ABSTRACT
Many epidemiological studies have shown the beneficial effects of a largely plant-based diet, and the strong association between the consumption of a Mediterranean-type diet with healthy aging including a lower risk of cognitive decline. The Mediterranean diet is characterized by a high intake of olive oil, fruits and vegetables and is rich in dietary fiber and polyphenols – both of which have been postulated to act as important mediators of these benefits. Polyphenols are large molecules produced by plants to protect them from environmental threats and injury. When ingested by humans, as little as 5% of these molecules are absorbed in the small intestine with the majority metabolized by the gut microbiota into absorbable simple phenolic compounds. Flavan-3-ols, a type of flavonoid, contained in grapes, berries, pome fruits, tea, and cocoa have been associated with many beneficial effects on several risk factors for cardiovascular disease, cognitive function and brain regions involved in memory formation. Both preclinical and clinical studies suggest that these brain and heart benefits can be attributed to endothelial vascular effects and anti-inflammatory properties among others. More recently the gut microbiota has emerged as a potential modulator of the aging brain and intriguingly polyphenols have been shown to alter microbiota composition and be metabolized by different microbial species. However, there is a need for well controlled studies in large populations to identify predictors of response, particularly given the vast inter-individual variation of human gut microbiota.
Introduction
It is estimated that more than 2 billion people will be over 60 years by 2050 [Citation1] and this demographic shift will be accompanied by an increase in the prevalence of cognitive dysfunction and neurodegenerative disorders, ranging from mild cognitive impairment to more severe forms of neurocognitive disorders such as Alzheimer’s disease (AD) and Parkinson’s disease (PD) [Citation2]. To reduce the risk of developing neurodegenerative disorders, the World Health Organization has highlighted the need to understand the lifestyle factors underlying this trend. A predominantly plant-based diet offers several health benefits including reduction of disease risk, and promotion of healthy aging [Citation3–8]. The traditional Mediterranean diet is one of the best-studied examples of a predominantly plant-based diet [Citation9,Citation10]. Consuming this diet is positively associated with reduced risk of several pathologies, including major depressive disorder (MDD), AD, PD, and cardiovascular diseases [Citation11–16], as well as improved cognitive function [Citation17–20], based on epidemiological and observational studies. Regularly consuming the Mediterranean diet has also been associated with reduced microvascular brain damage [Citation21–23]. The Mediterranean-like DASH diet (Dietary Approaches to Stop Hypertension) has been associated with reduced cognitive decline in older subjects [Citation24–26] and has been reported to reduce several cardiovascular risk factors in clinical studies [Citation27–30]. Likewise, the MIND diet (Mediterranean-DASH Intervention for Neurodegenerative Delay) has been associated with larger total brain volume, better cognitive function, decreased risk of dementia, slower cognitive decline, and fewer symptoms of MDD [Citation17–20]. Even though correlational, these studies suggest that largely plant-based diets enhance brain function and may even improve cerebral vascular function [Citation31].
Historically, nutrition science has focused on reductionist accounts of essential nutrients needed for healthy aging, such as vitamins and minerals. However, in recent decades, the field has broadened its perspective to encompass the multifaceted roles of dietary bioactives, which are not considered essential nutrients but can play important roles in disease prevention and healthy aging. These bioactives, found in various plant-based foods, exert a range of beneficial effects, including antioxidant and anti-inflammatory properties. This shift highlights a more holistic approach to nutrition and acknowledges the importance of a varied diet rich in non-essential nutrients. Interestingly, the MIND diet maximizes nutrients with a positive effect on neurodegenerative disorders [Citation26], with an emphasis on berries. Indeed, regular consumption of berries is associated with a reduced risk of AD, PD, and with delayed cognitive decline [Citation32–34]. Higher consumption of polyphenols (PPs) contained in these berries has been proposed as one of the responsible factors. Fruits including apples, grapes, pears, berries, and cherries harbor up to 200–300 mg of PPs per 100 g of fresh weight [Citation34]. The scientific consensus is that PP are most likely responsible for the health benefits of these foods, but knowledge gaps remain regarding the types of PP that exert such effects, the optimal doses, and their mechanism(s) of action.
The overall goal of this review is to explore the role of the gut microbiota in the modulation of dietary PPs into bioactive compounds with the potential for protection from age-related decline and neurodegeneration.
Polyphenols
This is a large and diverse group of molecules with two significant roles in plant health: (1) defense mechanisms that respond to homeostatic perturbations such as drought, UV light, pests, and diseases [Citation35], and (2) as part of a signaling code between the root system of the plant and soil microbes in close proximity. In vitro studies have reported antioxidant properties of PPs but this is in fact a limited bioactivity when they are ingested by humans [Citation36]. Indeed, while PPs were initially endorsed for their antioxidant potential by the FDA in 2012, the endorsement was removed five years later. Even though various health benefits have been associated with the consumption of these molecules, they are not considered micronutrients as they have no intrinsic nutrient value [Citation37]. The richest sources of PPs are vegetables, fruits, tea, pome fruits, and some berries. The PP content in these foods can vary significantly due to factors like geographical location, climate, UV exposure, chemical fertilizers, fruit ripening, storage, processing, and cooking. For example, cooking adds complexity by thermal degradation of PPs, altering concentrations and bioavailability, and thus impacting the nutritional profile of plant-based foods [Citation38]. Over 8,000 PP compounds have been discovered in various plant species, and these are classified into four subclasses: phenolic acids (∼30% of all PP), stilbenes, lignans, and flavonoids (∼60% of all PP). The largest family of PP, the flavonoids, can be divided into six further subclasses: flavan-3-ols (also known as flavanols), flavonols, flavanones, flavones, anthocyanins, and isoflavones [Citation34]. Berries contain several subclasses of flavonoids including flavan-3-ols, anthocyanins, proanthocyanins, stilbenes, tannins, and non-flavonoids such as phenolic acids (for detailed reviews see [Citation39,Citation40]).
Anthocyanins are generally the major PPs in berries and have received the most research interest for their potential benefits to human health, which include neuroprotection [Citation41]. PP profiles in berries show huge variation depending on the species of berries, geographical location, and soil conditions, and PP profiles are also influenced by ripening levels, type of growing (for example conventionally grown vs regenerative organic), processing of foods, and storage conditions [Citation42], which have significant consequences on their health effects. Due to their large size, flavonoids are poorly absorbed in the small intestine (5–10%), reaching very low plasma levels following consumption, based on human bioavailability studies. Most PPs reach the ileum and colon where they interact with the gut microbiota resulting in extensive chemical modification of the parent compounds into small phenolic, absorbable metabolites [Citation43–45]. But they also cause changes to gut microbiota composition [Citation46,Citation47] and potentially alter its metabolic activity and functioning.
Animal studies have revealed that the gut microbiota influences brain structure, function, and behavior through several signaling pathways, including neural, neuroendocrine, and immune-mediated communication channels [Citation13,Citation48,Citation49]. Gut microbiota-generated metabolites and molecules from dietary, host, and de novo sources can indirectly influence brain function by modulating the mucosal barrier, the gut-associated immune system, and vascular health and function, or directly by modulating synaptic plasticity, neuroinflammation, oxidative stress, and Aβ pathology [Citation50]. Preclincial evidence suggests that the kynurenine pathway is able to modulate N-methyl-D-aspartate receptor function [Citation51,Citation52]. Activation of these receptors play an important role in excitotoxicity and neuroplasticity. Some of the potential neuroactive candidates include phenolic catabolites such as phenyl-γ-valerolactones derived from flavan-3-ol-containing foods [Citation53–58]. Phenyl-γ-valerolactones have also been used as a biomarker of flavan-3-ol intake. Thus, gut bacteria and the molecules they generate via the metabolism of PPs are crucial to understanding how PPs affect the brain. The last decade has seen a growing appreciation for the role of non-essential nutrient constituents or dietary bioactives in disease prevention and healthy aging [Citation59]. As an example, several dietary intervention studies have reported that the flavan-3-ol, epicatechin, plays a secondary role in the prevention of diabetes, and plays primary and secondary roles in the prevention of cardiovascular disease [Citation60]. However, the mechanisms of action underlying such health benefits remain incompletely understood.
This review will focus on the most studied family of PP, the flavonoids, presenting results of preclinical and clinical studies. The flavan-3-ols that reside within the family of flavonoids represent some of the more widely consumed PP, including epicatechin [Citation61]. Results of human observational studies and clinical trials that have investigated the associations between flavonoid intake and brain health, as well as the prevention of these PPs in the development of neurodegenerative disorders, are critically reviewed. Further, key mechanistic studies that describe and conceptualize the role of flavonoids in the brain-gut microbiome axis are discussed.
Preclinical evidence for biological mechanisms mediating brain health benefit
The health benefits of flavonoids in humans have been proposed to be primarily a result of gut microbial effects, vascular and endothelial effects, anti-inflammatory effects, and to a lesser degree antioxidant effects.
Indirect brain effects of flavonoids through modulation of vascular function
For healthy vascular function, endothelial cells produce a range of substances, including nitric oxide (NO) which plays an important vasodilatory role [Citation62]. Reduced availability of NO as a result of oxidative stress and free radicals causes endothelial dysfunction [Citation63]. Anthocyanins can activate endothelial NO synthase, leading to increased NO production, resulting in vasodilation which has been observed in in vitro and in vivo studies [Citation64,Citation65]. This is most likely due to the modulation of different signal transduction pathways, such as the adenosine monophosphate-activated protein kinase and the phosphatidylinositol 3-kinase/Akt pathways [Citation66]. In preclinical studies, flavonoids have demonstrated vasodilatory effects in the cardiovascular system [Citation67,Citation68], and vasorelaxant effects in the pulmonary artery [Citation69]. In clinical studies, the intake of flavonoids have been associated with a reduction in blood pressure [Citation70,Citation71], arterial stiffness [Citation71], and increased vasodilation [Citation70,Citation72]. Additionally, flavonoids have been shown to regulate the overproduction and activation of cytokines, modulate the gene expression of several pro-inflammatory molecules, and inhibit the activity of enzymes associated with inflammation [Citation73].
Indirect flavonoid effects on the gut-brain-axis mediated by the gut microbiota
The ability of flavonoids to influence gut microbiota composition, relative abundance, and functionality can potentially affect the brain through several gut-brain signaling pathways, including hormonal, neuronal, and immune-mediated channels () (reviewed in [Citation13]).
Figure 1. Gut interaction and health effects of polyphenols. The small intestine absorbs a limited amount of intact dietary polyphenols into the systemic circulation system where they probably exert a small transient antioxidant effect on the body and the brain. Most of the polyphenols ingested into the body interact with the gut microbiome (prebiotic, antibiotic effects) and go on to be metabolized into small, phenolic compounds. A quantity of these metabolites have exhibited beneficial effects on the brain and the cardiovascular system (see text for details).
