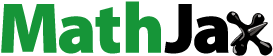
Abstract
Most chronic stress protocols are too laborious or do not abide by the two main characteristics of the stress concept: uncontrollability and unpredictability. The goal of this study was to establish a simple and reliable model of chronic stress, while maintaining the main features of the concept. Animals were exposed to chronic movement restraint with variable duration (2, 4 or 6 h, in an unpredictable schedule) for 3 weeks and assessed in several physiological and behavioral readouts known to reflect chronic stress states. Body weight, levels of plasma corticosterone, hippocampal pro-and anti-inflammatory cytokines, anxiety-like (novelty suppressed feeding and elevated plus maze) and motivated behaviors (sucrose negative contrast test and forced swim test) were evaluated three days after the end of the chronic protocol. Stressed animals had a lower body weight gain, higher levels of cytokines in the hippocampus, reduced suppression of a low concentration sucrose solution and increased immobility in the forced swim test. Based on these data, we suggest that chronic movement restraint with variable duration may be a suitable and simple protocol for the study of changes induced by chronic stress and for the testing of possible treatments relevant to psychiatry.
Introduction
Animal models are essential for the study of pathophysiological changes and the development of new treatments for chronic stress-associated conditions. The stress concept includes two main features: uncontrollability and unpredictability (Koolhaas et al., Citation2011). Currently, one of the most commonly used stress paradigms involves exposure to chronic unpredictable mild stress (CUMS) (Bambico et al., Citation2015; Hamani, Machado, et al., Citation2012; Willner, Citation1997). This model, however, presents disadvantages, including its laborious application (Yan, Cao, Das, Zhu, & Gao, Citation2010) and the demand for a proper experimental setting (Yin, Guven, & Dietis, Citation2016). Moreover, the sequence and length of stressors add a complexity that makes replication a challenge among different groups (Slattery & Cryan, Citation2017). Reproducibility is sometimes a problem, even when experiments are conducted by different investigators in the same laboratory (Lezak, Missig, & Carlezon, Citation2017; Yan et al., Citation2010). Finally, the establishment of a stable baseline for sucrose preference after stress makes this protocol excessively long, often lasting several weeks. This ultimately creates barriers for ethical approval and makes the model somewhat cumbersome from a practical perspective (Yan et al., Citation2010; Yin et al., Citation2016).
Another commonly used chronic stress protocol is social defeat, which may be run intermittently or continuously (e.g., the defeated animal returns to its home-cage after each session or stays in the resident home-cage separated by a barrier) (Vasconcelos, Stein, & de Almeida, Citation2015). In a recent publication, we showed that levels of corticosterone (CORT) were 80 times higher after the first defeat compared to baseline. This value, however, was reduced by half after the 10th defeat session, indicating a clear habituation (Macedo et al., Citation2018). Chronic movement restraint is a simple, widely used and easily replicable stress paradigm (Harris, Citation2015). Though it is uncontrollable, most studies employing different lengths and periods show the development of habituation (for review, see (Grissom & Bhatnagar, Citation2009)). Previous studies have demonstrated that rats submitted to 3 weeks of movement restraint for 6 h not only develop anxiety- and depressive-like behaviors but also present morphological brain changes that emulate those observed in chronically stressed and depressed patients (Bloss, Janssen, McEwen, & Morrison, Citation2010; Galea et al., Citation1997). Male rats exposed to 6 h of restraint daily display lower levels of CORT after 14 and 21 days compared to the first stress session, also indicating habituation (Galea et al., Citation1997).
Chronic stress activates the hypothalamic-pituitary-adrenal (HPA) axis (for review, see (de Kloet, Joëls, & Holsboer, Citation2005)), increases glucocorticoid concentrations, and activates microglia in several brain areas (Réus et al., Citation2015; Tynan et al., Citation2010). It is associated with the release of inflammatory mediators, such as prostaglandins, cytokines and chemokines, which further potentiate the activity of the HPA axis, particularly through the release of interleukin 1β (IL-1β) into the hypothalamus (Wohleb, McKim, Sheridan, & Godbout, Citation2014). Furthermore, increased levels of IL-α in the ventral hippocampus have been associated with the development of stress vulnerability in rats, with concomitant increases in ACTH and CORT plasma levels, and decreased social interaction following repeated social defeat (Pearson-Leary, Eacret, & Bhatnagar, Citation2019).
In the present study, we tested a modified chronic restraint paradigm by exposing the animals to variable lengths of stress, so the model would maintain the main characteristics of the stress concept: uncontrollability and unpredictability. To validate this protocol, animals were assessed for some of the physiological and behavioral endpoints that best represent exposure to chronic stress.
Materials and methods
This study was conducted in two experiments approved by the Ethics Committee on Animal Use (CEUA) of the Universidade Federal de São Paulo, following ethical standards of the National Council for Control of Animal Experimentation (CONCEA) under protocol number 4384140316.
Animals
A total of 87, 8 weeks-old, male Wistar rats were used. The animals weighed 250–280 g upon arrival and were allowed to acclimate to the animal facility for 1 week before the beginning of the experiments. They were single housed for the entire period with food and water provided ad libitum under a 12/12 h light/dark cycle (lights on at 7:00 h) and controlled room temperature (23 ± 1 °C).
Experimental design
Two experiments were conducted with the animals being divided in two groups: Experiment 1 – Control (CTL, n = 10) and Chronic Unpredictable Movement Restraint (CUMR, n = 18); Experiment 2 – CTL (n = 26) and CUMR (n = 33). The sample size in Experiment 2 was made up from three replications. All animals were weighed in the beginning of the experiment and once a week throughout the protocol ().
Chronic stress protocol with movement restraint of variable duration
Upon arrival in the animal facility rats were allowed 1 week of habituation to the experimenter, during which they were handled daily. Thereafter, stressed animals were placed in a plastic tube with 6.5 cm in diameter and 21 cm in length, with a guillotine door on one end and wire mesh for ventilation on the other. Every day the stress session began between 08:00 and 09:00 a.m.
Rats were kept inside the restrainer for 2, 4 or 6 h/day during 3 weeks (), except for weekends. The duration of each stress session was randomly established, except for Fridays, when the animals were restrained for 2 h, and weighted afterwards. This ensured that all animals were submitted to the same stress duration by the end of the protocol. All sampling procedures and behavioral testing started 72 h after the end of the protocol, so as to avoid residual effects of restraint and to determine whether our modified protocol could produce enduring effects (e.g., if the changes would persist beyond the end of the chronic stress).
Table 1. Schedule of chronic stress by movement restraint with variable duration.
Experiment 1
Seventy-two hours after the end of CUMR, animals were decapitated. Trunk blood was collected, brains were removed and maintained in the ultra-freezer (at −80 °C) for later analysis. Blood samples were collected in 1.5 mL plastic tubes containing EDTA (7.5%) and centrifuged at 1209 g for 20 min at 4 °C. Plasma was collected, frozen and stored at −20 °C for later analysis of corticosterone (CORT) levels.
