ABSTRACT
DNA methylation plays an important role in reproduction. Tibetan pigs maintain high sperm motility, even under extreme conditions at high altitudes. However, the DNA methylation mechanisms underlying testicular plateau adaptations in Tibetan pigs remain unclear. We determined the genomic DNA methylation level in the testicular tissues of Tibetan pigs living at high altitudes (TP) and of Yorkshire pigs that migrated to high altitudes (YP) using the fluorescence method. Quantitative real-time PCR and western blots were used to measure the gene and protein expression levels, respectively, of three DNA methyltransferases (DNMT1, DNMT3A, and DNMT3B) and hypoxia-inducible factor 2α (HIF2α). We found that the genomic DNA methylation level and the mRNA and protein expression levels of the four genes were all significantly lower in the testicular tissues of TP than YP. In short, compared with YP, the downregulation of DNA methyltransferases in the TP testes led to lower levels of genomic DNA methylation, which would facilitate higher sperm motility. Meanwhile, HIF2α expression in the TP testes was down-regulated, which might contributes to TP testes’ adaption to the plateau environment. This study provided new insights into the DNA methylation mechanisms underlying plateau adaptations in TP testicles.
1. Introduction
In mammals, DNA methylation predominantly occurs at the C-5 position of cytosine in CpG dinucleotides, except for short genomic regions called CpG islands (CGIs) (Mason et al. Citation2012; Suzuki and Bird Citation2008). DNA methylation plays an important role in regulating the expression of gene transcription, and methylation can also lead to long-term alterations in gene expression (Falck et al. Citation2012; Feinberg Citation2007). DNA methylation is critical to reproduction (An et al. Citation2018). DNA methylation is catalyzed by the DNA methyltransferase (DNMT) family that includes three main members, DNMT1, DNMT3a, and DNMT3b. Hypoxia-inducible factor 2α (HIF2α) is encoded by endothelial PAS domain protein 1 (EPAS1); the factor plays an important role in the HIFs pathway and is closely related to high altitude chronic hypoxia (Pan et al. Citation2018). HIF2α regulates a large and diverse subset of transcription factors and coregulators that contribute to its diverse roles in hypoxia (Li et al. Citation2019). HIF2α is required for spermatogenesis in the postnatal mouse testis (Gruber et al. Citation2010). The Tibetan pig, known as the treasure of the plateau is an important pig breed that has adapted to the harsh plateau environment (Yang et al. Citation2011). Changes in DNA methylation of pituitaries during the initiation of puberty in gilts (young female pig-aged at 160 days) have been investigated at the genome scale using reduced representation bisulfite sequencing (Yuan et al. Citation2019). Dynamics of DNA methylation and DNMT expression during gametogenesis and early development have been investigated in the scallop (Patinopecten yessoensis) using the fluorescence method, transcriptomes, and in situ hybridization (Li et al. Citation2019). Indigenous Tibetan pigs residing at high altitudes were compared with other Tibetan pigs that had migrated to neighboring low-altitude areas using comparative transcriptomics and DNA methylation analysis (Jin et al. Citation2018). Though Tibetan pigs that live in high altitudes (TP) have smaller testicles and less ejaculatory volume than Yorkshire pigs that migrated to high altitudes (YP), sperm motility of TP can reach above 0.8 (Ren et al. Citation2017), suggesting that TP testicles are well adapted to the plateau environment. However, though global DNA methylation level and the mRNA expression of DNA methyltransferase (DNMT3A) and hypoxia-inducible factor 2α (HIF2α) in the testicular tissues of TP have only been investigated in two-week-old pigs (Zhao et al. Citation2017), no similar reports are available for TP after sexual maturity.
