al. Citation1996; Carulli et al. Citation1999 ) IL-2, and TNF-α (Mantzoros et al. Citation1998). However, Mantzoros et al. (Citation1998) for the first time reported that leptin concentration is altered by zinc deficiency. Supplemental Zn increases IL-2 and TNF-α which would be the reason for the increase in leptin concentration (Mantzoros et al. Citation1998).
The pancreas is very sensitive to Zn metabolism and losses Zn as soon as dietary level of zinc decreases (Roth and Kirchgessner Citation1981). Zinc is more necessary in β than α cells for the production, storage and release of insulin (Baltaci and Mogulkoc Citation2012). Zinc is accompanied with insulin in the pancreas and pancreatic concentrations are severely reduced by dietary deficiency. Each insulin molecule contains two to four atoms of Zn (McDowell Citation2003). Serum insulin level severely decreased with low zinc diet and could be again increase by sufficiently high zinc supplementation (Roth and Kirchgessner Citation1981). Different mechanisms have been proposed for the effect of zinc on insulin activity, including the role of zinc in the modulation of insulin receptor, tyrosine kinase activity. Zinc has been shown to enhance tyrosine kinase phosphorylation more than other cations (Konukoglu et al. Citation2004). Zinc deficiency increases insulin degradation and possibly insulin resistance of peripheral tissues increases in zinc-deficient cases (Roth and Kirchgessner Citation1981).
Lynch et al. (Citation2001) suggest that zinc acts at many steps in amino acid and insulin cell-signaling pathways, including mechanistic target of rapamycin (mTOR). The additive effects of zinc on these steps may increase insulin and nutritional signaling. Roth and Kirchgessner (Citation1981) in their review noted that after glucose stimulation, zinc-deficient rats had unaltered pro-insulin contents, and they showed a reduced glucose tolerance, lowered serum insulin content, and a high total insulin-like activity. Animals with low Zn in their diet showed reduction in serum Zn, and by glucose administration, had no change, whereas it increased in fair fed group.
It is possible that increased serum leptin and insulin in our research is dependent on increased Zn level in serum. Zinc increases the activity of insulin signaling pathway. In vivo zinc deficiency causes not only a decrease in insulin secretion but also decreases cellular reaction to insulin. Therefore, zinc deficiency may decrease the ob gene expression, which is stimulated by insulin (Baltaci and Mogulkoc Citation2012). In the study performed by Delavaud et al. (Citation2002), insulin had no effect on plasma leptin and they claimed that glucose might be involved in the regulation of leptin released by adipose tissue. However, based on our data, glucose is similar in both treatments and in all probability, insulin, Zn, or both are the main factors. Mangian et al. (Citation1998) reported lower blood concentrations of leptin in Zn deficient rats, which suggest that leptin is down regulated during zinc deficiency, thus providing a regulatory signal for increased food intake.
Block et al. (Citation2003) established that adiposity modulates the ability of insulin to increase plasma leptin. Leptin and insulin circulate in proportion to the body’s fat content (Schwartz et al. Citation2000) and this is in agreement with our data, which shows that obese cows had higher serum insulin, and leptin compared to low BCS cows. Positive correlation was found between insulin and leptin in obese cows (0.59, P = 0.0001). Also, BCS was positively correlated with leptin levels (Ehrhardt et al. Citation2000; Delavaud et al. Citation2002) and confirms that this is true in well-fed cows but not in underfed cows (Delavaud et al. Citation2002). Garcia et al. (Citation2012) reported that high leptin concentrations were associated with lower zinc concentrations in women with obesity. However, in our study, obese cows had lower serum zinc, whereas in obese cows, positive correlation between serum Zn and leptin was significant (0.41, P = 0.009) which is opposite to that of Chen et al. (Citation2000). In contrast to our study, the results of Chen et al. (Citation2000) are based on the aggregation of all the data and not only the obese cows.
NEFA & BHBA
In many species in late pregnancy and early lactation, insulin resistance could be developed (Hayirli Citation2006). Reduced insulin sensitivity concomitant with the decrease in its concentration alters glucose utilization in the body by making glucose less available to insulin responsive cells. The decreased sensitivity also reduces insulin control of lipolysis and NEFA mobilization (Stanley Citation2005; Hayirli Citation2006) and hyperlipidemia could occur (Allen et al. Citation2009).