CORT plasma levels
Samples were taken from the freezer 24 h prior to quantification of hormone levels. After thawing, 100 µL of each plasma sample and standard curve samples (0, 10, 25, 50,100, 250, 500 and 1000 ng/mL of corticosterone – Sigma-Aldrich) were transferred to borosilicate glass tubes and added 50 µL of internal standard (cortisol – Sigma-Aldrich – 10 µg/mL in MeOH:H2O, 1:1, v/v + 0.1% formic acid) and 100 µL of protein precipitating ZnSO4 (1 M). The tubes were vortexed for 30 s and 1 mL of diethyl alcohol was added to each tube. Samples were homogenized for 60 s and centrifuged for 5 min. Thereafter, they were frozen for 40 min and the supernatant transferred to another borosilicate glass tube. Samples were then evaporated in the sample concentrator with compressed nitrogen for 20 min, resuspended with 100 µL of MeOH:H2O, 1:1, v/v + 0.1% of formic acid, vortexed for 10 s, transferred to vials and sealed (Cabbia, Consoli, & Suchecki, Citation2018). CORT levels were determined in duplicate by high-performance liquid chromatography (HPLC – Shimadzu – Class VP) followed by mass spectrometry (MS – Waters – Quattro Micro), using Phenomenex – Kinectex column (50 mm × 2.1 mm × 2.7 mm). The mobile phase consisted of MeOH:H2O (70:30) and was delivered at a flow rate of 0.15 mL/min (Li et al., Citation2014; Samtani & Jusko, Citation2007).
Hippocampal cytokines levels
The hippocampi were dissected, weighed and homogenized in 0.01 M Tris-hydrochloride solution (pH 7.6) containing 5.8% sodium chlorine, 10% glycerol, 1% Nonidet P 40 (NP-40), 0.4% ethylenediamine tetraacetic acid (EDTA) and protease (cat# M222-1 mL; lot# 1295C056; Amresco) and phosphatase inhibitors (cat# B15001-A e B; lot# 510011; Biotool). This dilution was performed in a 3:1 ratio.
MILLIPEX® MAP Rat Cytokine/Chemokine Magnetic Bead Panel (cat# RECYMAG-65K; lot# 2854609) kit and MAGPIX® equipment were used to quantify IL-1α, IL-1β, IL-2, IL-6, IL-10 and TNF-α levels.
A 96-well plate was sensitized with 200 µL of the buffer solution supplied by the manufacturer under agitation for 10 min at room temperature between 20 and 25 °C. Subsequently, 25 µL of standard solution, 25 µL of buffer solution, 25 µL of the solution containing the cytokines beads and 25 µL of sample solution (hippocampal homogenized solution) were added to each well. The plates were incubated at 4 °C under agitation overnight and washed twice. Detection antibodies (25 µL) were added and incubated for 1 h, following which 25 µL of Streptavidin-Phycoerythrin were added and incubated for another 30 min under agitation at room temperature. The plate was again washed twice and 125 µL of Drive fluid was added under agitation for 5 min and the plates were placed in the MAGIPIX® equipment and red by Luminex technology.
After this procedure, total protein levels were determined with the Bradford method for normalization of cytokines levels. Homogenates were diluted in distilled water in a proportion of 1:1000. The standard curve was prepared using albumin at concentrations of 0, 5, 10, 20, 40, 60, 80 and 100 µg/mL. Thereafter 10 µL of standard and sample solutions were placed on a plate with 250 µL of the Bradford solution (Bio-Rad). The Biotek reader was used to read at the 595 nm wavelength, with the blank reading being subtracted from the standard curves and samples. For linear regression, absorption values of the standard curve were used. Calibration curve interpolation and dilution correction were performed in order to obtain total protein concentrations.
Experiment 2
Behavioral tests
Testing started 72 h after the end of the CUMR protocol and lasted 7 days; animals were subsequently tested in the sucrose negative contrast test, novelty suppressed feeding, elevated plus maze and forced swim test with a 24-h interval between tests (). Behavioral tests and neurochemical assays were conducted in different groups of animals so that the tests would not interfere with the analyses of biological samples.
Sucrose negative contrast test (SNCT)
This test was performed during three consecutive days (between 3 and 6 days after the end of the stress protocol). On the first and second days of testing, one bottle containing 200 mL of water and the other 200 mL of a 15% sucrose solution were offered to the animals. On the third day, the sucrose solution was changed to 2.1% (da Silva Rocha-Lopes, Machado, & Suchecki, Citation2018; Verma, Hellemans, Choi, Yu, & Weinberg, Citation2010). The bottles of sucrose solution and water were alternated daily to avoid place preference bias. The bottles were weighed before and 24 h after being offered to the animals to estimate the volume. This protocol does not require food or water deprivation. It is expected that animals reduce the 2.1% solution intake (lower palatability), compared to the 15% solution.
Sucrose preference was calculated every day as a ratio between the volumes of consumed sucrose and liquid.
Novelty suppressed feeding test (NSF)
Immediately after the SNCT animals were fasted for 24 h (Bodnoff, Suranyi-Cadotte, Aitken, Quirion, & Meaney, Citation1988) in order to increase their motivation to eat, following a previously used protocol (Bambico et al., Citation2015; Citation2018). The NSF was performed 7 days after the end of the CUMR (1 day after SNCT) in a 30 × 50 cm cage with 20 cm high walls. In the center of the box there was a 2 cm high, 8 cm × 8 cm platform, where a pellet of food was placed. The room was maintained brightly lit (250 lux) and each rat was placed on one corner of the box and allowed to free exploration. The latency for the animals to bite the pellet was recorded. If the animal did not eat during the test, a maximum duration of 600 s was attributed.
Elevated plus maze test (EPM)
On the following day, 8 days after the end of CUMR, each animal was individually evaluated for 5 min in a wooden apparatus with four cross-shaped arms (two open and two enclosed by 40 cm-high walls), 50 cm long, 10 cm wide and elevated 60 cm above the ground; each arm was divided into three segments. Initially, the rat was placed in the 10 cm × 10 cm center square facing one of the open arms. The session was conducted under low lighting (10 lux) and controlled room temperature (22 ± 2 °C). The following parameters were evaluated: entries in open (EOA) and closed arms (ECA): the animal’s entry into an arm was considered only when it entered with all four paws in the segment; time spent in open (TOA) and closed arms (TCA) and segments crossed (each arm was divided into three segments, SOA and SCA). For each parameter, results were transformed in percentage of exploration of the open arms, using the equation % = OA/(OA + CA).
Forced swim test (FST)
The animals underwent two consecutive days of swimming in a cylinder filled with water (at approximately 25 °C) at a level that prevented them from touching the bottom with their tails or reaching the upper border (40 cm). On the first day of testing, they were placed in the water for 15 min (training session). During the second testing day, i.e., 10 days after the end of CUMR, animals were placed in the water for 5 min. The frequency of immobility, swimming or climbing was registered at every 5 s, in a total of 60 observations, as previously described (Hamani et al., Citation2014; Hamani, Giacobbe, et al., Citation2012; Miragaia et al., Citation2018).