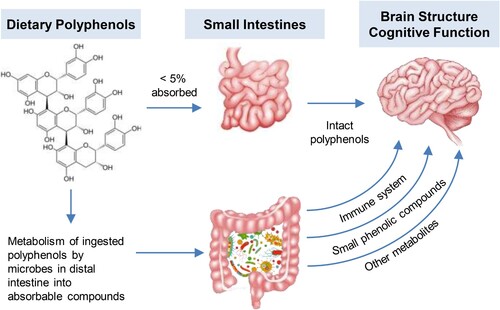
Several preclinical studies have demonstrated the anti-inflammatory effects of a range of flavonoids through the gut microbiota. For example, the administration of isoflavones [Citation74], flavan-3-ols [Citation75], and anthocyanins [Citation76] increased anti-inflammatory short-chain fatty acid (SCFA) levels in mice, which are generated by the microbial metabolism of microbiota accessible carbohydrates (MACs). The increase in the relative abundance of Akkermansia muciniphila in response to flavonoid administration was associated with enhanced intestinal barrier integrity and a reduction in gut-based and systemic inflammation in preclinical studies [Citation77–79]. Anthocyanin administration in healthy mice showed similar effects of microbial changes, which were associated with improved gut barrier function and a reduction in inflammation, including reduced brain inflammation through supplementation of Aronia berry [Citation76], acai berry [Citation80], or bilberry [Citation81]. Additional studies have demonstrated the neuroprotective effects of alterations in gut microbiota composition as a result of anthocyanin supplementation [Citation82,Citation83]. Moreover, the flavonoid, quercetin, has demonstrated anti-inflammatory properties [Citation84], resulting in significant reductions in systemic inflammatory markers IL-4 and IL-5 in the bronchoalveolar lavage fluid (BALF) in mouse models. Quercetin was also shown to block the NF-kB activation pathway [Citation85]. Rutin has been reported to reduce proinflammatory cytokines and decrease oxidative stress [Citation86,Citation87].
Kaempferol was shown to reduce oxidative stress and the inflammatory response following spinal cord injury in a rat model, by down-regulating the ROS-dependent MAKs-NF-kB signaling pathway [Citation88]. By inhibiting microglia activation in a rat model of cerebral ischemia and reperfusion, kaempferol was reported to reduce cerebral inflammation demonstrating both blood–brain barrier protective effects and anti-inflammatory effects [Citation89]. Catechin flavonoids have been reported to increase the relative abundance of the SCFA-producing bacterium Akkermansia in a mouse model [Citation90]. The anti-inflammatory properties of this PP have been reported in rodent studies, observing attenuation of IL-1 beta production by the inhibition of pro-IL-1B expression via downregulation of NF-kB p38 MAPK and TLR signaling [Citation91]. The catechin, epigallocat-echin-3-gallate (EGCG), has also been reported to attenuate neuroinflammation, specifically microglial inflammation, as well as neurotoxicity, via the inhibition of canonical NLRP3 and non-canonical caspase-11-dependent inflammasome activation via the TLR4/NF-kB pathway [Citation92].
Direct antioxidant effects of flavonoids in vitro
The direct antioxidant effects of flavonoids include the chelation of metal ions and the scavenging of free radicals from reactive oxygen species [Citation66,Citation93,Citation94] which have been observed in in vitro studies. This has resulted in the incorrect assumption that PPs exert their beneficial effects in humans through direct antioxidant effects. Indirect antioxidant effects include suppression of stress-related signaling pathways by modulation of ligand–receptor interactions such as tumor necrosis factor α (TNF- α) and its receptor [Citation95]. A significant reduction of oxidative DNA damage has been observed in preclinical studies with blueberry flavonoids, strongly indicating the occurrence of PP-mediated antioxidant activity [Citation96]. The size of the PP, and the absence of intestinal enzymes to break down the molecules into smaller, absorbable metabolites leads to poor absorption in the proximal small intestine. Only certain gut microbes perform this chemical transformation; thus, the generation and absorption of several phenolic acids occur with increasing microbial load towards the distal small intestine, particularly the colon. Microbial metabolism of PPs results in simple phenolic compounds that are suggested to have beneficial cardiovascular and brain effects, presumably mediated by effects on endothelium-dependent vasodilation, a reduction of blood pressure, inflammation, and platelet activation. Like other polyphenols, flavanols act as probiotics for several species in the microbial ecosystem in addition to the effects of their metabolites on the host. We hypothesize that similar to the fiber-induced increase in diversity and richness of the gut microbiome, eating a flavanol-rich diet will increase microbial diversity, including the increase in microbial strains that can metabolize them.
In summary, based on such mechanistic studies in animals, the evidence indicates that flavonoids may influence brain health through modulation of the gut microbiota, which may lead to increases in mucus and SCFA-producing gut microbes, improved gut barrier function, and a reduction in systemic and brain inflammation. Major limitations of the quoted studies include small sample size and the restriction to only male rodent animals. Future studies should be conducted in larger animal cohorts with different species and mixed genders to provide a more comprehensive understanding of the outcomes and to assess potential gender-specific effects. Although the quoted studies provide valuable insight into the potential effects of PPs on the gut microbiome and their potential therapeutic effects in treating cognitive decline and neurodegenerative disorders, translating animal studies to clinical human outcomes is often challenging, due to the disparities in gut microbial composition, metabolism, and responses to interventions. Mirroring the true complexity and variability of human physiology and gut microbiota may not be possible through mouse and/or rat models, while pigs may be a better animal model. Ultimately, well-controlled clinical trials are required to reproduce these findings in human cohorts and demonstrate clinically meaningful effects of PPs on the human gut microbiome and their subsequent impact on cognitive decline and neurodegenerative disorders.
Clinical evidence supporting the potential effect of PPs on cognitive function
The suggested daily consumption of PP is approximately 1 g/day [Citation97]. Several factors influence the daily intake of PP among individuals, such as dietary habits, geographical location, sex, age, and socio-economic background. A 2018 review found that total PP intake based on geographical location was highest in Japan (1500 mg/day) in comparison to North America and South America, and European countries (800 and 900 mg/day, respectively). Total flavonoid intake was greatest in Australia and Poland (600 mg/day), compared with lower daily intakes in other countries, for example, the USA and South America (200–400 mg/day) [Citation98]. The daily PP intake of the Mediterranean diet is approximately 664 mg/day, with flavonoids and phenolic acids being the primary subclasses (259 and 363 mg/day, respectively) [Citation99].
Nutrition is key in preventing and slowing cognitive decline [Citation100,Citation101]. Evidence from studies evaluating the effects of the MIND and DASH diets indicates the protective effects of PPs on cognitive decline [Citation17,Citation102,Citation103] and lower AD risk in both men and women [Citation104–106]. MIND concordance scores from zero to one were determined to evaluate the intake of PPs by the study participants. An annual clinical evaluation was conducted whereby foods from the previous 12 months were recorded. The low frequency of administration and the recall bias associated with this questionnaire, along with the self-report of diet, are significant limitations of this study which likely biased these results [Citation17,Citation102,Citation103]. Despite these limitations, PPs are likely important mediators of the reported dietary effects [Citation107–109], with a 42% increase in flavonoid intake (from 296.8 mg/day to >421 mg/day), specifically monomeric flavan-3-ols (or catechins), oligomeric flavan-3-ols (or procyanidins), and anthocyanins being associated with lower AD risk [Citation110]. Based on mechanistic studies in animal models, the observed PP effects are likely to be related to the anti-inflammatory and vasodilatory action of PP metabolites on the dentate gyrus and other brain regions [Citation111–113] implicated in AD pathogenesis [Citation114,Citation115]. Multimodal brain imaging, a biomarker of structural and functional brain integrity [Citation116] has contributed to our understanding of the neurobiology of cognitive function and disease progression in AD [Citation115,Citation117,Citation118].
Cognitive decline and neurodegenerative disorders are associated with functional and structural alterations in the hippocampus, in particular the dentate gyrus, anterior/posterior cingulate, insula, striatum, brainstem, default mode, and executive control networks [Citation119–122]. In a study that included stroke-free male and female participants from the Framingham Heart Study Offspring Cohort (n = 2086, mean age 60.6 years), white matter hyper-intensities volume, a measure of brain degeneration, was inversely associated with a 120.6% increase in total daily flavonoid consumption (>525 mg/day), including flavan-3-ols, monomeric flavan-3-ols (or catechins) and oligomeric flavan-3-ols (or procyanidins) [Citation123].
provides a list of some of the clinical studies supporting a potentially beneficial effect of PP on cognitive function. One PP intervention study conducted in 90 elderly individuals (aged 64–82 years old) with mild cognitive impairment showed that high cocoa flavan-3-ol intake (993 mg/day, 8 weeks) improved cognitive function, as measured by a verbal fluency test (VFT), Mini-Mental State Examination (MMSE), and Trail Making Test (TMT) A and B [Citation124]. Similarly, supplementation with cocoa flavonoids (900 mg/day, 3 months) in a healthy cohort of 34 male and female individuals aged 50 to 69 years old enhanced hippocampal dentate gyrus function in older adults. The authors proposed that this mechanism of mitigating age-related cognitive decline may be attributed to a specific augmentation in capillary density in the dentate gyrus [Citation125]. These effects are likely mediated by gut microbial-derived PP metabolites rather than the parent flavonoids.
Table 1. Description of the main findings of the clinical studies supporting a potential effect of PP on cognitive function.
The NU-AGE study profiled the gut microbiota in 612 elderly male and female subjects (aged 65–79 years) on a year-long PP-rich dietary intervention compared to the control group. Adherence to the NU-AGE diet was associated with increased short/branched-chain fatty acid production and lower production of secondary bile acids (BAs). In addition, overall specific microbiome alterations and lower inflammatory markers were positively associated with markers of lower frailty and improved cognitive function [Citation126].
In a longitudinal study, 16,010 female participants completed a food intake questionnaire every four years (these participants also participated in the Harvard Nurses’ Health study) [Citation32]. Questions included how often certain foods, such as blueberries and strawberries were consumed on a weekly basis. Through multiplication, the information was recalculated into a quantifiable amount of flavonoid intake. After 20 years, dietary patterns and cognitive tests were performed on the participants in two-year intervals. It was found that a higher intake of flavonoids (high flavonoid median of 684.1 mg/day, compared to low flavonoid median of 145.4 mg/day) through strawberries and blueberries was equivalent to approximately 1.5 to 2.5 years of delayed cognitive aging (after adjusting for income). The correlations observed between repeated measures of berry intake (p = 0.2–0.3) were modest, likely indicating genuine changes in berry consumption within individuals over time. These changes could be attributed to variations in berry availability by year and region. Even though performed in a large sample, one major limitation of this study is that the questionnaire was completed every four years, which is likely to introduce significant recall bias and results in less accurate or incomplete information due to participants’ reliance on memory over an extended period.
In a cross-sectional study involving 1003 Japanese male and female subjects older than 70 years, MMSE results showed that improved cognitive function was associated with higher consumption of green tea (>2 cups/day), which is high in catechins [Citation127]. In a randomized, double-blind, placebo-controlled trial involving 12,741 participants (aged 35–60 years) and spanning 13 years, intake of flavonoids, catechins, theaflavins, flavan-3-ols, and phenolic acids were associated with long-term language ability and better verbal memory, as assessed using Forward and Backward Digit Span, the RI-48 Cued Recall test, the TMT and phonemic and semantic fluency [Citation128]. A recent six-month double-blind randomized controlled trial of 131 participants (44, 42, and 45 participants in the wild blueberry group, placebo group, and reference group, respectively) aged 65 years and above showed that a flavonoid-rich wild blueberry intervention significantly improved the processing speed among the wild blueberry group. This improvement effectively restored their processing speed to a level on par with that of the reference group, as assessed by the Cambridge Neurological Test Automated Battery (CANTAB) and event-related potentials (ERP) [Citation129].
A study on flavonoid intake and its effects on cognitive function followed US female participants from the Harvard Nurses’ Health study from 2001–2006 (n = 49,493 women), and men from a health professionals follow-up study from 1986–2002 (n = 27,842) [Citation130]. Participants reported their dietary intake in seven semiquantitative food frequency questionnaires as well as their own cognitive changes. It was reported that lower rates of subjective cognitive decline were associated with self-reported consumption of several flavonoid-rich foods. However, longitudinal studies have their limitations, especially with respect to the validity of each person’s subjective evaluation of their dietary pattern and their cognitive function, the limited validity of food frequency questionnaires, as well as other confounding variables. These can include different concentrations of PPs actually consumed due to different foods containing varying concentrations. Those who eat more fruits and vegetables, or more PP-containing foods, may lead healthier lifestyles overall. Thus, despite the impressive number of participants, the results must be interpreted with caution and should not be misinterpreted as demonstrating causal effects between flavonoid intake and cognitive function.
