ABSTRACT
Although polyunsaturated fatty acids (PUFAs) have gained attention because of their physiological effects on inflammation and stress, their derivatives, termed lipid mediators, have been rarely examined, especially in laying hens. In this study, we aimed to investigate PUFA and lipid mediator profiles of laying hens after feeding 0, 0.9, 1.8, or 3.6% (w/w) dietary flaxseed (Lintex170) for 4 weeks. We also monitored the indices of inflammation (serum proinflammatory cytokines) and stress (serum corticosterone, ratio of heterophils to lymphocytes). In this study, flaxseed reduced the omega-6 to omega-3 FA ratio and increased several omega-3 FA-derived lipid mediators. Furthermore, levels of the proinflammatory cytokine tumour necrosis factor alpha and two stress indices (corticosterone, heterophil to lymphocyte ratio) were decreased when fed the 3.6% flaxseed diet. Overall, laying performance indices were also significantly improved by flaxseed treatment. These findings suggest that flaxseed may alleviate the stress state of laying hens by enriching omega-3-derived lipid mediators, which could improve laying performance.
1. Introduction
Polyunsaturated fatty acids (PUFAs) have been reported to broadly regulate homeostatic and inflammatory processes, either directly or through transformations to bioactive metabolic compounds such as lipid mediators (Zarate et al. Citation2017). PUFAs can be classified into two principal families, omega-3 and omega-6 fatty acids, depending on the position of the first double bond from the methyl group (Mariamenatu and Abdu Citation2021). Among them, linoleic acid (LA), an omega-6 fatty acid and alpha-linolenic acid (ALA), an omega-3 fatty acid, are essential fatty acids that must be obtained from the diet; omega-3 fatty acids cannot be converted to omega-6 fatty acids, and vice versa in mammals and poultry (Alagawany et al. Citation2021). When they enter the body, they undergo bioconversion by elongases and desaturases, which result in the conversion of LA to arachidonic acid (AA) and ALA to eicosapentaenoic acid (EPA) and docosahexaenoic acid (DHA) (Shahidi and Ambigaipalan Citation2018). These PUFAs are incorporated into the cell membrane and used as resources to produce and release various derivatives termed lipid mediators, which are converted by cell membrane-incorporated enzymes such as lipoxygenases (LOXs), cyclooxygenases (COXs), and cytochrome P450 (CYP450) (Greene et al. Citation2011).
Lipid mediators are known for their roles in the onset and resolution of inflammation and the systemic profile of the lipid mediators can be nutritionally controllable depending on the quantity of ingested precursors such as omega-6 and omega-3 fatty acids (Serhan et al. Citation2015). Although the action mechanisms of the lipid mediators derived from both omega-3 and omega-6 fatty acids are too complex to be simplified in the physiological regulation of inflammation and its resolution (Shearer and Walker Citation2018), the various lipid mediators generally act as signal molecules that mainly mediate their anti-inflammatory and pro-resolution activity through G-coupled protein receptors (e.g. GPR32, GPR18, ChemR23, GPR37, and LGR6) (Hallisey et al. Citation2020). Omega-3-derived lipid mediators, including specialized pro-resolving lipid mediators, have been a focus because of their impact on the resolution of inflammation (Arnardottir et al. Citation2021). Cytokines are regulators of host responses to various immunological states, including inflammation. Some cytokines, such as tumour necrosis factor (TNF), interleukin-1 (IL-1), and interleukin-6 (IL-6), act as promoters of inflammation and are therefore called proinflammatory cytokines (Dinarello Citation2000). They play a key role in the acute phase response. However, when they persist in their actions so that inflammation is not resolved, chronic inflammation occurs, resulting in various problems in the body, such as tissue damage. Levels of circulating proinflammatory cytokines are elevated in several inflammatory diseases, such as Crohn’s disease (Gabay Citation2006; Nejm et al. Citation2017; Szczeklik et al. Citation2012) and obesity (Borges et al. Citation2018). Recently, the contribution of the network between lipid mediators and cytokines in immune homeostasis has been investigated. In general, the literature has reported that omega-6 derived lipid mediators increase proinflammatory cytokines, and omega-3-derived lipid mediators increase the level of anti-inflammatory cytokines and suppress that of proinflammatory cytokines (Schmitz and Ecker Citation2008). For these reasons, a balanced intake of omega-6 and omega-3 fatty acids is essential for homeostasis associated with inflammation control.
However, modern human diets and animal feeds commonly contain excessive levels of omega-6 fatty acids and low levels of omega-3 fatty acids. This is because of the high dependence on omega-6-rich resources, such as corn and soybean, to manufacture cooking oils and animal feeds. The ratio of omega-6 to omega-3 in modern diets was reported to have increased to approximately 20:1 from 1:1 in the past (Simopoulos Citation2016). Because fatty acid composition in the inner body has also been influenced by this omega-6 and omega-3 fatty acid imbalance in the diet, an increase in the incidence of chronic inflammatory diseases could occur (Khadge et al. Citation2018). Studies have found that omega-3 fatty acids counter the effects of chronic inflammatory diseases associated with cardiovascular diseases (Han et al. Citation2018; Nandish et al. Citation2018; Prasad Citation2019), central nervous system diseases (Czyż et al. Citation2016), diabetes (Zhu et al. Citation2020), obesity (Liu et al. Citation2021), and several cancers (D'Angelo et al. Citation2020; Liang et al. Citation2020; Tu et al. Citation2020). Similarly, the omega-6 to omega-3 fatty acid ratio in animal feed is also distorted. One of the rational inferences would be that livestock animals might experience problems such as chronic inflammation during feeding, which increases stress and adverse effects on their performance (Kang et al. Citation2020; Sevim et al. Citation2020). Therefore, restoration of the fatty acid composition through supplementation with omega-3 fatty acids in diets and feeds could be an applicable strategy to improve both human and animal health by recovering immune homeostasis.
Flaxseed has been widely used as a dietary supplement of omega-3 fatty acids in humans and animals because it contains abundant amounts of ALA, representing approximately 53% of total fatty acids (Moallem Citation2018). However, whole flaxseed has been known to contain anti-nutritional factors such as mucilage, cyanogenic glycosides, phytic acid and trypsin inhibitors, which could induce adverse effects such as malabsorption of primary bile acids and excretion of secondary bile acids (Mangi et al. Citation2021). One of the common treatments available to reduce the side effects of flaxseed is extrusion (Imran et al. Citation2015).
The literature has reported on omega-3 fatty acid-fortified livestock products through supplementation with omega-3 fatty acid-rich resources such as flaxseed (Antruejo et al. Citation2011; Gjerlaug-Enger et al. Citation2015; Zachut et al. Citation2010). However, according to a review of the literature, few reports have investigated the physiological effects of feeding omega-3 fatty acid-rich resources to livestock animals. In the present study, the effect of flaxseed supplementation was investigated in laying hens: specifically, the improvement in the omega-6 to omega-3 fatty acid ratio in eggs and animal physiology and health was assessed mainly by profiling lipid mediators after feeding. Based on the regulation of inflammation by lipid mediators and cytokines, this study’s hypothesis was that the supplementation of omega-3 fatty acids to laying hens could improve their performance by alleviating inflammation and stress during the laying period. To examine this hypothesis, the profile of lipid mediators and representative proinflammatory cytokines in serum, corticosterone levels, and the heterophil to lymphocyte ratio (H/L) for stress indices and the performance of laying hens were monitored and analyzed after supplementation with dietary flaxseed.
