Abstract
Stimuli and responses that occur in close temporal contiguity are bound to each other and stored in short-term episodic traces or event files. A repetition of any of the features within an event file results in the retrieval of the entire event file and can influence responding. Along with task-relevant features, event files also contain task-irrelevant features, which are also bound to responses – distractor-response binding. In the present study, the distractor-response binding effect was examined under stress. Stress was induced via a Cold Pressor Test (CPT) and was manipulated between subjects. Distractor-response binding effects were measures at pre- and post-intervention. The CPT produced reliable effects on cortisol measurements and subjective ratings, however, no difference in the distractor-response binding effects between the groups was observed. Results are discussed against the background of the inconsistent results in the literature with respect to stimulus-response binding and stress.
Introduction
Everyday life often includes repetitive actions that always have to be carried out in a similar fashion in similar situations, for instance pressing a button to open the microwave when the timer goes off, or pressing the accelerator when the traffic light turns green. These situations require the association of a particular stimulus with a particular response. Such associations or bindings are stored together in episodic representations (S-R bindings, see Henson et al., Citation2014 for an overview). In the long-term, these instances accumulate over time, which leads to the automatization of the responses (Logan, Citation1988). However, even in the short term, these episodic representations can influence behavior. According to the Theory of Event Coding (Hommel et al., Citation2001) all stimulus and response features of a particular event are bound together and stored in an event file. Repeating any of the stimulus or response features contained in an event file, leads to the retrieval of the entire event file. These processes of integration and retrieval are assumed to underlie a large variety of action control paradigms (see the BRAC framework; Frings et al., Citation2020).
Interestingly, event files contain not only the features of task-relevant stimuli but also task-irrelevant stimuli, which are also bound to the response–distractor-response binding (e.g. Frings et al., Citation2007). Thus, repeating an irrelevant feature or a distractor will also retrieve the previous event file. Repeating the distractor has different outcomes on behavior depending upon whether the response is also repeated or not. If the response is repeated, then repeating the distractor facilitates responding. The repeated distractor retrieves the old event file and with it the response information, which is still appropriate since the response has to be repeated. If, however, the response is to be changed, then a repetition of the distractor will interfere with responding, since the event file retrieved by the repeated distractor contains response information that is no longer appropriate. Thus, two responses, one retrieved and one computed via the algorithmic route based on the stimulus are in conflict, which increases reaction times and/or error rates. The distractor-response binding effect is a robust effect and has been observed with a number of different stimuli, such as visual (e.g. Frings et al., Citation2007), auditory (e.g. Moeller, Rothermund, & Frings, Citation2012), and tactile stimuli (Moeller & Frings, Citation2011). It has also been observed to be largely independent of central attentional resources (e.g. Hommel, Citation2005; Moeller & Frings, Citation2015), although it has been shown to be modulated by certain types of attention, such as spatial attention (Moeller & Frings, Citation2014) and feature-based attention (Singh, Moeller, Koch, & Frings, Citation2018).
Stress and Stimulus-Response binding
Stress has become increasingly more prevalent in daily life, and it has consequences on cognition. Stress presumably affects cognition and behavior via the activation of the hypothalamus-pituitary-adrenal (HPA) axis and the release of cortisol. In response to stress, the hypothalamus releases corticotropic releasing hormone into the blood stream, this causes the release of adrenocorticotropic hormone by the pituitary gland, which leads to the release of cortisol by the adrenal cortex (Bear et al., Citation2016; Russell & Lightman, Citation2019). Increased cortisol influences learning and memory processes; cortisol binds to mineralocorticoid (MR) and glucocorticoid receptors (GR) in the hippocampus and modulates learning and memory. Both types of receptors influence different stages or processes of learning, with MR receptors mainly modulating the reactivity to novel stimuli or situations and GR receptors modulating the consolidation process (De Kloet et al., Citation1999). Additionally, stress influences cognition via an increase of dopamine, which impairs prefrontal cortext (PFC) functioning (Arnsten & Goldman-Rakic, Citation1998). Broadly, stress impairs performance on tasks relying on the PFC, and facilitates performance on tasks relying on structures like the basal ganglia or amygdala (Arnsten Citation2009; Citation2015). Stress has been shown to impair performance on PFC reliant tasks like working memory tasks (Arnsten Citation2009; Citation2015; Luethi et al., Citation2008; Olver et al., Citation2015), and influence memory processes including enhancement of memory consolidation (Roozendaal, 2009; Schwabe et al., Citation2012) and impairment of memory retrieval (Roozendaal, 2009; Schwabe et al., Citation2012), and facilitation of habit formation (Arnsten, Citation2009). In general, the effects of stress on cognition would seem to depend upon the type of task and the structures involved; stress impairs performance on more complex tasks requiring flexible thinking, but improves performance on simpler tasks that are well established.
Bindings between stimuli and responses can be considered a form of associative learning (e.g. Logan, Citation1988) or stimulus-response learning (Dobbins, et al., Citation2004; Henson et al., Citation2014) or at least they may contribute to this type of learning (Moeller & Frings, Citation2017a; Citation2017). Parts of the PFC and the basal ganglia have both been implicated, along with other brain structures, in binding processes (e.g. Murray et al., Citation2000). In their review, Murray et al., (Citation2000) suggested important roles for the basal ganglia and ventral and orbital PFC in visuomotor mappings, while the involvement of the dlPFC was not completely clear. Similarly, both the PFC and basal ganglia are involved in learning processes, with the basal ganglia being involved more strongly in the early learning stage and the PFC in the later stages (Pasupathy & Miller, Citation2005). Based on previous literature, one prediction with respect to distractor-response bindings is that stress boosts binding effects. Stress results in a higher turnover of dopamine, which impairs PFC functioning, but enhances functioning in other areas, like the basal ganglia (Arnsten Citation2009), which has been implicated in bindings and early leaning stages. The PFC has been known to be involved in goal directed behavior, and an impairment of this functioning would result in an impairment of goal directed behavior and consequently more attention being allocated to irrelevant stimuli. Studies measuring ERP correlates of task performance under stress have found smaller P3 amplitudes in stress conditions a component, which reflects task related evaluation of targets (Shackman et al., Citation2011; Sänger et al., Citation2014; see Polich, Citation2007 for a review of the P3 component). These smaller P3 amplitudes were interpreted as less efficient resource allocation to the task-relevant stimulus and possible diversion of attention to irrelevant stimuli (Shackman et al., Citation2011; Sänger et al., Citation2014). This inefficient allocation of attention to only the task-relevant features might result in larger distractor-response binding effects. Additionally, dopamine has been observed to boost learning processes (e.g. Arnsten, Citation2009; Wise, Citation2004). Given that the basal ganglia is involved in binding processes and in early learning stages, it is possible that the learning of these bindings are boosted. Evidence for the role of dopamine in the binding process comes from a study examining the effect of affect on stimulus-response bindings. Colzato et al. (Citation2007) found that visuomotor binding was boosted after the presentation of pictured inducing positive affect, however, this effect was largely restricted to bindings between task-relevant features and the response. The authors interpreted this as evidence of the involvement of the dopaminergic system on binding processes (see also Colzato et al., Citation2007 for a similar argument).