Flavan-3-ols
Recent studies have replaced self-reported PP intake levels with blood levels of the flavan-3-ol metabolite phenyl-γ-valerolactone, providing for the first-time accurate estimates of PP intake. Cocoa flavan-3-ols have received attention for their effects on cognitive function in older adults. One study (n = 90, aged 61–85 years, males and females) conducted over a two-month period reported that high cocoa flavan-3-ol consumption (993 mg/day) reduced metrics of age-related cognitive dysfunction, compared to the low flavan-3-ol group (48 mg/day), as assessed through changes in systolic blood pressure, homeostatic model assessment for insulin resistance, and plasma isoprostane concentrations. Cognitive function was evaluated using the MMSE, the TMT A and B, and the VFT. MMSE scores found no difference between groups, however significant improvements (p < 0.0001) in TMT A and B and VFT scores were found in the high compared with the low flavan-3-ol group, suggesting improved cognitive function through test factors such as greater processing speed, executive function, spatial awareness, and motor skills. The authors suggested these cognitive improvements could be attributed to improvements in insulin sensitivity, blood pressure, insulin resistance (P < 0.0001, respectively), and lipid peroxidation (P = 0.001) observed throughout the study [Citation131]. Several mechanisms have been implicated in the antidiabetic effects of flavan-3-ols, including the glucose absorption rate in the gut, glucose peripheral uptake, glucose secretion, the modulation of beta cell function, the modulation of insulin secretion, and the modulation of the incretin [Citation132].
High cocoa flavon-3-ol consumption (770 mg/day, 12 weeks) in 211 healthy male and female individuals (50–75 years) improved hippocampal-dependent list-learning performance in participants with low dietary intake at baseline, compared to the placebo group [Citation61]. Blood samples were taken at the beginning and end of the intervention to test for phenyl-γ-valerolactone metabolites. The study also examined brain activity in a small subset of participants (n = 12) and observed that following cocoa flavan-3-ol consumption, the dentate gyrus was the only hippocampal region that showed an increase in basal metabolism. Another study also reported a similar finding in that intake of cocoa flavan-3-ols contributed to improved cognitive function associated with increased dentate gyrus function. Indeed, Brickman et al. found that daily consumption of 900 mg cocoa flavan-3-ol supplements for three months in 50–69-year-old male and female participants (n = 37) enhanced dentate gyrus function, indexed by cerebral blood volume-fMRI measurement and cognitive testing [Citation125].
Moreover, in a younger cohort (n = 18, aged 18–45 years) of male subjects, a high flavan-3-ol daily intake of 681.4 mg through supplementation resulted in not only greater, but faster cerebral tissue oxygenation, compared to the low flavan-3-ol group (4.1 mg/day). This study suggests that the flavan-3-ol-mediated increase in levels of circulating NO plays a role not only in improved peripheral endothelial function but also benefits cerebrovascular reactivity. Due to the small cohort of only male participants within this study, further analysis in a larger cohort with both male and female participants is needed to validate these results [Citation133]. Cerebrovascular reactivity (CVR) to CO2, mediated by the NO pathway, is widely accepted as a key biomarker of cerebrovascular health and has been closely associated with cognitive function in health and disease states. While widely accepted as a key biomarker of cerebrovascular health, the underlying mechanism of CVR is unclear as the blood-oxygen-level-dependent signal is affected by multiple parameters, including cerebral blood volume, cerebral blood flow, and oxygen metabolism [Citation134]. As reduced CVR may be associated with cognitive decline as a result of vascular dysfunction [Citation134], this study suggests that continued intake of flavan-3-ols may delay age-related decline in later life [Citation133]. Similarly, 5-(3’.3’-dihydroxyphenyl-γ-Valerolactone), a major flavan-3-ol metabolite associated with green tea intake, was reported to inhibit TNF-α-stimulated adhesion of monocytic cells in the range 7.5–30 μM to human umbilical vein endothelial cells [Citation135]. This suggests that metabolites of green tea PPs, specifically some phenyl-γ-valerolactones, exert anti-inflammatory effects.
Moreover, a randomized, double-blind, controlled clinical trial also conducted in young healthy male adults (n = 30, aged 18–45) showed that the physiological response to acute mental stress was reduced following a dose of 681.5 mg cocoa flavan-3-ol supplementation, compared to the low flavan-3-ol group (<4 mg). In this study, an 8-minute Paced-Auditory-Serial-Addition-Task was employed to induce mental stress, while blood pressure, forearm blood flow, cardiovascular activity, endothelial function, and brachial blood pressure were measured as physiological responses. Results showed improved peripheral blood flow during stress and associated prevention in stress-induced endothelial dysfunction, suggesting increased flavan-3-ol intake can attenuate stress-induced reduction in vascular integrity [Citation136].
In summary, recent well-controlled studies, using the biomarker phenyl-γ-valerolactone as a more accurate measure of flavanol intake have significantly improved the quality and validity of earlier studies using estimates based on food frequency questionnaires. This approach offers a more precise and objective means of assessing dietary flavanol consumption and its potential health effects, thus contributing to a more robust understanding of the relationships between flavanol intake and health outcomes. Even though the underlying mechanism of action remains unknown, based on preclinical studies, it may be due to an indirect effect of dietary flavan-3-ols increasing vasodilation and improvement of endothelial function [Citation137]. It has also been proposed that flavan-3-ol intake reduces the stress-induced inflammatory response thereby improving cognitive function, although this requires more research to verify [Citation137]. Further research is also needed to determine why flavan-3-ol effects were limited to the dentate gyrus, a very small brain region.
Quercetin
Quercetin, a flavonoid commonly found in tea, onions, grapes, and cranberries has been shown to be associated with a reduction in vascular inflammation and blood pressure. Supplementation given to 96 participants (42 male, 54 female) for six weeks with 150 mg of quercetin daily lowered the blood pressure of participants with a high risk of cardiovascular disease. No significant disparities between sex groups were seen following supplementation [Citation138]. It decreased systolic blood pressure by 2.9 mmHg (P < 0.01) in the subgroup of hypertensive subjects and by 3.7 mmHg (P < 0.001) in the subgroup of younger adults aged 25 to 50 years. A 500 mg daily dose of a quercetin supplement for ten weeks in women (n = 72) with type 2 diabetes resulted in a decrease in plasma levels of TNF-α and interleukin compared to the placebo group [Citation139], results that are consistent with preclinical studies [Citation84,Citation140–142]. Due to its anti-inflammatory effect, quercetin may be beneficial in conjunction with other treatments for neuroinflammatory and cardiovascular diseases. A recent study of 70 male participants aged 60 to 79 years showed that increased quercetin intake (50 mg/day) over a period of 24 weeks significantly reduced age-related cognitive decline. The authors speculated that this reduction was mediated by the improvement of depressive symptoms and increased motivation, compared to the placebo group. However, the mechanism by which quercetin-rich onion improves cognition remains unclear, although it may be a consequence of emotional improvement, as conducted by CADi2 (a dementia mass screening test) [Citation143].
Anthocyanins
Anthocyanins are a subset of flavonoids usually found in berries. Similar to cocoa flavan-3-ols, some studies [Citation144,Citation145] have reported that anthocyanins increase vasodilation and reduce systemic inflammation, two effects that may lead to improved cognitive function. Participants with high plasma levels of low-density lipoprotein cholesterol (n = 150 males, aged 40 to 65 years) who consumed a purified anthocyanin mixture (320 mg/d) twice daily for 24 weeks in a randomized, double-blind, controlled study showed decreases in serum levels of IL-1β soluble vascular cell adhesion molecule-1 (sVCAM-1) and high-sensitivity C-reactive protein (hsCRP) test. Reductions in these systemic inflammatory markers are changes associated with a lower risk of cardiovascular disease [Citation146].
Several controlled human studies have provided evidence to support the beneficial effect of regular blueberry consumption on cognitive function in older patients with cognitive decline. In a 12-week randomized-controlled study assessing cognitive outcomes in older adults (n = 49 male and females aged 70 years and over) with mild-to-moderate dementia, consuming 200 ml/day of cherry juice, resulted in improvements in verbal fluency, short-term memory, and long-term memory, as well as a reduction in systolic blood pressure, compared to the placebo group [Citation147]. Numerous studies have also reported that increased anthocyanin intake through blueberry supplementation is associated with improved cognitive function [Citation148]. A randomized controlled study involving 122 male and female adults (65 to 80 years) who consumed blueberry-rich extract supplements (1000 mg/day) over six months reported improvements in cognitive tasks related to working and episodic memory and decreased cardiovascular risk factors [Citation149]. A randomized, placebo-controlled study over 16 weeks in male and female adults (aged 65 years and over) showed that visual-spatial memory and semantic access were significantly improved following blueberry supplementation. Trends in increased processing speed were also identified but these findings were non-significant (p = 0.08) [Citation150]. Consumption of blueberries (269 mg/day of anthocyanin) for 16 weeks in an elderly male and female cohort (n = 16, aged 68 to 92 years) resulted in greater activation in the left pre-central gyrus, left middle frontal gyrus, and left inferior parietal lobe in participants when performing a task that involves the working memory as indexed by fMRI imaging and compared with control participants [Citation151]. A six-month randomized controlled trial in 133 participants with mild cognitive impairment aged 65–80 years investigating blueberry consumption (35 grams of powdered sachets/day?) on serum uric acids concentrations found that significant sex-specific differences prevailed during the study, whereby notable disparities between the placebo and blueberry groups between the three-month and six-month intervals were exclusively observed in male participants and not in their female counterparts, thus, highlighting that future studies should include a sufficient sample size that is large enough for analysis to be stratified by gender [Citation152].
Catechins
Catechins are found in apples, persimmons, berries, tea, and cocoa. Epigallocatechin gallate, commonly found in green tea, is the most researched catechin [Citation153]. It is thought that the metabolites derived from EGCG may contribute to the health benefits associated with catechins, but few studies have investigated the independent function of catechins in humans in terms of cognitive function and reductions in inflammation.
Three months of daily green tea consumption containing 227 mg catechins in 12 elderly, two male and ten female, participants (mean age, 88 years) with cognitive dysfunction was associated with improved cognitive performance, as measured by MMSE-J score changes [Citation154]. However, the study is limited by its small sample size (n = 12) and should be replicated in a larger cohort for more reliable results. A meta-analysis of 70 male and female participants in 13 randomized controlled trials on the effects of catechin intake through green tea consumption improved blood pressure, particularly having a greater effect in those with systolic blood pressure ≥ 130 mmHg [Citation155]. This is thought to be due to vasodilation induced by increasing plasma NO concentration [Citation155]. Thus, catechins may also improve cognitive function through vasodilation, reduction in arterial blood pressure, and anti-inflammatory effects.
Clinical studies to date have offered valuable insights into the therapeutic ability of PPs in treating cognitive decline and neurodegenerative disorders. However, although these findings are encouraging, there is still a need for more robust and rigorous randomized controlled trials in larger cohorts to replicate and solidify the therapeutic potential of PPs, as well as pave the way for more targeted interventions to combat cognitive neurodegeneration. Additionally, investigating sex-based differences in PP intervention throughout cohorts of future studies may provide further insight into the unique needs of both men and women, enhancing the effectiveness and precision of therapeutic approaches for cognitive decline and neurodegenerative disorders.