2. Materials and methods
2.1. Materials
The basal diet used in this study was commercial laying hen feed product provided by a domestic feed manufacturing company (Cargill Agri Purina, Inc., Seoul, Republic of Korea). Dietary flaxseed was obtained from a commercial product, Lintex170 (HANYOU BNF Co., Ltd, Seoul, Republic of Korea) which was developed by Seoul National University and HANYOU BNF Co., Ltd and manufactured by HANYOU BNF Co., Ltd. The product comprises 85% extruded flaxseed and 15% starch source; 17% of the product is ALA.
The nutrient compositions of the basal diet and dietary flaxseed used in this study are listed in . The lipid mediator standards were as follows: 14, 15-epoxyeicosatrienoic acid (EET)-d11, 5(S)hydroxyeicosatetraenoic acid (HETE)-d8, leukotriene B4 (LTB4)-d4, prostaglandin D2 (PGD2)-d4 and AA-d8 (Cayman Chemical, Ann Arbor, MI, USA). The fatty acid methyl ester standard (Supelco® 37 Component FAME Mix, FAME 37), internal standard (tridecanoic acid, C13:0), potassium hydroxide, and sulfuric acid were purchased from Sigma-Aldrich (St. Louis, MO, USA). HPLC-grade water, acetonitrile, isopropyl alcohol, and hexane were purchased from J.T. Baker (Avantor Performance Material, Inc., Center Valley, PA, USA). A Strata-x-33-μm polymerized solid reverse-phase extraction column (Cat no.8B-S100-UBJ) was purchased from Phenomenex (Torrance, CA, USA).
Table 1. Nutrient composition of experimental diet.
2.2. Animals, treatments, and management
The experiments associated with laying hens (n = 200, 33 weeks old, Lohmann Brown-Lite, initial body weight (kg): 1.89 ± 0. 05) were conducted at the Seoul National University Animal Farm (Pyeongchang, Republic of Korea). The protocol for this experiment was reviewed and approved by the Institutional Animal Care and Use Committee of Seoul National University (IACUC No. SNU-180219-1). The hens were randomly selected from 2000 hens of experimental animal farms and divided randomly into four groups with five hens per cage (48 cm × 45 cm × 45 cm, width × depth × height) and 10 cages per group. Fifty hens were assigned to each of the following diet treatments for 4 weeks. The experimental diets were prepared by mixing each assigned proportion (w/w) of Lintex170 (extruded flaxseed product) into the basal diet as follows: (1) commercial basal diet (C), (2) basal diet with 0.9% (w/w) Lintex170 (T1), (3) basal diet with 1.8% (w/w) Lintex170 (T2), and (4) basal diet with 3.6% (w/w) Lintex170 (T3). The ratio of omega-6 to omega-3 of each feed was 21.82 (C), 8.04 (T1), 5.28 (T2), and 2.94 (T3), calculated by comparison with the FAME 37 peak pattern (). Each experimental diet was offered (600 g per cage) daily, and fresh water was offered ad libitum during the experimental period. The hen house was maintained at approximately 25 °C, was lit for 10 h/day, and auto-ventilated by a built-in Information and Communications Technology (ICT) management automation system during the experimental period.
Table 2. Fatty acid compositions of experimental diets.
2.3. Sample and data collection
The performance of each cage was also assessed. The number of eggs and their weights were recorded daily. Abnormal eggs, such as broken, cracked, or shell-less eggs, were also recorded daily. Feed intake was measured weekly, and the weekly feed conversion ratio [feed consumed (g)/egg mass (g)] was calculated. Egg samples [10 eggs/(group × week)] were randomly selected and analysed for albumen height, Haugh unit, eggshell thickness, and egg fatty acid profile. Albumen height was measured using a micrometre. The Haugh unit was calculated with egg weight by using the following formula: Haugh unit = 100 × log (H + 7.57 − 1.7 × W0.37), where H is albumen height (mm) and W is egg weight (g) (Eisen et al. Citation1962). Eggshell thickness was determined by averaging measurements taken at three locations on the egg (air cell, equator, and sharp end) by using a dial pipe gauge. After these procedures, 1 g of egg yolk was separated and collected in a glass tube to measure the egg fatty acid profile.
Blood samples were collected from 10 randomly selected hens per group at Weeks 0, 2, and 4. They were collected in two tubes: BD Vacutainer SSTTM I Advance Tubes (Becton Dickinson, Le Pont de Claix, France) for sampling serum and V-TubeTM EDTA K3 Tubes (AB MEDICAL, Republic of Korea) to measure complete blood cell count. Blood samples were centrifuged at 3,887 × g for 10 min, and the supernatant serum was transferred to 1.5 mL plastic tubes and stored at −20 °C until use. Complete blood cell counts were immediately determined after collecting whole blood samples as quickly as possible using a hematology analyser (Advia 2120i, Siemens; Erlangen, Germany).
2.4. Gas chromatography for fatty acid profile
The fatty acid profiles were analysed using feed, egg, and serum samples (feed, 1 g × 3 replicates; egg, 1 g × 5 replicates; serum, 500 μL × 5 replicates). Fatty acids were extracted and methylated in one tube via the direct methylation method (O'Fallon et al. Citation2007) with some modifications. Briefly, tridecanoic acid (0.5 mg) was added to the samples in 15 mL glass tubes, followed by the addition of 5.3 mL of methanol and 700 μL of 10 N KOH. The tubes were incubated in a water bath at 55 °C for 1.5 h with brief vortexing every 20 min. Next, the tubes were chilled at RT, and 580 μL of 24 N H2SO4 was added. The incubation and chilling steps were repeated. Finally, 3 mL of hexane was added, and the sample was vortexed for 5 min and centrifuged at 3,000 rpm for 5 min. The upper phase was transferred to a GC vial (Agilent, Santa Clara, CA, USA) and analysed with a gas chromatography-flame ionisation detector (GC-FID, Agilent 7890B, Santa Clara, CA, USA) with SP-2560 (100 m × 0.25 mm, L × I.D; 0.2 μm, df, Sigma-Aldrich, St. Louis, MO, USA). FAME 37 was used as a reference for peak identification. The operating conditions of GC-FID followed the FAME 37 manual (oven temperature: 140 °C, 5 min; ramp: 240 °C at 4 °C/min and hold for 28 min; injector and detector temperature: 260 °C; split ratio: 1:30; injection volume: 1 μL).