However, although the above findings might imply stronger binding effects under stress, it is unlikely that the relationship between dopamine and binding processes follows a linear function; rather it is more likely that it follows an inverted U-shaped function. In support of this, Colzato et al., (Citation2008) found that in subjects with smaller binding effects in the baseline measurement, stress resulted in larger binding effects, whereas the opposite was true for participants with large binding effects in the baseline condition. Although Colzato et al., (Citation2008) observed attenuated binding effects in their study, this effect was only restricted to the bindings between task-relevant features and the response, leaving the bindings between task-irrelevant features and response unaffected. They suggested that this was due to a narrowing of attention to only the task-relevant features as suggested by Chajut & Algom (Citation2003). Based on Colzato et al.’s (Citation2008) findings, binding effects for relevant stimuli and responses should be attenuated under stress, but distractor-response bindings should remain unaffected.
On the other hand, however, in contrast to the findings of Colzato et al., (Citation2008), Frings, Larra, Gräbner, Moeller, and Schächinger (Citation2013) found that stress disrupts even bindings between irrelevant features and responses. In their study, using a DRB task pre- and post-stress-application, binding effects remained unchanged in the stress group, while they increased from pre- to post-block in the control group. The authors suggested that the impairment resulted from a cortisol-induced impairment of retrieval. Indeed, while stress and cortisol, can enhance memory encoding and consolidation (Roozendaal et al., Citation2009) and even enhance stimulus-response learning (Guenzel et al., Citation2014) the timing of the stress is crucial for its effect. Stress before encoding or consolidation can enhance stimulus-response learning (e.g. Guenzel, et al., Citation2014), however stress at time of retrieval impairs memory retrieval (Schwabe & Wolf, Citation2013). Since the retrieval of the previously constructed episodic trace is crucial to the binding effect, impairment of this retrieval would lead to a disruption of the binding process under conditions of stress, as observed by Frings et al., (Citation2013). Additionally, Plessow, Fischer, Kirschbaum, and Goschke (Citation2011) observed smaller interference effects due to an irrelevant feature after congruent trials in a Simon task. They interpreted these findings as stronger task shielding under stress. Thus, if task shielding is increased under stress the distractors should have less of an impact on responding than in the control condition, leading to smaller binding effects in the stress condition. Similarly, Chajut and Algom (Citation2003) suggested that since stress is resource intensive, it impairs for instance working memory functions, however, in order to deal with diminished resources it narrows focus on to the relevant stimuli, thereby improving selective attention. This narrowing of attention should also lead to a smaller impact of irrelevant information under stress, i.e. smaller distractor-response binding effects under stress. Thus, these findings would lead to the prediction of attenuated distractor-response binding effects under stress.
The present study
On theoretical grounds, stress effects on distractor-based binding effects could be expected or not – we reviewed some arguments for or against this possible modulation above. Yet, on empirical grounds, the existing studies are so few that it is hard to make to a conclusion. Colzato et al., (Citation2008) found stress effects only on target-binding while Frings et al., (Citation2013) observed no increase in distractor-response binding in the stress group compared to the control group and hence concluded somewhat indirectly that stress disrupts binding effects. It is unlikely that the difference in the results of the above-mentioned studies is completely explained by differences in the design implemented. In both studies reliable distractor-response binding effects were observed in the control conditions, indicating that implemented paradigm / task could reliably measure distractor-response binding effects. Additionally, in both studies the stress protocol led to reliable changes in physiological measures like cortisol concentrations. Thus, although the design of the present study resembles the design of the Frings et al. (Citation2013) more closely, neither outcome is more or less likely solely as a result of choice of experimental design. Given that both studies used rather small sample sizes (N = 17 and N = 22), the aim of the present study was to examine and replicate the influence of stress on the distractor-response binding effects with a larger sample against the background of the somewhat conflicting results observed in the literature. To this end, stress was induced via the CPT. Distractor-response binding effects were measured before and after the stress test and compared with a control group.
Method
Participants
Fifty five students from the University of Trier participated in the experiment. The CPT group consisted on 28 participants (8 male), median age was 22.5 years (range 18–36). The control group consisted of 27 participants (6 male), median age was 23 years (range 19 − 36). All participants reported normal or corrected-to-normal vision. All participants were screened for any exclusion criteria that would prevent their participation in the experiment. Complete exclusion criteria are reported in Appendix 1. Participants received partial course credit or monetary reimbursement for participation in the experiment. Concerning power analysis we followed the recommendation to have at least a 150% larger sample than in the original study (as suggested by Simonsohn, Citation2015). The study was approved by the Ethics Committee of the University of Trier (approval reference 50/2017) and participants gave their informed consent prior to their participation in the study.
Design
The design consisted of a 2 (time: pre-stress vs. post-stress) × 2 (response relation: repetition vs. change) × 3 (distractor relation: repetition vs. change vs. ignored repetition) × 2 (group: CPT-Stress vs. controlFootnote1), with the factor group as a between-subjects factor and all other factors within-subjects factors.