The gut microbiome
The gut microbiota refers to the microbial groups associated with the human gut, while the term microbiome refers to the catalog of these microbes and their genes. Until recently, the potential benefits of PP intake on the brain have largely been related to their antioxidant effects observed in in vitro systems [Citation156–159]. Both in vitro assays with human gut microbiota and in vivo preclinical and clinical studies have demonstrated the ways in which PPs can modulate gut microbes to promote the growth of beneficial bacteria while inhibiting the growth of pathogenic bacteria [Citation160]. Some bacteria that have been identified in the metabolization of polyphenols are Eubacterium ramulus, Bifidobacterium spp., and Flavonifractor plautii [Citation161]. Based on inter-individual variations in the abundance of microbial taxa able to metabolize PPs, different metabotypes have been identified [Citation162–164]. For example, Eubacterium is associated with the metabolization of flavonoids, while Lactobacillus and Bifidobacterium release hydroxycinnamic acids within the colon [Citation161]. Ma et al. conducted a meta-analysis and systematic review revealing that health-promoting Lactobacillus and Bifidobacterium abundance significantly increased by 220% and 56%, respectively, and Clostridium pathogenic species were decreased in abundance following polyphenol supplementation [Citation165]. It is also proposed that the phytoactive metabolites resulting from the consumption of flavonoids, following their breakdown within the gut microbiome, engage with cellular and molecular targets (such as signaling pathways). This interaction is believed to enhance neuronal connectivity and facilitate improved blood circulation in the brain, including vascular and peripheral flow, particularly when in conjunction with exercise [Citation166].
Current evidence suggests a role of the gut microbiome in central nervous system (CNS) disorders, with a particular focus on the brain-gut microbiome (BGM) system playing a distinct function in neurodegenerative processes [Citation167–171]. The realization that the microbiota could be targeted for modulating brain function across the lifespan has been a complete paradigm shift in neuroscience. Various factors that influence the composition of the microbiota also modulate brain function and behavior [Citation172]. A human study carried out on 63 healthy adults aged 67–83 years found a correlation between cognitive performance (spatial working memory and paired-associate learning measured by (ERP and CANTAB) and alpha diversity of gut microbiota. Furthermore, the research postulated that diminished cognitive performance (i.e. as an indicator of cognitive impairment) was associated with reduced diversity in the gut microbiome [Citation173]. In brain aging, there is a growing appreciation of the role of the microbiome, although this is still largely restricted to animal studies [Citation174–176]. It is proposed that many of the beneficial effects of nutrition on brain health may be mediated by microbially generated metabolites [Citation48,Citation172,Citation176]. For example, phenyl-γ-valerolactones produced exclusively by the gut microbiota from flavan-3-ol-rich foods exert anti- β-amyloid oligomer activity and reduce memory deterioration, as well as neuroinflammation, in vivo [Citation55]. Likewise, xanthohumol, quercetin, and phlorotannin extract reduced anxiety and depressive symptoms in a rat model. In this study, the phenolic-derived gut microbial metabolite, xanthohumol, profoundly altered microbial diversity and composition, and improved brain-derived neurotrophic factor plasma levels [Citation177]. Similarly, a preclinical study showed that amyloid-beta load was reduced by urolithin A, a gut metabolite produced from the metabolism of ellagic acid. This was shown to improve spatial memory, exploratory behavior, and fear response in mice [Citation178]. Additionally, S-equol, a phenolic-derived gut metabolite of soy isoflavone daidzein, has shown therapeutic evidence in preclinical and some small-scale clinical studies of targeting arterial stiffness, tau phosphorylation, reducing amyloid-beta induced neurotoxicity, as well as having antioxidant and anti-inflammatory effects [as reviewed in [Citation179]].
Conclusions
Largely plant-based diets such as the traditional Mediterranean diet, and the related DASH and MIND diets have been associated with a wide range of brain and behavioral, cardiovascular, and metabolism-related health benefits based on a large body of epidemiological evidence (reviewed in [Citation13]). However, future well-controlled and strategically designed intervention studies are essential to unequivocally identify a causal role for specific dietary components and these benefits for PP. While many factors may be involved, the main mediators are likely to be dietary fiber and PPs and their interactions with the gut microbiota and the gut-associated immune system. PPs and fiber are both large molecules that require microbial metabolic transformation into smaller molecules for absorption in the distal, small, and large intestines. The beneficial effects of different members of the flavonoid family of PPs on cognitive function have been demonstrated in a number of human largely epidemiological studies and some have identified the brain changes underlying these benefits [Citation41,Citation61,Citation125,Citation127,Citation128,Citation131,Citation147,Citation151,Citation154,Citation180]. The mechanisms responsible for flavonoid-associated benefits in brain function, as well as cognitive and behavioral parameters, have been studied mainly in animal models. These include indirect brain effects involving vascular, endothelial, and immune activation in the brain, many of which may be mediated by microbiome-related immune signals, such as inflammatory cytokines, anti-inflammatory SCFAs, lipopolysaccharides, and microbe-associated molecular patterns. In addition, a number of neurotransmitters and hormones affecting the brain are either generated by or under partial control of certain gut microbes. Examples of microbe-derived neuroactive molecules include tryptophan metabolites, kynurenine, indoles, and certain secondary BAs. However, the number and amount of these neuroactive and hormonal microbial metabolites reaching the brain show significant inter-individual variability.
Furthermore, limitations remain as the great majority of existing studies fail to measure the great effect that confounding factors may have on PP concentrations. When conducting global intervention studies, it is essential to account for the diverse food compositions and the effect that parameters such as cooking, pesticides, food ripening, climate, etc. have on the overall results of the study. Thus, knowing the systemic concentration of biomarkers, such as the flavan-3-ol metabolite phenyl- γ- valerolactone or hippuric acid is currently the only reliable way to minimize these confounders [Citation181]. Additionally, human studies directly measuring the strains of bacteria present, such as Bifidobacteria, Lactobacillus, Eubacterium, and Akkermansia, should be conducted to investigate their modulatory effects on the impact of dietary PPs on cognitive benefits. Now that scientists appreciate the essential role the gut microbiota plays in transforming ingested PPs into absorbable metabolites, future high-quality controlled clinical trials that take inter-individual differences in gut microbiota composition into account should help unravel how this class of botanicals may play significant roles in brain health.
Disclosure statement
No potential conflict of interest was reported by the author(s).
Data availability statement
All data used in this manuscript is publicly available and referenced throughout.
Additional information
Funding
References
- United Nations. Department of economic and social affairs, population division (2013). World Population Ageing; 2013.
- Emmady PD, Tadi P. Dementia. In StatPearls; StatPearls Publishing: Treasure Island (FL); 2022.
- Kanner J. Dietary advanced lipid oxidation endproducts are risk factors to human health. Mol Nutr Food Res. 2007;51(9):1094–101. doi:10.1002/mnfr.200600303.
- Kastorini C-M, Milionis HJ, Esposito K, Giugliano D, Goudevenos JA, Panagiotakos DB. The effect of Mediterranean diet on metabolic syndrome and its components. J Am Coll Cardiol. 2011;57(11):1299–313. doi:10.1016/j.jacc.2010.09.073.
- Khalili H, Håkansson N, Chan SS, Chen Y, Lochhead P, Ludvigsson JF, et al. Adherence to a Mediterranean diet Is associated with a lower risk of later-onset Crohn’s disease: results from two large prospective cohort studies. Gut. 2020;69(9):1637–44. doi:10.1136/gutjnl-2019-319505.
- Kim H, Caulfield LE, Garcia-Larsen V, Steffen LM, Coresh J, Rebholz CM. Plant-based diets Are associated With a lower risk of incident cardiovascular disease, cardiovascular disease mortality, and all-cause mortality in a general population of middle-aged adults. J Am Heart Assoc. 2019;8(16). doi:10.1161/JAHA.119.012865.
- Kojima G, Avgerinou C, Iliffe S, Walters K. Adherence to Mediterranean diet reduces incident frailty risk: systematic review and meta-analysis. J Am Geriatr Soc. 2018;66(4):783–8. doi:10.1111/jgs.15251.
- Willett W, Sacks F, Trichopoulou A, Drescher G, Ferro-Luzzi A, Helsing E, Trichopoulos D. Mediterranean diet pyramid: a cultural model for healthy eating. Am J Clin Nutr. 1995;61(6):1402S–6S. doi:10.1093/ajcn/61.6.1402S.
- Scarmeas N, Anastasiou CA, Yannakoulia M. Nutrition and prevention of cognitive impairment. Lancet Neurol. 2018;17(11):1006–15. doi:10.1016/S1474-4422(18)30338-7.
- Valls-Pedret C, Sala-Vila A, Serra-Mir M, Corella D, de la Torre R, Martínez-González MÁ, et al. Mediterranean diet and age-related cognitive decline. JAMA Intern Med. 2015;175(7):1094. doi:10.1001/jamainternmed.2015.1668.
- Coelho-Júnior HJ, Trichopoulou A, Panza F. Cross-sectional and longitudinal associations between adherence to Mediterranean diet with physical performance and cognitive function in older adults: a systematic review and meta-analysis. Ageing Res Rev. 2021;70:101395. doi:10.1016/j.arr.2021.101395.
- Gu Y, Brickman AM, Stern Y, Habeck CG, Razlighi QR, Luchsinger JA, et al. Mediterranean diet and brain structure in a multiethnic elderly cohort. Neurology. 2015;85(20):1744–51. doi:10.1212/WNL.0000000000002121.
- Horn J, Mayer DE, Chen S, Mayer EA. Role of diet and its effects on the Gut microbiome in the pathophysiology of mental disorders. Transl Psychiatry. 2022;12(1):164. doi:10.1038/s41398-022-01922-0.
- Lourida I, Soni M, Thompson-Coon J, Purandare N, Lang IA, Ukoumunne OC, Llewellyn DJ. Mediterranean diet, cognitive function, and dementia. Epidemiology. 2013;24(4):479–89. doi:10.1097/EDE.0b013e3182944410.
- Sofi F, Macchi C, Abbate R, Gensini GF, Casini A. Mediterranean diet and health status: an updated meta-analysis and a proposal for a literature-based adherence score. Public Health Nutr. 2014;17(12):2769–82. doi:10.1017/S1368980013003169.
- Valls-Pedret C, Lamuela-Raventós RM, Medina-Remón A, Quintana M, Corella D, Pintó X, et al. Polyphenol-Rich foods in the Mediterranean diet are associated with better cognitive function in elderly subjects at high cardiovascular risk. J Alzheimer’s Dis. 2012;29(4):773–82. doi:10.3233/JAD-2012-111799.
- Morris MC, Tangney CC, Wang Y, Sacks FM, Barnes LL, Bennett DA, Aggarwal NT. Mind diet slows cognitive decline with aging. Alzheimer’s Dementia. 2015;11(9):1015–22. doi:10.1016/j.jalz.2015.04.011.
- Cherian L, Wang Y, Holland T, Agarwal P, Aggarwal N, Morris MC. Dash and Mediterranean-dash intervention for neurodegenerative delay (MIND) diets Are associated With fewer depressive symptoms over time. The J Gerontology: Ser A. 2021;76(1):151–6. doi:10.1093/gerona/glaa044.
- Melo van Lent D, O’Donnell A, Beiser AS, Vasan RS, DeCarli CS, Scarmeas N, et al. Mind diet adherence and cognitive performance in the framingham heart study. J Alzheimer’s Dis. 2021;82(2):827–39. doi:10.3233/JAD-201238.
- Morris MC, Tangney CC, Wang Y, Sacks FM, Bennett DA, Aggarwal NT. Mind diet associated with reduced incidence of Alzheimer’s disease. Alzheimer’s Dementia. 2015;11(9):1007–14. doi:10.1016/j.jalz.2014.11.009.
- Gardener H. Mediterranean diet and white matter hyperintensity volume in the Northern Manhattan study. Arch Neurol. 2012;69(2):251. doi:10.1001/archneurol.2011.548.
- Luciano M, Corley J, Cox SR, Valdés Hernández MC, Craig LCA, Dickie DA, et al. Mediterranean-Type diet and brain structural change from 73 to 76 years in a Scottish Cohort. Neurology. 2017;88(5):449–55. doi:10.1212/WNL.0000000000003559.