To address this knowledge gap, we herein investigated genomic DNA methylation in the testicular tissues of TP and YP after sexual maturity using the fluorescence method. Quantitative real-time PCR and western blots were used to measure the gene and protein expression levels, respectively, of three DNA methyltransferases (DNMT1, DNMT3A, and DNMT3B) and HIF2α. Our results provide new insights into the DNA methylation mechanisms underlying the adaptation of TP testicles to the plateau, and the findings herein can serve as a basis for the improvement of TP fecundity and the establishment of molecular breeding.
2. Materials and methods
2.1. Ethics statements and animal samples
Experiments were performed using pigs from two different populations: Tibetan pigs living in the highlands (Linzhi, 3,000 m, TP) and Yorkshire pigs that migrated from lowland (Beijing, 100 m) to the highlands (Linzhi, 3,000 m, YP) about three years ago. The pigs used were raised in the Tibet Agricultural & Animal Husbandry University Farm. Ten six-month-old boars from each population were castrated. The whole testicular tissues of each pig were collected separately and immediately stored at −80°C.
2.2. Primers design and synthesis
Primers were designed across introns using Primer Premier 5.0 (Premier Biosoft, Palo Alto, CA, USA; ). The housekeeping gene β-actin was used as an internal standard. Primers were synthesized by Sangon Bioengineering (Beijing) Co., LTD (China).
Table 1. Primers used for real time PCR.
2.3. DNA extraction and global genomic methylation level detection
Genomic DNA was isolated from testicular tissues using phenol–chloroform extraction. Ten samples were then diluted to 100 ng/mL. The DNA methylation levels of each sample were determined using a MethylFlashTM Methylated DNA Quantification Kit (Fluorometric) (P-1035-96; Epigentek Group Inc., New York, USA) following the manufacturer’s instructions. In brief, standard curves were derived, DNA binding was performed, and the signal was detected using a NanoQuant infinite M200 ELISA (TECAN Inc., Mannedorf, Switzerland). Finally, the amount of DNA methylation (5 mC) was calculated.
2.4. RNA extraction, cDNA synthesis, and quantitative real-time PCR
Total RNA was extracted from the testicles using TRIZOL Reagent (Invitrogen, San Diego, CA, USA) and separated using electrophoresis in a 1% agarose gel to verify integrity. After treatment with DNase I, 1 μg of RNA in a 20 μL reaction volume was reverse transcribed into cDNA using a SuperRT cDNA Kit (D7180M; Beyotime Ltd., Shanghai, China), following the manufacturer’s instructions. We used the housekeeping gene β-actin as the internal standard. Quantitative real-time PCR (qRT-PCR) was conducted on the Bio-Rad CFX96 System (Bio-Rad, California, USA). For each sample, the target gene and the internal reference gene were amplified in the same plate. Calibrations were performed based on three replicates. The thermal cycling conditions were 95°C for 15 min, 40 cycles of PCR (95°C for 10 s, 60°C for 20 s, and 72°C for 30 s), then 95°C for 10 s. A melting curve was constructed by increasing the temperature from 65 °C to 95 °C in sequential steps of 0.5 °C for 5 s during which fluorescence was measured, and then cooling at 4°C. Each reaction mixture contained 10.0 μL 2× SYBR Green qPCR SuperMix (Transgen, Beijing, China), 1.0 μL cDNA, 0.4 μL of each primer (10.0 nmol/μL), and ddH2O water to adjust the volume to 20.0 μL. Gene expression levels were calculated using the 2-ΔΔCt method, as previously described (Livak and Schmittgen Citation2001).