In this study, despite an increased level of insulin in HZn treatment, NEFA concentration increased. One possible explanation might be the fact that high level of zinc had increased leptin in HZn in contrast to CZn. Block et al. (Citation2001) and Konigsson et al. (Citation2008) reported that leptin negatively correlated to NEFA, which is not consistent with our findings.
Many articles indicated that increased concentration of insulin (Stanley Citation2005; Hayirli Citation2006; Allen et al. Citation2009) or increasing efficacy of insulin (Smith et al. Citation2007) around parturition, reduces lipolysis and decreases blood level of NEFA or inversely by the reduction in insulin level, lipid degradation would be increased. However, in our trial, not only serum insulin had increased, but also leptin level increased simultaneously. Leptin activates lipid oxidation by inducing the expression of enzymes of lipid oxidation (Ahima & Flier, Citation2000) and the effect of leptin on lipolysis, had significantly increased NEFA Which is consistent with Ajuwon et al. (Citation2003) findings. As expected, overweight cows had higher NEFA concentrations and this occur as serum insulin concentration was higher in obese cows. However, increased leptin was more remarkable.
Decreased concentration of BHBA (significant difference was seen only on day 7 of lactation) may be due to the increase in insulin. Insulin in the liver stimulates lipogenesis and inhibits ketogenesis (Hayirli Citation2006). Insulin increases peripheral tissue ketone utilization, and alters enzyme activities and availability of substrates involved in ketogenesis in the liver (Hayirli Citation2006). Rico et al. (Citation2015) reported no significant effect of BCS on plasma concentration of BHBA. However, in this trial, on day 7 of post calving, in spite of reduction in serum BHBA in HZn group, obese cows had significantly higher BHBA than thin cows. Obese cows mobilize more NEFA than thin cows (Sundrum Citation2015) In addition, development of fatty liver has been found to impair the gluconeogenesis in the liver tissue, which lowers blood glucose and decreases insulin secretion. This, in turn, would support greater lipid mobilization, and increased rate of fatty acid uptake by the liver and increased BHBA (Adewuyil et al. Citation2005).
BCS and feed intake
Body condition was scored with 1–5 scales based on Wildman et al. (Citation1982), and supplemented zinc had no effect on BCS and this is in agreement with Cope et al. (Citation2009), Chandra et al. (Citation2013) and Nayeri et al. (Citation2014). In addition, organic or inorganic forms of zinc does not seem to have effect on BCS (Cope et al. Citation2009; Nayeri et al. Citation2014). Increased amount of zinc increased leptin but BCS was not influenced by leptin.
Many researchers confirmed the positive effect of zinc on appetite and feed intake (Brody Citation1999; McDowell Citation2003; Taneja et al. Citation2012). In this trial, due to the increasing amount of leptin and insulin around parturition, feed consumption was expected to reduce. This research was conducted in the herd and we were not able to measure feed consumption for each cow. However daily consumption of each treatment was measured and no difference was observed.
Several items such as mineral composition in the gut, phytate, different kinds of proteins and amino acids, EDTA and organic acids (Lonnerdal Citation2000) modulate zinc absorption. HZn treatment by stimulating growth of papilla causes an increase in VFA absorption, which could prevent accumulation of VFA and stabilize ruminal pH (Hayirli Citation2006) and promotes the rates of absorption of nutrients and stomach evacuation process. Nayeri et al. (Citation2014) reported that supplementation of 75 mg/kg zinc-methionine (ZnMet) decreased feed intake in contrast to 75 mg/kg zinc sulfide (ZnS) during both the prepartum and postpartum periods. They compared two forms of zinc and they had no control treatment. May be, if they had had control treatment, they might have observed less feed intake. Zinc deficiency in rat decreased leptin concentration but simultaneous decrease in feed intake was observed (Mangian et al. Citation1998). It seems that feed intake depends more on zinc concentration than plasma leptin level.