2.5. Ultra-performance liquid chromatography for serum lipid mediator profile
Lipid mediators in serum were separated by the solid-phase extraction method (Kortz et al. Citation2014) using a Strata-x-33-μm polymerized solid reverse-phase extraction column. Briefly, the columns were activated with 3.5 mL of methanol followed by the same amount of water for equilibration. Samples were prepared by adding 10 ng of each deuterated internal standard to 1 mL of serum with 200 μL of methanol and 800 μL of distilled water. Samples were then loaded onto columns and washed with 10% methanol (3.5 mL) in distilled water. Finally, the samples were eluted using 1 mL of methanol. These samples were concentrated using a Speed-Vac concentrator (Labconco, Kansas City, MO, USA), resuspended in 90 μL of solvent A (70:30:0.02, water:acetonitrile:acetic acid, v:v:v) and stored at −80 °C until use.
The Ultra-Performance Liquid Chromatography (UPLC) analyses and tandem mass spectrometry (MS/MS) analyses followed reported methods (Lee et al. Citation2016a). The Skyline 21.1 software package (MacCoss Laboratory, Seattle, WA, USA) was used to determine the peak area of each lipid from raw data. The extracted peak areas were normalized by internal standard.
2.6. Enzyme-linked immunosorbent assay for serum proinflammatory cytokine and corticosterone levels
The levels of serum TNF-alpha, IL-1beta, IL-6 and corticosterone were determined using Week 0 and Week 4 serum samples (5 replicates per group) by using ELISA kits specific for chicken TNF-alpha, IL-1beta, IL-6, and corticosterone (CUSABIO, Wuhan, China) and following the manufacturer’s method.
2.7. Complete blood cell count for heterophils to lymphocytes (H/L) ratio
For assessing the stress state of hens, the H/L ratios were measured using the complete blood cell count method. First, 10 μL blood samples in EDTA K3 tubes were collected to make blood smears on glass slides. The smears were stained with Wright’s stain. White blood cells, such as heterophils, lymphocytes, eosinophils, and monocytes, were identified using a compound microscope (Axio Scope.A1, Carl Zeiss, Germany), and the ratio of H/L was calculated.
2.8. Statistical analysis
Using SAS 9.3, significant differences among the four groups were determined by one-way ANOVA with post-hoc Tukey Honest Significant Difference tests. For the comparison of the before and after flaxseed supplementation in each group, paired two-sided Student’s t-tests were conducted with data from Weeks 0 and 4. The fixed effect (Trt) in this model was a flaxseed (Lintex 170) treatment. Orthogonal polynomial contrasts were also used to validate the linear (Lin) and quadratic (Quad) effects when the level of Lintex 170 supplementation increased (* p < 0.05; ** p < 0.01; *** p < 0.001). Variability in the data is shown as pooled SEM. Partial least squares discriminant analysis (PLS-DA) plots of quantified lipid mediators and variable importance in projection (VIP) plots of 15 lipid mediators (VIP scores Top 15) were performed using the MetaboAnalyst 4.0 website (Chong et al. Citation2018) to determine the discriminant lipid mediators.
3. Results
3.1. Flaxseed improved ratio of omega-6 to omega-3
Flaxseed supplementation improved the omega-6 to omega-3 ratios in egg samples (). The omega-6 to omega-3 ratio decreased with increasing flaxseed concentration. The average ratios in eggs at week 4 for the different treatment groups were 9.81 (T1), 6.87 (T2) and 4.16 (T3), compared with 25.85 in the basal diet (C). The content of each PUFA component was also similar between egg and serum samples. Likewise, the average ratios in serum samples were 8.33 (T1), 5.66 (T2), and 4.08 (T3), as compared with the value of 19.23 in the basal diet (C) (Fig. S1).
Table 3. Fatty acid compositions of egg yolk samples at the end of the experimentTable Footnote1.
3.2. Flaxseed altered serum lipid mediator profile
Exploiting the multiple-reaction monitoring method based on UPLC-MS/MS, the profiles of lipid mediators in serum samples of laying hens at week 4 were investigated. A total of 91 lipid mediators were identified in the serum samples (Table S1).
Partial least square discriminant analysis (PLS-DA) was performed to observe the dissimilarities of the lipid mediators among the groups ()). PLS-DA plot analysis revealed clustering among groups according to flaxseed concentration in the corresponding PLS-DA score plot including Component 1 (19.4%) and Component 2 (11.7%). ) shows the VIP plot of 15 lipids (VIP scores of Top 15) that were differentially regulated among the four groups, which shows that individual lipid mediator species, such as hydroxyoctadecatrienoic acid (HOTrE), prostaglandin F3 alpha (PGF3α), and hydroxydocosahexaenoic acid (HDoHE), mainly contributed to the clustering among groups.
Figure 1. (A) Partial least squares discriminant analysis (PLS-DA) plot of lipid mediators obtained from the serum of laying hens fed 0, 0.9, 1.8 and 3.6% (w/w) flaxseed for 4 weeks. (B) Variable importance in projection (VIP) plot of 15 lipid mediators (VIP scores Top 15) that were differentially regulated among groups. C, 0% (w/w) flaxseed; T1, C + 0.9% (w/w) flaxseed; T2, C + 1.8% (w/w) flaxseed; T3, C + 3.6% (w/w) flaxseed. The colored boxes indicate the relative concentration of each group per lipid mediator. Lipid mediators whose relative concentrations were significantly differed among group are marked in bold italic (one-way ANOVA, P < 0.05).
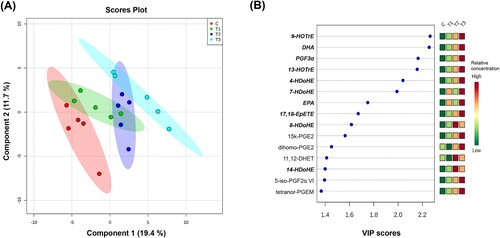
Next, the concentrations of serum PUFAs and lipid mediators among groups were analysed by one-way ANOVA and orthogonal polynomial contrasts to determine the effects of flaxseed on the host lipid profile. In the case of PUFAs, the level of AA, an omega-6 PUFA, was lower in the flaxseed-fed groups (T1, T2, and T3) than in the C group ()), whereas the levels of EPA and DHA were higher in the T3 group ((b,c)). In addition, concentrations of several lipid mediators were altered by flaxseed treatment. Levels of lpid mediators derived from the omega-3 fatty acids, ALA (9-HOTrE, p = 0.004 for Trt, <0.001 for Lin) ()), EPA (PGF-3α, p = 0.038 for Trt) ()) and DHA (4-HDoHE, p = 0.022 for Trt, 0.006 for Lin) ()), increased linearly with flaxseed addition. Additionally, the levels of one ALA-derived lipid mediator, 13-HOTrE (p = 0.014 for Quad) (Fig. S2); two EPA-derived lipid mediators, 5-HEPE (p = 0.045 for Lin) (Fig. S3A) and 9-HEPE (p = 0.023 for Lin) (Fig. S3B); and three DHA-derived lipid mediators, 8-HDoHE (p = 0.013 for Trt, 0.017 for Lin, and 0.022 for Quad) (Fig. S4A), and 14-HDoHE (p = 0.026 for Lin) (Fig. S4B), and 16-HDoHE (p = 0.018 for Lin) (Fig. S4C), were also increased by dietary supplementation with flaxseed.