2.5. Western blots
Total proteins were isolated from the testicular tissues using RIPA Lysis Buffer (P0013B, Beyotime Ltd., Shanghai, China). A few minutes before use, we added PMSF (ST506, Beyotime Ltd., Shanghai, China) to the lysate to make a final concentration of 1 mM PMSF. We added 150–250 µL lysate per 20 mg of tissue. Lysate mixtures were homogenized using a Mixer Mill MM400 (Retsch, Haan, Germany) for 3 min and then centrifuged at 12,000×g for 30 min at 4°C. The protein content in the supernatant was measured using an enhanced BCA protein assay kit (P0010S, Beyotime, Ltd., Shanghai, China). After denaturation, approximately 30 μg of the total proteins was separated using sodium dodecyl sulfate-polyacrylamide gel electrophoresis (SDS-PAGE) with a 4% stacking gel and a 10% separating gel. Following electrophoresis, proteins were transferred to polyvinylidene fluoride (PVDF) membranes (FFP39, Beyotime Ltd., Shanghai, China) for 2.5 h at 300 mA using a Bio-Rad Criterion Blotter (P0023B, Beyotime Ltd., Shanghai, China). Membranes were blocked overnight in blocking buffer (P0071, Beyotime Ltd., Shanghai, China), transversely cut at the position indicated by the marker (P0023A, Beyotime Ltd., Shanghai, China) and incubated with the appropriate primary antibody () diluted in primary antibody dilution buffer (P0023A; Beyotime Ltd., Shanghai, China), then gently shaken at room temperature for 2 h. After three washes with phosphate-buffered saline containing 0.1% Tween 20 (PBST), membranes were incubated with the appropriate secondary antibody () diluted in secondary antibody dilution buffer (P0023D; Beyotime Ltd., Shanghai, China) for 1 h. After three washes in Tris-buffered saline with Tween for 30 min, immune complexes on the membranes were visualized using a BeyoECL Plus (P0018S, A0216; Beyotime Ltd., Shanghai, China), following the manufacturer’s instructions. To determine expression levels of DNMT1, DNMT3A, DNMT3B, and HIF2α relative to ACTIN, western blots were analyzed using Image J 1.44 (NIH, Bethesda, MD, USA).
Table 2. Details of the antibodies used for western blots.
2.6. Statistical analyses
Statistical analyses were performed using IBM SPSS Statistics v17.0 (SPSS Inc. Released 2008. SPSS Statistics for Windows, Version 17.0. Chicago: SPSS Inc.). Graphs were prepared using SigmaPlot 10.0 (Systat Software, San Jose, CA, USA). One-way analysis of variance (ANOVA) was used to calculate the significance of differences between the two groups. All quantitative data are presented as mean ± standard error (S.E.). We considered P < 0.05 (*) statistically significant, and P < 0.01 (**) extremely statistically significant.
3. Results
3.1. Genomic DNA methylation level
At the six points used in the standard curve, there were 0, 0.5, 1.0, 2.0, 5.0, and 10.0 ng of the positive control (50% methylated DNA; (a)). The equation of the fitted curve was y = 392.32x+ 323.61 (R2=0.9876). Genomic DNA methylation levels in the TP testes (0.5291 ± 0.058)% were significantly lower than genomic DNA methylation levels in the YP testes (0.8041 ± 0.044%) (P < 0.01; (b)).
Figure 1. (a) The standard curve for quantifying genomic DNA methylation. (b) Genomic DNA methylation levels in testes. (c) Expression of DNA methyltransferases (DNMT1, DNMT3A, DNMT3B) and hypoxia-inducible factor 2α (HIF2α) mRNA in testes. (d) Western blot (WB) analysis of the four proteins (DNMT1, DNMT3A, DNMT3B and HIF2 α). ACTIN was used as a loading control. (e) Statistical results of WB for the four proteins in testes. Each bar represents mean ± standard error (S.E.), * Significant difference (P < 0.05), ** Extreme significant difference (P < 0.01). TP = Tibetan pig (n = 10); YP = Yorkshire pig (n = 10).