Milk yield and composition
Dietary supplementation of high level of zinc could increase milk yield. Kellogg et al. (Citation2004) in his review mentioned that increasing ZnMet improves humoral immunity and udder health and increases lactation performance. He also described the synthesis of more keratin from teat canals by cows that consumed ZnMet, which could help the udder health between milking. Kellogg (Citation2004), Cope et al. (Citation2009) and Nayeri et al. (Citation2014) stated that improved claw health increased cow health and welfare. In addition, increased gastrointestinal integrity and improved intestinal villi could be responsible for increased milk performance (Vallee and Falchuk Citation1993; Nayeri et al. Citation2014). By supplementation of ZnMet, milk production response was independent of the level of production as milk production increased even in high producing herds (Kellogg, Citation2004). However, Wiking et al. (Citation2008) reported no difference in milk yield or energy corrected milk by feeding with 80 mg/kg Zn (as ZnO).
Moreover, concerning an increase in insulin in this research, increased milk production to some extent could be attributed to insulin. Hayirli (Citation2006) reported a quadratic increase in milk yield of early lactating cows (day 5) injected with a single dose of slow release insulin. Milk yield responses to insulin are also inconsistent in the literature (Hayirli Citation2006).
HZn did not significantly affect milk composition with respect to percentage of fat, protein and lactose and it is in agreement with Kellogg (Citation2004) and Wiking et al. (Citation2008) who studied on ZnMet and ZnO, respectively.
Zinc is the trace element in milk and is present in large concentration and literature reports average values from 3.5 to 4 mg/kg (Anderson Citation1992). The transport of zinc from maternal blood to milk seems not to be perfect. Low level of Zn in breast milk is a result of defect in Zn transportation from the blood to milk (Vallee and Falchuk Citation1993).
Zn concentration in the feed cannot directly affect the milk level of Zn (Pechova et al. Citation2006; Cope et al. Citation2009; Wiking et al. Citation2008). Supplementation of zinc and saturated fat to diet significantly increased plasma Zn and milk Zn concentration. In all probability, transfer of fat to milk is a facilitator of transferring Zn to milk (Wiking et al. Citation2008).
Increased milk Zn compared between treatments was significant in our samples on days 1 and 7 of lactation. In our previous research (unpublished data) on high producing cows (mean = 43 ± 7.8 kg/d), supplemented zinc in the diet up to 300 mg/kg made no significant difference. Cope et al. (Citation2009) by supplementation of 370 mg/kg organic zinc also reported similar result. May be due to high milk production, this amount of zinc was not sufficient to increase milk Zn. Inspite of increase in milk Zn on days 1 and 7 of lactation, by increasing milk yield as days of lactation increases, milk Zn was not affected by HZn.
Calves IgG and immunity
Deficiency of no other element causes serious impairment as that of zinc and the deficiency of zinc is considered among the major causes of immunodeficiency (Vallee and Falchuk Citation1993). Dietary zinc has effects on CD+ 4 cells, T helper cells functions, and consequently, cell-mediated immunity (Friedman and Halaas Citation1998; Baltaci and Mogulkoc Citation2012) and increased production of IL-2, and TNF-α, (Mantzoros et al. Citation1998; Baltaci and Mogulkoc Citation2012). These cytokines could be responsible for the synthesis and increased concentration of serum leptin (Mantzoros et al. Citation1998; Ahima and Flier Citation2000).
Leptin stimulates inflammatory response, T-lymphocyte proliferation, and Th1 cytokine production, demonstrating that leptin is an important link between nutrition and immunity. Leptin deficient mice (ob/ob mice) suffered immune abnormalities. The decrease in leptin weakens the immune system (Friedman and Halaas Citation1998; Ahima and Flier Citation2000).
Concerning similarity between the structure of leptin and leptin receptors with cytokines, leptin could also be considered as a cytokine and has a significant role in immune response (Ahima and Flier Citation2000; Baltaci and Mogulkoc Citation2012).
Nayeri et al. (Citation2014) reported 20% increase in colostrum IgG concentrations by supplementation of 75 mg/kg ZnMet in contrast to ZnS in multiparous cows. However, Kinkaid et al. (Citation1997) claimed that Zn had no effect on mitogen-induced lymphocyte blastogenesis, interleukin-2 production, lymphocyte cytotoxicity, or phagocytic and intracellular killing ability of blood neutrophils in calves fed with Zn as ZnO or ZnMet or ZnLys.