Figure 2. Bar plots of relative concentrations of polyunsaturated fatty acids from the serum of laying hens fed 0, 0.9, 1.8 and 3.6% (w/w) flaxseed for 4 weeks. AA (A), EPA (B), and DHA (C) were determined in the sera of laying hens fed diets containing flaxseed for 4 weeks and measured by LC-MS/MS with 5 replicates per group. AA, arachidonic acid; EPA, eicosapentaenoic acid; DHA, docosahexaenoic acid; C, 0% (w/w) flaxseed; T1, C + 0.9% (w/w) flaxseed; T2, C + 1.8% (w/w) flaxseed; T3, C + 3.6% (w/w) flaxseed.
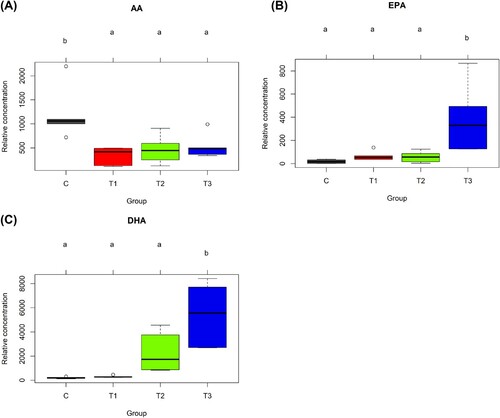
Figure 3. Bar plots of relative concentrations of lipid mediators from serum of laying hens fed 0, 0.9, 1.8 and 3.6% (w/w) flaxseed for 4 weeks. 9-HOTrE, ALA-derived lipid mediator (A), PGF3α, EPA-derived lipid mediator (B), and 4-HDoHE, DHA-derived lipid mediator (C) were determined in the sera of laying hens fed diets containing flaxseed for 4 weeks and measured by LC-MS/MS with 5 replicates per group. HOTrE, hydroxyoctadecatrienoic acid; PGF, prostaglandin F; HDoHE, hydroxydocosahexaenoic acid; C, 0% (w/w) flaxseed; T1, C + 0.9% (w/w) flaxseed; T2, C + 1.8% (w/w) flaxseed; T3, C + 3.6% (w/w) flaxseed.
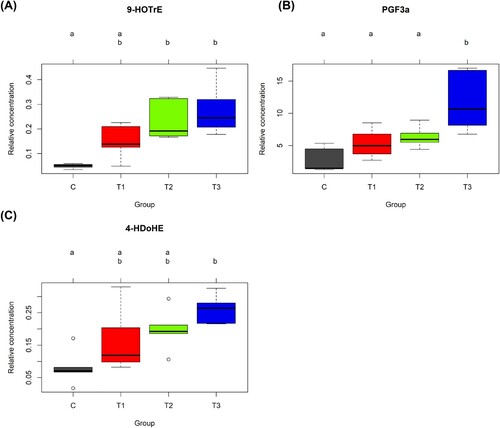
3.3. Flaxseed decreased proinflammatory cytokine levels in serum
For evaluating the effect of dietary flaxseed-induced changes in lipid mediator profiles on inflammatory processes, cytokine levels were examined in serum samples from laying hens (). No significant differences among groups were observed at any level of TNF-alpha, IL-1beta, or IL-6 in serum samples at week 4. However, when comparing the values of Weeks 0 and 4 per group, the levels of TNF-alpha (p = 0.043) were significantly decreased in the T3 group.
Table 4. The levels of serum proinflammatory cytokine (TNF-alpha, IL-1beta and IL-6), serum corticosterone, and heterophil to lymphocyte ratio in experimental hens at week 0 and week 4Table Footnote1
3.4. Flaxseed suppressed serum corticosterone levels and lowered the H/L ratio
In the investigation of the effect of flaxseed supplementation on stress state, two stress indices, serum corticosterone level and H/L ratio, were examined (). During the experiment, the T3 group had significantly lower serum corticosterone levels than in Week 0 (p = 0.046). Similarly, the T2 (p = 0.015) and T3 (p < 0.001) groups also showed significantly decreased H/L ratios compared with those in Week 0. The results showed that flaxseed supplementation decreased both stress indices.
3.5. Performance and egg quality
By supplementing with flaxseed, laying performance such as average egg weight and feed conversion ratio, was changed (). Average egg weight was elevated in all treatment groups during the overall period (p < 0.001 for Trt, 0.022 for Lin, and <0.001 for Quad). Although no significant difference was observed among the groups in feed intake during the experiment, the feed conversion ratio improved over the entire period (p < 0.001 for Trt, 0.018 for Lin, < 0.001 for Quad). The average egg weight and feed conversion ratio were the highest in the T2 group, which showed a quadratic effect of flaxseed supplementation. Collectively, dietary supplementation with flaxseed was observed to have positive effects on the performance of laying hens. Additionally, indices of egg quality (Haugh unit and eggshell thickness) showed no notable improvement with flaxseed supplementation ().
Table 5. Effects of flaxseed in the diet on the performance and egg quality of laying hens during 33–37 weeks of ageTable Footnote1
4. Discussion
Because PUFAs have been studied for their crucial roles in controlling inflammation, the imbalance in the omega-6 to omega-3 PUFA ratio in modern human diets due to excessive content of omega-6 fatty acids has been suspected to be a possible cause of many diseases involving chronic inflammation (Subash-Babu and Alshatwi Citation2018). Thus, restoration of balance is a relevant issue, and sufficient intake of omega-3 fatty acid-rich diets is often recommended as a solution for the maintenance of a healthy life.
In the livestock industry, corn, an ingredient with a high content of omega-6 fatty acids, is mainly used as an energy source in animal feed (Ayaşan et al. Citation2020). Thus, the omega-6 to omega-3 PUFA ratio of livestock products such as meat, eggs, and milk is generally unbalanced, which could adversely affect the health of consumers. Hence, studies have attempted to produce omega-3 fatty acid-fortified livestock products, and dietary supplementation with omega-3 fatty acid-rich feed ingredients, such as flaxseed, has resulted in the successful production of meats, eggs, and milk with an improved omega-6 to omega-3 fatty acid ratio (Anjum et al. Citation2013; Lee et al. Citation2021; Rico et al. Citation2021). However, although studies on livestock products with a balanced omega-6 to omega-3 PUFA ratio from the view of consumers have been conducted, few studies have reported the physiological effects of omega-6 to omega-3 PUFA ratio-modulated feeds from the view of livestock health. Specifically, lipid mediator profiles of livestock have rarely been studied, despite their strong biological impact on chronic inflammation.
Laying hens are prone to exposure to inflammatory stimuli and stress because of the practices used: high stocking density, intensive production cycles, and relatively harsh breeding environments. Therefore, this study aimed to evaluate the potential availability of dietary flaxseed as a functional feed additive by modulating lipid mediator profiles and alleviating the chronic inflammation and stress states in laying hens associated with animal welfare problems. With the goal of mimicking stress conditions caused by dense stocking, the stocking density (432 cm2/hen) was set to be higher than that in other studies on laying hens (Erensoy et al. Citation2021; Liebers et al. Citation2019; Wang et al. Citation2021).