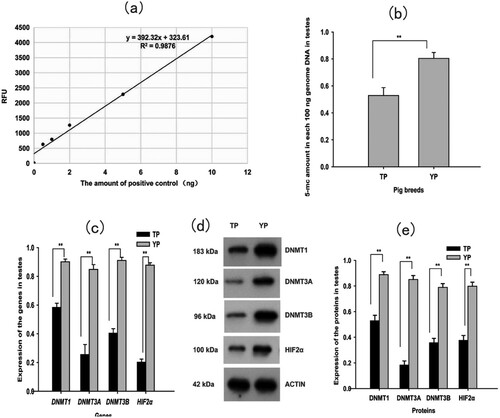
3.2. Quantitative real-time PCR
The mRNA expression levels of the four genes tested (DNMT1, DNMT3A, DNMT3B, and HIF2α) in the testes of TP and YP were determined using qRT-PCR. PCR efficiencies of DNMT1, DNMT3A, DNMT3B, HIF2α, and β-actin genes were within 95%–105% of levels that are satisfactory for qRT-PCR (Supplementary Figure 1 and Supplementary Figure 2). Expression levels of the four genes tested (DNMT1, DNMT3A, DNMT3B, and HIF2α) mRNA are shown in (c). We found that the mRNA expression levels of the four genes tested (DNMT1, DNMT3A, DNMT3B, and HIF2α) were all significantly lower in the TP testes as compared with the YP testes (P < 0.01; (c)).
3.3. Western blot
Western blots showed that the differences in protein expression levels between TP and YP were consistent with the differences in gene expression. That is, the expression levels of the four proteins tested (DNMT1, DNMT3A, DNMT3B, and HIF2α) were significantly lower in the TP testes as compared with the YP testes (P < 0.01; (d), (e), and Supplementary Figure 3).
4. Discussion
The DNMT family includes three main members, DNMT1, DNMT3a, and DNMT3b. DNA methylation, which controls DNA transcription, is a well-known epigenetic mechanism (Falck et al. Citation2012). Genome-wide profiling indicated that sperm DNA methylation was associated with buffalo (Bubalus bubalis) fertility (Verma et al. Citation2014). In mice, exposure to di-(2-ethylhexyl) phthalate (DEHP) during pregnancy may increase the level of DNA methylation in the testis of the offspring; this genetic modification may be an important cause of toxic reproductive lesions (Wu et al. Citation2009). Sperm DNA methylation status may be used as an early diagnostic marker for testicular changes not detected by conventional toxicity analysis (Sakai et al. Citation2018). Inhibition of DNA methylation in astrocytes may be a new therapeutic strategy for treating glutamate-induced excitotoxicity (Zhao et al. Citation2019).
DNA methylation levels might affect the ability of sperm to cause pregnancy during assisted reproduction and thus might be a useful area of study (Tunc and Tremellen Citation2009). The methylation of sperm DNA is impeded by damage due to oxidative stress (del Mazo et al. Citation1994). Alteration of the DNA methylation status of donor cells impaired the developmental competence of cloned porcine embryos (Huan et al. Citation2016). Levels of genome DNA methylation in two-week-old TP were significantly lower than those of two-week-old YP (Zhao et al. Citation2017), consistent with our results. That is, after sexual maturity, the level of genomic DNA methylation in the TP was significantly lower than in the YP. DNA methylation is catalyzed by DNA methyltransferases (DNMTs) through the transfer of the methyl group from S-adenosylmethionine (AdoMet) to cytosine (Bestor Citation2000), thus hindering the binding of transcription factors to promoters and inhibiting transcription (Jones and Takai Citation2001). DNMT1, DNMT3A, and DNMT3B are important DNA methylation transferases (Wu et al. Citation2007). DNMT1 replicates the DNA methylation pattern during DNA replication, while DNMT3A and DNMT3B are de novo DNA methyltransferases (Goll and Bestor Citation2005). The timing of meiosis entry depends on a marker generated by DNMT3A in the testes (Yaman and Grandjean Citation2006). De novo DNA methylation plays a role in nucleolar inactivation, the expression of DNMT3A transcripts, and the nucleolar localization of the DNMT3A protein in human testicular and fibroblast cells (Galetzka et al. Citation2006). The mRNA and protein expression levels of the DNMT3A gene in cancerous testicular tissues were significantly higher than those of the DNMT3A gene in normal testicular tissues (Chen et al. Citation2014). Chronic low-dose exposure to uranium increased the global methylation level in the rat testes as well as the mRNA expression levels of the DNA methyltransferase genes DNMT1 and DNMT3A/B; this DNA methylation profile was maintained across generations (Elmhiri et al. Citation2018). Here, both mRNA and protein expression levels of the three DNA methyltransferases (DNMT1, DNMT3A, and DNMT3B) were significantly lower in the TP testicular tissues than those in the YP testicular tissues. Thus, the levels of genomic DNA methylation in the TPs were significantly lower than those in the YPs. However, mRNA expression levels of DNMT1 and DNMT3A in the TP lungs were significantly higher than those in the YP lungs. This inconsistency might be due to differences between the tissues examined.