Statistical analysis
Weekly variation of body weight was calculated with the equation [(final weight – initial weight)*100] – 100. Statistical analysis of weekly variations in body weight and the sucrose preference was done with repeated measures two-way ANOVA with Group (CTL, Stress) and Time as main factors (Week for body weight gain and Day for sucrose preference), followed by unprotected Newman-Keuls post-hoc analysis. Group comparisons for all other parameters were performed with the Student’s-t test. Pearson’s correlation tests were carried out to correlate levels of hippocampal cytokines. A significance level of p ≤ 5% was considered for all tests.
Results
Experiment 1
Variation of body weight gain
There were main effects of Group (F(1,26) = 19.608; p ≤ .0002), Time (F(2,52) = 435.886; p ≤ .00001) and an interaction between Group × Time (F(2,52) = 9.924; p < .0003). While animals in both groups had an increase in weight over time, stressed animals gained significantly less weight than controls during all weeks (p’s < .005; ).
Figure 2. Chronic stress decreased body weight gain in Experiments 1 (A) and 2 (B). Experiment 1 included 10 CTL and 18 CUMR rats. Experiment 2 included 26 CTL and 33 CUMR animals. *Difference from controls, p < 0.001; #difference from the first week, p < 0.001; ‡difference from the second week, p < 0.01 (two-way ANOVA repeated measures). Values are shown as mean ± s.e.m.
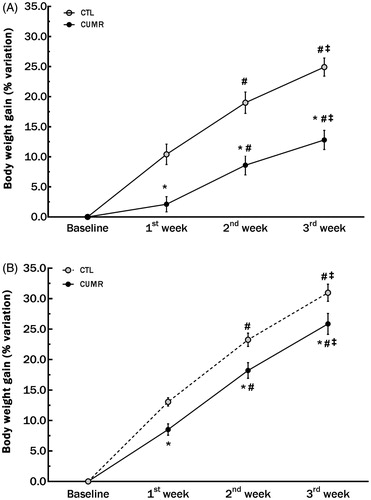
Neurobiological factors
Chronic stress was associated with increased levels of almost all measured hippocampal cytokines compared to controls (), except for IL-1α and TNF-α (nearly significant p < .09). These included IL-1β (t(26) = 3.002; p < .005), IL-2 (t(26) = 2.593; p < .02), IL-6 (t(26) = 2.093; p < .05), IL-10 (t(26) = 3.112; p = .005), results that were obtained after samples were normalized for protein content, which was lower in CUMR animals compared to CTL (CTL = 48.50 ± 14.01 × CUMR = 37.00 ± 6.02; t(26) = 3.04; p < .01).
Figure 3. Chronic stress increased pro-inflammatory cytokine levels (normalized by total protein level in the tissue) in the hippocampus. (A) IL-1α; p < 0.2; (B) IL-1β; p = 0.003; (C) IL-2; p < 0.02; (D) IL-6; p < 0.03; (E) IL-10; p = 0.002; (F) TNF-α; p < 0.09. Values are shown as mean ± s.e.m. of 10 CTL and 18 CUMR rats. *Difference from CTL.
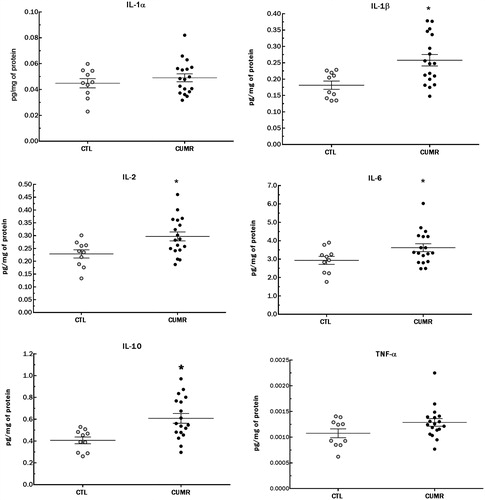
As shown in , there were moderate and strong positive correlations between all cytokines (r = 0.63–0.97; p’s < .001).
Table 2. Correlation matrix between hippocampal cytokines.
There was no group difference in CORT levels 72 h after the end of chronic stress protocol (t(23) = 1.183; p < .3; ).
Table 3. Results of corticosterone (CORT) levels, novelty suppressed feeding (NSF) and elevated plus maze (EPM) tests.
Experiment 2
Variation of body weight gain
In this experiment we found a significant Time effect [F(2,114) = 542.351; p < .00001], with all animals gaining weight compared to the previous week (p’s < .0002). We also found a significant Group effect [F(1,57) = 8.17; p < .006). Unprotected Newman-Keuls test showed that CUMR animals gained less weight than CTL in all time-points (p’s < .02; ).
Because the magnitude of the differences in weight gain between CTL and CUMR groups was not the same in Experiments 1 and 2, a collapsed analysis was conducted across experiments. We found a main effect of Group [F(1,170) = 19.933; p < .00005], with stressed animals gaining less weight than CTLs (p’s < .001 in all weeks), and main effect of Time [F(2,170) = 669.698; p < .000005], with a progressive increase along the 3 weeks of stress (p’s < .0001). There was a nearly significant interaction between these factors [F2,170) = 2.57; p < .08].
Behavioral tests
In the SNCT, main effects of Group [F1,57) = 4,301; p < .05] and Time [F(2,114) = 7.152; p < .002] were found. An unprotected post-hoc analysis revealed that only CTL animals had a reduction in preference for the sucrose solution on day 3 (2.1%) compared to preference on day 2 (15% solution; p < .002), as well as to the preference of CUMR rats on day 3 (p < .002). These results are shown in .
Figure 4. Chronic stress did not reduced sucrose preference for the less palatable solution rats and increased the frequency of immobility and reduced the frequency of swimming of CUMR animals in the forced swim test. (A) Sucrose preference in the Sucrose Negative Contrast Test; (B) behaviors in the forced swim test. Values are shown as mean ± s.e.m. of n = 26 CTL and 33 CUMR rats. *Difference from CTL, p < 0.001 (Student’s t test); ‡difference from day 2 (two-way ANOVA repeated measures); p = 0.003.
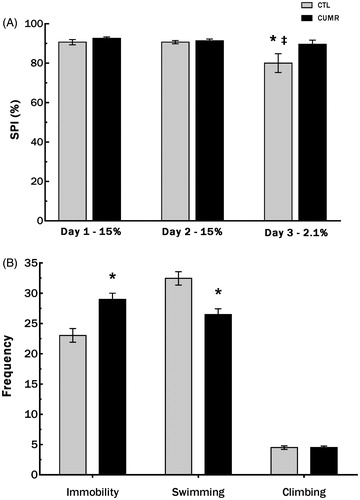
No differences between groups were observed in the NSF (latency to the first bite, t(26) 1.07, p < .3; ) or in the EPM when CTL and CUMR animals were compared (% entries in open arms t(57) = 0.39, p < .70); % of time in open arms t(57) = 0.37, p < .8; % of segments crossed in open arms t(57) = 0.04; p < 1.00; ).