The supplementation levels of dietary flaxseed used in this study were set up to implement the FAO-recommended human nutrition omega-6 to omega-3 ratio (4:1) (FAO Citation2010). In a preliminary experiment, laying hens were fed with flaxseed supplementation at various concentrations for two weeks and 3.6% (w/w) dietary flaxseed was suggested as an optimum treatment level because the omega-6 to omega-3 ratio reached nearly 4:1 in Week 2 after feeding (data not shown). Considering a report that more than 5% dietary flaxseed had undesirable effects such as growth depression in chicks (Feng et al. Citation2003), from the optimum concentration of 3.6% (w/w), a half [1.8% (w/w)] and a quarter [0.9% (w/w)] amounts of dietary flaxseed were tested to determine linear and quadratic effects on laying hens.
As shown in , the supplementation of flaxseed resulted in a decrease in the omega-6 to omega-3 fatty acid ratios in laying hens as the omega-3 fatty acid content increased. Notably, the 3.6% (w/w) dietary flaxseed supplement group (T3) stably maintained a 4:1 ratio of omega-6 to omega-3 fatty acid in both eggs and sera after 4 weeks. Similar results were observed in a prior study by our research group (Lee et al. Citation2016b). When flaxseed oil (ALA, 55%) was supplemented in the diet of laying hens, 0.5% and 1.0% (w/w) of flaxseed oil treatment groups showed an omega-6 to omega-3 ratio of approximately 4:1 and 2:1 in eggs, respectively, in the second week after feeding. In addition, ALA-rich flaxseed oil treatment prominently increased not only ALA but also long-chain omega-3 fatty acids such as EPA and DHA in eggs. Consistent with the prior result, this study showed increases in the proportion of ALA (egg: 0.3–2.5; serum: 0.4–2.4) and DHA (egg: 0.5–2.0; serum: 0.5–2.0) in both eggs and sera at the fourth week after feeding (Fig. S1).
Notably, the results in the prior and present studies showed that the omega-6 to omega-3 fatty acid ratio in laying hens changed proportionally with supplementation levels of flaxseed sources. These findings suggest that the fatty acid composition and omega-6 to omega-3 ratio in livestock can be controlled by appropriate feed resources.
PUFAs are released from the cell membrane when the expression of phospholipase A2 is induced by inflammatory stimuli (e.g. lipopolysaccharide); next, the free form of PUFAs is transformed into a series of lipid mediators by COXs, LOXs, and CYP450 (Mouchlis and Dennis Citation2019). Other human studies have reported that dietary omega-3 fatty acid increases the omega-3 fatty acid content of erythrocyte membranes (Drobnic et al. Citation2017; Jacek et al. Citation2020), and dietary flaxseed probably also modulates the fatty acid composition of erythrocytes, which might have a direct effect on the alteration of lipid mediator profile in laying hens.
From a total of 91 lipid mediators identified by UPLC-MS/MS, nine lipid mediators (9-HOTrE, 13-HOTrE, PGF3α, 5-HEPE, 9-HEPE, 4-HDoHE, 8-HDoHE, 14-HDoHE, and 16-HDoHE) showed significant alterations among the groups by both one-way ANOVA and contrast analysis. Notably, these lipid mediators originated from omega-3 fatty acids. Thus, it can be inferred that dietary flaxseed enriched the lipid mediators whose precursors were omega-3 fatty acids. Although few studies have investigated the lipid mediator profile and its shift by diet in laying hens, the finding is consistent with other studies using humans fed with fish oil (Lundstrom et al. Citation2013) or mice fed with flaxseed oil (Sawane et al. Citation2019).
Omega-3 fatty acids and their derivatives have been reported to exert anti-inflammatory effects (Ren et al. Citation2007). For example, the ALA-derived lipid mediators, 9-HOTrE and 13-HOTrE, the levels of which were increased by flaxseed supplementation in this study, have anti-inflammatory effects by inactivating the nod-like receptor family, the pyrin domain containing 3 (NLRP3) inflammasome (Kumar et al. Citation2016; Yang et al. Citation2017; Zahradka et al. Citation2017). Likewise, EPA-derived lipid mediators (PGF3α, 5-HEPE, 9-HEPE) and DHA-derived lipid mediators (4-HDoHE, 8-HdoHE, 14-HDoHE and 16-HDoHE) have been identified as anti-inflammatory lipid metabolites that counteract proinflammatory signals in other animals such as mice, rats and monkeys (Devassy et al. Citation2016; Nagatake et al. Citation2018; Onodera et al. Citation2017; Wang et al. Citation2017; Yang et al. Citation2014). 5-HEPE is particularly recognized as a potent inducer of regulatory T cells that play a pivotal role in regulating excessive immune responses (Onodera et al. Citation2017).
In addition to changes in lipid mediator profiles, serum levels of several representative proinflammatory cytokines and stress indices were investigated to determine whether dietary flaxseed affects the host’s inflammation and stress state, as many studies have demonstrated the crosstalk between inflammation and stress such as stress-stimulated production of proinflammatory cytokines, through the activation of the nuclear factor kappa B (NF-κB) signalling pathway (Chen et al. Citation2018).
As a result, although the serum levels of proinflammatory cytokines such as IL-1beta and IL-6 showed only ambiguous tendencies among the groups, the serum level of TNF-alpha and two stress indices (corticosterone, H/L ratio) in laying hens (Kaab et al. Citation2018) were significantly reduced by dietary flaxseed supplementation, which indicates its potential effects on alleviating inflammation and the stress state of laying hens. The decreased concentration of the proinflammatory cytokine, TNF-alpha, by dietary omega-3 fatty acid is also consistent with a prior study (Flock et al. Citation2014). Collectively, the results confirmed our original hypothesis because dietary supplementation of omega-3 fatty acid (ALA) from flaxseed could increase the omega-3 derived anti-inflammatory lipid mediators and reduce the strass indices in laying hens.
The flaxseed-fed hens also showed an improvement in overall laying performance. Slight increases in metabolizable energy (Mcal/kg; C: 2.70, T1: 2.72, T2: 2.74, T3: 2.79) and fat content (g/kg feed; C: 2.50, T1: 2.78, T2: 3.07, T3: 3.64) may positively affect laying performance, due to the extra addition of the extruded flaxseed product to the basal diets in test groups (T1 to T3). These differences were considered acceptable to validate the effect of feed additives in other studies (Aguillon-Paez et al. Citation2020; Damaziak et al. Citation2021; Tamiru et al. Citation2021). Compositional differences in other calculated nutrients between the control group and test groups, such as crude protein, crude fibre, crude ash, calcium, phosphorus, and amino acids were all less than 1% of the total feed. Thus, what is inferred is that dietary flaxseed contributes to improving the performance of laying hens by enriching omega-3-derived lipid mediators and alleviating inflammation and stress states.