HIF2α plays an important role in regulating adaptations to hypoxic environments (Beall et al. Citation2010; Kaelin and Ratcliffe Citation2008; Rytkonen et al. Citation2013). Low O2 levels in the male gonad regulate germ cell homeostasis in this organ via HIF2α (Gruber et al. Citation2010). Hypoxia induces vascular endothelial growth factor (VEGF) gene transcription in human osteoblast-like cells through HIF2α (Akeno et al. Citation2001). VEGF supports germ cell survival and sperm production in bulls (Caires et al. Citation2009). HIF2α is important in pulmonary vascular remodeling and pulmonary hypertension, where it possibly is involved in upregulating the levels of endothelin1 (ET1) and norepinephrine (NE) (Brusselmans et al. Citation2003). The Tibetan version of HIF2α is associated with not only the relatively low hemoglobin level as a polycythemia protectant but also a low pulmonary vasoconstriction response in Tibetans. The down-regulation of HIF2α contributes to the molecular basis of Tibetans’ adaption to high-altitude hypoxia (Peng et al. Citation2017). Mutations in EPAS1 and transcriptional regulation differences in EGLN1 causing changes in downstream target transactivation are associated with a blunted hypoxic response (Graham and McCracken Citation2019). HIF2A mRNA expression levels in teleost gills and skin were significantly increased in acute hypoxia but decreased significantly after long-term hypoxia exposure; this suggested that teleosts might use different mechanisms to adapt to acute and long-term hypoxia (Jiang et al. Citation2015). The expression of HIF2α mRNA is significantly lower in the peripheral blood lymphocytes of Tibetans as compared with those of Han Chinese, suggesting that Tibetans respond less vigorously to hypoxic challenges. It is possible that as part of long-term hypoxic adaptations, erythropoietin expression is decreased in Tibetans via the downregulation of HIF2α; this process might reduce hemoglobin production, increase the speed of blood flow, enhance vascular ventilation, and maintain bodily oxygen homeostasis (Petousi et al. Citation2014). HIF2α mRNA expression in the testicular tissues of two-week-old Tibetan boars was significantly lower than in the testicular tissues of two-week-old YP (Zhao et al. Citation2017). These results were consistent with our findings.
In summary, our findings have identified a possible mechanism of plateau adaptation in the TP testes based on DNA methylation. Such an adaptation would be necessary to maintain the normal spermatogenic function of the testicles. On the one hand, compared with YP, the downregulation of DNA methyltransferases in the TP testes led to lower levels of genomic DNA methylation, which would facilitate higher sperm motility. On the other hand, HIF2α expression in the TP testes was down-regulated, which might contributes to TP testes’ adaption to the plateau environment. This study has provided new insights into the DNA methylation mechanisms underlying plateau adaptations in TP testicles; our findings may serve as a basis for the improvement of TP fecundity and the establishment of molecular breeding.
TAAR_1882465_Supplementary_Figures
Download Zip (61.3 KB)Acknowledgements
We thank LetPub (www.letpub.com) for its linguistic assistance during the preparation of this manuscript.