In the FST, CUMR animals showed lower swimming (t(57) = 4.117; p < .001) and greater immobility frequency (t(57) = 3.884; p < .0003), with no differences in climbing, compared to CTL (t(57) = 0.04; p = 1.00; ).
Discussion
In the present study, we showed that chronic stress induced by movement restraint of variable duration reduced body weight gain, increased hippocampal cytokine levels, and altered motivated behaviors, as assessed in the SNCT and FST. However, no changes were observed in CORT plasma levels and anxiety-like behavior. As a matter of clarification, the main purpose of the study was not to compare different models of chronic stress. The variable length of restraint added the unpredictability necessary to fulfill the main characteristics of the stress definition (Koolhaas et al., Citation2011). We believe that the present protocol has the advantage of combining technical simplicity with unpredictability and uncontrollability.
Physiological alterations, such as reduction in body weight gain and increased relative weight of the adrenal glands are indicative of chronic stress. These effects are commonly seen after exposure to different types of chronic stress, including subordinated rats in the visible burrow system (Tamashiro et al., Citation2007), chronic rapid eye movement sleep restriction (da Silva Rocha-Lopes et al., Citation2018), chronic unpredictable mild stress (Riaz et al., Citation2015), social defeat (Macedo et al., Citation2018) and repeated restraint stress (Chiba et al., Citation2012; Chotiwat, Kelso, & Harris, Citation2010). In the comprehensive review paper by Koolhaas and colleagues (Citation2011), chronic movement restraint during the active, but not the resting period, was shown to reduce body weight gain. These results, however, were presented in the context of a review, and methodological details, such as the length of the sessions and the intensity of the stressor (sometimes called restraint and other times called immobilization) were not presented. Therefore, the discrepancy between these and our results may lie on methodological differences. In the current study, stress was applied in the light phase resulting in less body weight gain than in CTL rats. This suggests that daily variations in restraint duration may have prevented habituation, indicating that this could be a good model for the evaluation of changes induced by chronic unpredictable stress. We note that the magnitude of variation in body weight gain was not the same in our two experiments, although CUMR animals always gained less weight than CTLs. We cannot pinpoint a clear reason to explain these findings, since both experiments were conducted exactly in the same way. Global statistical analyses considering all animals (pooled across experiments) confirmed the lesser weight gain in CUMR compared to CTL rats. In regards to the relative weight of the adrenal glands, we have observed in a different study (unpublished data) that the present protocol resulted in heavier adrenals compared to controls.
Another widely used way to evaluate the intensity of stress is the measurement of plasma CORT levels. This parameter can be assessed in the absence (basal) or in response to a challenge (stress response). Results of such measures, however, should be interpreted with caution, since the stress response depends on perceived predictability and controllability of the stimulus, which shows individual variability even within the same species (Koolhaas et al., Citation2011). Though augmented CORT levels in response to acute stress have been reported (Calvo & Volosin, Citation2001; Grissom & Bhatnagar, Citation2009), chronic stress may not always lead to similar findings. Repetition of homotypic stimuli may prepare the organism to anticipate the expected events, leading to a habituation of the CORT response (reviewed in Grissom & Bhatnagar, Citation2009; Koolhaas et al., Citation2011). The introduction of an unexpected increase in the length of restraint stress may restore the CORT response to the level seen after the first exposure (Kearns & Spencer, Citation2013). These results, however, are still disputed, as one study showed that chronic restraint stress for 2 h or 6 h/day for 4, 12 or 20 days elicited an increase in CORT levels when applied during the light, but not during the dark phase (Retana-Márquez et al., Citation2003). The similarity in CORT levels between groups in the present study may be explained by the fact that basal unstimulated blood sampling took place 72 h after the end of the protocol, possibly allowing the recovery of hormonal levels. Still, CORT levels of CUMR animals were approximately 40% above those of CTLs. In future studies it will be important to evaluate the hormone response of these animals to a heterotypic stressor.
Increased plasma CORT levels induced by acute stress seem to be related to emotional disorders, such as anxiety-like behaviors (Calvo & Volosin, Citation2001). The lack of augmented CORT as seen in the present study may be somehow associated with the normal performance of CUMR animals in the NSF and EPM. These are widely used tests to measure anxiety-like behavior, which is known to be influenced by previous exposure to stress (Barsy, Leveleki, Zelena, & Haller, Citation2010; Chiba et al., Citation2012). It is important to mention that the NSF protocol, although often used by our group (Bambico et al., Citation2015; Cabbia et al., Citation2018; Miragaia et al., Citation2018), imposed a long period of fasting (24 to 48 h) to the animals. Whether this prolonged fasting interferes with the responses observed with other testing protocols needs to be addressed in the future. The lack of behavioral effects of chronic stress on the elevated plus maze might have been due to the interval between the end of the protocol and the execution of the test (8 days). Nonetheless, in agreement with our results, a previous study showed that acute restraint stress reduced exploratory behavior in the EPM, whereas chronic exposure to the same stimulus completely eliminated this effect (Padovan & Guimarães, Citation2000).
It has been suggested that inflammation can be a link between stress and depression (for review, see (Jaremka, Lindgren, & Kiecolt-Glaser, Citation2013)). We found significantly higher levels of pro-inflammatory cytokines, as well as the anti-inflammatory cytokine IL-10, in the hippocampus of CUMR animals. Studies have previously demonstrated high levels of pro-inflammatory cytokines both in the plasma and in various brain regions after exposure to different types of stress, including crowding (Gądek-Michalska, Tadeusz, Rachwalska, & Bugajski, Citation2016), foot shock (Han et al., Citation2015), immobilization (Oh, Kim, Choi, Kim, & Kim, Citation2015), CUMS (Dong et al., Citation2017) and restraint stress (Tan, Wang, Chen, Long, & Zou, Citation2017). The same cytokines found to be elevated in our study were also increased in medication-free depressed patients; 12 weeks after antidepressant treatment plasma cytokine levels were reduced, except for IL-2, which was undetectable in more than 50% of the patients (Dahl et al., Citation2014). Because depressive disorders are associated with broad changes in pro- and anti-inflammatory cytokines (Dahl et al., Citation2014; Ménard, Pfau, Hodes, & Russo, Citation2017), we performed pairwise correlation tests between cytokines and found highly significant positive correlations. This suggests that the proposed chronic stress model leads to a general recruitment of microglia cells, which are responsive to various different stressors, including chronic restraint (Calcia et al., Citation2016). These results are in line with a recent study reporting the induction of depressive-like behavior, microglial activation and increased hippocampal levels of both pro- (IL-1β, IL-6 and IL-18) and anti-inflammatory (IL-4 and IL-10) cytokines after 12 weeks of chronic unpredictable stress (Wang et al., Citation2018).