- Scarmeas N, Luchsinger JA, Stern Y, Gu Y, He J, DeCarli C, et al. Mediterranean diet and magnetic resonance imaging–assessed cerebrovascular disease. Ann Neurol. 2011;69(2):257–68. doi:10.1002/ana.22317.
- MillerIII E, Erlinger T, Sacks F, Svetkey L, Charleston J, Lin P, et al. A dietary pattern that lowers oxidative stress increases antibodies to oxidized LDL: results from a randomized controlled feeding study. Atherosclerosis. 2005;183(1):175–82. doi:10.1016/j.atherosclerosis.2005.04.001.
- Smith PJ, Blumenthal JA, Babyak MA, Craighead L, Welsh-Bohmer KA, Browndyke JN, et al. Effects of the dietary approaches to stop hypertension diet, exercise, and caloric restriction on Neurocognition in overweight adults With high blood pressure. Hypertension. 2010;55(6):1331–8. doi:10.1161/HYPERTENSIONAHA.109.146795.
- Tangney CC, Li H, Wang Y, Barnes L, Schneider JA, Bennett DA, Morris MC. Relation of DASH- and Mediterranean-like dietary patterns to cognitive decline in older persons. Neurology. 2014;83(16):1410–6. doi:10.1212/WNL.0000000000000884.
- Appel LJ, Moore TJ, Obarzanek E, Vollmer WM, Svetkey LP, Sacks FM, et al. A clinical trial of the effects of dietary patterns on blood pressure. N Engl J Med. 1997;336(16):1117–24. doi:10.1056/NEJM199704173361601.
- Azadbakht L, Surkan PJ, Esmaillzadeh A, Willett WC. The dietary approaches to stop hypertension eating plan affects C-reactive protein, coagulation abnormalities, and hepatic function tests among Type 2 diabetic patients. J Nutr. 2011;141(6):1083–8. doi:10.3945/jn.110.136739.
- Blumenthal JA, Babyak MA, Sherwood A, Craighead L, Lin P-H, Johnson J, et al. Effects of the dietary approaches to stop hypertension diet alone and in combination With exercise and caloric restriction on insulin sensitivity and lipids. Hypertension. 2010;55(5):1199–205. doi:10.1161/HYPERTENSIONAHA.109.149153.
- Gadgil MD, Appel LJ, Yeung E, Anderson CAM, Sacks FM, Miller ER. The effects of carbohydrate, unsaturated Fat, and protein intake on measures of insulin sensitivity. Diabetes Care. 2013;36(5):1132–7. doi:10.2337/dc12-0869.
- Martínez-Lapiscina EH, Clavero P, Toledo E, Estruch R, Salas-Salvadó J, San Julián B, et al. Mediterranean diet improves cognition: the PREDIMED-NAVARRA randomised trial. J Neurol Neurosurg Psychiatry. 2013;84(12):1318–25. doi:10.1136/jnnp-2012-304792.
- Devore EE, Kang JH, Breteler MMB, Grodstein F. Dietary intakes of berries and flavonoids in relation to cognitive decline. Ann Neurol. 2012;72(1):135–43. doi:10.1002/ana.23594.
- Gao X, Cassidy A, Schwarzschild MA, Rimm EB, Ascherio A. Habitual intake of dietary flavonoids and risk of Parkinson disease. Neurology. 2012;78(15):1138–45. doi:10.1212/WNL.0b013e31824f7fc4.
- Pandey KB, Rizvi SI. Plant polyphenols as dietary antioxidants in human health and disease. Oxid Med Cell Longev. 2009;2(5):270–8. doi:10.4161/oxim.2.5.9498.
- Beckman CH. Phenolic-Storing cells: keys to programmed cell death and periderm formation in wilt disease resistance and in general defence responses in plants? Physiol Mol Plant Pathol. 2000;57(3):101–10. doi:10.1006/pmpp.2000.0287.
- Cory H, Passarelli S, Szeto J, Tamez M, Mattei J. The role of polyphenols in human health and food systems: a mini-review. Front Nutr. 2018: 5. doi:10.3389/fnut.2018.00087.
- Mena P, Calani L, Bruni R, Del Rio D. Bioactivation of high-molecular-weight polyphenols by the Gut microbiome. In: Diet-Microbe interactions in the Gut. Elsevier; 2015. p. 73–101. doi:10.1016/B978-0-12-407825-3.00006-X.
- Polia F, Pastor-Belda M, Martínez-Blázquez A, Horcajada M-N, Tomás-Barberán FA, García-Villalba R. Technological and biotechnological processes To enhance the bioavailability of dietary (Poly)Phenols in humans. J Agric Food Chem. 2022;70(7):2092–107. doi:10.1021/acs.jafc.1c07198.
- Neveu V, Perez-Jimenez J, Vos F, Crespy V, du Chaffaut L, Mennen L, et al. Phenol-explorer: an online comprehensive database on polyphenol contents in foods. Database. 2010;2010:bap024–bap024. doi:10.1093/database/bap024.
- Szajdek A, Borowska EJ. Bioactive compounds and health-promoting properties of berry fruits: a review. Plant Foods Hum Nutr. 2008;63(4):147–56. doi:10.1007/s11130-008-0097-5.
- Gonçalves AC, Nunes AR, Falcão A, Alves G, Silva LR. Dietary effects of anthocyanins in human health: a comprehensive review. Pharmaceuticals. 2021;14(7):690. doi:10.3390/ph14070690.
- Foito A, McDougall GJ, Stewart D. Evidence for health benefits of berries. In: Annual plant reviews online. Wiley; 2018. p. 105–48. doi:10.1002/9781119312994.apr0600.
- de Ferrars RM, Czank C, Zhang Q, Botting NP, Kroon PA, Cassidy A, Kay CD. The pharmacokinetics of anthocyanins and their metabolites in humans. Br J Pharmacol. 2014;171(13):3268–82. doi:10.1111/bph.12676.
- Mena P, Bresciani L, Brindani N, Ludwig IA, Pereira-Caro G, Angelino D, et al. Phenyl-γ-Valerolactones and phenylvaleric acids, the main colonic metabolites of flavan-3-Ols: synthesis, analysis, bioavailability, and bioactivity. Nat Prod Rep. 2019;36(5):714–52. doi:10.1039/C8NP00062J.
- Williamson G, Clifford MN. Colonic metabolites of berry polyphenols: The missing link to biological activity? Br J Nutr. 2010;104(S3):S48–66. doi:10.1017/S0007114510003946.
- Istas G, Wood E, Le Sayec M, Rawlings C, Yoon J, Dandavate V, et al. Effects of Aronia Berry (Poly)Phenols on vascular function and Gut Microbiota: a double-blind randomized controlled trial in adult Men. Am J Clin Nutr. 2019;110(2):316–29. doi:10.1093/ajcn/nqz075.
- Vendrame S, Guglielmetti S, Riso P, Arioli S, Klimis-Zacas D, Porrini M. Six-week consumption of a wild blueberry powder drink increases Bifidobacteria in the human Gut. J Agric Food Chem. 2011;59(24):12815–20. doi:10.1021/jf2028686.
- Cryan JF, O’Riordan KJ, Cowan CSM, Sandhu KV, Bastiaanssen TFS, Boehme M, et al. The microbiota-Gut-Brain Axis. Physiol Rev. 2019;99(4):1877–2013. doi:10.1152/physrev.00018.2018.
- Needham BD, Kaddurah-Daouk R, Mazmanian SK. Gut microbial molecules in behavioural and neurodegenerative conditions. Nat Rev Neurosci. 2020;21(12):717–31. doi:10.1038/s41583-020-00381-0.
- Mayer EA, Nance K, Chen S. The Gut–brain axis. Annu Rev Med. 2022;73(1):439–53. doi:10.1146/annurev-med-042320-014032.
- Forrest CM, Khalil OS, Pisar M, Darlington LG, Stone TW. Prenatal inhibition of the Tryptophan–Kynurenine pathway alters synaptic plasticity and protein expression in the Rat hippocampus. Brain Res. 2013;1504:1–15. doi:10.1016/j.brainres.2013.01.031.
- Savitz J. The kynurenine pathway: a finger in every Pie. Mol Psychiatry. 2020;25(1):131–47. doi:10.1038/s41380-019-0414-4.
- Esteban-Fernández A, Rendeiro C, Spencer JPE, del Coso DG, de Llano MDG, Bartolomé B, et al. Neuroprotective effects of selected microbial-derived phenolic metabolites and aroma compounds from wine in human SH-SY5Y neuroblastoma cells and their putative mechanisms of action. Front Nutr. 2017: 4. doi:10.3389/fnut.2017.00003.
- Figueira I, Garcia G, Pimpão RC, Terrasso AP, Costa I, Almeida AF, et al. Polyphenols journey through blood-brain barrier towards neuronal protection. Sci Rep. 2017;7(1):11456. doi:10.1038/s41598-017-11512-6.
- Ruotolo R, Minato I, La Vitola P, Artioli L, Curti C, Franceschi V, et al. Flavonoid-derived human Phenyl-γ-Valerolactone metabolites selectively detoxify amyloid-β oligomers and prevent memory impairment in a mouse model of Alzheimer’s disease. Mol Nutr Food Res. 2020;64(5). doi:10.1002/mnfr.201900890.
- Wang J, Hodes GE, Zhang H, Zhang S, Zhao W, Golden SA, et al. Epigenetic modulation of inflammation and synaptic plasticity promotes resilience against stress in mice. Nat Commun. 2018;9(1):477. doi:10.1038/s41467-017-02794-5.
- Westfall S, Caracci F, Zhao D, Wu Q, Frolinger T, Simon J, et al. Microbiota metabolites modulate the T helper 17 to regulatory T cell (Th17/Treg) imbalance promoting resilience to stress-induced anxiety- and depressive-like behaviors. Brain Behav Immun. 2021;91:350–68. doi:10.1016/j.bbi.2020.10.013.
- Yamasaki TR, Ono K, Ho L, Pasinetti GM. Gut microbiome-modified polyphenolic compounds inhibit α-synuclein seeding and spreading in α-synucleinopathies. Front Neurosci. 2020: 14. doi:10.3389/fnins.2020.00398.
- Ottaviani JI, Heiss C, Spencer JPE, Kelm M, Schroeter H. Recommending flavanols and procyanidins for cardiovascular health: revisited. Mol Aspects Med. 2018;61:63–75. doi:10.1016/j.mam.2018.02.001.
- Ottaviani JI, Borges G, Momma TY, Spencer JPE, Keen CL, Crozier A, et al. The metabolome of [2-14C](−)-epicatechin in humans: implications for the assessment of efficacy, safety and mechanisms of action of polyphenolic bioactives. Sci Rep. 2016;6(1):29034. doi:10.1038/srep29034.
- Sloan RP, Wall M, Yeung L-K, Feng T, Feng X, Provenzano F, et al. Insights into the role of diet and dietary flavanols in cognitive aging: results of a randomized controlled trial. Sci Rep. 2021;11(1):3837. doi:10.1038/s41598-021-83370-2.
- Steven S, Frenis K, Oelze M, Kalinovic S, Kuntic M, Bayo Jimenez MT, et al. Vascular inflammation and oxidative stress: major triggers for cardiovascular disease. Oxid Med Cell Longev. 2019;2019:1–26. doi:10.1155/2019/7092151.
- Cyr AR, Huckaby LV, Shiva SS, Zuckerbraun BS. Nitric oxide and endothelial dysfunction. Crit Care Clin. 2020;36(2):307–21. doi:10.1016/j.ccc.2019.12.009.