Disclosure statement
No potential conflict of interest was reported by the authors.
Additional information
Funding
References
- Akeno N, Czyzyk-Krzeska MF, Gross TS, Clemens TL. 2001. Hypoxia induces vascular endothelial growth factor gene transcription in human osteoblast-like cells through the hypoxia-inducible factor-2alpha. Endocrinology. 142(2):959–962. doi:10.1210/endo.142.2.8112.
- An X, Ma H, Han P, Zhu C, Cao B, Bai Y. 2018. Genome-wide differences in DNA methylation changes in caprine ovaries between oestrous and dioestrous phases. J Anim Sci Biotechnol. 9:85. doi:10.1186/s40104-018-0301-x.
- Beall CM, Cavalleri GL, Deng L, Elston RC, Gao Y, Knight J, Li C, Li JC, Liang Y, McCormack M, et al. 2010. Natural selection on EPAS1 (HIF2alpha) associated with low hemoglobin concentration in Tibetan highlanders. Proc Natl Acad Sci USA. 107(25):11459–11464. doi:10.1073/pnas.1002443107.
- Bestor TH. 2000. The DNA methyltransferases of mammals. Hum Mol Genet. 9(16):2395–2402. doi:10.1093/hmg/9.16.2395.
- Brusselmans K, Compernolle V, Tjwa M, Wiesener MS, Maxwell PH, Collen D, Carmeliet P. 2003. Heterozygous deficiency of hypoxia-inducible factor-2alpha protects mice against pulmonary hypertension and right ventricular dysfunction during prolonged hypoxia. J Clin Invest. 111(10):1519–1527. doi:10.1172/JCI15496.
- Caires KC, de Avila J, McLean DJ. 2009. Vascular endothelial growth factor regulates germ cell survival during establishment of spermatogenesis in the bovine testis. Reproduction. 138(4):667–677. doi:10.1530/REP-09-0020.
- Chen BF, Gu S, Suen YK, Li L, Chan WY. 2014. microRNA-199a-3p, DNMT3A, and aberrant DNA methylation in testicular cancer. Epigenetics. 9(1):119–128. doi:10.4161/epi.25799.
- del Mazo J, Prantera G, Torres M, Ferraro M. 1994. DNA methylation changes during mouse spermatogenesis. Chromosome Res. 2(2):147–152. doi:10.1007/bf01553493.
- Elmhiri G, Gloaguen C, Grison S, Kereselidze D, Elie C, Tack K, Benderitter M, Lestaevel P, Legendre A, Souidi M. 2018. DNA methylation and potential multigenerational epigenetic effects linked to uranium chronic low-dose exposure in gonads of males and females rats. Toxicol Lett. 282:64–70. doi:10.1016/j.toxlet.2017.10.004.
- Falck E, Groenhagen A, Muhlisch J, Hempel G, Wunsch B. 2012. Genome-wide DNA methylation level analysis by micellar electrokinetic chromatography and laser-induced fluorescence detection after treatment of cell lines with azacytidine and antifolates. Anal Biochem. 421(2):439–445. doi:10.1016/j.ab.2011.09.027.
- Feinberg AP. 2007. Phenotypic plasticity and the epigenetics of human disease. Nature. 447(7143):433–440. doi:10.1038/nature05919.
- Galetzka D, Tralau T, Stein R, Haaf T. 2006. Expression of DNMT3A transcripts and nucleolar localization of DNMT3A protein in human testicular and fibroblast cells suggest a role for de novo DNA methylation in nucleolar inactivation. J Cell Biochem. 98(4):885–894. doi:10.1002/jcb.20798.
- Goll MG, Bestor TH. 2005. Eukaryotic cytosine methyltransferases. Annu Rev Biochem. 74:481–514. doi:10.1146/annurev.biochem.74.010904.153721.