Nayeri et al. (Citation2014) described that zinc plays an important role in the synthesis of colostrum IgG and its transport to newborn calves. In our research, by increasing Zn level in the diet, colostrum and serum IgG and serum total protein significantly increased. There was a positive correlation between colostral and serum IgG in the control treatment (0.69, P = 0.02) but by increasing the level of dietary Zn, this correlation value decreased (0.59, P = 0.07). Excess amount of zinc in HZn treatment can increase colostrum and serum IgG but the absorption of IgG does not increased correspondingly (amount of IgG in serum increased but the rate of absorption decreased).
In relation to increased serum IgG of HZn calves, we expected to see differences in scores of health aspects, as Conneely et al. (Citation2014) describes that serum IgG at 24 h of age could be effective on heath scores and attributed insignificant differences in health scores to equal amount of serum IgG of calves (Conneely et al. Citation2014). Insignificancy of different aspects of calves’ health scores could be attributed to the hygienic environment and enough surveillance of workers. Not only calves of our study, but also all calves of farm were in good and healthy condition and by the primary signs of illness, farms veterinarian visits them. This is confirmed by high percent of zero score (96.3%) and low percent of score three (0.15%). In all probability, this is the reason for the insignificancy between treatments.
SCC
Due to the important role of Zn in cell division and protein synthesis, it has become necessary for health and epithelial tissue integrity (McDowell Citation2003). Zinc deficiency reduces humoral and cellular immune responses. Decreased immunity in Zn deficient subjects is attributed to reduced cell-mediated immune response and natural killer cell activity, spleen and thymus atrophy, and decreased T-dependent and independent anti-body-mediated responses (Kellogg, Citation2004).
Somatic cell count is dependent on the immune system (Kellogg, Citation2004) and physically healthy udder (Capuco et al. Citation1992). The effect of zinc supplementation on the reduction of SCC has been studied in several papers (Pechova et al. Citation2006; Cope et al. Citation2009; Nayeri et al. Citation2014) and all of them emphasize the effect of immunity on SCC. Supplemented zinc also decreased udder edema in heifers (Campbell and Miller Citation1998). However, by considering the effect of leptin on the immune system (mentioned earlier), there is no enough study regarding the role of leptin on SCC. In our study, by increasing the amount of zinc, increase in cows leptin level and calves IgG and decrease in SCC were observed which are strongly dependent on the immune system. May be reduction in SCC contributed to increased leptin level.
Acknowledgments
The authors thank the Agricultural Sciences and Natural Resources University of Khuzestan. We would like to thank the farm staff at Dam-e Asil in Tehran for their assistance during the study.
Disclosure statement
No potential conflict of interest was reported by the authors.
ORCID
Morteza Chaji http://orcid.org/0000-0002-9336-4094
References
- Adewuyil AA , Gruysi E , van Eerdenburg FJCM. 2005. Non esterified fatty acids (NEFA) in dairy cattle. Vet Q. 27:117–126. doi:10.1080/01652176.2005.9695192.
- Ahima RS , Flier JS. 2000. Leptin. Annu Rev Physiol. 62:413–437. doi: 10.1146/annurev.physiol.62.1.413
- Ajuwon KM , Kuske JL , Anderson DB , Hancock DL , Houseknecht KL , Adeola O , Spurlock ME. 2003. Chronic leptin administration increases serum NEFA in the pig and differentially regulates PPAR expression in adipose tissue. J Nutr Biochem. 14:576–583. doi:10.1016/S0955-2863(03)00104-9.
- Allen MS , Bradford BJ , Oba M. 2009. Board-invited review: The hepatic oxidation theory of the control of feed intake and its application to ruminants. J Anim Sci. 87:3317–3334. doi:10.2527/jas.2009-1779.
- Alloway BJ. 2008. Zinc in soils and crop nutrition. 2nd ed. Brussels and Paris : IZA and IFA.
- Anderson R. 1992. Comparison of trace elements in milk of four species. J Dairy Sci. 75:3050–3055. doi: 10.3168/jds.S0022-0302(92)78068-0
- Atkinson DJ , von Keyserlingk MAG , Weary DM. 2017. Benchmarking passive transfer of immunity and growth in dairy calves. J Dairy Sci. 100:3773–3782. doi:10.3168/jds.2016-11800.
- Baltaci AK , Mogulkoc R. 2012. Leptin and zinc relation: In regulation of food intake and immunity. Indian J Endocrinol Metab. 16:611–616. doi:10.4103/2230-8210.105579.