Materials
The experiment was run using the E-Prime Software, Version 2.0. Stimuli consisted of the letters D, F, J, and K each mapped to a different response. Targets and distractors were both drawn from the same stimulus set. Stimuli were always presented in the center of the screen. The target stimulus was presented in red color (R: 255, G: 0, B: 0) in the center and the distractors were presented in green color (R: 0, G: 255, B: 0) on either side of the target in a flanker formation. The background was black. Stimuli were presented in 18-point Courier New font. A response box with five horizontally places response buttons connected to the computer was used to record the responses. The central response button was not used.
Stress test
The Cold Pressor Test (CPT) was implemented as the stress manipulation. The CPT is a widely used tool, which was first described by Hines and Brown (Citation1932). In the present experiment, a modified version of the CPT for feet was used (Larra et al., Citation2015). In the modified version, instead of immersing the non-dominant hand in cold water, participants immerse both feet in cold water. This modified version has been reliably used to induce stress. In the CPT for feet, participants immersed both feet, up to their ankles, in ice water (temperature ca. 2–4°C) for 3 min. The ice water was placed before the participants, who were seated before the computer, and they were instructed when to immerse their feet in the water and when they could take their feet out. Participants were not informed about the time left. A total of five salivary probes per participant was collected; baseline (T1), immediately before the CPT (T2), 15 min post CPT (T3), 30 min post CPT (T4), and 60 min post CPT (T5). The timing of the salivary samples was based on previous literature (Larra et al., Citation2015), who observed peak salivary cortisol levels at 15 and 30 min post CPT. Salivary samples were collected using Salivette Tubes (Sarstadt, Germany). Participants were instructed on the correct usage of the Salivette Tubes, they then collected the saliva themselves and handed it to the experimenter. After the CPT, participants were provided with a towel to dry their feet. The salivary probes were stored at about −20° C until the analysis. In the control group, participants followed the same protocol with the exception that were asked to place their feet in a basin of lukewarm water (about 30 C) instead of in cold water.
Distractor-Response binding paradigm
Participants performed a letter identification task. Participants were presented with a centrally presented target in red with two flanking distractors in green. Targets and distractors all belonged to the same stimulus set (D, F, J, & K). Each trial began with a fixation cross at the center of the screen for 1000 ms. Following the fixation cross the prime was presented and remained on the screen until a response had been registered. After the prime, a blank screen was presented for 500 ms, following which the probe was presented and remained on screen until a response had been registered. The trial procedure is presented in . For the distractor-response binding effect, a combination of response relation (repetition vs. change) and distractor relation (repetition vs. change) was implemented. In response repetition trials (RR), the response from the prime was repeated in the probe. In response change trials (RC), the prime and the probe required different responses. In distractor repetition trials (DR), the distractors from the prime were repeated in the probe. In distractor change trials (DC), the distractor in the prime and the probe were different. Additionally ignored repetition trials (IR), the distractor from the prime was presented as the target in the probe and the probe distractors were changed, were included as filler trials, but not analyzed for the distractor-response binding effect. Thus, in response repetition – distractor repetition trials (RRDR), both the prime response and the prime distractor were repeated in the probe. In the response repetition – distractor change trials (RRDC), the prime response is repeated in the probe, but the distractor is changed. In response change – distractor repetition trials (RCDR) the prime distractor was repeated in the probe but the response was changed. In the response change – distractor change trials (RCDC), both the prime response and prime distractor were changed in the probe. Distractor-response binding effects are computed as following (RRDC – RRDR) – (RCDC – RCDR).
Procedure
Before recruitment, participants were screened for any exclusion criteria that might prevent their participation in the experiment. Participants were tested individually, and data collection for the recruited participants was restricted to afternoon to early evenings (ca. 12:00 − 18:00 h) to avoid large fluctuations in baseline cortisol levels due to time of day. Participants were first informed to the experimental procedure and then asked to sign a consent form, indicating their willingness to participate in the experiment. Electrodes for heart rate measurement were then affixed to the participantFootnote2. Participants were then seated before a computer at a distance of about 60 cm and a salivary probe was collected. Task instructions were presented on the screen. Participants first worked through the pre-stress block of trials. After the pre-stress block, another salivary probe was collected. Depending on which group the participants were assigned to, they then underwent either a Cold Pressor Test Stress protocol, or a control protocol. The CPT protocol as used in Larra et al. (Citation2015) was implemented in the present study. Following the CPT/control protocol, participants did a filler task (crossword puzzles) for 10 min. After the filler task participants did a post-stress block of trials starting 13 min after the start of the CPT protocol. Salivary probes were collected at 15 and 30 min after the stress/control protocol. The timing of the salivary probes was based on previous findings using the same paradigm (Larra et al., Citation2015), and peak cortisol concentrations were expected at 15 and 30 min post CPT. In order to ensure the timing of the salivary probes, breaks were programmed into the post-stress block at which time point the salivary probes were to be collected. If the participant completed the post-stress block before the stress +30 min mark, they remained seated in the same position until the salivary probe was collected. After the post-stress block, participants remained in the lab until 60 min after the stress/control protocol, at which point the final salivary probe was collected. During this time, participants completed three questionnaires, the German Version of the Cognitive Failures Questionnaire (CFQ-D), the German version of the Social Interaction Anxiety Scale (SIAS), and the German version of the Gaze Anxiety Rating Scale (GARS). Once the final salivary probe was collected, participants were compensated for their participation and allowed to leave. The experimental procedure is presented in . Instructions for the participants’ task were presented on screen. Participants were asked to respond to a centrally presented target letter with the appropriate button press.
Biochemical analyses of salivary samples
For cortisol analysis, a time-resolved fluorescence immunoassay (Dressendörfer, Kirschbaum, Rohde, Stahl & Strasburger, Citation1992) was used. Hundred microlitres of saliva were used for duplicate analysis (50 µl per well). Salivary AA was analyzed using the chromogenic molecule 2-Chloro-4-nitrophenyl-a-D-maltotrioside (Lorentz, Gütschow & Renner, Citation1999). Saliva was diluted 1:200 with assay diluent. Sixteen microlitres of the diluted saliva were used for duplicate analysis (8 µl per well). The intra-assay coefficients of variation as well as the corresponding inter-assay coefficients of variation were all below 10%.