5. Conclusions
The results of this study imply that dietary supplementation of flaxseed to laying hens could enable the production of omega-3 PUFA-fortified eggs and alleviate inflammation and stress indices by upregulating the anti-inflammatory lipid mediators originating from omega-3 fatty acids, which consequently improved overall laying performance. Therefore, a conclusion is that adjusting the physiological balance between omega-6 and omega-3 PUFA would benefit productivity and the health of farm animals. This report is the first report demonstrating changes in lipid mediator profiles by a feed additive in laying hens. Moreover, this study suggests that flaxseed might be used as a new category of functional feed additive with anti-inflammatory and anti-stress effects in the livestock industry associated with animal welfare issues. In addition, research on differentially regulated lipid mediators depending on dietary supplementation of lipid resources would expand understanding of the mechanisms underlying the regulation of immune homeostasis in livestock animals.
TAAR_2000416_Supplementary_material
Download MS Word (351.5 KB)Disclosure statement
No potential conflict of interest was reported by the author(s).
Additional information
Funding
References
- Aguillon-Paez YJ, Romero LA, Diaz GJ. 2020. Effect of full-fat sunflower or flaxseed seeds dietary inclusion on performance, egg yolk fatty acid profile and egg quality in laying hens. Anim Nutr. Jun. 6:179–184. Epub 2020/06/17.
- Alagawany M, Elnesr SS, Farag MR, El-Sabrout K, Alqaisi O, Dawood MAO, Soomro H, Abdelnour SA. 2021. Nutritional significance and health benefits of omega-3, -6 and -9 fatty acids in animals. Anim Biotechnol. doi:10.1080/10495398.2020.1869562.
- Anjum FM, Haider MF, Khan MI, Sohaib M, Arshad MS. 2013. Impact of extruded flaxseed meal supplemented diet on growth performance, oxidative stability and quality of broiler meat and meat products. Lipids Health Dis 12:1–12.
- Antruejo A, Azcona JO, Garcia PT, Gallinger C, Rosmini M, Ayerza R, Coates W, Perez CD. 2011. Omega-3 enriched egg production: the effect of alpha -linolenic omega -3 fatty acid sources on laying hen performance and yolk lipid content and fatty acid composition. Br Poult Sci. 52:750–760. Epub 2012/01/10.
- Arnardottir H, Pawelzik SC, Wistbacka UO, Artiach G, Hofmann R, Reinholdsson I, Braunschweig F, Tornvall P, Religa D, Back M. 2021. Stimulating the resolution of inflammation through omega-3 polyunsaturated fatty acids in COVID-19: rationale for the COVID-omega-F trial. Front Physiol. 11:624657. doi:10.3389/fphys.2020.624657.
- Atteh J, Leeson S. 1985. Effects of dietary fat level on laying hens fed various concentrations of calcium. 64:2090-2097.
- Ayaşan T, Çetinkaya N, Aykanat S, Çelik C. 2020. Nutrient contents and in vitro digestibility of different parts of corn plant. S Afr J Anim Sci. 50(2):302–309.
- Borges MD, Franca EL, Fujimori M, Silva S, de Marchi PG, Deluque AL, Honorio-Franca AC, de Abreu LCJE, Metabolic, Targets ID-D. 2018. Relationship between proinflammatory cytokines/chemokines and adipokines in serum of young adults with obesity.18:260-267.
- Chen J, Lobb IT, Morin P, Novo SM, Simpson J, Kennerknecht K, von Kriegsheim A, Batchelor EE, Oakley F, Stark LA. 2018. Identification of a novel TIF-IA-NF-kappaB nucleolar stress response pathway. Nucleic Acids Res 46:6188–6205. Epub 2018/06/07.
- Chong J, Soufan O, Li C, Caraus I, Li S, Bourque G, Wishart DS, Xia J. 2018. Metaboanalyst 4.0: towards more transparent and integrative metabolomics analysis. Nucleic Acids Res 46:W486–W494. Epub 2018/05/16.
- Czyż K, Bodkowski R, Herbinger G, Librowski T. 2016. Omega-3 fatty acids and their role in central nervous system-a review.23:816-831.
- Damaziak K, Riedel J, Marzec A, Kowalska H, Niemiec J, Gozdowski D, Cholcha I. 2021. Effects of replacement genetically modified soybean meal by a mixture of: linseed cake, sunflower cake, guar meal and linseed oil in laying hens diet. production results and eggs quality. Anim Feed Sci Tech. 271:114729. doi:10.1016/j.anifeedsci.2020.114729.
- D'Angelo S, Motti ML, Meccariello R. 2020. omega-3 and omega-6 polyunsaturated fatty acids. Obesity and Cancer. Nutrients. 12(9):2751.
- Devassy JG, Yamaguchi T, Ibrahim N, Gabbs M, Winter T, Ravandi A, Aukema HM. 2016. Dietary alpha-linolenic acid (ALA) rich flax Oil elevates renal and hepatic docsosahexaenoic acid (DHA) derived bioactive lipids. FASEB J. 30:pp.267-5.
- Dinarello CA. 2000. Proinflammatory cytokines. Chest. 118:503–508. Epub 2000/08/11.
- Drobnic F, Rueda F, Pons V, Banquells M, Cordobilla B, Domingo JC. 2017. Erythrocyte omega-3 fatty acid content in elite athletes in response to omega-3 supplementation: A dose-response pilot study. J Lipids. 2017. doi:10.1155/2017/1472719.
- Eisen E, Bohren B, McKean HJPS. 1962. The haugh unit as a measure of egg albumen quality. Poultry Sci. 41:1461–1468.
- Erensoy K, Sarica M, Noubandiguim M, Dur M, Aslan R. 2021. Effect of light intensity and stocking density on the performance, egg quality, and feather condition of laying hens reared in a battery cage system over the first laying period. Trop Anim Health Prod. 53(2):1–13. doi:10.1007/s11250-021-02765-5.
- FAO. 2010. Fats and fatty acids in human nutrition. Report of an expert consultation, 10-14 November 2008, Geneva.
- Feng DY, Shen YR, Chavez ER. 2003. Effectiveness of different processing methods in reducing hydrogen cyanide content of flaxseed. J Sci Food Agr. 83:836–841.
- Flock MR, Skulas-Ray AC, Harris WS, Gaugler TL, Fleming JA, Kris-Etherton PM. 2014. Effects of supplemental long-chain omega-3 fatty acids and erythrocyte membrane fatty acid content on circulating inflammatory markers in a randomized controlled trial of healthy adults. Prostag Leukotr Ess. 91:161–168.
- Gabay C. 2006. Interleukin-6 and chronic inflammation. Arthritis Res Ther. 8(2):1–6.
- Gjerlaug-Enger E, Haug A, Gaarder M, Ljokjel K, Stenseth RS, Sigfridson K, Egelandsdal B, Saarem K, Berg P. 2015. Pig feeds rich in rapeseed products and organic selenium increased omega-3 fatty acids and selenium in pork meat and backfat. Food Sci Nutr. 3:120–128. Epub 2015/04/04.