- Block SS , Butler WR , Ehrhardt RA , Bell AW , Van Amburgh ME , Boisclair YR. 2001. Decreased concentration of plasma leptin in periparturient dairy cows is caused by negative energy balance. J Endocrinol. 171:339–348. doi: 10.1677/joe.0.1710339
- Block SS , Rhoads RP , Bauman DE , Ehrhardt RA , McGuire MA , Crooker BA , Griinari JM , Mackle TR , Weber WJ , Van Amburgh ME , Boisclair YR. 2003. Demonstration of a role for insulin in the regulation of leptin in lactating dairy cows. J Dairy Sci. 86:3508–3515. doi: 10.3168/jds.S0022-0302(03)73955-1
- Brody T. 1999. Nutritional biochemistry. 2nd ed. San Diego : Academic Press.
- Campbell MH , Miller JK. 1998. Effect of supplemental dietary vitamin E and zinc on reproductive performance of dairy cows and heifers fed excess iron. J Dairy Sci. 81:2693–2699. doi: 10.3168/jds.S0022-0302(98)75826-6
- Capuco AV , Bright SA , Pankey JW , Wood DL , Miller RH , Britman J. 1992. Increased susceptibility to intramammary infection following removal of teat canal keratin. J Dairy Sci. 75:2126–2130. doi: 10.3168/jds.S0022-0302(92)77972-7
- Carulli L. , Ferrari S. , Bertolini M. , Tagliafico E. 1999. Regulation of ob gene expression: evidence for epinephrine induced suppression in human obesity. Journal of Clinical Endocrinology & Metabolism. 84:3309–3312. doi: 10.1210/jcem.84.9.6007
- Chalmeh A , Pourjafar M , Nazifi S , Momenifar F , Mohamadi M. 2015. Insulin resistance in different physiological states of high producing Holstein dairy cows. Acta Scientiae Veterinariae. 43:1255–1261.
- Chandra G , Aggarwal A , Singh AK , Kumar M , Upadhyay RC. 2013. Effect of vitamin E and zinc supplementation on energy metabolites, lipid peroxidation, and milk production in peripartum Sahiwal cows. Asian Australas J Anim Sci. 26:1569–1576. doi:10.5713/ajas.2012.12682.
- Chen MD , Song YM , Lin PY. 2000. Zinc may be a mediator of leptin production in humans. Life Sci. 66:2143–2149. doi:10.4049/jimmunol.1103579 doi: 10.1016/S0024-3205(00)00541-5
- Conneely M , Berry DP , Sayers R , Murphy JP , Doherty ML , Lorenz I , Kennedy E. 2014. Does iodine supplementation of the prepartum dairy cow diet affect serum immunoglobulin G concentration, iodine, and health status of the calf? J Dairy Sci. 97:5120–5130. doi:10.3168/jds.2013-7867.
- Cope CM , Mackenzie AM , Wilde D , Sinclair LA. 2009. Effects of level and form of dietary zinc on dairy cow performance and health. J Dairy Sci. 92:2128–2135. doi: 10.3168/jds.2008-1232
- Delavaud C , Ferlay A , Faulconnier Y , Bocquier F , Kann G , Chilliard Y. 2002. Plasma leptin concentration in adult cattle: effects of breed, adiposity, feeding level, and meal intake. J Anim Sci. 80:1317–1328. doi: 10.2527/2002.8051317x
- Ehrhardt RA , Slepetis RM , Siegal-Willott J , Van Amburgh ME , Bell AW , Boisclair YR. 2000. Development of a specific radioimmunoassay to measure physiological changes of circulating leptin in cattle and sheep. J Endocrinol. 166:519–528. doi: 10.1677/joe.0.1660519
- Friedman JM , Halaas JL. 1998. Leptin and the regulation of body weight in mammals. Nature. 395:763–770. doi: 10.1038/27376
- Garcia OP , Ronquillo D , Caamao MC , Camacho M , Long KZ , Rosado JL. 2012. Zinc, vitamin A, and vitamin C status are associated with leptin concentrations and obesity in Mexican women: results from a cross-sectional study. Nutr Metab. 9:59–67. doi:10.1186/1743-7075-9-59.