Results
Cortisol data
Salivary cortisol concentrations
A 2 (Group: CPT vs. control) × 5 (Time: T1 vs. T2 vs. T3 vs. T4 vs. T5) mixed MANOVA with the factor Group as a between-Subjects factor and Time as a within-Subjects factor was run (). A significant main effect of time was observed, F (4, 50)=11.61, p<.001, ηp2=.48, indicating higher cortisol concentrations at T3 and T4 compared to the other time points. The main effect of group approached significance, F (1, 53)=11.61, p=.069, ηp2=.06. Importantly, the interaction of time x group was significant, F (4, 50)=5.31, p=.001, ηp2=.30, indicating that only in the CPT group the cortisol concentrations varied as a function of time in the pre- and post-block measurements, but not in the control group (). Post-hoc comparisons indicated significant differences between cortisol concentrations for the CPT group between T1 vs T3, t(27),=4.83, p<.001, T1 vs. T4 t(27),=2.99, p<.001, T2 vs T3 t(27),=4.83, p<.001., T2 vs T4 t(27),=3.21, p=.003, T3 vs T5, t(27),=4.93, p<.001, and T4 vs T5, t(27),=4.63, p<.001. In the control group the only significant differences were between T2 and T5, t(26),=2.46, p=.021, T3 and T5, t(26),=3.53, p=.002, and T4 vs. T5, t(26),=3.76, p=.001. For the analysis of cortisol responders vs. non-responders in the CPT group a criterion of a 1.5 nmol/l increase from baseline measurement to peak was used (Miller, Plessow, Kirschbaum, & Stalder, Citation2013). This resulted in 14 Participants classified as responders and 14 classified as non-responders. A 2 (Cortisol response: Responders vs. non-responders) × 5 (Time: T1 vs. T2 vs. T3 vs. T4 vs. T5) mixed MANOVA with the factor Group as a between-Subjects factor and Time as a within-Subjects factor was run for the subjects in the CPT group (). The main effect of time was significant, F (4, 23)=14.84, p<.001, ηp2=.72, the main effect of cortisol response was also significant, F (1, 26)=13.30, p = .001, ηp2=.34. The interaction of time x cortisol response was significant, F (4, 23)=9.69, p<.001, ηp2=.63.
Figure 3. Mean salivary cortisol (left panel), comparison of cortisol responder vs. non-responders (center panel), and alpha amylase (right panel) concentrations in the CPT and control groups.
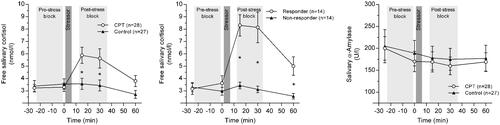
Table 1. Mean subjective stress ratings (SD in parenthesis).
Alpha-amylase concentrations
A 2 (Group: CPT vs. control) x 5 (Time: T1 vs. T2 vs. T3 vs. T4 vs. T5) mixed MANOVA with the factor Group as a between-Subjects factor and Time as a within-Subjects factor was run. Neither the main effect of time, F (4, 50)=1.84, p=.135, ηp2=.13, nor the main effect of group, F (1, 53)=0.11, p=.748, ηp2=.00 reached significance. The interaction of time and group, F (4, 50)=0.21, p=.930, ηp2=.02 also did not reach significance. The alpha-amylase concentrations are presented in .
Subjective stress ratings
Subjective stress ratings on each of the four items (discomfort, pain, stress, difficulty) were analyzed with t-tests for independent groups with a bonferroni corrected alpha level of 0.05 / 4=.0125. For all of the items significant differences were observed between the groups; discomfort t(37)=21.43, p<.001, with the CPT group showing higher levels of discomfort (M = 8.25, SD = 1.78) compared to the control group (M = 0.39, SD = 0.71). For pain t(27)=14.87, p<.001, with the CPT group showing higher pain ratings (M = 7.04, SD = 2.49) compared to the control group (M = 0.02, SD = 0.10). For stress, t(30)=11.20, p<.001, with the CPT group showing higher stress ratings than the control group (M = 5.80, SD = 2.35) compared to the control group (M = 0.20, SD = 0.49). For difficulty, t(31)=10.98, p<.001, with the CPT group showing higher difficulty ratings (M = 6.61, SD = 2.87) compared to the control group (M = 0.44, SD = 0.71). Mean stress ratings are depicted in .
Questionnaires
No significant differences between the groups was observed on any of the three questionnaire scores. For the Cognitive Failures Questionnaire, the data of 5 participants were excluded due to incomplete or incorrectly completed questionnaires. The scores were computed for each participant and analyzed in a one-way ANOVA. There was no significant difference between the CPT and the control group, F(1, 49)=0.24, p=.630. For the Social Interaction Anxiety Scale the data of 6 participants were excluded due to incomplete or incorrectly completed questionnaires. The scores were computed for each participant and analyzed in a one-way ANOVA. There was no significant difference between the CPT and the control group, F(1, 47)=0.07, p=.787. For the Gaze Anxiety Rating Scale, the data of two participants were excluded due to incomplete or incorrectly completed questionnaires. The scores were computed for each participant and analyzed in a one-way ANOVA for the fear and anxiety subscales as well as the total score. There was no significant difference between the CPT and the control group, all Fs < 0.780, all ps>.381.
Behavioral data
RTs. Only trials with correct responses to both the prime and the probe were included in the RT analyses. RTs shorter than 200 ms and longer than 1.5 inter quartile ranges above the third quartile of the participants individual RT distribution were excluded. This resulted in a total of 15.18% of trials being excluded, 4.76% incorrect prime response, 4.29% incorrect probe response, 0.005% RTs shorter than 200 ms, and 6.12% RTs longer than 1.5 inter quartile ranges above the third quartile of the participant RT distribution. Mean RTs are depicted in .
Table 2. Mean RTs (SD in parenthesis) for each condition.