Reduced body weight gain seen in CUMR animals may be related to increased IL-6 levels, a cytokine involved in metabolic activity and mechanisms of anorexia (Swiergiel & Dunn, Citation2006). The increase in hippocampal pro-inflammatory cytokines and impairment in motivated behaviors observed in our study strengthens the evidence that stress-induced neuroinflammation is likely related to depressive-like behavior (Jaremka et al., Citation2013; Tan et al., Citation2017). Studies in depressive patients show controversial results regarding IL-10 levels (Ménard et al., Citation2017), with increased (Dahl et al., Citation2014; Simon et al., Citation2008) and decreased plasma levels in slow medication responders (Fornaro, Rocchi, Escelsior, Contini, & Martino, Citation2013). In the present study, we found elevated levels of IL-10 in the hippocampus. Overall, an increase in anti-inflammatory cytokines has been proposed to be a compensatory alteration to a generalized inflammatory state (Simon et al., Citation2008). Mechanisms responsible for this effect remain controversial and are yet to be fully elucidated (Han et al., Citation2015; Voorhees et al., Citation2013). It is speculated that this cytokine penetrates the brain through a dysfunctional blood-brain barrier in an attempt to mitigate stress-induced immune imbalance (Banks, Citation2005). IL-10 interacts with the microglia M2 phenotype and improves coping response to stress and vulnerability to other stressors (Han et al., Citation2015).
In the present study, we found a slight but significant reduction in the intake of the less palatable solution only in the CTL group. This means that animals noticed a change in palatability that did not happen in CUMRs. Most studies use the sucrose preference test to assess anhedonic-type behavior. We chose to use the SNCT instead because it is well-established in our laboratory and does not require previous training or fasting. We note, however, that the results of this test in the literature are quite controversial. Its rationale is that after two days of drinking a 15% sucrose solution, hedonic animals suppress (or reduce) the intake when exposed to a 2.1% solution. In our studies, we have found that both CTL and stressed animals exhibit a reduction of intake of the 2.1% solution, with controls showing responses of a greater magnitude (Cabbia et al., Citation2018; da Silva Rocha-Lopes et al., Citation2018; Girardi, Zanta, & Suchecki, Citation2014; Miragaia et al., Citation2018).
In the FST there was a significant difference between groups, with CUMR animals displaying increased immobility and decreased swimming without changes in climbing. The interpretation of FST results is debatable and this test is subject to several influencing biological factors, such as age, sex, animal strain, and pretest conditions, such as exposure to stressors, housing and diet (Bogdanova, Kanekar, D’Anci, & Renshaw, Citation2013). On one hand, it has been claimed to be a useful screener for anti-depressant drugs (Slattery & Cryan, Citation2012). On the other hand, some groups have used it as a model of depressive-like behavior, associating greater immobility with behavioral despair (Ferraz et al., Citation2011; Porsolt, Bertin, & Jalfre, Citation1977). Currently, a different interpretation has been presented, stating that different behaviors displayed in this test may reflect a coping strategy when the animal is faced with an inescapable stressor (de Kloet & Molendijk, Citation2016; Molendijk & de Kloet, Citation2015). Regardless of the interpretation, our results indicate that chronic restraint stress alters the behavioral response in this test, facilitating the occurrence of immobility. This could reflect an increase in behavioral despair or a shift from an active to a passive stress coping style. Because the test was carried out seven days after the end of chronic stress, we can affirm that this effect was not due to the previous stress session, but likely related to changes in neurochemical systems that modulate this behavior. One such change is the increase in pro-inflammatory cytokines in the hippocampus, since it has been reported that this neurobiological feature is associated with increased immobility in the FST (Tan et al., Citation2017). Interestingly, some studies show that an effective reduction of pro-inflammatory cytokine levels increases active behaviors in the FST (Fernandes & Gupta, Citation2019; Krügel, Fischer, Radicke, Sack, & Himmerich, Citation2013; Tan et al., Citation2017), although this outcome is not consensual (Deak et al., Citation2005).
It shall be noted that the present stress protocol involves single housing, which could be considered an additional stress to the animals, thus leading to altered emotional/affective behavior. However, a previous study demonstrated that 7 weeks of single housing may be necessary to induce anhedonia in male rats (Sarkar & Kabbaj, Citation2016). Another point worth discussing is the number of tests and the long testing period in Experiment 2, inasmuch as behaviors were evaluated throughout a 7-day interval between the first and last tests. Consecutive testing could have produced an accumulation of challenges that might have interfered with the animals’ behavioral response. Nonetheless, only the behaviors assessed in the first and last evaluations were altered by the chronic stress protocol, both related to motivated behaviors. In addition, the CTL group was submitted to the same behavioral procedures. If the sequence of tests was to influence the animals’ behaviors, there should have been a progressive impairment in both groups, which was not the case.
Different stress paradigms result in cytokine changes in the hippocampus and hypothalamus (as discussed above). In addition, commonly reported findings in chronic stress studies are a decrease in neurogenesis, BDNF and microglial activation (Bambico et al., Citation2015; Bisht, Sharma, & Tremblay, Citation2018; Gould, McEwen, Tanapat, Galea, & Fuchs, Citation1997; Hamani, Machado, et al., Citation2012; Surget et al., Citation2011). Future studies will have to be conducted using our proposed paradigm to ascertain that similar changes are observed, further strengthening its construct validity.
Conclusion
In this study we elaborated a chronic stress model that has a few advantages over previously published paradigms, namely uncontrollability and unpredictability. In addition, its implementation was not as complex as CUMS with animals showing reliable signs of stress by the end of 3 weeks. Physiological and behavioral indices that validate this protocol included a decrease in motivated behavior, increased levels of hippocampal cytokines and a significant decrease in weight gain over time. These results show that chronic restraint stress with variable duration may be a useful tool for studying chronic stress-induced changes.
Acknowledgements
The authors thank Marcos Vinicius Bunscheit for his technical help.
Disclosure statement
No potential conflict of interest was reported by the authors.
Additional information
Funding
Notes on contributors
Karla De Michelis Mograbi
K.M.M. conducted the experiments and statistical analysis and wrote the first draft of the manuscript, D.S. and C.H. proposed the experimental design, supervised data collection, run the statistical analysis, discussed data interpretation and wrote the manuscript, S.G.S. determined the cytokines levels and L.C. proposed the experimental design and supervised the work.
Deborah Suchecki
K.M.M. conducted the experiments and statistical analysis and wrote the first draft of the manuscript, D.S. and C.H. proposed the experimental design, supervised data collection, run the statistical analysis, discussed data interpretation and wrote the manuscript, S.G.S. determined the cytokines levels and L.C. proposed the experimental design and supervised the work.
Sérgio Gomes da Silva
K.M.M. conducted the experiments and statistical analysis and wrote the first draft of the manuscript, D.S. and C.H. proposed the experimental design, supervised data collection, run the statistical analysis, discussed data interpretation and wrote the manuscript, S.G.S. determined the cytokines levels and L.C. proposed the experimental design and supervised the work.