- Hardie LJ , Guilhot N , Trayhurn P. 1996. Regulation of leptin production in cultured mature white adipocytes. Hormone Metab Res J. 28:685–689. doi: 10.1055/s-2007-979878
- Hayirli A. 2006. The role of exogenous insulin in the complex of hepatic lipidosis and ketosis associated with insulin resistance phenomenon in postpartum dairy cattle. Vet Res Commun. 30:749–774. doi:10.1007/s11259-006-3320-6.
- Hudgens KA , Tyler JW , Besser TE , Krytenberg DS. 1996. Optimizing performance of a qualitative zinc sulfate turbidity test for passive transfer of immunoglobulin G in calves. Am J Vet Res. 57:1711–1713.
- Hutjens MF. 2010. Benchmarking your feed efficiency, feed costs, and income over feed cost. Western Can Dairy Semin Adv Dairy Technol. 22:3–10.
- Kellogg DW , Tomlinson DJ , Socha MT , Johnson AB. 2004. Review: effects of zinc methionine complex on milk production and somatic cell count of dairy cows: Twelve-trial summary. Profess Anim Sci. 20:295–301. doi: 10.15232/S1080-7446(15)31318-8
- Kinkaid RL , Chew BP , Cronrath JD. 1997. Zinc oxide and amino acids as sources of dietary zinc for calves: effects on uptake and immunity. J Dairy Sci. 80:1381–1388. doi: 10.3168/jds.S0022-0302(97)76067-3
- Kinkaid RL , Cronrath JD. 1992. Zinc concentration and distribution in mammary secretions of peripartum cows. J Dairy Sci. 75:481–484. doi: 10.3168/jds.S0022-0302(92)77784-4
- Konigsson K , Savoini G , Govoni N , Invernizzi G , Prandi A , Kindahl H , Veronesi MC. 2008. Energy balance, leptin, NEFA and IGF-I plasma concentrations and resumption of postpartum ovarian activity in Swedish red and white breed cows. Acta Vet Scand. 50:3–10. doi:10.1186/1751-0147-50-3.
- Konukoglu D , Turhan MS , Ercan M , Serin O. 2004. Relationship between plasma leptin and zinc levels and the effect of insulin and oxidative stress on leptin levels in obese diabetic patients. J Nutr Biochem. 15:757–760. doi:10.1016/j.jnutbio.2004.07.007.
- Kume S , Yamamoto E , Kudo T , Toharmat T , Nonaka J. 1998. Effect of parity on mineral concentration in milk and plasma of Holstein cows during early lactation. Asian Australas J Anim Sci. 11:133–138. doi: 10.5713/ajas.1998.133
- Leury BL , Baumgard LH , Block SS , Segoale N , Ehrhardt RA , Rhoads RP , Bauman DE , Bell AW , Boisclair YR. 2003. Effect of insulin and growth hormone on plasma leptin in periparturient dairy cows. Am J Physiol Regulat Integrat Comparit Physiol. 285:R1107–R1115. doi: 10.1152/ajpregu.00320.2003
- Lonnerdal B. 2000. Dietary factors influencing zinc absorption. J Nutr. 130(supp.):1378S–1383S. doi: 10.1093/jn/130.5.1378S
- Lynch CJ , Patson BJ , Goodman SA , Trapolsi D , Kimball S. 2001. Zinc stimulates the activity of the insulin and nutrient-regulated protein kinase mTOR. Am J Physiol-Endocrinol Metab. 281:E25–E34. doi: 10.1152/ajpendo.2001.281.1.E25
- Mangian HF , Lee RG , Paul GL , Emmert JL , Shay NF. 1998. Zinc deficiency suppresses plasma leptin concentrations in rats. Nutr Biochem. 9:47–51. doi: 10.1016/S0955-2863(97)00165-4
- Mantzoros CS , Prasad AS , Beck FWJ , Grabowski S , Kaplan J , Adair C , Brewer GJ. 1998. Zinc may regulate serum leptin concentrations in humans. J Am College Nutr. 17:270–275. doi:10.1080/07315724.1998.10718758.
- Marreiro DN , Fisberg M , Cozzolino SM. 2004. Zinc nutritional status and its relationships with hyperinsulinemia in obese children and adolescents. Biol Trace Elem Res. 100:137–149. doi: 10.1385/BTER:100:2:137
- Marreiro DN , Geloneze B , Tambascia MA , Lerario AC , Halpern A , Cozzolino SMF. 2006. Effect of zinc supplementation on serum leptin levels and insulin resistance of obese women. Biol Trace Elem Res. 112:109–118. doi: 10.1385/BTER:112:2:109
- Martino G , Matera MG , Martino B , Vacca C , Martino S , Rossi F. 1993. Relationship between zinc and obesity. J Med Chem. 24:177–183.