- Greene ER, Huang S, Serhan CN, Panigrahy D. 2011. Regulation of inflammation in cancer by eicosanoids. Prostag Oth Lipid M. 96:27–36.
- Grobas S, Mendez J, Lazaro R, de Blas C, Mateos GG. 2001. Influence of source and percentage of fat added to diet on performance and fatty acid composition of egg yolks of two strains of laying hens. Poultry Sci. 80:1171–1179.
- Hallisey VM, Kipper FC, Moore J, Gartung A, Bielenberg DR, Petrik J, Lawler J, Panigrahy D, Serhan CN. 2020. Pro-Resolving lipid mediators and anti-angiogenic therapy exhibit synergistic anti-tumor activity via Resolvin receptor activation. FASEB J. 34(S1):1–1.
- Han H, Qiu FB, Zhao HF, Tang HY, Li XH, Shi DX. 2018. Dietary flaxseed oil improved western-type diet-induced atherosclerosis in apolipoprotein-E knockout mice. J Funct Foods. 40:417–425.
- Imran M, Anjum FM, Ahmad N, Khan MK, Mushtaq Z, Nadeem M, Hussain S. 2015. Impact of extrusion processing conditions on lipid peroxidation and storage stability of full-fat flaxseed meal. Lipids in Health and Disease. 14(1):1–9.
- Jacek M, Hrncirova D, Rambouskova J, Dlouhy P, Tuma P. 2020. Effect of food with low enrichment of N-3 fatty acids in a two-month diet on the fatty acid content in the plasma and erythrocytes and on cardiovascular risk markers in healthy young men. Nutrients. 12(8):2207.
- Kaab H, Bain MM, Eckersall PD. 2018. Acute phase proteins and stress markers in the immediate response to a combined vaccination against newcastle disease and infectious bronchitis viruses in specific pathogen free (SPF) layer chicks. Poult Sci. 97:463–469. Epub 2017/11/29.
- Kang S, Kim DH, Lee S, Lee T, Lee KW, Chang HH, Moon B, Ayasan T, Choi YH. 2020. An acute, rather than progressive, increase in temperature-humidity index has severe effects on mortality in laying hens. Front Vet Sci. 7:568093. doi:10.3389/fvets.2020.568093.
- Khadge S, Sharp JG, Thiele GM, McGuire TR, Klassen LW, Duryee MJ, Britton HC, Dafferner AJ, Beck J, Black PN, et al. 2018. Dietary omega-3 and omega-6 polyunsaturated fatty acids modulate hepatic pathology. J Nutr Biochem. 52:92–102. Epub 2017/11/28.
- Kortz L, Dorow J, Ceglarek U. 2014. Liquid chromatography-tandem mass spectrometry for the analysis of eicosanoids and related lipids in human biological matrices: a review. J Chromatogr B Analyt Technol Biomed Life Sci. 964:1–11. Epub 2014/03/04.
- Kumar N, Gupta G, Anilkumar K, Fatima N, Karnati R, Reddy GV, Giri PV, Reddanna P. 2016. 15-Lipoxygenase metabolites of alpha-linolenic acid, [13-(S)-HPOTrE and 13-(S)-HOTrE], mediate anti-inflammatory effects by inactivating NLRP3 inflammasome. Sci Rep. 6(1):1–14.
- Lee JW, Mok HJ, Lee DY, Park SC, Ban MS, Choi J, Park CG, Ahn YS, Kim KP, Kim HD. 2016a. UPLC-MS/MS-based profiling of eicosanoids in RAW264.7 cells treated with lipopolysaccharide. Int J Mol Sci. 17(4):508.
- Lee JY, Kang SK, Heo YJ, Shin DW, Park TE, Han GG, Jin GD, Lee HB, Jung E, Kim HS, et al. 2016b. Influence of flaxseed oil on fecal microbiota, egg quality and fatty acid composition of egg yolks in laying hens. Curr Microbiol. 72:259–266. Epub 2015/11/29.
- Lee SH, Kim YB, Kim DH, Lee DW, Lee HG, Jha R, Lee KW. 2021. Dietary soluble flaxseed oils as a source of omega-3 polyunsaturated fatty acids for laying hens. Poult Sci. 100(8):101276. doi:10.1016/j.psj.2021.101276.
- Liang P, Henning SM, Guan J, Grogan T, Elashoff D, Cohen P, Aronson WJ. 2020. Effect of dietary omega-3 fatty acids on castrate-resistant prostate cancer and tumor-associated macrophages. Prostate Cancer Prostatic Dis 23:127–135. Epub 2019/08/24.
- Liebers CJ, Schwarzer A, Erhard M, Schmidt P, Louton H. 2019. The influence of environmental enrichment and stocking density on the plumage and health conditions of laying hen pullets. Poult Sci. 98:2474–2488. Epub 2019/02/05.
- Liu R, Chen L, Wang Z, Zheng X, Hou Z, Zhao D, Long J, Liu J. 2021. Omega-3 polyunsaturated fatty acids prevent obesity by improving tricarboxylic acid cycle homeostasis. J Nutr Biochem. 88:108503. doi:10.1016/j.jnutbio.2020.108503.
- Lundstrom SL, Yang J, Brannan JD, Haeggstrom JZ, Hammock BD, Nair P, O'Byrne P, Dahlen SE, Wheelock CE. 2013. Lipid mediator serum profiles in asthmatics significantly shift following dietary supplementation with omega-3 fatty acids. Mol Nutr Food Res. 57:1378–1389.
- Mangi MH, Hussain T, Shahid MS, Sabir N, Kalhoro MS, Zhou XM, Yuan JM. 2021. Effects of flaxseed and multi-carbohydrase enzymes on the cecal microbiota and liver inflammation of laying hens. Animals-Basel. 11(3):600. doi:10.3390/ani11030600.
- Mariamenatu AH, Abdu EM. 2021. Overconsumption of omega-6 polyunsaturated fatty acids (PUFAs) versus deficiency of omega-3 PUFAs in modern-day diets: The disturbing factor for their “balanced antagonistic metabolic functions” in the human body. J Lipids. 2021:8848161. doi:10.1155/2021/8848161.
- Moallem U. 2018. Invited review: roles of dietary n-3 fatty acids in performance, milk fat composition, and reproductive and immune systems in dairy cattle. J Dairy Sci. 101:8641–8661. Epub 2018/08/14.
- Mouchlis VD, Dennis EA. 2019. Phospholipase A2 catalysis and lipid mediator lipidomics. Biochim Biophys Acta Mol Cell Biol Lipids. 1864:766–771. Epub 2019/03/25.
- Nagatake T, Shiogama Y, Inoue A, Kikuta J, Honda T, Tiwari P, Kishi T, Yanagisawa A, Isobe Y, Matsumoto N, et al. 2018. The 17,18-epoxyeicosatetraenoic acid-G protein-coupled receptor 40 axis ameliorates contact hypersensitivity by inhibiting neutrophil mobility in mice and cynomolgus macaques. J Allergy Clin Immunol. 142:470–484. e412. Epub 2017/12/31.