- Iwasaki-Kurashige K, Loyaga-Rendon RY, Matsumoto H, Tokunaga T, Azuma H. Possible mediators involved in decreasing peripheral vascular resistance with blackcurrant concentrate (BC) in hind-limb perfusion model of the Rat. Vascul Pharmacol. 2006;44(4):215–23. doi:10.1016/j.vph.2005.12.001.
- Zhu Y, Xia M, Yang Y, Liu F, Li Z, Hao Y, et al. Purified anthocyanin supplementation improves endothelial function via NO-CGMP activation in hypercholesterolemic individuals. Clin Chem. 2011;57(11):1524–33. doi:10.1373/clinchem.2011.167361.
- Mozos I, Flangea C, Vlad DC, Gug C, Mozos C, Stoian D, et al. Effects of anthocyanins on vascular health. Biomolecules. 2021;11(6):811. doi:10.3390/biom11060811.
- Al-Numair KS, Chandramohan G, Veeramani C, Alsaif MA. Ameliorative effect of Kaempferol, a flavonoid, on oxidative stress in Streptozotocin-induced diabetic rats. Redox Rep. 2015;20(5):198–209. doi:10.1179/1351000214Y.0000000117.
- Al-Numair KS, Veeramani C, Alsaif MA, Chandramohan G. Influence of Kaempferol, a flavonoid compound, on membrane-bound ATPases in Streptozotocin-induced diabetic rats. Pharm Biol. 2015;53(9):1372–8. doi:10.3109/13880209.2014.982301.
- Mahobiya A, Singh TU, Rungsung S, Kumar T, Chandrasekaran G, Parida S, Kumar D. Kaempferol-Induces Vasorelaxation via endothelium-independent pathways in Rat isolated pulmonary artery. Pharmacol Rep. 2018;70(5):863–74. doi:10.1016/j.pharep.2018.03.006.
- Heiss C, Jahn S, Taylor M, Real WM, Angeli FS, Wong ML, et al. Improvement of endothelial function with dietary flavanols is associated With mobilization of circulating Angiogenic cells in patients with coronary artery disease. J Am Coll Cardiol. 2010;56(3):218–24. doi:10.1016/j.jacc.2010.03.039.
- Heiss C, Sansone R, Karimi H, Krabbe M, Schuler D, Rodriguez-Mateos A, et al. Impact of Cocoa Flavanol intake on Age-dependent vascular stiffness in healthy men: a randomized, controlled, double-masked trial. Age (Omaha). 2015;37(3):56. doi:10.1007/s11357-015-9794-9.
- Silva H, Lopes NMF. Cardiovascular effects of Caffeic Acid and its derivatives: a comprehensive review. Front Physiol. 2020: 11. doi:10.3389/fphys.2020.595516.
- Al-Khayri JM, Sahana GR, Nagella P, Joseph BV, Alessa FM, Al-Mssallem MQ. Flavonoids as potential anti-inflammatory molecules. A review. Molecules. 2022;27(9):2901. doi:10.3390/molecules27092901.
- Chen Q, Wang B, Wang S, Qian X, Li X, Zhao J, et al. Modulation of the Gut microbiota structure with probiotics and Isoflavone alleviates metabolic disorder in ovariectomized mice. Nutrients. 2021;13(6):1793. doi:10.3390/nu13061793.
- Li J, Chen C, Yang H, Yang X. Tea polyphenols regulate Gut microbiota dysbiosis induced by antibiotic in Mice. Food Res Int. 2021;141:110153. doi:10.1016/j.foodres.2021.110153.
- Liu X, Martin DA, Valdez JC, Sudakaran S, Rey F, Bolling BW. Aronia Berry polyphenols have matrix-dependent effects on the Gut microbiota. Food Chem. 2021;359:129831. doi:10.1016/j.foodchem.2021.129831.
- Anhê FF, Roy D, Pilon G, Dudonné S, Matamoros S, Varin TV, et al. A polyphenol-rich cranberry extract protects from diet-induced obesity, insulin resistance and intestinal inflammation in association with increased akkermansia Spp. population in the Gut microbiota of mice. Gut. 2015;64(6):872–83. doi:10.1136/gutjnl-2014-307142.
- Pan P, Lam V, Salzman N, Huang Y-W, Yu J, Zhang J, Wang L-S. Black raspberries and their anthocyanin and fiber fractions alter the composition and diversity of Gut Microbiota in F-344 Rats. Nutr Cancer. 2017;69 (6):943–51. doi:10.1080/01635581.2017.1340491.
- Zhang L, Carmody RN, Kalariya HM, Duran RM, Moskal K, Poulev A, et al. Grape proanthocyanidin-induced intestinal bloom of Akkermansia Muciniphila Is dependent on its baseline abundance and precedes activation of host genes related to metabolic health. J Nutr Biochem. 2018;56:142–51. doi:10.1016/j.jnutbio.2018.02.009.
- Song H, Shen X, Deng R, Zhang Y, Zheng X. Dietary anthocyanin-rich extract of Açai protects from diet-induced obesity, liver steatosis, and insulin resistance with modulation of Gut microbiota in Mice. Nutrition. 2021;86:111176. doi:10.1016/j.nut.2021.111176.
- Nakano H, Wu S, Sakao K, Hara T, He J, Garcia S, et al. Bilberry anthocyanins ameliorate NAFLD by improving dyslipidemia and Gut microbiome dysbiosis. Nutrients. 2020;12(11):3252. doi:10.3390/nu12113252.
- Marques C, Fernandes I, Meireles M, Faria A, Spencer JPE, Mateus N, Calhau C. Gut microbiota modulation accounts for the neuroprotective properties of anthocyanins. Sci Rep. 2018;8(1):11341. doi:10.1038/s41598-018-29744-5.
- Si X, Bi J, Chen Q, Cui H, Bao Y, Tian J, et al. Effect of blueberry anthocyanin-rich extracts on peripheral and hippocampal antioxidant defensiveness: The analysis of the serum fatty acid species and Gut microbiota profile. J Agric Food Chem. 2021;69(12):3658–66. doi:10.1021/acs.jafc.0c07637.
- Hädrich G, Vaz GR, Maidana M, Kratz JM, Loch-Neckel G, Favarin DC, et al. Anti-Inflammatory effect and toxicology analysis of oral delivery Quercetin Nanosized emulsion in rats. Pharm Res. 2016;33(4):983–93. doi:10.1007/s11095-015-1844-6.
- Rogerio AP, Dora CL, Andrade EL, Chaves JS, Silva LFC, Lemos-Senna E, Calixto JB. Anti-Inflammatory effect of quercetin-loaded Microemulsion in the airways allergic inflammatory model in mice. Pharmacol Res. 2010;61(4):288–97. doi:10.1016/j.phrs.2009.10.005.
- Iova GM, Calniceanu H, Popa A, Szuhanek CA, Marcu O, Ciavoi G, Scrobota I. The antioxidant effect of curcumin and rutin on oxidative stress biomarkers in experimentally induced periodontitis in Hyperglycemic Wistar Rats. Molecules. 2021;26(5):1332. doi:10.3390/molecules26051332.
- Niture NT, Ansari AA, Naik SR. Anti-Hyperglycemic activity of rutin in streptozotocin-induced diabetic rats: an effect mediated through cytokines, antioxidants and lipid biomarkers. Indian J Exp Biol. 2014;52(7):720–7.
- Liu Z, Yao X, Sun B, Jiang W, Liao C, Dai X, et al. Pretreatment with Kaempferol attenuates Microglia-mediate neuroinflammation by inhibiting MAPKs–NF–ΚB signaling pathway and pyroptosis after secondary spinal cord injury. Free Radic Biol Med. 2021;168:142–54. doi:10.1016/j.freeradbiomed.2021.03.037.
- Li W-H, Cheng X, Yang Y-L, Liu M, Zhang S-S, Wang Y-H, et al. Kaempferol attenuates neuroinflammation and blood brain barrier dysfunction to improve neurological deficits in cerebral ischemia/reperfusion Rats. Brain Res. 2019;1722:146361. doi:10.1016/j.brainres.2019.146361.
- Wu Z, Huang S, Li T, Li N, Han D, Zhang B, et al. Gut Microbiota from green Tea polyphenol-dosed mice improves intestinal epithelial homeostasis and ameliorates experimental colitis. Microbiome. 2021;9(1):184. doi:10.1186/s40168-021-01115-9.
- Lee HA, Song YR, Park MH, Chung H, Na HS, Chung J. Catechin ameliorates porphyromonas Gingivalis- induced inflammation via the regulation of TLR2/4 and inflammasome signaling. J Periodontol. 2020;91(5):661–70. doi:10.1002/JPER.18-0004.
- Zhong X, Liu M, Yao W, Du K, He M, Jin X, et al. Epigallocatechin-3-gallate attenuates microglial inflammation and neurotoxicity by suppressing the activation of canonical and noncanonical inflammasome via TLR4/NF-κB pathway. Mol Nutr Food Res. 2019;63(21). doi:10.1002/mnfr.201801230.
- Chen X, Li H, Wang Z, Zhou Q, Chen S, Yang B, et al. Quercetin protects the vascular endothelium against iron overload damages via ROS/ADMA/DDAH II/ENOS/NO pathway. Eur J Pharmacol. 2020;868:172885. doi:10.1016/j.ejphar.2019.172885.
- Zaabalawi A, Astley C, Renshall L, Beards F, Lightfoot AP, Degens H, et al. Tetramethoxystilbene-Loaded liposomes restore reactive-oxygen-species-mediated attenuation of dilator responses in Rat aortic vessels Ex vivo. Molecules. 2019;24(23):4360. doi:10.3390/molecules24234360.
- Erlejman AG, Jaggers G, Fraga CG, Oteiza PI. TNFα-Induced NF-ΚB activation and cell oxidant production Are modulated by hexameric procyanidins in caco-2 cells. Arch Biochem Biophys. 2008;476(2):186–95. doi:10.1016/j.abb.2008.01.024.
- Dulebohn RV, Yi W, Srivastava A, Akoh CC, Krewer G, Fischer JG. Effects of blueberry (Vaccinium Ashei) on DNA damage, lipid peroxidation, and phase II enzyme activities in Rats. J Agric Food Chem. 2008;56(24):11700–6. doi:10.1021/jf802405y.
- Williamson G, Holst B. Dietary Reference Intake (DRI) value for dietary polyphenols: are we heading in the right direction? Br J Nutr. 2008;99(S3):S55–8. doi:10.1017/S0007114508006867.
- Del Bo’ C, Bernardi S, Marino M, Porrini M, Tucci M, Guglielmetti S, et al. Systematic review on polyphenol intake and health outcomes: is there sufficient evidence to define a health-promoting polyphenol-rich dietary pattern? Nutrients. 2019;11(6):1355. doi:10.3390/nu11061355.
- Castro-Barquero S, Lamuela-Raventós R, Doménech M, Estruch R. Relationship between Mediterranean dietary polyphenol intake and obesity. Nutrients. 2018;10(10):1523. doi:10.3390/nu10101523.
- McGarel C, Pentieva K, Strain JJ, McNulty H. Emerging roles for folate and related B-vitamins in brain health across the lifecycle. Proc Nutr Soc. 2015;74(1):46–55. doi:10.1017/S0029665114001554.
- Qin B, Plassman BL, Edwards LJ, Popkin BM, Adair LS, Mendez MA. Fish intake Is associated with slower cognitive decline in Chinese older adults. J Nutr. 2014;144(10):1579–85. doi:10.3945/jn.114.193854.
- Berendsen AM, Kang JH, Feskens EJM, de Groot CPGM, Grodstein F, van de Rest O. Association of long-term adherence to the mind diet with cognitive function and cognitive decline in American women. J Nutr Health Aging. 2018;22(2):222–9. doi:10.1007/s12603-017-0909-0.