- McDowell LR. 2003. Minerals in animal and human nutrition. 2nd ed. Amsterdam : Elsevier Science Press.
- McEwan AD , Fisher EW , Selman IE , Penhale JP. 1970. A turbidity test for the estimation of immune globulin levels in neonatal calf serum. Clin Chim Acta. 27:155–163. doi: 10.1016/0009-8981(70)90390-6
- National Research Council . 1989. Nutrient requirements of dairy cattle. 6th rev. ed. Washington, DC : Natl. Acad. Press.
- National Research Council . 2001. Nutrient requirements of dairy cattle. 7th rev. ed. Washington, DC : Natl. Acad. Press.
- Nayeri A , Upah NC , Sucu E , Sanz-Fernandez MV , Defrain JM , Gorden PJ , Baumgard LH. 2014. Effect of the ratio of zinc amino acid complex to zinc sulfate on the performance of Holstein cows. J Dairy Sci. 97:4392–4404. doi:10.3168/jds.2013-7541.
- Niswender KD , Schwartz MW. 2003. Insulin and leptin revisited: adiposity signals with overlapping physiological and intracellular signaling capabilities. Front Neuroendocrinol. 24:1–10. doi:10.1016/S0091-3022(02)00105-X.
- Pechova A , Pavlata L , Lokajova E. 2006. Zinc supplementation and somatic cell count in milk of dairy cows. Acta Vet Brno. 75:355–361. doi:10.2754/avb200675030355.
- Rico JE , Bandaru VVR , Dorskind JM , Haughey NJ , McFadden JW. 2015. Plasma ceramides are elevated in overweight Holstein dairy cows experiencing greater lipolysis and insulin resistance during the transition from late pregnancy to early lactation. J Dairy Sci. 98:7757–7770. doi:10.3168/jds.2015-9519.
- Roth HP , Kirchgessner M. 1981. Zinc and insulin metabolism. Biol Trace Elem Res. 3:13–32. doi: 10.1007/BF02789121
- Schwartz MW. 2006. Central nervous system regulation of food intake. Obesity. 14(Supp. Feb):1S–8S. doi: 10.1038/oby.2006.275
- Schwartz MW , Wood SC , Baskin DG. 2000. Central nervous system control of food intake [Insight review article]. Nature. 404:661–671. doi: 10.1038/35007534
- Smith KL , Stebulis SE , Waldron MR , Overton TR. 2007. Prepartum 2,4-thiazolidinedione alters metabolic dynamics and dry matter intake of dairy cows. J Dairy Sci. 90:3660–3670. doi:10.3168/jds.2006-650.
- Stanley CC. 2005. Regulation of glucose metabolism in dairy cattle [Ph.D Thesis]. Louisiana State University and Agricultural and Mechanical College.
- Sundrum A. 2015. Metabolic disorders in the transition period indicate that the dairy cows’ ability to adapt is overstressed. Animals (Basel). 5:978–1020. doi:10.3390/ani5040395.
- Taneja SK , Jain M , Mandal R , Megha K. 2012. Excessive zinc in diet induces leptin resistance in Wistar rat through increased uptake of nutrients at intestinal level. J Trace Elem Med Biol. 26:267–272. doi:10.1016/j.jtemb.2012.03.002.
- Vallee BL , Falchuk KH. 1993. The biochemical basis of zinc physiology. Physiol Rev. 73:79–118. doi: 10.1152/physrev.1993.73.1.79
- Wiking L , Larsen T , Sehested J. 2008. Transfer of dietary zinc and fat to milk evaluation of milk fat quality, milk fat precursors, and mastitis indicators. J Dairy Sci. 91:1544–1551. doi:10.3168/jds.2007-0716.
- Wildman EE , Jones GM , Wagner PE , Boman RL. 1982. A dairy cow body condition scoring system and its relationship to selected production characteristics. J Dairy Sci. 65:495–501. doi: 10.3168/jds.S0022-0302(82)82223-6