Probe RTs were analyzed in a 2 (time: pre-stress vs. post-stress) × 2 (response relation: repetition vs. change) × 2 (distractor relation: repetition vs. change) × 2 (Group: CPT vs. control) mixed MANOVA, with the factors response relation and distractor relation as within-Subjects factors and the factor group as a between-Subjects factor. Pillai’s trace was used as the criterion. A significant main effect of time was observed, F(1, 53)=85.69, p<.001, ηp2=.62, indicating shorter response times in the post-stress block (M = 570 ms, SD = 88 ms) compared to the pre-stress block (M = 610 ms, SD = 98 ms). A significant main effect of response relation was observed, F(1, 53)=366.36, p<.001, ηp2=.87, indicating faster responding in the response repetition trials (M = 499 ms, SD = 84 ms) compared to the response change trials (M = 689 ms, SD = 112 ms). A significant main effect of distractor relation was observed, F(1, 53)=46.92, p<.001, ηp2=.47, indicating faster responding in the distractor repetition trials (M = 584 ms, SD = 92 ms) compared to the distractor change trials (M = 595 ms, SD = 92 ms). The interaction of time × response relation reached significance, F(1, 53)=11.29, p= .001, ηp2=.18, indicating a larger difference in the response times for the response repetition vs. response change trials in the pre stress condition compared to the post stress condition (199 ms vs 183 ms, t(54)=3.26, p=.002). The response relation x distractor relation interaction was significant, F(1, 53)=48.16, p<.001, ηp2=.48, indicating that the distractor repetition effect differed in response repetition vs response change conditions (22 ms vs. −1.26 ms, t(54)=7.12, p<.001), i.e. an overall distractor-response binding effect. However the relevant 4-way interaction of time × response relation × distractor relation × group was not significant, F(1, 53)=0.00, p=.991, ηp2=.00, indicating no difference in the binding effects as a function of time and group (). All main effects and interactions are reported in .
Figure 4. Mean distractor-response binding effects for the pre- and post-stress blocks for the stressed group and control group, for RTs (upper panel) and error rates (lower panel).
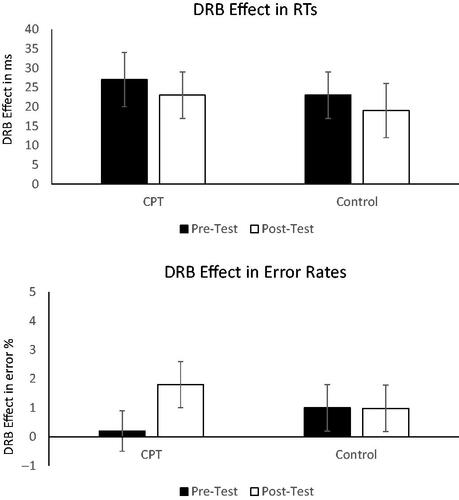
Table 3. Results of the RT analysis.
Error rates
Mean error rates are depicted in . The same analysis was run on the error rates. Only trials with a correct response to the prime were considered for the analysis. The main effect of response relation was significant, F(1, 53)=115.34, p<.001, ηp2=.69, indicating fewer errors in the response repetition (M = 0.48%, SD = 0.68) trials compared to the response change (M = 4.44%, SD = 3.02%) trials. The interaction of time × response relation reached significance, F(1, 53)=4.26, p=.044, ηp2=.07, indicating a larger difference in the error rates for the response repetition vs. response change trials in the pre stress compared to the post stress condition (4.24% vs 3.69%, t(54)=2.15, p=.036). The response relation × distractor relation interaction was significant, F(1, 53)=7.78, p= .007, ηp2=.13, indicating that the distractor repetition effect differed in response repetition vs response change conditions (0.18% vs −0.81%, t(54)=2.82, p<.001), i.e. an overall distractor-response binding effect. The relevant four way interaction of time × response relation × distractor relation × group, F(1, 53)=0.99, p=.324, ηp2=.02, did not reach significance, indicating that there was no difference in the binding effects as a function of time and group (). All main effects and interactions are reported in .
Table 4. Mean error rates (SD in parenthesis) for each condition.
Table 5. Results of the analysis of error rates.
In order to gather further evidence for the non-significant effect of stress on the DRB effect, a bayesian t-test was conducted (JASP Version 0.14). The dependent variable was the difference of the DRB effect pre-stress and post-stress. The bayes factor for the t-test for independent samples was BF01=3.68, indicating that the data is 3.68 times more likely under the null hypothesis which is considered moderate (Lee & Wagenmakers, Citation2013) to positive evidence (Kass & Raftery, Citation1995).
Discussion
The aim of the present study was to examine the effects of stress on the distractor binding effect with a large sample. Participants were assigned to either a control group or the experimental group. Distractor-response binding effects were measured at baseline before the stress or control protocol and after stress or control protocol. The CPT produced reliable differences in both subjective stress ratings as well as in salivary cortisol concentrations. However, no difference in the strength of the binding effects from baseline measurement to post stress measurement was observed, suggesting that stress does not modulate distractor-response bindings – this result was confirmed by Bayesian analysis suggesting that is 3.68 times more likely to observe the data pattern under the null hypothesis.
The present findings are in line with the findings of Colzato et al. (Citation2008). While they observed significant impairments in the binding effects between relevant features and responses after the application of the CPT, they observed no such impairments in the binding effects for irrelevant features and responses. Thus, their results suggest that only task-relevant binding effects are influenced by stress. They concluded that dopamine turnover was responsible for the modulation. Increased dopamine impairs PFC functioning and thus also goal directed behavior (Shackman et al., Citation2011), while at the same time enhancing basal ganglia functioning (Arnsten, Citation2009) which is implicated in bindings (Murray et al., Citation2000). At the same time, in order to compensate for these impairments under stress, attention is narrowed to only the task-relevant stimuli thus, paradoxically, increasing selectivity (Chajut & Algom, Citation2003). This narrowing of attention might explain why distractor-related effects are not modulated. In line with this idea, Colzato et al., (Citation2008) observed a modulation of stress on task-relevant stimulus-response bindings but not on task-irrelevant bindings. The present results would seem to support the idea that bindings between distractors, i.e. irrelevant stimuli and responses, are unaffected by stress.