Luciene Covolan
K.M.M. conducted the experiments and statistical analysis and wrote the first draft of the manuscript, D.S. and C.H. proposed the experimental design, supervised data collection, run the statistical analysis, discussed data interpretation and wrote the manuscript, S.G.S. determined the cytokines levels and L.C. proposed the experimental design and supervised the work.
Clement Hamani
K.M.M. conducted the experiments and statistical analysis and wrote the first draft of the manuscript, D.S. and C.H. proposed the experimental design, supervised data collection, run the statistical analysis, discussed data interpretation and wrote the manuscript, S.G.S. determined the cytokines levels and L.C. proposed the experimental design and supervised the work.
References
- Bambico, F.R., Bregman, T., Diwan, M., Li, J., Darvish-Ghane, S., Li, Z., … Hamani, C. (2015). Neuroplasticity-dependent and -independent mechanisms of chronic deep brain stimulation in stressed rats. Translational Psychiatry, 5, e674–e674. doi:10.1038/tp.2015.166
- Bambico, F.R., Comai, S., Diwan, M., Hasan, S.M.N., Conway, J.D., Darvish-Ghane, S., … Nobrega, J.N. (2018). High frequency stimulation of the anterior vermis modulates behavioural response to chronic stress: Involvement of the prefrontal cortex and dorsal raphe? Neurobiology of Disease, 116, 166–178. doi:10.1016/j.nbd.2018.03.011
- Banks, W.A. (2005). Blood-brain barrier transport of cytokines: A mechanism for neuropathology. Current Pharmaceutical Design, 11, 973–984. doi:10.2174/1381612053381684
- Barsy, B., Leveleki, C., Zelena, D., & Haller, J. (2010). The context specificity of anxiety responses induced by chronic psychosocial stress in rats: A shift from anxiety to social phobia? Stress, 13, 230–237. doi:10.3109/10253890903296389
- Bisht, K., Sharma, K., & Tremblay, M. (2018). Chronic stress as a risk factor for Alzheimer’s disease: Roles of microglia-mediated synaptic remodeling, inflammation, and oxidative stress. Neurobiology of Stress, 9, 9–21. doi:10.1016/j.ynstr.2018.05.003
- Bloss, E.B., Janssen, W.G., McEwen, B.S., & Morrison, J.H. (2010). Interactive effects of stress and aging on structural plasticity in the prefrontal cortex. Journal of Neuroscience, 30, 6726–6731. doi:10.1523/JNEUROSCI.0759-10.2010
- Bodnoff, S.R., Suranyi-Cadotte, B., Aitken, D.H., Quirion, R., & Meaney, M.J. (1988). The effects of chronic antidepressant treatment in an animal model of anxiety. Psychopharmacology, 95, 298–302. doi:10.1007/BF00181937
- Bogdanova, O.V., Kanekar, S., D’Anci, K.E., & Renshaw, P.F. (2013). Factors influencing behavior in the forced swim test. Physiology & Behavior, 118, 227–239. doi:10.1016/j.physbeh.2013.05.012
- Cabbia, R., Consoli, A., & Suchecki, D. (2018). Association of 24 h maternal deprivation with a saline injection in the neonatal period alters adult stress response and brain monoamines in a sex-dependent fashion. Stress, 21, 333–346. doi:10.1080/10253890.2018.1456525
- Calcia, M.A., Bonsall, D.R., Bloomfield, P.S., Selvaraj, S., Barichello, T., & Howes, O.D. (2016). Stress and neuroinflammation: A systematic review of the effects of stress on microglia and the implications for mental illness. Psychopharmacology (Berl), 233, 1637–1650. doi: 10.1007/s00213-016-4218-9
- Calvo, N., & Volosin, M. (2001). Glucocorticoid and mineralocorticoid receptors are involved in the facilitation of anxiety-like response induced by restraint. Neuroendocrinology, 73, 261–271. doi:10.1159/000054643
- Chiba, S., Numakawa, T., Ninomiya, M., Richards, M.C., Wakabayashi, C., & Kunugi, H. (2012). Chronic restraint stress causes anxiety- and depression-like behaviors, downregulates glucocorticoid receptor expression, and attenuates glutamate release induced by brain-derived neurotrophic factor in the prefrontal cortex. Progress in Neuro-Psychopharmacology and Biological Psychiatry, 39, 112–119. doi:10.1016/j.pnpbp.2012.05.018
- Chotiwat, C., Kelso, E.W., & Harris, R.B. (2010). The effects of repeated restraint stress on energy balance and behavior of mice with selective deletion of CRF receptors. Stress, 13, 203–213. doi:10.3109/10253890903207527
- da Silva Rocha-Lopes, J., Machado, R.B., & Suchecki, D. (2018). Chronic REM sleep restriction in juvenile male rats induces anxiety-like behavior and alters monoamine systems in the amygdala and hippocampus. Molecular Neurobiology, 55, 2884–2896. doi:10.1007/s12035-017-0541-3
- Dahl, J., Ormstad, H., Aass, H.C., Malt, U.F., Bendz, L.T., Sandvik, L., … Andreassen, O.A. (2014). The plasma levels of various cytokines are increased during ongoing depression and are reduced to normal levels after recovery. Psychoneuroendocrinology, 45, 77–86. doi:10.1016/j.psyneuen.2014.03.019
- de Kloet, E.R., Joëls, M., & Holsboer, F. (2005). Stress and the brain: From adaptation to disease. Nature Reviews Neuroscience, 6, 463–475. doi:10.1038/nrn1683
- de Kloet, E.R., & Molendijk, M.L. (2016). Coping with the forced swim stressor: Towards understanding an adaptive mechanism. Neural Plasticity, 2016, 1–13. doi:10.1155/2016/6503162
- Deak, T., Bellamy, C., D’Agostino, L.G., Rosanoff, M., McElderry, N.K., & Bordner, K.A. (2005). Behavioral responses during the forced swim test are not affected by anti-inflammatory agents or acute illness induced by lipopolysaccharide. Behavioural Brain Research, 160, 125–134. doi:10.1016/j.bbr.2004.11.024
- Dong, X.Z., Wang, D.X., Lu, Y.P., Yuan, S., Liu, P., & Hu, Y. (2017). Antidepressant effects of Kai-Xin-San in fluoxetine-resistant depression rats. Brazilian Journal of Medical and Biological Research, 50, e6161. doi:10.1590/1414-431x20176161
- Fernandes, J., & Gupta, G.L. (2019). N-acetylcysteine attenuates neuroinflammation associated depressive behavior induced by chronic unpredictable mild stress in rat. Behavioural Brain Research, 364, 356–365. doi:10.1016/j.bbr.2019.02.025
- Ferraz, A.C., Delattre, A.M., Almendra, R.G., Sonagli, M., Borges, C., Araujo, P., … Lima, M.M. (2011). Chronic ω-3 fatty acids supplementation promotes beneficial effects on anxiety, cognitive and depressive-like behaviors in rats subjected to a restraint stress protocol. Behavioural Brain Research, 219, 116–122. doi:10.1016/j.bbr.2010.12.028