- Cherian L, Wang Y, Fakuda K, Leurgans S, Aggarwal N, Morris M. Mediterranean-Dash intervention for neurodegenerative delay (mind) diet slows cognitive decline after stroke. J Prev Alzheimers Dis. 2019: 1–7. doi:10.14283/jpad.2019.28.
- Román GC, Jackson RE, Gadhia R, Román AN, Reis J. Mediterranean diet: The role of long-chain ω-3 fatty acids in fish; polyphenols in fruits, vegetables, cereals, coffee, Tea, cacao and wine; probiotics and vitamins in prevention of stroke, age-related cognitive decline, and Alzheimer disease. Rev Neurol (Paris). 2019;175(10):724–41. doi:10.1016/j.neurol.2019.08.005.
- Scarmeas N, Luchsinger JA, Mayeux R, Stern Y. Mediterranean diet and Alzheimer disease mortality. Neurology. 2007;69(11):1084–93. doi:10.1212/01.wnl.0000277320.50685.7c.
- Shah R. The role of nutrition and diet in Alzheimer disease: a systematic review. J Am Med Dir Assoc. 2013;14(6):398–402. doi:10.1016/j.jamda.2013.01.014.
- Lamport DJ, Saunders C, Butler LT, Spencer JP. Fruits, vegetables, 100% juices, and cognitive function. Nutr Rev. 2014;72(12):774–89. doi:10.1111/nure.12149.
- Kennedy DO. Polyphenols and the human brain: plant “secondary metabolite” ecologic roles and endogenous signaling functions drive benefits. Adv Nutr. 2014;5(5):515–33. doi:10.3945/an.114.006320.
- Rabassa M, Cherubini A, Zamora-Ros R, Urpi-Sarda M, Bandinelli S, Ferrucci L, Andres-Lacueva C. Low levels of a urinary biomarker of dietary polyphenol are associated with substantial cognitive decline over a 3-year period in older adults: the invecchiare in chianti study. J Am Geriatr Soc. 2015;63(5):938–46. doi:10.1111/jgs.13379.
- Shishtar E, Rogers GT, Blumberg JB, Au R, Jacques PF. Long-Term dietary flavonoid intake and risk of Alzheimer disease and related dementias in the Framingham Offspring Cohort. Am J Clin Nutr. 2020;112(2):343–53. doi:10.1093/ajcn/nqaa079.
- Janssen CIF, Jansen D, Mutsaers MPC, Dederen PJWC, Geenen B, Mulder MT, Kiliaan AJ. The effect of a high-Fat diet on brain plasticity, inflammation and cognition in female ApoE4-knockin and ApoE-knockout Mice. PLoS One. 2016;11(5):e0155307. doi:10.1371/journal.pone.0155307.
- Saiyasit N, Chunchai T, Apaijai N, Pratchayasakul W, Sripetchwandee J, Chattipakorn N, Chattipakorn SC. Chronic high-Fat diet consumption induces an alteration in plasma/brain neurotensin signaling, metabolic disturbance, systemic inflammation/oxidative stress, brain apoptosis, and dendritic spine loss. Neuropeptides. 2020;82:102047. doi:10.1016/j.npep.2020.102047.
- Pistell PJ, Morrison CD, Gupta S, Knight AG, Keller JN, Ingram DK, Bruce-Keller AJ. Cognitive impairment following high Fat diet consumption is associated with brain inflammation. J Neuroimmunol. 2010;219(1–2):25–32. doi:10.1016/j.jneuroim.2009.11.010.
- Lemprière S. New tools could improve Alzheimer disease diagnosis from structural MRI. Nat Rev Neurol. 2020;16(6):297. doi:10.1038/s41582-020-0357-z.
- Promteangtrong C, Kolber M, Ramchandra P, Moghbel M, Houshmand S, Schöll M, et al. Multimodality imaging approach in Alzheimer disease. Part I: structural MRI, functional MRI, diffusion tensor imaging and magnetization transfer imaging. Dement Neuropsychol. 2015;9(4):318–29. doi:10.1590/1980-57642015DN94000318.
- Long X, Chen L, Jiang C, Zhang L. Prediction and classification of Alzheimer disease based on quantification of MRI deformation. PLoS One. 2017;12(3):e0173372. doi:10.1371/journal.pone.0173372.
- Aguilar C, Muehlboeck J-S, Mecocci P, Vellas B, Tsolaki M, Kloszewska I, et al. Application of a MRI based index to longitudinal atrophy change in Alzheimer disease, mild cognitive impairment and healthy older individuals in the AddNeuroMed Cohort. Front Aging Neurosci. 2014: 6. doi:10.3389/fnagi.2014.00145.
- Cash DM, Ridgway GR, Liang Y, Ryan NS, Kinnunen KM, Yeatman T, et al. The pattern of atrophy in familial Alzheimer disease: volumetric MRI results from the DIAN study. Neurology. 2013;81(16):1425–33. doi:10.1212/WNL.0b013e3182a841c6.
- Lazarou I, Nikolopoulos S, Dimitriadis SI, (Yiannis) Kompatsiaris I, Spilioti M, Tsolaki M. Is brain connectome research the future frontier for subjective cognitive decline? A systematic review. Clin Neurophysiol. 2019;130(10):1762–80. doi:10.1016/j.clinph.2019.07.004.
- Guo H, Song X, Vandorpe R, Zhang Y, Chen W, Zhang N, et al. Evaluation of common structural brain changes in aging and Alzheimer disease with the Use of an MRI-based brain atrophy and lesion index: a comparison between T1WI and T2WI at 1.5 T and 3 T. Am J Neuroradiology. 2014;35(3):504–12. doi:10.3174/ajnr.A3709.
- Lieb W. Association of plasma leptin levels with incident Alzheimer disease and MRI measures of brain aging. JAMA. 2009;302(23):2565. doi:10.1001/jama.2009.1836.
- Tarroun A, Bonnefoy M, Bouffard-Vercelli J, Gedeon C, Vallee B, Cotton F. Could linear MRI measurements of hippocampus differentiate normal brain aging in elderly persons from Alzheimer disease? Surg Radiol Anat. 2007;29(1):77–81. doi:10.1007/s00276-006-0163-3.
- Shishtar E, Rogers GT, Blumberg JB, Au R, DeCarli C, Jacques PF. Flavonoid intake and MRI markers of brain health in the Framingham Offspring Cohort. J Nutr. 2020;150(6):1545–53. doi:10.1093/jn/nxaa068.
- Desideri G, Kwik-Uribe C, Grassi D, Necozione S, Ghiadoni L, Mastroiacovo D, et al. Benefits in cognitive function, blood pressure, and insulin resistance through cocoa flavanol consumption in elderly subjects with mild cognitive impairment. Hypertension. 2012;60(3):794–801. doi:10.1161/HYPERTENSIONAHA.112.193060.
- Brickman AM, Khan UA, Provenzano FA, Yeung L-K, Suzuki W, Schroeter H, et al. Enhancing dentate gyrus function with dietary flavanols improves cognition in older adults. Nat Neurosci. 2014;17(12):1798–803. doi:10.1038/nn.3850.
- Ghosh TS, Rampelli S, Jeffery IB, Santoro A, Neto M, Capri M, et al. Mediterranean diet intervention alters the Gut microbiome in older people reducing frailty and improving health status: the NU-AGE 1-year dietary intervention across five European countries. Gut. 2020;69(7):1218–28. doi:10.1136/gutjnl-2019-319654.
- Kuriyama S, Hozawa A, Ohmori K, Shimazu T, Matsui T, Ebihara S, et al. Green Tea consumption and cognitive function: a cross-sectional study from the Tsurugaya Project. Am J Clin Nutr. 2006;83(2):355–61. doi:10.1093/ajcn/83.2.355.
- Kesse-Guyot E, Fezeu L, Andreeva VA, Touvier M, Scalbert A, Hercberg S, Galan P. Total and specific polyphenol intakes in midlife Are associated with cognitive function measured 13 years Later3. J Nutr. 2012;142(1):76–83. doi:10.3945/jn.111.144428.
- Cheatham CL, Canipe LG, Millsap G, Stegall JM, Chai SC, Sheppard KW, Lila MA. Six-month intervention with wild blueberries improved speed of processing in mild cognitive decline: a double-blind, placebo-controlled, randomized clinical trial. Nutr Neurosci. 2023;26(10):1019–33. doi:10.1080/1028415X.2022.2117475.
- Yeh T-S, Yuan C, Ascherio A, Rosner BA, Willett WC, Blacker D. Long-Term dietary flavonoid intake and subjective cognitive decline in US men and women. Neurology. 2021;97(10):e1041–e1056. doi:10.1212/WNL.0000000000012454.
- Mastroiacovo D, Kwik-Uribe C, Grassi D, Necozione S, Raffaele A, Pistacchio L, et al. Cocoa flavanol consumption improves cognitive function, blood pressure control, and metabolic profile in elderly subjects: the Cocoa, Cognition, and Aging (CoCoA) study—a randomized controlled trial. Am J Clin Nutr. 2015;101(3):538–48. doi:10.3945/ajcn.114.092189.
- Márquez Campos E, Jakobs L, Simon M-C. Antidiabetic effects of flavan-3-Ols and their microbial metabolites. Nutrients. 2020;12(6):1592. doi:10.3390/nu12061592.
- Gratton G, Weaver SR, Burley CV, Low KA, Maclin EL, Johns PW, et al. Dietary flavanols improve cerebral cortical oxygenation and cognition in healthy adults. Sci Rep. 2020;10(1):19409. doi:10.1038/s41598-020-76160-9.
- Kim D, Hughes TM, Lipford ME, Craft S, Baker LD, Lockhart SN, et al. Relationship between cerebrovascular reactivity and cognition Among people with risk of cognitive decline. Front Physiol. 2021: 12. doi:10.3389/fphys.2021.645342.
- Lee CC, Kim JH, Kim JS, Oh YS, Han SM, Park JHY, et al. 5-(3′,4′-Dihydroxyphenyl-γ-Valerolactone), a major microbial metabolite of proanthocyanidin, attenuates THP-1 monocyte-endothelial adhesion. Int J Mol Sci. 2017;18(7):1363. doi:10.3390/ijms18071363.
- Baynham R, Veldhuijzen van Zanten JJCS, Johns PW, Pham QS, Rendeiro C. Cocoa flavanols improve vascular responses to acute mental stress in young healthy adults. Nutrients. 2021;13(4):1103. doi:10.3390/nu13041103.
- Hodgson JM, Croft KD. Dietary flavonoids: effects on endothelial function and blood pressure. J Sci Food Agric. 2006;86(15):2492–8. doi:10.1002/jsfa.2675.
- Egert S, Bosy-Westphal A, Seiberl J, Kürbitz C, Settler U, Plachta-Danielzik S, et al. Quercetin reduces systolic blood pressure and plasma oxidised Low-density lipoprotein concentrations in overweight subjects with a high-cardiovascular disease risk phenotype: a double-blinded, placebo-controlled cross-over study. Br J Nutr. 2009;102(7):1065–74. doi:10.1017/S0007114509359127.
- Zahedi M, Ghiasvand R, Feizi A, Asgari G, Darvish L. Does quercetin improve cardiovascular risk factors and inflammatory biomarkers in women with type 2 diabetes: a double-blind randomized controlled clinical trial. Int J Prev Med. 2013;4(7):777–85.
- Boots AW, Drent M, de Boer VCJ, Bast A, Haenen GRMM. Quercetin reduces markers of oxidative stress and inflammation in sarcoidosis. Clin Nutr. 2011;30(4):506–12. doi:10.1016/j.clnu.2011.01.010.
- Lee KS, Park SN. Cytoprotective effects and mechanisms of Quercetin, Quercitrin and Avicularin Isolated from Lespedeza Cuneata G. Don against ROS-induced cellular damage. J Ind Eng Chem. 2019;71:160–6. doi:10.1016/j.jiec.2018.11.018.