On the other hand, the present results are in some conflict with the results of Frings et al. (Citation2013) who found disruptions in the distractor-response binding effects after stress compared to a control group. They concluded that cortisol related disruptions of retrieval were the underlying cause of the reduced binding effects under stress. However, the present findings do not lend support to this conclusion. Thus, although stress before retrieval can impair hippocampus-dependent memory retrieval, especially for emotional stimuli (Schwabe & Wolf, Citation2013) the present results would suggest that it does not seem to interfere with the retrieval of temporary bindings. In fact, however, the effects for stress group the effects in the present study and in the Frings et. al. (Citation2013) study are perfectly comparable – in both experiments the binding effect did not change between pre- and post-block; yet, what is different in the present study is that also the control group did not show any difference between pre- and post-block while in the Frings et al. (Citation2013) study the control group showed larger binding in the post-block. Although it is not unusual that binding effects increase somewhat during an experiment this increase might depend on context factors (e.g. many trials that make retrieval of responses efficient; Kanwisher et al., Citation1995). Even though there are small differences between the present study and the Frings et al. study it does not seem very likely that these differences elicit context effects that could explain the differences for the control groups. More likely seems that the higher power of the present study is a more reliable measure for distractor-based binding effects than the original study. In concert with the results of Colzato et al. (Citation2008) it can be concluded that i) distractor-response binding effects are not modulated by stress (a result that all studies agree on) and ii) that the indirect evidence for the disruptive influence of stress on binding seems at least not very reliable.
It should be noted that in the present results, although a significant effect of stress was observed on salivary cortisol, no such effect was observed in the alpha amylase concentrations. It has been known that catecholamines can influence memory for certain events, for instance glucocorticoids and noradrenaline together can enhance memory for stressful events (e.g. Roozendaal, et al., Citation2009; Schwabe & Wolf, Citation2013). In the present study, no increase in adrenal output was observed. Two possible reasons for this finding are, firstly, that adrenal output was not influenced by the CPT, or secondly, the increase in adrenal output was not evident due to the timing of the collection of salivary samples. Firstly, if the stress manipulation in the present study did not result in an increased adrenal output, and this could be an explanation for the lack of stress effects on distractor-response binding. However, since the memory enhancement is generally observed for stressful or emotional events, it is unlikely to have played a major role in the present study, since the stimuli used here were neutral. Additionally, the effect of stress on memory is dependent upon whether the consolidation or retrieval of the memory is affected. While consolidation may be enhanced (e.g. Guenzel et al., Citation2014; Roozendaal et al., Citation2009), retrieval is impaired (Schwabe & Wolf, Citation2013). In the present paradigm, both the encoding and the retrieval processes were subject to the stress manipulation. Secondly, due to the timing of the study, it is possible that the increase in adrenal output was not visible. The post-stress salivary samples were collected at stress +15 min, +30 min and +45 min. The stress +15 min and +30 min correspond to the peak cortisol concentration, however salivary alpha-amylase concentrations are generally observed earlier (e.g. Larra et al., Citation2015; using the same protocol). Thus it is possible that although the stress protocol led to an increase in salivary alpha amylase concentrations, this change was not evident due to the timing of the collection of salivary samples.
Stimulus-Response binding effects in general are assumed to function in an automatic fashion (albeit top-down influences are known to modulate binding effects). That is, according to the recent BRAC framework (Frings et al., Citation2020) the integration as well as the retrieval processes which contribute to binding effects, seem to be immune to the effect of stress. Of course, stress falls neither in the category of bottom-up nor top-down influences but rather is a different state in which the cognitive system integrates and retrieves events. Still it is a valuable assurance that action control mechanism that are based on irrelevant features or stimuli are not affected by stress, since even irrelevant stimuli might be informative in certain situations in which the automatic retrieval / or behavioral control of the appropriate action might be of value. Thus, feature binding and retrieval contribute to actions irrespective and independent of the state (at least concerning stress) in which the cognitive system actually operates.
Disclosure statement
The authors report no conflict of interest.
Additional information
Funding
Notes
1 Along with the CPT and control groups, a Socially Evaluated Cold Pressor Test (adapted from Schwabe, Haddad, & Schächinger, Citation2008, for feet) group was also implemented. Since the CPT and SECPT groups did not differ from each other, the SECPT group is not reported here. None of the analyses reported here were affected by the exclusion of the SECPT group
2 Heart rate was measured using the ECG ANS-Recorder (Neurocor, Germany), with the electrodes mounted on the participants’ chest. These data were however not analysed.