- Fornaro, M., Rocchi, G., Escelsior, A., Contini, P., & Martino, M. (2013). Might different cytokine trends in depressed patients receiving duloxetine indicate differential biological backgrounds. Journal of Affective Disorders, 145, 300–307. doi:10.1016/j.jad.2012.08.007
- Gądek-Michalska, A., Tadeusz, J., Rachwalska, P., & Bugajski, J. (2016). Psychosocial stress inhibits additional stress-induced hyperexpression of NO synthases and IL-1β in brain structures. Pharmacological Reports, 68, 1178–1196. doi:10.1016/j.pharep.2016.09.003
- Galea, L.A., McEwen, B.S., Tanapat, P., Deak, T., Spencer, R.L., & Dhabhar, F.S. (1997). Sex differences in dendritic atrophy of CA3 pyramidal neurons in response to chronic restraint stress. Neuroscience, 81, 689–697. doi:10.1016/S0306-4522(97)00233-9
- Girardi, C.E., Zanta, N.C., & Suchecki, D. (2014). Neonatal stress-induced affective changes in adolescent Wistar rats: Early signs of schizophrenia-like behavior. Frontiers in Behavioral Neuroscience, 8, 319. doi:10.3389/fnbeh.2014.00319
- Gould, E., McEwen, B.S., Tanapat, P., Galea, L.A., & Fuchs, E. (1997). Neurogenesis in the dentate gyrus of the adult tree shrew is regulated by psychosocial stress and NMDA receptor activation. The Journal of Neuroscience, 17, 2492–2498. doi:10.1523/JNEUROSCI.17-07-02492.1997
- Grissom, N., & Bhatnagar, S. (2009). Habituation to repeated stress: Get used to it. Neurobiology of Learning and Memory, 92, 215–224. doi:10.1016/j.nlm.2008.07.001
- Hamani, C., Amorim, B.O., Wheeler, A.L., Diwan, M., Driesslein, K., Covolan, L., … Nobrega, J.N. (2014). Deep brain stimulation in rats: Different targets induce similar antidepressant-like effects but influence different circuits. Neurobiology of Disease, 71, 205–214. doi:10.1016/j.nbd.2014.08.007
- Hamani, C., Giacobbe, P., Diwan, M., Balbino, E.S., Tong, J., Bridgman, A., … Nobrega, J.N. (2012). Monoamine oxidase inhibitors potentiate the effects of deep brain stimulation. American Journal of Psychiatry, 169, 1320–1321. doi:10.1176/appi.ajp.2012.12060754
- Hamani, C., Machado, D.C., Hipólide, D.C., Dubiela, F.P., Suchecki, D., Macedo, C.E., … Nobrega, J.N. (2012). Deep brain stimulation reverses anhedonic-like behavior in a chronic model of depression: Role of serotonin and brain derived neurotrophic factor. Biological Psychiatry, 71, 30–35. doi:10.1016/j.biopsych.2011.08.025
- Han, A., Yeo, H., Park, M.J., Kim, S.H., Choi, H.J., Hong, C.W., & Kwon, M.S. (2015). IL-4/10 prevents stress vulnerability following imipramine discontinuation. Journal of Neuroinflammation, 12, 197. doi:10.1186/s12974-015-0416-3
- Harris, R.B. (2015). Chronic and acute effects of stress on energy balance: Are there appropriate animal models? American Journal of Physiology-Regulatory, Integrative and Comparative Physiology, 308, R250–R265. doi:10.1152/ajpregu.00361.2014
- Jaremka, L.M., Lindgren, M.E., & Kiecolt-Glaser, J.K. (2013). Synergistic relationships among stress, depression, and troubled relationships: Insights from psychoneuroimmunology. Depression and Anxiety, 30, 288–296. doi:10.1002/da.22078
- Kearns, R.R., & Spencer, R.L. (2013). An unexpected increase in restraint duration alters the expression of stress response habituation. Physiology & Behavior, 122, 193–200. doi:10.1016/j.physbeh.2013.03.029
- Koolhaas, J.M., Bartolomucci, A., Buwalda, B., de Boer, S.F., Flügge, G., Korte, S.M., … Fuchs, E. (2011). Stress revisited: A critical evaluation of the stress concept. Neuroscience & Biobehavioral Reviews, 35, 1291–1301. doi:10.1016/j.neubiorev.2011.02.003
- Krügel, U., Fischer, J., Radicke, S., Sack, U., & Himmerich, H. (2013). Antidepressant effects of TNF-α blockade in an animal model of depression. Journal of Psychiatric Research, 47, 611–616. doi:10.1016/j.jpsychires.2013.01.007
- Lezak, K.R., Missig, G., & Carlezon, W.A. (2017). Behavioral methods to study anxiety in rodents. Dialogues in Clinical Neuroscience, 19, 181–191.
- Li, H., Liu, X., Poh, Y., Wu, L., Zhou, Q.G., & Cai, B.C. (2014). Rapid determination of corticosterone in mouse plasma by ultra fast liquid chromatography-tandem mass spectrometry. Biomedical Chromatography, 28, 1860–1863. doi:10.1002/bmc.3232
- Macedo, G.C., Morita, G.M., Domingues, L.P., Favoretto, C.A., Suchecki, D., & Quadros, I.M.H. (2018). Consequences of continuous social defeat stress on anxiety- and depressive-like behaviors and ethanol reward in mice. Hormones and Behavior, 97, 154–161. doi:10.1016/j.yhbeh.2017.10.007
- Ménard, C., Pfau, M.L., Hodes, G.E., & Russo, S.J. (2017). Immune and neuroendocrine mechanisms of stress vulnerability and resilience. Neuropsychopharmacology, 42, 62–80. doi:10.1038/npp.2016.90
- Miragaia, A.S., de Oliveira Wertheimer, G.S., Consoli, A.C., Cabbia, R., Longo, B.M., Girardi, C.E.N., & Suchecki, D. (2018). Maternal deprivation increases anxiety- and depressive-like behaviors in an age-dependent fashion and reduces neuropeptide Y expression in the amygdala and hippocampus of male and female young adult rats. Frontiers in Behavioral Neuroscience, 12, 159.
- Molendijk, M.L., & de Kloet, E.R. (2015). Immobility in the forced swim test is adaptive and does not reflect depression. Psychoneuroendocrinology, 62, 389–391. doi:10.1016/j.psyneuen.2015.08.028
- Oh, H.A., Kim, D.E., Choi, H.J., Kim, N.J., & Kim, D.H. (2015). Anti-stress effects of 20(S)-protopanaxadiol and 20(S)-protopanaxatriol in immobilized mice. Biological & Pharmaceutical Bulletin, 38, 331–335. doi:10.1248/bpb.b14-00669
- Padovan, C.M., & Guimarães, F.S. (2000). Restraint-induced hypoactivity in an elevated plus-maze. Brazilian Journal of Medical and Biological Research, 33, 79–83. doi:10.1590/S0100-879X2000000100011
- Pearson-Leary, J., Eacret, D., & Bhatnagar, S. (2019). Interleukin-1α in the ventral hippocampus increases stress vulnerability and inflammation-related processes. Stress, In press. doi:10.1080/10253890.2019.1673360
- Porsolt, R.D., Bertin, A., & Jalfre, M. (1977). Behavioral despair in mice: A primary screening test for antidepressants. Archives Internationales de Pharmacodynamie et de Therapie, 229, 327–336.