- Lei X, Chao H, Zhang Z, Lv J, Li S, Wei H, et al. Neuroprotective effects of quercetin in a mouse model of brain ischemic/reperfusion injury via anti-apoptotic mechanisms based on the Akt pathway. Mol Med Rep. 2015;12(3):3688–96. doi:10.3892/mmr.2015.3857.
- Nishihira J, Nishimura M, Kurimoto M, Kagami-Katsuyama H, Hattori H, Nakagawa T, et al. The effect of 24-week continuous intake of quercetin-rich onion on Age-related cognitive decline in healthy elderly people: a randomized, double-blind, placebo-controlled, parallel-group comparative clinical trial. J Clin Biochem Nutr. 2021;69(2):21–17. doi:10.3164/jcbn.21-17.
- Fairlie-Jones L, Davison K, Fromentin E, Hill A. The effect of anthocyanin-rich foods or extracts on vascular function in adults: a systematic review and meta-analysis of randomised controlled trials. Nutrients. 2017;9(8):908. doi:10.3390/nu9080908.
- Mattioli R, Francioso A, Mosca L, Silva P. Anthocyanins: a comprehensive review of their chemical properties and health effects on cardiovascular and neurodegenerative diseases. Molecules. 2020;25(17):3809. doi:10.3390/molecules25173809.
- Zhu Y, Ling W, Guo H, Song F, Ye Q, Zou T, et al. Anti-Inflammatory effect of purified dietary anthocyanin in adults with hypercholesterolemia: a randomized controlled trial. Nutr, Metab Cardiovasc Dis. 2013;23(9):843–9. doi:10.1016/j.numecd.2012.06.005.
- Kent K, Charlton K, Roodenrys S, Batterham M, Potter J, Traynor V, et al. Consumption of anthocyanin-rich cherry juice for 12 weeks improves memory and cognition in older adults with mild-to-moderate dementia. Eur J Nutr. 2017;56(1):333–41. doi:10.1007/s00394-015-1083-y.
- Travica N, D’Cunha NM, Naumovski N, Kent K, Mellor DD, Firth J, et al. The effect of blueberry interventions on cognitive performance and mood: a systematic review of randomized controlled trials. Brain Behav Immun. 2020;85:96–105. doi:10.1016/j.bbi.2019.04.001.
- Whyte A, Cheng N, Fromentin E, Williams C. A randomized, double-blinded, placebo-controlled study to compare the safety and efficacy of Low dose enhanced wild blueberry powder and wild blueberry extract (ThinkBlueTM) in maintenance of episodic and working memory in older adults. Nutrients. 2018;10(6):660. doi:10.3390/nu10060660.
- Krikorian R, Kalt W, McDonald JE, Shidler MD, Summer SS, Stein AL. Cognitive performance in relation to urinary anthocyanins and their flavonoid-based products following blueberry supplementation in older adults at risk for dementia. J Funct Foods. 2020;64:103667. doi:10.1016/j.jff.2019.103667.
- Boespflug EL, Eliassen JC, Dudley JA, Shidler MD, Kalt W, Summer SS, et al. Enhanced neural activation with blueberry supplementation in mild cognitive impairment. Nutr Neurosci. 2018;21(4):297–305. doi:10.1080/1028415X.2017.1287833.
- Cheatham C, Vazquez-Vidal I, Medlin A, Voruganti V. Blueberry consumption affects serum uric acid concentrations in older adults in a Sex-specific manner. Antioxidants. 2016;5(4):43. doi:10.3390/antiox5040043.
- Khan N, Mukhtar H. Tea polyphenols in promotion of human health. Nutrients. 2018;11(1):39. doi:10.3390/nu11010039.
- Ide K, Yamada H, Takuma N, Park M, Wakamiya N, Nakase J, et al. Green Tea consumption affects cognitive dysfunction in the elderly: a pilot study. Nutrients. 2014;6(10):4032–42. doi:10.3390/nu6104032.
- Khalesi S, Sun J, Buys N, Jamshidi A, Nikbakht-Nasrabadi E, Khosravi-Boroujeni H. Green Tea catechins and blood pressure: a systematic review and meta-analysis of randomised controlled trials. Eur J Nutr. 2014;53(6):1299–311. doi:10.1007/s00394-014-0720-1.
- Arcambal A, Taïlé J, Couret D, Planesse C, Veeren B, Diotel N, et al. Protective effects of antioxidant polyphenols against hyperglycemia-mediated alterations in cerebral endothelial cells and a mouse stroke model. Mol Nutr Food Res. 2020;64(13). doi:10.1002/mnfr.201900779.
- Bariexca T, Ezdebski J, Redan B, Vinson J. Pure polyphenols and cranberry juice high in anthocyanins increase antioxidant capacity in animal organs. Foods. 2019;8(8):340. doi:10.3390/foods8080340.
- Campos-Esparza MR, Sánchez-Gómez MV, Matute C. Molecular mechanisms of neuroprotection by Two natural antioxidant polyphenols. Cell Calcium. 2009;45(4):358–68. doi:10.1016/j.ceca.2008.12.007.
- Singh M, Arseneault M, Sanderson T, Murthy V, Ramassamy C. Challenges for research on polyphenols from foods in Alzheimer’s disease: bioavailability, metabolism, and cellular and molecular mechanisms. J Agric Food Chem. 2008;56(13):4855–73. doi:10.1021/jf0735073.
- Dueñas M, Muñoz-González I, Cueva C, Jiménez-Girón A, Sánchez-Patán F, Santos-Buelga C, et al. A survey of modulation of Gut microbiota by dietary polyphenols. Biomed Res Int. 2015;2015:1–15. doi:10.1155/2015/850902.
- Corrêa TAF, Rogero MM, Hassimotto NMA, Lajolo FM. The Two-Way polyphenols-microbiota interactions and their effects on obesity and related metabolic diseases. Front Nutr. 2019: 6. doi:10.3389/fnut.2019.00188.
- Cortés-Martín A, Selma MV, Tomás-Barberán FA, González-Sarrías A, Espín JC. Where to look into the puzzle of polyphenols and health? The postbiotics and Gut microbiota associated with human metabotypes. Mol Nutr Food Res. 2020;64(9). doi:10.1002/mnfr.201900952.
- Selma MV, González-Sarrías A, Salas-Salvadó J, Andrés-Lacueva C, Alasalvar C, Örem A, et al. The Gut microbiota metabolism of pomegranate or walnut ellagitannins yields two urolithin-metabotypes that correlate with cardiometabolic risk biomarkers: comparison between normoweight, overweight-obesity and metabolic syndrome. Clin Nutr. 2018;37(3):897–905. doi:10.1016/j.clnu.2017.03.012.
- Barroso E, Muñoz-González I, Jiménez E, Bartolomé B, Moreno-Arribas MV, Peláez C, et al. Phylogenetic profile of Gut microbiota in healthy adults after moderate intake of Red wine. Mol Nutr Food Res. 2017;61(3). doi:10.1002/mnfr.201600620.
- Ma G, Chen Y. Polyphenol supplementation benefits human health via Gut microbiota: a systematic review via meta-analysis. J Funct Foods. 2020;66:103829. doi:10.1016/j.jff.2020.103829.
- Cheatham CL, Nieman DC, Neilson AP, Lila MA. Enhancing the cognitive effects of flavonoids with physical activity: is there a case for the Gut microbiome? Front Neurosci. 2022;16; doi:10.3389/fnins.2022.833202.
- Reddy VP, Aryal P, Robinson S, Rafiu R, Obrenovich M, Perry G. Polyphenols in Alzheimer’s disease and in the Gut–Brain axis. Microorganisms. 2020;8(2):199. doi:10.3390/microorganisms8020199.
- Cryan JF, O’Riordan KJ, Sandhu K, Peterson V, Dinan TG. The Gut microbiome in neurological disorders. Lancet Neurol. 2020;19(2):179–94. doi:10.1016/S1474-4422(19)30356-4.
- Dinan TG, Cryan JF. Gut instincts: microbiota as a Key regulator of brain development, ageing and neurodegeneration. J Physiol. 2017;595(2):489–503. doi:10.1113/JP273106.
- Sun M, Ma K, Wen J, Wang G, Zhang C, Li Q, et al. A review of the brain-Gut-microbiome axis and the potential role of microbiota in Alzheimer’s disease. J Alzheimer’s Dis. 2020;73(3):849–65. doi:10.3233/JAD-190872.
- Bonfili L, Cecarini V, Gogoi O, Gong C, Cuccioloni M, Angeletti M, et al. Microbiota modulation as preventative and therapeutic approach in Alzheimer’s disease. FEBS J. 2021;288(9):2836–55. doi:10.1111/febs.15571.
- Mayer EA, Knight R, Mazmanian SK, Cryan JF, Tillisch K. Gut microbes and the brain: paradigm shift in neuroscience. J Neurosci. 2014;34(46):15490–6. doi:10.1523/JNEUROSCI.3299-14.2014.
- Canipe LG, Sioda M, Cheatham CL. Diversity of the Gut-microbiome related to cognitive behavioral outcomes in healthy older adults. Arch Gerontol Geriatr. 2021;96:104464. doi:10.1016/j.archger.2021.104464.
- Boehme M, van de Wouw M, Bastiaanssen TFS, Olavarría-Ramírez L, Lyons K, Fouhy F, et al. Mid-Life microbiota crises: middle Age Is associated with pervasive neuroimmune alterations that Are reversed by targeting the Gut microbiome. Mol Psychiatry. 2020;25(10):2567–83. doi:10.1038/s41380-019-0425-1.
- Cryan JF, Boehme M, Dinan TG. Is the fountain of youth in the Gut microbiome? J Physiol. 2019;597(9):2323–4. doi:10.1113/JP277784.
- Scott KA, Ida M, Peterson VL, Prenderville JA, Moloney GM, Izumo T, et al. Revisiting Metchnikoff: age-related alterations in microbiota-Gut-Brain axis in the mouse. Brain Behav Immun. 2017;65:20–32. doi:10.1016/j.bbi.2017.02.004.
- Donoso F, Egerton S, Bastiaanssen TFS, Fitzgerald P, Gite S, Fouhy F, et al. Polyphenols selectively reverse early-life stress-induced behavioural, neurochemical and microbiota changes in the Rat. Psychoneuroendocrinology. 2020;116:104673. doi:10.1016/j.psyneuen.2020.104673.
- Ballesteros-Álvarez J, Nguyen W, Sivapatham R, Rane A, Andersen JK. Urolithin A reduces amyloid-beta load and improves cognitive deficits uncorrelated with plaque burden in a mouse model of Alzheimer’s disease. Geroscience. 2023;45(2):1095–113. doi:10.1007/s11357-022-00708-y.
- Sekikawa A, Wharton W, Butts B, Veliky CV, Garfein J, Li J, et al. Potential protective mechanisms of S-equol, a metabolite of Soy isoflavone by the Gut microbiome, on cognitive decline and dementia. Int J Mol Sci. 2022;23(19):11921. doi:10.3390/ijms231911921.
- Yeh TS, Yuan C, Ascherio A, Rosner BA, Willett WC, Blacker D. Long-Term dietary flavonoid intake and subjective cognitive decline in US Men and women. Neurology. 2021;97(10):e1041–56. doi:10.1212/WNL.0000000000012454.
- Sesso HD, Manson JE, Aragaki AK, Rist PM, Johnson LG, Friedenberg G, et al. Effect of Cocoa Flavanol supplementation for the prevention of cardiovascular disease events: The COcoa supplement and multivitamin outcomes study (COSMOS) randomized clinical trial. Am J Clin Nutr. 2022;115(6):1490–500. doi:10.1093/ajcn/nqac055.