References
- Arnsten, A. F., & Goldman-Rakic, P. S. (1998). Noise stress impairs prefrontal cortical cognitive function in monkeys: evidence for a hyperdopaminergic mechanism. Archives of General Psychiatry, 55(4), 362–368. https://doi.org/https://doi.org/10.1001/archpsyc.55.4.362
- Arnsten, A. F. T. (2009). Stress signalling pathways that impair prefrontal cortex structure and function. Nature Reviews Neuroscience, 10(6), 410–422. https://doi.org/https://doi.org/10.1038/nrn2648
- Arnsten, A. F. T. (2015). Stress weakens prefrontal networks: Molecular insults to higher cognition. Nature Neuroscience, 18(10), 1376–1385. https://doi.org/https://doi.org/10.1038/nn.4087
- Bear, M. F., Connors, B. W., & Paradiso, M. A. (2016). Neuroscience: Exploring the brain. 4th Edition. Philadelphia, PA: Wolters Kluwer
- Chajut, E., & Algom, D. (2003). Selective attention improves under stress: Implications for theories of social cognition. Journal of Personality and Social Psychology, 85(2), 231–248. https://doi.org/https://doi.org/10.1037/0022-3514.85.2.231
- Colzato, L. S., Kool, W., & Hommel, B. (2008). Stress modulation of visuomotor binding. Neuropsychologia, 46(5), 1542–1548. https://doi.org/https://doi.org/10.1016/j.neuropsychologia.2008.01.006
- Colzato, L. S., van Wouwe, N. C., & Hommel, B. (2007). Feature binding and affect: Emotional modulation of visuo-motor integration. Neuropsychologia, 45(2), 440–446. https://doi.org/https://doi.org/10.1016/j.neuropsychologia.2006.06.032
- De Kloet, E. R., Oitzl, M. S., & Joëls, M. (1999). Stress and cognition: are corticosteroids good or bad guys? Trends in Neurosciences, 22(10), 422–426. https://doi.org/https://doi.org/10.1016/S0166-2236(99)01438-1
- Dobbins, I. G., Schnyer, D. M., Verfaellie, M., & Schacter, D. L. (2004). Cortical activity reductions during repetition priming can result from rapid response learning. Nature, 428(6980), 316–319. https://doi.org/https://doi.org/10.1038/nature02400
- Dressendörfer, R. A., Kirschbaum, C., Rohde, W., Stahl, F., & Strasburger, C. J. (1992). Synthesis of a cortisol-biotin conjugate and evaluation as a tracer in an immunoassay for salivary cortisol measurement. The Journal of Steroid Biochemistry and Molecular Biology, 43(7), 683–692. https://doi.org/https://doi.org/10.1016/0960-0760(92)90294-S
- Frings, C., Larra, M. F., Gräbener, A., Moeller, B., & Schächinger, H. (2013). Stress disrupts distractor-based retrieval of SR episodes. Biological Psychology, 93(1), 58–64. https://doi.org/https://doi.org/10.1016/j.biopsycho.2013.01.013
- Frings, C., Hommel, B., Koch, I., Rothermund, K., Dignath, D., Giesen, C., Kiesel, A., Kunde, W., Mayr, S., Moeller, B., Möller, M., Pfister, R., & Philipp, A. (2020). Binding and retrieval in action control (BRAC). Trends in Cognitive Sciences, 24(5), 375–313. ). https://doi.org/10.1016/j.tics.2020.02.004. https://doi.org/https://doi.org/10.1016/j.tics.2020.02.004
- Frings, C., Rothermund, K., & Wentura, D. (2007). Distractor repetitions retrieve previous responses to targets. Quarterly Journal of Experimental Psychology, 60(10), 1367–1377. https://doi.org/https://doi.org/10.1080/17470210600955645
- Guenzel, F. M., Wolf, O. T., & Schwabe, L. (2014). Glucocorticoids boost stimulus-response memory formation in humans. Psychoneuroendocrinology, 45, 21–30. https://doi.org/https://doi.org/10.1016/j.psyneuen.2014.02.015
- Henson, R. N., Eckstein, D., Waszak, F., Frings, C., & Horner, A. J. (2014). Stimulus-response bindings in priming. Trends in Cognitive Sciences, 18(7), 376–384. https://doi.org/https://doi.org/10.1016/j.tics.2014.03.004
- Hines, E. A., Jr,., & Brown, G. E. (1936). The cold pressor test for measuring the reactibility of the blood pressure: data concerning 571 normal and hypertensive subjects. American Heart Journal, 11(1), 1–9. https://doi.org/https://doi.org/10.1016/S0002-8703(36)90370-8
- Hommel, B. (2005). How much attention does an event file need? Journal of Experimental Psychology: Human Perception and Performance, 31(5), 1067–1082. https://doi.org/https://doi.org/10.1037/0096-1523.31.5.1067
- Hommel, B., Müsseler, J., Aschersleben, G., & Prinz, W. (2001). The Theory of Event Coding (TEC): A framework for perception and action planning. The. Behavioral and Brain Sciences, 24(5), 849–878. discussion 878-937. https://doi.org/https://doi.org/10.1017/S0140525X01000103
- Kanwisher, N., Driver, J., & Machado, L. (1995). Spatial repetition blindness is modulated by selective attention to color or shape. Cognitive Psychology, 29(3), 303–337. https://doi.org/https://doi.org/10.1006/cogp.1995.1017
- Kass, R. E., & Raftery, A. E. (1995). Bayes factors. Journal of the American Statistical Association, 90(430), 773–795. https://doi.org/https://doi.org/10.1080/01621459.1995.10476572
- Larra, M., F., Schilling, T.M., Röhrig, P., & Schächinger, H. (2015). Enhanced stress response by a bilateral feet compared to a unilateral hand cold pressor test. Stress (Amsterdam, Netherlands), 18(5), 589–596. https://doi.org/https://doi.org/10.3109/10253890.2015.1053452
- Lee, M. D., & Wagenmakers, E. J. (2013). Bayesian data analysis for cognitive science: A practical course. Cambridge university press.
- Logan, G. D. (1988). Toward an instance theory of automatization. Psychological Review, 95(4), 492–527. https://doi.org/https://doi.org/10.1037/0033-295X.95.4.492
- Lorentz, K., Gütschow, B., & Renner, F. (1999). Evaluation of a direct α-amylase assay using 2-chloro-4-nitrophenyl-α-D-maltotrioside.