- Retana-Márquez, S., Bonilla-Jaime, H., Vázquez-Palacios, G., Domínguez-Salazar, E., Martínez-García, R., & Velázquez-Moctezuma, J. (2003). Body weight gain and diurnal differences of corticosterone changes in response to acute and chronic stress in rats. Psychoneuroendocrinology, 28, 207–227. doi:10.1016/S0306-4530(02)00017-3
- Réus, G.Z., Fries, G.R., Stertz, L., Badawy, M., Passos, I.C., Barichello, T., … Quevedo, J. (2015). The role of inflammation and microglial activation in the pathophysiology of psychiatric disorders. Neuroscience, 300, 141–154. doi:10.1016/j.neuroscience.2015.05.018
- Riaz, M.S., Bohlen, M.O., Gunter, B.W., Quentin, H., Stockmeier, C.A., & Paul, I.A. (2015). Attenuation of social interaction-associated ultrasonic vocalizations and spatial working memory performance in rats exposed to chronic unpredictable stress. Physiology & Behavior, 152, 128–134. doi:10.1016/j.physbeh.2015.09.005
- Samtani, M.N., & Jusko, W.J. (2007). Quantification of dexamethasone and corticosterone in rat biofluids and fetal tissue using highly sensitive analytical methods: Assay validation and application to a pharmacokinetic study. Biomedical Chromatography, 21, 585–597. doi:10.1002/bmc.788
- Sarkar, A., & Kabbaj, M. (2016). Sex differences in effects of ketamine on behavior, spine density, and synaptic proteins in socially isolated rats. Biological Psychiatry, 80, 448–456. doi:10.1016/j.biopsych.2015.12.025
- Simon, N.M., McNamara, K., Chow, C.W., Maser, R.S., Papakostas, G.I., Pollack, M.H., … Wong, K.K. (2008). A detailed examination of cytokine abnormalities in Major Depressive Disorder. European Neuropsychopharmacology, 18, 230–233. doi:10.1016/j.euroneuro.2007.06.004
- Slattery, D.A., & Cryan, J.F. (2012). Using the rat forced swim test to assess antidepressant-like activity in rodents. Nature Protocols, 7, 1009–1014. doi:10.1038/nprot.2012.044
- Slattery, D.A., & Cryan, J.F. (2017). Modelling depression in animals: At the interface of reward and stress pathways. Psychopharmacology, 234, 1451–1465. doi:10.1007/s00213-017-4552-6
- Surget, A., Tanti, A., Leonardo, E.D., Laugeray, A., Rainer, Q., Touma, C., … Belzung, C. (2011). Antidepressants recruit new neurons to improve stress response regulation. Molecular Psychiatry, 16, 1177–1188. doi:10.1038/mp.2011.48
- Swiergiel, A.H., & Dunn, A.J. (2006). Feeding, exploratory, anxiety- and depression-related behaviors are not altered in interleukin-6-deficient male mice. Behavioural Brain Research, 171, 94–108. doi:10.1016/j.bbr.2006.03.024
- Tamashiro, K.L., Nguyen, M.M., Ostrander, M.M., Gardner, S.R., Ma, L.Y., Woods, S.C., & Sakai, R.R. (2007). Social stress and recovery: Implications for body weight and body composition. American Journal of Physiology-Regulatory, Integrative and Comparative Physiology, 293, R1864–R1874. doi:10.1152/ajpregu.00371.2007
- Tan, S., Wang, Y., Chen, K., Long, Z., & Zou, J. (2017). Ketamine alleviates depressive-like behaviors via down-regulating inflammatory cytokines induced by chronic restraint stress in mice. Biological & Pharmaceutical Bulletin, 40, 1260–1267. doi:10.1248/bpb.b17-00131
- Tynan, R.J., Naicker, S., Hinwood, M., Nalivaiko, E., Buller, K.M., Pow, D.V., … Walker, F.R. (2010). Chronic stress alters the density and morphology of microglia in a subset of stress-responsive brain regions. Brain, Behavior, and Immunity, 24, 1058–1068. doi:10.1016/j.bbi.2010.02.001
- Vasconcelos, M., Stein, D.J., & de Almeida, R.M. (2015). Social defeat protocol and relevant biomarkers, implications for stress response physiology, drug abuse, mood disorders and individual stress vulnerability: A systematic review of the last decade. Trends in Psychiatry and Psychotherapy, 37, 51–66. doi:10.1590/2237-6089-2014-0034
- Verma, P., Hellemans, K.G., Choi, F.Y., Yu, W., & Weinberg, J. (2010). Circadian phase and sex effects on depressive/anxiety-like behaviors and HPA axis responses to acute stress. Physiology & Behavior, 99, 276–285. doi:10.1016/j.physbeh.2009.11.002
- Voorhees, J.L., Tarr, A.J., Wohleb, E.S., Godbout, J.P., Mo, X., Sheridan, J.F., … Marsh, C.B. (2013). Prolonged restraint stress increases IL-6, reduces IL-10, and causes persistent depressive-like behavior that is reversed by recombinant IL-10. PLoS One, 8, e58488. doi:10.1371/journal.pone.0058488
- Wang, Y.L., Han, Q.Q., Gong, W.Q., Pan, D.H., Wang, L.Z., Hu, W., Yang, M., Li, B., Yu, J., & Liu Q. (2018). Microglial activation mediates chronic mild stress-induced depressive- and anxiety-like behavior in adult rats. Journal of Neuroinflammation, 15, 21. doi: 10.1186/s12974-018-1054-3
- Willner, P. (1997). Validity, reliability and utility of the chronic mild stress model of depression: A 10-year review and evaluation. Psychopharmacology, 134, 319–329. doi:10.1007/s002130050456
- Wohleb, E.S., McKim, D.B., Sheridan, J.F., & Godbout, J.P. (2014). Monocyte trafficking to the brain with stress and inflammation: A novel axis of immune-to-brain communication that influences mood and behavior. Frontiers in Neuroscience, 8, 447. doi:10.3389/fnins.2014.00447
- Yan, H.C., Cao, X., Das, M., Zhu, X.H., & Gao, T.M. (2010). Behavioral animal models of depression. Neuroscience Bulletin, 26, 327–337. doi:10.1007/s12264-010-0323-7
- Yin, X., Guven, N., & Dietis, N. (2016). Stress-based animal models of depression: Do we actually know what we are doing? Brain Research, 1652, 30–42. doi:10.1016/j.brainres.2016.09.027