- Graham AM, McCracken KG. 2019. Convergent evolution on the hypoxia-inducible factor (HIF) pathway genes EGLN1 and EPAS1 in high-altitude ducks. Heredity (Edinb) 122(6):819–832. doi:10.1038/s41437-018-0173-z.
- Gruber M, Mathew LK, Runge AC, Garcia JA, Simon MC. 2010. EPAS1 is required for spermatogenesis in the postnatal mouse testis. Biol Reprod. 82(6):1227–1236. doi:10.1095/biolreprod.109.079202.
- Huan YJ, Wu ZF, Zhang JG, Zhu J, Xie BT, Wang JY, Li JY, Xue BH, Kong QR, Liu ZH. 2016. Alteration of the DNA methylation status of donor cells impairs the developmental competence of porcine cloned embryos. J Reprod Dev. 62(1):71–77. doi:10.1262/jrd.2015-048.
- Jiang HP, Zhang C, Wang CC, Xu QH. 2015. Cloning of HIF1B and HIF2A to hypoxia in Gymnocypris genes and their expressions response dobula and Schizothorax prenanti. Freshwater Fisheries. 45(5):11–18.
- Jin L, Mao K, Li J, Huang W, Che T, Fu Y, Tang Q, Liu P, Song Y, Liu R, et al. 2018. Genome-wide profiling of gene expression and DNA methylation provides insight into low-altitude acclimation in Tibetan pigs. Gene. 642:522–532. doi:10.1016/j.gene.2017.11.074.
- Jones PA, Takai D. 2001. The role of DNA methylation in mammalian epigenetics. Science. 293(5532):1068–1070. doi:10.1126/science.1063852.
- Kaelin WG, Jr., Ratcliffe PJ, 2008. Oxygen sensing by metazoans: the central role of the HIF hydroxylase pathway. Mol Cell 30(4):393–402. doi:10.1016/j.molcel.2008.04.009.
- Li C, Li X, Xiao J, Liu J, Fan X, Fan F, Lei H. 2019. Genetic changes in the EPAS1 gene between Tibetan and Han ethnic groups and adaptation to the plateau hypoxic environment. PeerJ. 7:e7943. doi:10.7717/peerj.7943.
- Li Y, Zhang L, Li Y, Li W, Guo Z, Li R, Hu X, Bao Z, Wang S. 2019. “Dynamics of DNA methylation and DNMT expression during gametogenesis and early development of scallop Patinopecten yessoensis.” Mar Biotechnol (NY) 21(2):196–205. doi:10.1007/s10126-018-09871-w.
- Livak KJ, Schmittgen TD. 2001. Analysis of relative gene expression data using real-time quantitative PCR and the 2(-Delta Delta C(T)) method. Methods. 25(4):402–408. doi:10.1006/meth.2001.1262.
- Mason K, Liu Z, Aguirre-Lavin T, Beaujean N. 2012. Chromatin and epigenetic modifications during early mammalian development. Anim Reprod Sci. 134(1-2):45–55. doi:10.1016/j.anireprosci.2012.08.010.
- Pan H, Chen Q, Qi S, Li T, Liu B, Liu S, Ma X, Wang B. 2018. Mutations in EPAS1 in congenital heart disease in Tibetans. Biosci Rep. 38(6):1–7. doi:10.1042/BSR20181389.
- Peng Y, Cui C, He Y, Ouzhuluobu, Zhang H, Yang D, Zhang Q, Bianbazhuoma, Yang L, He Y, et al. 2017. “Down-regulation of EPAS1 transcription and genetic adaptation of Tibetans to high-altitude hypoxia.” Mol Biol Evol 34(4):818–830. doi:10.1093/molbev/msw280.
- Petousi N, Croft QP, Cavalleri GL, Cheng HY, Formenti F, Ishida K, Lunn D, McCormack M, Shianna KV, Talbot NP, et al. 2014. Tibetans living at sea level have a hyporesponsive hypoxia-inducible factor system and blunted physiological responses to hypoxia. J Appl Physiol (1985). 116(7):893–904. doi:10.1152/japplphysiol.00535.2013.