- Nandish SKM, Kengaiah J, Ramachandraiah C, Shivaiah A, Chandramma GK, Kemparaju K, Sannaningaiah D. 2018. Anticoagulant, antiplatele and fibrin clot hydrolyzing activities of flax seed buffer extract. Pharmacogn Mag. 14:S175–S183.
- Nejm MB, Haidar AA, Hirata AE, Oyama LM, de Almeida AG, Cysneiros RM, Cavalheiro EA, Scorza CA, Scorza FA. 2017. Fish oil supplementation reduces heart levels of interleukin-6 in rats with chronic inflammation due to epilepsy. Front Neurol. 8:263. doi:10.3389/fneur.2017.00263.
- O'Fallon JV, Busboom JR, Nelson ML, Gaskins CT. 2007. A direct method for fatty acid methyl ester synthesis: application to wet meat tissues, oils, and feedstuffs. J Anim Sci. 85:1511–1521.
- Onodera T, Fukuhara A, Shin J, Hayakawa T, Otsuki M, Shimomura I. 2017. Eicosapentaenoic acid and 5-HEPE enhance macrophage-mediated treg induction in mice. Sci Rep. 7(1):1–11.
- Prasad K. 2019. Importance of flaxseed and its components in the management of hypertension. Int J Angiol. 28:153–160. Epub 2019/08/28.
- Ren J, Chung S, Chemistry F. 2007. Anti-inflammatory effect of α-linolenic acid and its mode of action through the inhibition of nitric oxide production and inducible nitric oxide synthase gene expression via NF-κB and mitogen-activated protein kinase pathways.55:5073-5080.
- Rico DE, Gervais R, Schwebel L, Lebeuf Y, Chouinard PY. 2021. Production performance and oxidative stability of milk enriched with n-3 fatty acids in Holstein cows fed flaxseed meal. Can J Anim Sci. 101:329–341.
- Sawane K, Nagatake T, Hosomi K, Hirata S, Adachi J, Abe Y, Isoyama J, Suzuki H, Matsunaga A, Fukumitsu S, et al. 2019. Dietary omega-3 fatty acid dampens allergic rhinitis via eosinophilic production of the anti-allergic lipid mediator 15-hydroxyeicosapentaenoic acid in mice. Nutrients. 11(12):2868. doi:10.3390/nu11122868.
- Schmitz G, Ecker J. 2008. The opposing effects of n-3 and n-6 fatty acids. Prog Lipid Res. 47:147–155. Epub 2008/01/17.
- Serhan CN, Chiang N, Dalli J, Levy BD. 2015. Lipid mediators in the resolution of inflamma. Csh Perspect Biol. 7(2):a016311. doi:10.1101/cshperspect.a016311.
- Sevim B, Gümüş E, Harman H, Ayaşan T, Başer E, Altay Y, Akbulut K. 2020. Effects of dietary rosemary essential oil on growth performance, carcass traits and some hematological values of chukar partridge. Turkish JAF Sci Tech. 8(2):430–435.
- Shahidi F, Ambigaipalan P. 2018. Omega-3 polyunsaturated fatty acids and their health benefits. Annu Rev Food Sci T. 9:345–381.
- Shearer GC, Walker RE. 2018. An overview of the biologic effects of omega-6 oxylipins in humans. Prostaglandins Leukot Essent Fatty Acids. 137:26–38. Epub 2018/10/09.
- Simopoulos AP. 2016. An increase in the omega-6/omega-3 fatty acid ratio increases the risk for obesity. Nutrients 8(3):128. doi:10.3390/nu8030128.
- Subash-Babu P, Alshatwi AA. 2018. Effects of increasing ratios of dietary omega-6/omega-3 fatty acids on human monocyte immunomodulation linked with atherosclerosis. J Funct Foods. 41:258–267.
- Szczeklik K, Owczarek D, Pytko-Polonczyk J, Kesek B, Mach TH. 2012. Proinflammatory cytokines in the saliva of patients with active and non-active crohn's disease. Pol Arch Med Wewn. 122:200–208. Epub 2012/04/28.
- Tamiru B, Alkhtib A, Tamiru M, Demeke S, Burton E, Tolemariam T, Debela L, Janssens GPJ. 2021. Evaluation of dried papaya pomace meal in laying hen diets. Vet Med Sci. 7(5):1914–1920.
- Tu M, Wang W, Zhang G, Hammock BD. 2020. Omega-3 polyunsaturated fatty acids on colonic inflammation and colon cancer: roles of lipid-metabolizing enzymes involved. Nutrients. 12(11):3301. doi:10.3390/nu12113301.
- Wang CJ, Liu WL, Yao L, Zhang XJ, Zhang X, Ye CJ, Jiang HF, He JL, Zhu Y, Ai D. 2017. Hydroxyeicosapentaenoic acids and epoxyeicosatetraenoic acids attenuate early occurrence of nonalcoholic fatty liver disease. Brit J Pharmacol. 174:2358–2372.
- Wang J, Zhang C, Zhang T, Yan L, Qiu L, Yin H, Ding X, Bai S, Zeng Q, Mao X, et al. 2021. Dietary 25-hydroxyvitamin D improves intestinal health and microbiota of laying hens under high stocking density. Poult Sci. 100(7):101132. doi:10.1016/j.psj.2021.101132.
- Yang J, Oh YT, Wan D, Watanabe RM, Hammock BD, Youn JH. 2017. Postprandial effect to decrease soluble epoxide hydrolase activity: roles of insulin and gut microbiota. J Nutr Biochem. 49:8–14. Epub 2017/09/02.
- Yang P, Jiang Y, Fischer SM. 2014. Prostaglandin E3 metabolism and cancer. Cancer Lett 348:1–11. Epub 2014/03/25.
- Zachut M, Arieli A, Lehrer H, Livshitz L, Yakoby S, Moallem U. 2010. Effects of increased supplementation of n-3 fatty acids to transition dairy cows on performance and fatty acid profile in plasma, adipose tissue, and milk fat. J Dairy Sci. 93:5877–5889. Epub 2010/11/26.
- Zahradka P, Neumann S, Aukema HM, Taylor CG. 2017. Adipocyte lipid storage and adipokine production are modulated by lipoxygenase-derived oxylipins generated from 18-carbon fatty acids. Int J Biochem Cell Biol. 88:23–30. Epub 2017/05/04.
- Zarate R, el Jaber-Vazdekis N, Tejera N, Perez JA, Rodriguez C. 2017. Significance of long chain polyunsaturated fatty acids in human health. Clin Transl Med. 6(25):1–19. doi:10.1186/s40169-017-0153-6.
- Zhu L, Sha L, Li K, Wang Z, Wang T, Li Y, Liu P, Dong X, Dong Y, Zhang X, et al. 2020. Dietary flaxseed oil rich in omega-3 suppresses severity of type 2 diabetes mellitus via anti-inflammation and modulating gut microbiota in rats. Lipids Health Dis 19(20):1–16. doi:10.1186/s12944-019-1167-4.