- Luethi, M., Meier, B., & Sandi, C. (2008). Stress effects on working memory, explicit memory, and implicit memory for neutral and emotional stimuli in healthy men. Frontiers in Behavioral Neuroscience, 2, 5. https://doi.org/https://doi.org/10.3389/neuro.08.005.2008
- Miller, R., Plessow, F., Kirschbaum, C., & Stalder, T. (2013). Classification criteria for distinguishing cortisol responders from nonresponders to psychosocial stress: evaluation of salivary cortisol pulse detection in panel designs. Psychosomatic Medicine, 75(9), 832–840. https://doi.org/https://doi.org/10.1097/PSY.0000000000000002
- Moeller, B., & Frings, C. (2011). Remember the touch: Tactile distractors retrieve previous responses to targets. Experimental Brain Research, 214(1), 121–130. https://doi.org/https://doi.org/10.1007/s00221-011-2814-9
- Moeller, B., Rothermund, K., & Frings, C. (2012). Integrating the irrelevant sound: Grouping modulates the integration of irrelevant auditory stimuli into event files. Experimental Psychology, 59(5), 258–264. https://doi.org/https://doi.org/10.1027/1618-3169/a000151
- Moeller, B., & Frings, C. (2014). Attention meets binding: Only attended distractors are used for the retrieval of event files. Attention, Perception, & Psychophysics, 76(4), 959–978. https://doi.org/https://doi.org/10.3758/s13414-014-0648-9
- Moeller, B., & Frings, C. (2015). Distractor-response bindings in dual task scenarios. Visual Cognition, 23(4), 516–531. https://doi.org/https://doi.org/10.1080/13506285.2015.1041437
- Moeller, B., & Frings, C. (2017). Dissociation of binding and learning processes. Attention, Perception, & Psychophysics, 79(8), 2590–2605. https://doi.org/https://doi.org/10.3758/s13414-017-1393-7
- Moeller, B., & Frings, C. (2017). Overlearned responses hinder SR binding. Journal of Experimental Psychology: Human Perception and Performance, 43(1), 1. https://doi.org/https://doi.org/10.1037/xhp0000341
- Murray, E. A., Bussey, T. J., & Wise, S. P. (2000). Role of prefrontal cortex in a network for arbitrary visuomotor mapping. Experimental Brain Research, 133(1), 114–129. https://doi.org/https://doi.org/10.1007/s002210000406
- Olver, J. S., Pinney, M., Maruff, P., & Norman, T. R. (2015). Impairments of spatial working memory and attention following acute psychosocial stress. Stress and Health, 31(2), 115–123. https://doi.org/https://doi.org/10.1002/smi.2533
- Pasupathy, A., & Miller, E. K. (2005). Different time courses of learning-related activity in the prefrontal cortex and striatum. Nature, 433(7028), 873–876. https://doi.org/https://doi.org/10.1038/nature03287
- Plessow, F., Fischer, R., Kirschbaum, C., & Goschke, T. (2011). Inflexibly focused under stress: acute psychosocial stress increases shielding of action goals at the expense of reduced cognitive flexibility with increasing time lag to the stressor. Journal of Cognitive Neuroscience, 23(11), 3218–3227. https://doi.org/https://doi.org/10.1162/jocn_a_00024
- Polich, J. (2007). Updating P300: An integrative theory of P3a and P3b. Clinical Neurophysiology: Official Journal of the International Federation of Clinical Neurophysiology, 118(10), 2128–2148. https://doi.org/https://doi.org/10.1016/j.clinph.2007.04.019
- Roozendaal, B., McEwen, B.S., & Chattarji, S. (2009). Stress, memory and the amygdala. Nature Reviews Neuroscience, 10(6), 423–433. https://doi.org/https://doi.org/10.1038/nrn2651
- Russell, G., & Lightman, S. (2019). The human stress response. Nature Reviews Endocrinology, 15(9), 525–534. https://doi.org/https://doi.org/10.1038/s41574-019-0228-0
- Sänger, J., Bechtold, L., Schoofs, D., Blaszkewicz, M., & Wascher, E. (2014). The influence of acute stress on attention mechanisms and its electrophysiological correlates. Frontiers in Behavioral Neuroscience, 8, 353. https://doi.org/https://doi.org/10.3389/fnbeh.2014.00353
- Schwabe, L., Haddad, L., & Schachinger, H. (2008). HPA axis activation by a socially evaluated cold-pressor test. Psychoneuroendocrinology, 33(6), 890–895. https://doi.org/https://doi.org/10.1016/j.psyneuen.2008.03.001
- Schwabe, L., Joëls, M., Roozendaal, B., Wolf, O. T., & Oitzl, M. S. (2012). Stress effects on memory: An update and integration. Neuroscience & Biobehavioral Reviews, 36(7), 1740–1749. https://doi.org/https://doi.org/10.1016/j.neubiorev.2011.07.002
- Schwabe, L., & Wolf, O. T. (2013). Stress and multiple memory systems: From 'thinking' to 'doing'. Trends in Cognitive Sciences, 17(2), 60–68. https://doi.org/https://doi.org/10.1016/j.tics.2012.12.001
- Shackman, A., J., Maxwell, J.S., McMenamin, B.W., Greischar, L.L., & Davidson, R.J. (2011). Stress potentiates early and attenuates late stages of visual processing. The Journal of Neuroscience: The Official Journal of the Society for Neuroscience, 31(3), 1156–1161. https://doi.org/https://doi.org/10.1523/JNEUROSCI.3384-10.2011
- Singh, T., Moeller, B., Koch, I., & Frings, C. (2018). May I have your attention please: Binding of attended but response-irrelevant features. Attention, Perception, & Psychophysics, 80(5), 1143–1156. https://doi.org/https://doi.org/10.3758/s13414-018-1498-7
- Simonsohn, U. (2015). Small telescopes: Detectability and the evaluation of replication results. Psychological Science, 26(5), 559–569. https://doi.org/https://doi.org/10.1177/0956797614567341
- Wise, R. A. (2004). Dopamine, learning and motivation. Nature Reviews Neuroscience, 5(6), 483–494. https://doi.org/https://doi.org/10.1038/nrn1406
Appendix 1
Exclusion Criteria.
Participants were excluded if any of the following exclusion criteria applied participants who reported severe neurological or psychiatric disorders, participants who have been diagnosed with or have a family history of epilepsy or aneurysms, participants who reported dermatitis especially of the scalp or foot regions, metal implants in the head or upper arms, regular drug or regular steroid usage, or consumption of more than two units of alcohol per day, participants who reported regular usage of medication (e.g. cortison), participants who reported being pregnant or nursing at the time of testing, or who reported migraines, vascular anomalies, cochlear implants, tattoos in the head region, piercings in the head region (except eyes and nose), high or low blood pressure, Raynaud’s disease, cardio-vascular disease, pulmonary disease, metabolic disease, thrombosis, any other chronic disease, an syncope episode in the last 5 years, participation in a medical study within the last 6 months, problems in doing physical activity of medium difficulty (e.g. bicycling fast or uphill), or participants sensitive to cold.
Additionally, participants were instructed to only participate in the testing if they were in good health on the day of the testing. They were also instructed to avoid excessive alcohol consumption and prolonged exposure to the sun on the day before testing. They were instructed not to eat or drink anything 2 hours prior to the testing. They were also instructed to ensure that their upper body and feet are easily accessible, and to remove any tor rings or anklets before testing.