- Ren ZL, Song TZ, Zhang J, Peng WC, He ZZ, Zhao YL. 2017. Effect of rhodiola polysaccharide on frozen semen quality of Tibetan boar and levels of sperm genome DNA methylation. Chin J Vet Sci. 37(7):1385–1388,1393.
- Rytkonen KT, Akbarzadeh A, Miandare HK, Kamei H, Duan C, Leder EH, Williams TA, Nikinmaa M. 2013. Subfunctionalization of cyprinid hypoxia-inducible factors for roles in development and oxygen sensing. Evolution. 67(3):873–882. doi:10.1111/j.1558-5646.2012.01820.x.
- Sakai K, Ideta-Otsuka M, Saito H, Hiradate Y, Hara K, Igarashi K, Tanemura K. 2018. Effects of doxorubicin on sperm DNA methylation in mouse models of testicular toxicity. Biochem Biophys Res Commun. 498(3):674–679. doi:10.1016/j.bbrc.2018.03.044.
- Suzuki MM, Bird A. 2008. DNA methylation landscapes: provocative insights from epigenomics. Nat Rev Genet. 9(6):465–476. doi:10.1038/nrg2341.
- Tunc O, Tremellen K. 2009. Oxidative DNA damage impairs global sperm DNA methylation in infertile men. J Assist Reprod Genet. 26(9-10):537–544. doi:10.1007/s10815-009-9346-2.
- Verma A, Rajput S, De S, Kumar R, Chakravarty AK, Datta TK. 2014. Genome-wide profiling of sperm DNA methylation in relation to buffalo (Bubalus bubalis) bull fertility. Theriogenology. 82(5):750–759.e1. doi:10.1016/j.theriogenology.2014.06.012.
- Wu SD, Zhu J, Li YS, Gan LQ, Yuan XG, Xu MD, Wei GH. 2009. [Di-(2-ethylhexyl) phthalate increases the DNA methylation level of genomes in the mouse testis]. Zhonghua Nan Ke Xue. 15(10):876–881.
- Wu Y, Strawn E, Basir Z, Halverson G, Guo SW. 2007. Aberrant expression of deoxyribonucleic acid methyltransferases DNMT1, DNMT3A, and DNMT3B in women with endometriosis. Fertil Steril. 87(1):24–32. doi:10.1016/j.fertnstert.2006.05.077.
- Yaman R, Grandjean V. 2006. Timing of entry of meiosis depends on a mark generated by DNA methyltransferase 3a in testis. Mol Reprod Dev. 73(3):390–397. doi:10.1002/mrd.20430.
- Yang S, Zhang H, Mao H, Yan D, Lu S, Lian L, Zhao G, Yan Y, Deng W, Shi X, et al. 2011. The local origin of the Tibetan pig and additional insights into the origin of Asian pigs. PLoS One. 6(12):e28215. doi:10.1371/journal.pone.0028215.
- Yuan X, Li Z, Ye S, Chen Z, Huang S, Zhong Y, Zhang H, Li J, Zhang Z. 2019. Genome-wide DNA methylation analysis of pituitaries during the initiation of puberty in gilts. PLoS One. 14(3):e0212630. doi: 10.1371/journal.pone.0212630
- Zhao H, Sun P, Fan T, Yang X, Zheng T, Sun C. 2019. The effect of glutamate-induced excitotoxicity on DNA methylation in astrocytes in a new in vitro neuron-astrocyte-endothelium co-culture system. Biochem Biophys Res Commun. 508(4):1209–1214. doi:10.1016/j.bbrc.2018.12.058.
- Zhao YL, Zhang B, Wang ZX, Zhang HL, Zhang H. 2017. Correlation of the DNA methylation level and HIF2α mRNA expression in Testicle tissue of Tibetan Pig. Chin J Vet Sci. 37(11):2222–2226.